Chickpea (Cicer arietinum L.), also called garbanzo bean or Bengal gram, is an Old-World pulse and one of the seven Neolithic founder crops in the Fertile Crescent of the Near East(Reference Lev-Yadun, Gopher and Abbo1). Currently, chickpea is grown in over fifty countries across the Indian subcontinent, North Africa, the Middle East, southern Europe, the Americas and Australia. Globally, chickpea is the third most important pulse crop in production, next to dry beans and field peas(2). During 2006–9, the global chickpea production area was about 11·3 million ha, with a production of 9·6 million metric tonnes and an average yield of 849 kg/ha(2). India is the largest chickpea-producing country with an average production of 6·38 million metric tonnes during 2006–9, accounting for 66 % of global chickpea production(2). The other major chickpea-producing countries include Pakistan, Turkey, Australia, Myanmar, Ethiopia, Iran, Mexico, Canada and the USA.
There are two distinct types of cultivated chickpea: Desi and Kabuli. The Desi (microsperma) types have pink flowers, anthocyanin pigmentation on stems, and a coloured and thick seed coat. The Kabuli (macrosperma) types have white flowers, lack anthocyanin pigmentation on stems, and have white or beige-coloured seeds with a ram's head shape, a thin seed coat and a smooth seed surface(Reference Moreno and Cubero3). In addition, an intermediate type with pea-shaped seeds of local importance is recognised in India. The seed weight generally ranges from 0·1 to 0·3 g and 0·2 to 0·6 g in the Desi and Kabuli types, respectively(Reference Frimpong, Sinha and Tar'an4). The Desi types account for about 80–85 % of the total chickpea area and are mostly grown in Asia and Africa(Reference Pande, Siddique and Kishore5). The Kabuli types are largely grown in West Asia, North Africa, North America and Europe.
There is a growing demand for chickpea due to its nutritional value. In the semi-arid tropics, chickpea is an important component of the diets of those individuals who cannot afford animal proteins or those who are vegetarian by choice. Chickpea is a good source of carbohydrates and protein, together constituting about 80 % of the total dry seed mass(Reference Chibbar, Ambigaipalan and Hoover6, Reference Geervani7) in comparison with other pulses. Chickpea is cholesterol free and is a good source of dietary fibre (DF), vitamins and minerals(8, Reference Wood, Grusak, Yadav, Redden, Chen and Sharma9).
Globally, chickpea is mostly consumed as a seed food in several different forms and preparations are determined by ethnic and regional factors(Reference Muehlbauer and Tullu10, Reference Ibrikci, Knewtson and Grusak11). In the Indian subcontinent, chickpea is split (cotyledons) as ‘dhal’ and ground to make flour (‘besan’) that is used to prepare different snacks(Reference Chavan, Kadam and Salunkhe12, Reference Hulse13). In other parts of the world, especially in Asia and Africa, chickpea is used in stews and soups/salads, and consumed in roasted, boiled, salted and fermented forms(Reference Gecit14). These different forms of consumption provide consumers with valuable nutritional and potential health benefits.
Although chickpea is a member of the ‘founder crop package’(Reference Zohary and Hopf15) with potential nutritional/medicinal qualities, it has not received due attention for research like other founder crops (e.g. wheat or barley). Chickpea has been consumed by humans since ancient times owing to its good nutritional properties. Furthermore, chickpea is of interest as a functional food with potential beneficial effects on human health. Although other publications have described the physico-chemical and nutritional characteristics of chickpea, there is limited information on the relationship between its nutritional components and health benefits. This review attempts to bridge this void and investigates the literature regarding the nutritional value of chickpeas and their potential health benefits.
Chickpea grain composition
Classification of carbohydrates
Dietary carbohydrates are classified into two groups: (1) available (mono- and disaccharides), which are enzymatically digested in the small intestine, and (2) unavailable (oligosaccharides, resistant starch, non-cellulosic polysaccharides, pectins, hemicelluloses and cellulose), which are not digested in the small intestine(Reference Chibbar, Baga, Ganeshan, Wrigley, Corke and Walker16). The total carbohydrate content in chickpea is higher than that in pulses (Table 2). Chickpea contains monosaccharides (ribose, glucose, galactose and fructose), disaccharides (sucrose and maltose) and oligosaccharides (stachyose, ciceritol, raffinose and verbascose) (Table 1). The amount of these fractions varies, though not significantly, between the Desi and Kabuli genotypes (Table 1).
Table 1 Different carbohydrate fractions in chickpea seeds

K, Kabuli; D, Desi.
* Expressed as g/100 g dry weight. Numbers in parentheses indicate range.
† Expressed as mg/g.
‡ Expressed as a percentage of the dry weight of raw seed.
§ The type of chickpea is not specified.
∥ Expressed as g/kg.
Mono-, di- and oligosaccharides
Sánchez-Mata et al. (Reference Sánchez-Mata, Peñuela-Teruel and Cámara-Hurtado17) reported the following monosaccharide concentrations in chickpea: galactose, 0·05 g/100 g; ribose, 0·11 g/100 g; fructose, 0·25 g/100 g; glucose, 0·7 g/100 g. Maltose (0·6 %) and sucrose (1–2 %) have been reported to be the most abundant free disaccharides in chickpea(Reference Wood, Grusak, Yadav, Redden, Chen and Sharma9). Pulse seeds contain some of the highest concentrations of oligosaccharides among all the crops. Oligosaccharides are not absorbed or hydrolysed by the human digestive system but fermented by colonic bacteria to release gases or flatulence(Reference Kozlowska, Aranda and Dostalova18). α-Galactosides are the second most abundant carbohydrates in the plant kingdom after sucrose(Reference Jones, DuPont and Ambrose19, Reference Han and Baik20), and in chickpea, they account for about 62 % of total sugar (mono-, di- and oligosaccharides) content(Reference Sánchez-Mata, Peñuela-Teruel and Cámara-Hurtado17). The two important groups of α-galactosides present in chickpea are as follows: (1) raffinose family of oligosaccharides, including raffinose (trisaccharide), stachyose (tetrasaccharide) and verbascose (pentasaccharide)(Reference Han and Baik20), and (2) galactosyl cyclitols, including ciceritol (Table 1)(Reference Bernabé, Fenwick and Frias21). Ciceritol was isolated for the first time from chickpea seeds by Quemener & Brillouet(Reference Quemener and Brillouet22) and later confirmed by Bernabé et al. (Reference Bernabé, Fenwick and Frias21). Ciceritol and stachyose, two important galactosides in chickpea, constitute 36–43 % and 25 %, respectively, of total sugars (mono-, di- and oligosaccharides) in chickpea seeds(Reference Sánchez-Mata, Peñuela-Teruel and Cámara-Hurtado17, Reference Aguilera, Martín-Cabrejas and Benítez23).
α-Galactosides are neither absorbed nor hydrolysed in the upper gastrointestinal tract of humans, accumulating in the large intestine of the human digestive system. Humans lack α-galactosidase, the enzyme responsible for degrading these oligosaccharides(Reference Han and Baik20). Therefore, α-galactosides undergo microbial fermentation by colonic bacteria resulting in the production of hydrogen, methane and CO2, major components of flatulent gases(Reference Singh24). The expulsion of these gases is responsible for abdominal discomfort. Gas production is higher following chickpea consumption compared with other pulses, and this could be due to a higher content of oligosaccharides in chickpea(Reference Jaya, Naik and Venkataraman25, Reference Rao and Belavady26). Germination decreases the raffinose, stachyose and verbascose content(Reference Åman27). Chickpea has lower values for the absolute content of flatulent α-galactosides (1·56 g/100 g) compared with other pulses such as white beans (2·46 g/100 g), lentils (2·44 g/100 g) and pinto beans (2·30 g/100 g)(Reference Sánchez-Mata, Peñuela-Teruel and Cámara-Hurtado17).
Polysaccharides
Polysaccharides are high-molecular-weight polymers of monosaccharides present as storage carbohydrates (e.g. starch) or structural carbohydrates (e.g. cellulose) providing structural support(Reference Wood, Grusak, Yadav, Redden, Chen and Sharma9). Among the storage polysaccharides, chickpea has been reported to synthesise and store starch and not galactomannans(Reference Wood, Grusak, Yadav, Redden, Chen and Sharma9). Starch is the major storage carbon reserve in pulse seeds(Reference Chibbar, Ambigaipalan and Hoover6). It is made up of two large glucan polymers, amylose and amylopectin, in which the glucose residues are linked by α-(1 → 4) bonds to form a linear molecule and the linear molecule is branched by α-(1 → 6) linkages(Reference Chibbar, Ambigaipalan and Hoover6). The side chains of amylopectin are packed into different polymorphic forms in the lamellae of starch grains: ‘A’ type in cereals and ‘C’ type in pulses. The ‘C’ polymorph is considered to be of the intermediate type between the ‘A’ polymorph in cereals and the ‘B’ polymorph in tubers in packing density and structure(Reference Chibbar, Ambigaipalan and Hoover6). The content of starch varies from 41 to 50 % of the total carbohydrates(Reference Jambunathan and Singh28–Reference Özer, Karaköy and Toklu30), with the Kabuli types having more soluble sugars (sucrose, glucose and fructose) compared with the Desi types(Reference Jambunathan and Singh28). The total starch content of chickpea seeds has been reported to be about 525 g/kg DM, about 35 % of total starch has been considered to be resistant starch and the remaining 65 % as available starch(Reference Aguilera, Martín-Cabrejas and Benítez23, Reference Aguilera, Esteban and Benítez31). Cereals such as wheat have a higher amount of starch compared with chickpea(32), but chickpea seeds have a higher amylose content (30–40 v. 25 % in wheat)(Reference Williams, Singh, Saxena and Singh33, Reference Guillon and Champ34). In vitro starch digestibility values (ISDV) of chickpea vary from 37 to 60 %(Reference Zia-Ul-Haq, Iqbal and Ahmad35, Reference Khalil, Zeb and Mahood36) and are higher than other pulses such black grams, lentils and kidney beans(Reference Rehman and Shah37). However, the in vitro starch digestibility values of pulses, in general, are lower than cereals due to a higher amylose content(Reference Madhusudhan and Tharanathan38).
Dietary fibre
DF is the indigestible part of plant food in the human small intestine. It is composed of poly/oligosaccharides, lignin and other plant-based substances(39). DF can be classified into soluble and insoluble fibres. The soluble fibre is digested slowly in the colon, whereas the insoluble fibre is metabolically inert and aids in bowel movement(Reference Tosh and Yada40). The insoluble fibre undergoes fermentation aiding in the growth of colonic bacteria(Reference Tosh and Yada40). The total DF content (DFC) in chickpea is 18–22 g/100 g of raw chickpea seed(Reference Aguilera, Martín-Cabrejas and Benítez23, Reference Tosh and Yada40), and it has a higher amount of DF among pulses (Table 2). Soluble and insoluble DFC are about 4–8 and 10–18 g/100 g of raw chickpea seed, respectively(Reference Dalgetty and Baik29, Reference Rincón, Martínez and Ibáñez41). The fibre content of chickpea hulls on a dry weight basis is lower (75 %) compared with lentils (87 %) and peas (89 %)(Reference Dalgetty and Baik29). The lower DFC in chickpea hulls can be attributed to the difficulty in separating the hull from the cotyledon during milling.
The DFC of chickpea seeds is equal to or higher than that of other pulses such as lentils (Lens culinaris) and dry peas (Pisum sativum)(Reference Tosh and Yada40). The Desi types have a higher total DFC and insoluble DFC compared with the Kabuli types. This could be due to thicker hulls and seed coat in the Desi types (11·5 % of total seed weight) compared with the Kabuli types (only 4·3–4·4 % of total seed weight)(Reference Rincón, Martínez and Ibáñez41). Further, Wood et al. (Reference Wood, Knights and Choct42) have reported that the thinner seed coat in the Kabuli types is due to thinner palisade and parenchyma layers with fewer polysaccharides. Usually, no significant differences are found in soluble DFC between the Kabuli and Desi types due to the similar proportion of hemicelluloses that constitute a large part (about 55 %) of the total seed DF in the Kabuli and Desi types(Reference Singh43). The hemicellulosic sugar arabinose/rhamnose is present in appreciable amounts in hull and insoluble fibre fractions of chickpea(Reference Dalgetty and Baik29). Glucose is present in large amounts in hull and soluble fibre fractions of chickpea. Xylose is the major constituent of soluble fibre fractions in chickpea(Reference Dalgetty and Baik29).
Protein content
Protein–energy malnutrition is observed in infants and young children in developing countries, and includes a range of pathological conditions arising due to the lack of protein and energy in the diet(Reference Haider and Haider44). Malnutrition affects about 170 million people, especially preschool children and nursing mothers of developing countries in Asia and Africa(Reference Iqbal, Khalil and Ateeq45). Pulses provide a major share of protein and energy in the Afro-Asian diet. Among the different pulses, chickpea has been reported to have a higher protein bioavailability(Reference Yust, Pedroche and Giron-Calle46, Reference Sánchez-Vioque, Clemente and Vioque47).
The protein content in chickpea significantly varies as a percentage of the total dry seed mass before (17–22 %) and after (25·3–28·9 %) dehulling(Reference Hulse13, Reference Badshah, Khan and Bibi48). The differences in the crude protein concentration of Kabuli and Desi types have been inconsistent, showing significant differences in one instance (241 g/kg in ‘Kabuli’ v. 217 g/kg in ‘Desi’)(Reference Singh and Jambunathan49) and no differences in another instance (217 g/kg in ‘Kabuli’ v. 215 g/kg in ‘Desi’)(Reference Rincón, Martínez and Ibáñez41). The seed protein content of eight annual wild species of the genus Cicer ranged from 168 g/kg in Cicer cuneatum to 268 g/kg in Cicer pinnatifidum, with an average of 207 g/kg over the eight wild species(Reference Ocampo, Robertson and Singh50). Chickpea protein quality is better than some pulse crops such as black gram (Vigna mungo L.), green gram (Vigna radiata L.) and red gram (Cajanus cajan L.)(Reference Kaur, Singh and Sodhi51). Additionally, there is no significant difference in the protein concentration of raw chickpea seeds compared with some pulses such as black gram, lentils, red kidney bean and white kidney bean(Reference Rehman and Shah37).
Protein digestibility
The in vitro protein digestibility of raw chickpea seeds varies from 34 to 76 %(Reference Khalil, Zeb and Mahood36, Reference Khattak, Zeb and Bibi52, Reference Clemente, Sánchez-Vioque and Vioque53). Chitra et al. (Reference Chitra, Vimala and Singh54) found higher in vitro protein digestibility values for chickpea genotypes (65·3–79·4 %) compared with those for pigeon pea (C. cajan; 60·4–74·4 %), mung bean (V. radiata; 67·2–72·2 %), urd bean (V. mungo; 55·7–63·3 %) and soyabean (Glycine max; 62·7–71·6 %). The digestibility of protein from the Kabuli types is higher than that from the Desi types(Reference Sánchez-Vioque, Clemente and Vioque47, Reference Paredes-López, Ordorica-Falomir and Olivares-Vázquez55).
Amino acid profile
The amino acid profiles of chickpea seeds are presented in Table 3. There are some minor variations in the quantity of a few amino acids such as lysine, tyrosine, glutamic acid, histidine and the two combined aromatic amino acids (Table 3)(Reference Iqbal, Khalil and Ateeq45). Generally, sulphur-rich amino acids (methionine and cystine) are limiting in pulses. Commonly consumed food pulses such as chickpea, field pea, green pea, lentils and common beans have about 1·10 g/16 g N of methionine and cystine(Reference Wang and Daun56), the exceptions being cowpea, which has about 2·20 g/16 g N of methionine, and green pea, which has about 1·80 g/16 g N of cystine(Reference Iqbal, Khalil and Ateeq45). There are no significant differences in the amino acid profiles of Kabuli- and Desi-type chickpeas(Reference Wang and Daun56, Reference Wang, Gao and Zhang57). Amino acid deficiencies in chickpea (or other pulses) could be complemented by consuming cereals, which are rich in sulphur-containing amino acids(Reference Zia-Ul-Haq, Iqbal and Ahmad35). Pulses are usually consumed along with cereals, especially in Asian countries, thereby allowing the daily dietary amino acid requirements to be met.
Table 3 Amino acid content in chickpea seeds

K, Kabuli; D, Desi; N/D, not determined.
* Expressed as g/16 g N.
† The type of chickpea is not specified.
‡ Expressed as g/100 g.
§ Expressed as mg/g protein.
Fat content and fatty acid profile
The total fat content in raw chickpea seeds varies from 2·70 to 6·48 %(Reference Kaur, Singh and Sodhi51, Reference Alajaji and El-Adawy58). Shad et al. (Reference Shad, Pervez and Zafar59) reported lower values (about 2·05 g/100 g) for crude fat content in Desi-type chickpea varieties. Wood & Grusak(Reference Wood, Grusak, Yadav, Redden, Chen and Sharma9) reported a fat content of 3·40–8·83 and 2·90–7·42 % in Kabuli- and Desi-type chickpea seeds, respectively. Further, even higher levels (3·80–10·20 %) of fat content in chickpea have been reported(Reference Singh24). The fat content in chickpea (6·04 g/100 g) is higher than that in other pulses such as lentils (1·06 g/100 g), red kidney bean (1·06 g/100 g), mung bean (1·15 g/100 g) and pigeon pea (1·64 g/100 g), and also in cereals such as wheat (1·70 g/100 g) and rice (about 0·60 g/100 g)(32). Chickpea is composed of about 66 % PUFA, about 19 % MUFA and about 15 % SFA (Table 4). On average, oleic acid (OA) was higher in the Kabuli types and linoleic acid (LA) was higher in the Desi types (Table 4). Chickpea is a relatively good source of nutritionally important PUFA, LA (51·2 %) and monounsaturated OA (32·6 %). Chickpea has higher amounts of LA and OA compared with other edible pulses such as lentils (44·4 % LA; 20·9 % OA), peas (45·6 % LA; 23·2 % OA) and beans (46·7 % LA; 28·1 % OA)(Reference Wang and Daun56). LA is the dominant fatty acid in chickpea followed by OA and palmitic acid (Table 4).
Table 4 Fatty acid profiles of chickpea seeds

K, Kabuli; D, Desi; USDA, United States Department of Agriculture; ND, measured but not detected.
* Expressed as percentage of oil.
† The type of chickpea is not specified.
‡ Expressed as g/100 g.
§ Expressed as wt % of total elute.
Oil characteristics
Chickpea cannot be considered as an oilseed crop since its oil content is relatively low (3·8–10 %)(Reference Singh24, Reference Gül, Ömer and Turhan60) in comparison with other important oilseed pulses such as soyabean or groundnut. However, chickpea oil has medicinal and nutritionally important tocopherols, sterols and tocotrienols(Reference Zia-Ul-Haq, Ahmad and Iqbal61). The content of different sterols and tocopherols in chickpea is presented in Table 5. Sitosterol (72·52–76·10 %; Table 5) is the dominant sterol in chickpea oil followed by campesterol. The α-tocopherol content reported by the United States Department of Agriculture(32) is lower than the other reported values in Table 5. However, the α-tocopherol content in chickpea is relatively higher (8·2 mg/100 g) than other pulses such as lentils (4·9 mg/100 g), green pea (1·3 mg/100 g), red kidney bean (2·1 mg/100 g) and mung bean (5·1 mg/100 g)(32). The α-tocopherol content, coupled with the concentration of δ-tocopherol, which is a potent antioxidant property(Reference Tsaknis62), makes chickpea oil oxidatively stable and contributes to a better shelf life during storage(Reference Zia-ul-Haq, Ahmad and Ahmad63). TAG is the predominant neutral lipid in Desi chickpea oil and phospholipids are also found in oil(Reference Zia-Ul-Haq, Ahmad and Iqbal61).
Table 5 Important sterols and tocopherols in oil from chickpea seeds
(Mean values and standard deviations)
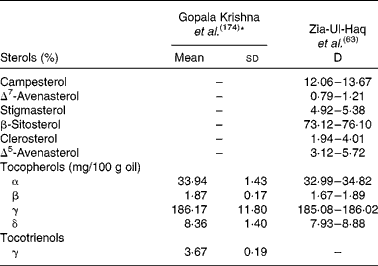
D, Desi.
* The type of chickpea is not specified.
The physico-chemical characteristics of chickpea oil are summarised in Table 6. The relative index values of chickpea (1·49) are higher than those of soyabean (1·46) and groundnut (1·47), the two important oil-bearing pulses(Reference Kirk and Sawyer64). The iodine values of chickpea oil (111·87–113·69, Wijs method) were also higher than the iodine values of groundnut (80–106, Wijs method) and Phaseolus vulgaris (80·5–92·3, Wijs method)(Reference Zia-Ul-Haq, Ahmad and Iqbal61, Reference Mabaleha and Yeboah65). Higher refractive index and iodine values indicate substantial unsaturation in chickpea oil, which is demonstrated by the dominance of LA content(Reference Zia-Ul-Haq, Ahmad and Iqbal61) (Table 4). The lower acid values observed for chickpea (Table 6) make its oil refining easier(Reference Siddhuraju, Becker and Makkar66). The peroxide value for chickpea oils (3·97–6·37 mequiv/kg; Table 6) was within the maximum limit of the Codex recommendation (10 mequiv/kg) for edible oils(Reference Kirk and Sawyer64).
Table 6 Physical and chemical characteristics of chickpea seed oil

D, Desi; MAG, monoacylglycerols; DAG, diacylglycerols.
Minerals
Chickpea, like other pulses, not only brings variety to the cereal-based daily diet of millions of people in Asia and Africa, but also provides essential vitamins and minerals(Reference Cabrera, Lloris and Giménez67, Reference Duhan, Khetarpaul and Bishnoi68). The different minerals present in chickpea seeds are presented in Table 7. Raw chickpea seeds (100 g) on an average provide about 5·0 mg/100 g of Fe, 4·1 mg/100 g of Zn, 138 mg/100 g of Mg and 160 mg/100 g of Ca. About 100 g of chickpea seeds can meet the daily dietary requirements of Fe (1·05 mg/d in males and 1·46 mg/d in females) and Zn (4·2 mg/d in males and 3·0 mg/d in females) and 200 g can meet that of Mg (260 mg/d in males and 220 mg/d in females)(69). There were no significant differences between the Kabuli and Desi genotypes except for Ca, with the Desi types having a higher content than the Kabuli types(Reference Wang and Daun56, Reference Ibáñez, Rinch and Amaro70). The amount of total Fe present in chickpea is lower (5·45 mg/100 g) compared with other pulse crops such as lentils (8·60 mg/100 g) and beans (7·48 mg/100 g)(Reference Quinteros, Farre and Lagarda71). Data on other minerals present in chickpea are very limited. Se, a nutritionally important essential trace element, is also found in chickpea seeds (8·2 μg/100 g)(32, Reference Cabrera, Lloris and Giménez67). Chickpea has been reported to have other trace elements including Al (10·2 μg/g), Cr (0·12 μg/g), Ni (0·26 μg/g), Pb (0·48 μg/g) and Cd (0·01 μg/g)(32, Reference Cabrera, Lloris and Giménez67). The quantities reported here for Al, Ni, Pb and Cd do not pose any toxicological risk.
Table 7 Mineral constituents (mg/100 g) of chickpea seeds

D, Desi; K, Kabuli; USDA, United States Department of Agriculture.
† Expressed as μg/g.
* The type of chickpea is not specified.
Vitamins
Vitamins are required in tiny quantities; this requirement is met through a well-balanced daily diet of cereals, pulses, vegetables, fruits, meat and dairy products. Pulses are a good source of vitamins. As shown in Table 8, chickpea can complement the vitamin requirement of an individual when consumed with other foods. Chickpea is a relatively inexpensive and good source of folic acid and tocopherols (both γ and α; Table 8)(Reference Ciftci, Ozkaya and Cevrimli72). It is a relatively good source of folic acid coupled with more modest amounts of water-soluble vitamins such as riboflavin (B2), pantothenic acid (B5) and pyridoxine (B6), and these levels are similar to or higher than those observed in other pulses (Table 9) (Reference Lebiedzińska and Szefer73). However, niacin concentration in chickpea is lower than that in pigeon pea and lentils (Table 9)(Reference Singh and Diwakar74).
Table 8 Vitamins in chickpea seeds

K, Kabuli; D, Desi; USDA, United States Department of Agriculture; ND, measured but not detected.
* Expressed as mg/100 g.
† The type of chickpea is not specified.
‡ Expressed as μg/100 g.
Table 9 Vitamin* content (mg/100 g) in different legumes(Reference Wang and Daun56)

Vit, vitamin; NA, not available.
* Vit A and B12 not detected in these legumes.
† Adopted from the United States Department of Agriculture(32).
‡ Expressed as μg/100 g.
Carotenoids
Plant carotenoids are lipid-soluble antioxidants/pigments responsible for bright colours (usually red, yellow and orange) of different plant tissues(Reference Bartley and Scolnik75). Carotenoids are classified into two types: (1) oxygenated, referred to as xanthophylls, which includes lutein, violaxanthin and neoxanthin, and (2) non-oxygenated, referred to as carotenes, which includes β-carotene and lycopene(Reference DellaPenna and Pogson76). The important carotenoids present in chickpea include β-carotene (Table 8), lutein, zeaxanthin, β-cryptoxanthin, lycopene and α-carotene. The average concentration of carotenoids (except lycopene) is higher in wild accessions of chickpea than in cultivated varieties or landraces (cv. Hadas)(Reference Abbo, Molina and Jungmann77). β-Carotene is the most important and widely distributed carotenoid in plants and is converted into vitamin A more efficiently than the other carotenoids(Reference Abbo, Molina and Jungmann77). On a dry seed weight basis, chickpea has a higher amount of β-carotene than ‘golden rice’ endosperm(Reference Abbo, Molina and Jungmann77, Reference Ye, Babili and Kioti78) or red-coloured wheats(32).
Isoflavones
Chickpea seeds contain several phenolic compounds(Reference Wood, Grusak, Yadav, Redden, Chen and Sharma9). Of these, two important phenolic compounds found in chickpea are the isoflavones biochanin A (5,7-dihydroxy-4′-methoxyisoflavone) and formononetin (7-hydroxy-4′-methoxyisoflavone)(Reference Wood, Grusak, Yadav, Redden, Chen and Sharma9). Other phenolic compounds detected in chickpea oil are daidzein, genistein, matairesinol and secoisolariciresinol(Reference Dixon79, Reference Champ80). The concentration of biochanin A is higher in Kabuli-type seeds (1420–3080 μg/100 g) compared with Desi-type seeds (838 μg/100 g)(Reference Mazur, Duke and Wahala81). The amount of formononetin in Kabuli- and Desi-type seeds is 215 μg/100 g and 94–126 μg/100 g, respectively(Reference Mazur, Duke and Wahala81).
Anti-nutritional factors
Despite the potential nutritional and health-promoting value of anti-nutritional factor (ANF), their presence in chickpea limits its biological value and usage as food. ANF interfere with digestion and also make the seed unpalatable when consumed in raw form by monogastric animal species(Reference Domoney, Shewry and Casey82). ANF can be divided into protein and non-protein ANF(Reference Duranti and Gius83). The non-protein ANF include alkaloids, tannins, phytic acid, saponins and phenolics, while the protein ANF include trypsin inhibitors, chymotrypsin inhibitors, lectins and antifungal peptides (Table 10)(Reference Roy, Boye and Simpson84, Reference Muzquiz, Wood, Yadav, Redden, Chen and Sharma85). Chickpea protease inhibitors are of two types: (1) Kunitz type – single-chain polypeptides of about 20 kDa with two disulphide bridges which inhibit the enzyme activity of trypsin but not chymotrypsin(Reference Srinivasan, Giri and Harsulkar86); and (2) Bowman–Birk inhibitors – which are also single-chain polypeptides of about 8 kDa in size with seven disulphide bridges which inhibit the enzyme activity of both trypsin and chymotrypsin(Reference Smirnoff, Khalef and Birk87, Reference Guillamon, Pedrosa and Burbano88). Protease inhibitors interfere with digestion by irreversibly binding with trypsin and chymotrypsin in the human digestive tract. They are resistant to the digestive enzyme pepsin and the stomach's acidic pH(Reference Roy, Boye and Simpson84). They negatively affect certain necessary enzymatic modifications required during food processing such as water-retaining capacity, gel-forming and foaming ability of different products(Reference Garcia-Cerreno89).
Table 10 Anti-nutritional factors in chickpea*
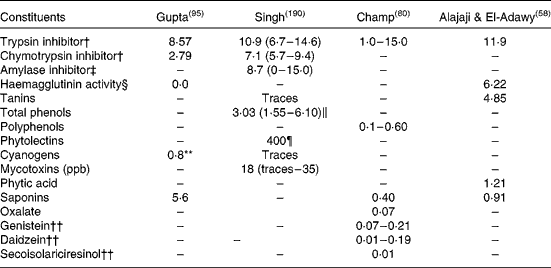
ppb, Parts per billion.
* The type of chickpea is not specified in any of the citations used.
† Expressed as units/mg protein.
‡ Expressed as units/g.
§ Expressed as units/mg sample.
∥ Expressed as mg/g.
¶ Expressed as units/g.
** Expressed as mg/100 g; others in g/100 g dry weight of sample.
†† Expressed as mg/100 g.
Phytic acid can bind to several important divalent cations (e.g. Fe, Zn, Ca and Mg) forming insoluble complexes and making them unavailable for absorption and utilisation in the small intestine(Reference Sandberg90–Reference Cheryan92). Tannins inhibit enzymes, reducing the digestibility and making chickpea astringent. Saponins are commonly found in several pulses including chickpea (Table 10)(Reference Oakenful and Sidhu93), giving the pulses a bitter taste and making them less preferable for consumption by humans and animals(Reference Birk, Peri and Liener94). Saponin content in chickpea (56 g/kg) is higher than that in other pulses such as green gram (16 g/kg), lentils (3·7–4·6 g/kg), faba bean (4·3 g/kg) and broad bean (3·5 g/kg)(Reference Gupta95).
Though the ANF act as limiting factors in chickpea consumption, they can be reduced or eliminated by soaking, cooking, boiling and autoclaving(Reference Alajaji and El-Adawy58). The ANF also have beneficial effects, which are discussed below.
Health benefits
Although pulses have been consumed for thousands of years for their nutritional qualities(Reference Kerem, Lev-Yadun and Gopher96), it is only during the past two to three decades that interest in pulses as food and their potential impact on human health has been revived. Chickpea consumption has been reported to have some physiological benefits that may reduce the risk of chronic diseases and optimise health (discussed in detail in the following paragraphs). Therefore, chickpeas could potentially be considered as a ‘functional food’ in addition to their accepted role of providing proteins and fibre. Different definitions are proposed that describe functional foods as: (1) ‘one encompassing healthful products, including modified food or ingredient that may provide health benefits beyond traditional ingredients’(Reference Milner97); (2) ‘foods that, by virtue of the presence of physiologically-active components, provide a health benefit beyond basic nutrition’(Reference Hasler98). As discussed above, chickpea is a relatively inexpensive source of different vitamins, minerals(Reference Wood, Grusak, Yadav, Redden, Chen and Sharma9, Reference Duke99, Reference Huisman, Van der Poel, Muehlbauer and Kaiser100) and several bioactive compounds (phytates, phenolic compounds, oligosaccharides, enzyme inhibitors, etc.) that could aid in potentially lowering the risk of chronic diseases. Due to its potential nutritional value, chickpea is gaining consumer acceptance as a functional food. Recent reports of the importance of chickpea consumption in relation to health are discussed below.
CVD, CHD and cholesterol control
In general, increased consumption of soluble fibre from foods results in reduced serum total cholesterol and LDL-cholesterol (LDL-C) and has an inverse correlation with CHD mortality(Reference Kushi, Meyer and Jacobs101–Reference Fehily and Sadler106). Usually, pulses and cereals have a comparable ratio of soluble to insoluble fibres per 100 g serving (about 1:3)(Reference Van Horn107). Chickpea seeds are a relatively cheap source of DF and bioactive compounds (e.g. phytosterols, saponins and oligosaccharides); coupled with its low glycaemic index (GI), chickpea may be useful for lowering the risk of CVD(Reference Duranti108). Chickpea has a higher total DFC (about 18–22 g)(Reference Tosh and Yada40) compared with wheat (about 12·7 g)(Reference Pittaway, Ahuja and Robertson109) and a higher amount of fat compared with most other pulses or cereals(Reference Williams, Singh, Saxena and Singh33, Reference Messina110). However, two PUFA, LA and OA, constitute almost about 50–60 % of chickpea fat. Intake of PUFA such as LA (the dominant fatty acid in chickpea; Table 4) has been shown to have a beneficial effect on serum lipids, insulin sensitivity and haemostatic factors, thereby it could be helpful in lowering the risk of CHD(Reference Hu, Manson and Willett111, Reference Sanders, Oakley and Miller112).
Isoflavones are diphenolic secondary metabolites that may lower the incidence of heart disease due to (1) the inhibition of LDL-C oxidation(Reference Tikkanen and Adlercreutz113, Reference Tikkanen, Wahala and Ojala114), (2) the inhibition of proliferation of aortic smooth muscle cells(Reference Pan, Ikeda and Takebe115) and (3) the maintenance of the physical properties of arterial walls(Reference van der Schouw, Pijpe and Lebrun116). Ferulic and p-coumaric acids are polyphenols that are found in chickpea seeds at low concentrations, and these have been shown to reduce blood lipid levels in rats(Reference Sharma117, Reference Sharma118). β-Carotene, the most studied carotenoid, is also present in chickpea seeds. Some cross-sectional and prospective studies have shown an inverse relationship between the incidence of CVD and plasma levels of antioxidants such as β-carotene and vitamin E(Reference Su, Bui and Kardinaal119). However, a large-scale randomised controlled trial (RCT) involving 22 071 healthy individuals demonstrated no benefit or harm of β-carotene supplementation (50 mg on alternate days) on CVD, although this study concluded that β-carotene supplementation could have some apparent benefits on subsequent vascular events(Reference Christen, Gaziano and Hennekens120). These neutral results have also been supported by several other intervention and prevention trials as reviewed by Stanner et al. (Reference Stanner, Hughes and Kelly121). Therefore, despite the evidence supporting the increased occurrence of CVD with a low intake of antioxidants or low levels of antioxidants in the plasma, there is at present no evidence from intervention trials to support the beneficial effect of β-carotene on CVD or CHD. The role of β-carotene, along with other vitamins or nutrients, in helping to reduce the incidence of CVD needs to be further investigated.
Foods rich in saponins have been reported to reduce plasma cholesterol by 16–24 %(Reference Thompson122). The mechanism of cholesterol reduction is by binding to dietary cholesterol(Reference Gestener, Assa and Henis123) or bile acids, thereby increasing their excretion through faeces(Reference Sidhu and Oakenful124, Reference Zulet and Martínez125). β-Sitosterol (the dominant phytosterol in chickpea) is helpful in decreasing serum cholesterol levels and the incidence of CHD(Reference Ling and Jones126–Reference Moreau, Whitaker and Hicks128). A higher intake of folic acid helps in reducing serum homocysteine concentrations, a risk factor for CHD(Reference Albert, Cook and Gaziano129). Folic acid supplementation has been shown to reduce homocysteine levels by 13·4–51·7 %(Reference Baker, Picton and Blackwood130–Reference Bazzano, Reynolds and Holder132). However, although a meta-analysis has shown an association between elevated levels of homocysteine and the risk of CHD and stroke(133), there are no RCT that indicate a benefit of folic acid supplementation on the risk of CVD, CHD or stroke.
A fibre-rich chickpea-based pulse (non-soyabean) diet has been shown to reduce the total plasma cholesterol levels in obese subjects(Reference Crujeiras, Parra and Abete134). This study was conducted on thirty obese subjects (BMI 32·0 (sd 5·3) kg/m2) with a mean age group of 36 (sd 8) years. The subjects were divided into two groups of fifteen each and fed with a hypoenergetic diet consisting of a chickpea-based pulse diet and a control diet (no pulses) for a period of 8 weeks (4 d/week). After 8 weeks, the total cholesterol levels in the chickpea-based pulse diet-fed group decreased from 215 to 182 mg/dl, whereas a smaller decrease (181 to 173 mg/dl) was observed for the control diet-fed group(Reference Crujeiras, Parra and Abete134). The proposed mechanism for this hypocholesterolaemic effect is the inhibition of fatty acid synthesis in the liver by fibre fermentation products such as propionate, butyrate and acetate(Reference Crujeiras, Parra and Abete134). SCFA (e.g. propionate) have been shown to inhibit both cholesterol and fatty acid biosynthesis by inhibiting acetate (provides acetyl-CoA) utilisation(Reference Wright, Anderson and Bridges135). Feeding a chickpea diet to rats also resulted in a favourable plasma lipid profile(Reference Yang, Zhou and Gu136). In this study, thirty healthy male ‘Sprague–Dawley’ rats were fed three different diets for 8 months: a normal-fat diet (5 g fat, 22 g protein and 1381 kJ/100 g); a high-fat diet (HFD; lard 20 % (w/w), sugar 4 % (w/w), milk powder 2 % (w/w) and cholesterol 1 % (w/w) into standard laboratory chow, which contained 25·71 g fat, 19·54 g protein and 1987 kJ/100 g diet); a HFD plus chickpea diet (same as the HFD, but 10 % crushed chickpea seeds replaced the standard chow; it contained 25·11 g fat, 19·36 g protein and 1965 kJ/100 g). Several pro-atherogenic factors, including TAG, LDL-C and LDL-C:HDL-cholesterol ratio, decreased with consuming the chickpea-based diet(Reference Yang, Zhou and Gu136). In eighty-four healthy ‘Sprague–Dawley’ rats divided into fourteen groups of six each fed diets containing chickpea (49–65·4 % of diet) and peas (46–62 % of diet) for 35 d, lower levels of plasma cholesterol were recorded(Reference Wang and McIntosh137). The decrease in cholesterol levels varied with the processing method used; extrusion and boiling had similar effects for chickpeas, whereas extrusion was most effective in peas. Phytosterols present in chickpea, along with other factors (e.g. isoflavones, oligosaccharides), reduce LDL-C levels in the blood by inhibiting the intestinal absorption of cholesterol due to the similarity in their chemical structure with cholesterol, thereby potentially reducing the risk of CHD(Reference Wood, Grusak, Yadav, Redden, Chen and Sharma9, Reference Pittaway, Ahuja and Robertson109).
Diabetes and blood pressure
Pulses such as chickpea have a higher amount of resistant starch and amylose(Reference Pittaway, Ahuja and Robertson109). Amylose has a higher degree of polymerisation (1667 glucose v. 540), rendering the starch in chickpea more resistant to digestion in the small intestine which ultimately results in the lower availability of glucose(Reference Pittaway, Ahuja and Robertson109, Reference Muir and O'Dea138). The lower bioavailability of glucose results in the slower entry of glucose into the bloodstream, thus reducing the demand for insulin which results in the lowering of the GI and insulinaemic postprandial response(Reference Kendall, Emam and Augustin139, Reference Osorio-Díaz, Agama-Acevedo and Mendoza-Vinalay140). The lowering of the GI is an important aspect in reducing both the incidence and the severity of type 2 diabetes(Reference Regina, Bird and Topping141). Further, increased consumption of resistant starch is related to improved glucose tolerance and insulin sensitivity(Reference James, Muir and Curtis102, Reference Tharanathan and Mahadevamma142, Reference Jenkins, Kendall and Augustin143). LA, a PUFA, is biologically important due to its involvement in the production of PG. PG are involved in the lowering of blood pressure and smooth muscle constriction(Reference Aurand, Woods and Wells144). Also, LA and linolenic acid are required for growth and performing different physiological functions(Reference Pugalenthi, Vadivel and Gurumoorthi145). Additionally, phytosterols, such as β-sitosterol, are helpful in reducing blood pressure(Reference Ling and Jones126–Reference Moreau, Whitaker and Hicks128). LA and β-sitosterol are the major PUFA and phytosterol, respectively, in chickpea seeds; therefore, chickpea seeds could be incorporated as part of a regular diet that may help to reduce blood pressure.
Inclusion of chickpea in a high-fat rodent feed reduced the deposition of visceral and ecotopic fats, resulting in hypolipidaemia and insulin-sensitising effects in rats(Reference Yang, Zhou and Gu136). Incorporation of chickpeas in a human study also led to improvements in fasting insulin and total cholesterol content(Reference Pittaway, Robertson and Ball146). Total cholesterol and fasting insulin were reduced by 7·7 mg/dl and 5·2 pmol/l, respectively. In this study, forty-five healthy individuals were fed with a minimum of 104 g chickpea/d for 12 weeks as part of their regular diet.
Cancer
Butyrate is a principal SCFA (about 18 % of the total volatile fatty acids) produced from the consumption of a chickpea diet (200 g/d) in healthy adults(Reference Fernando, Hill and Zello147). Butyrate has been reported to suppress cell proliferation(Reference Cummings, Stephen, Branch, Bruce, Correa, Lipkin, Tannenbaum and Wilkins148) and induce apoptosis(Reference Mathers149), which may reduce the risk of colorectal cancer. Butyrate inhibits histone deacetylase, which prevents DNA compaction and induces gene expression. It has also been suggested that butyrate shunts the cells along the irreversible pathway of maturation leading to cell death(Reference Mathers149). Inclusion of β-sitosterol (the major phytosterol in chickpea; Table 7) in a rat diet reduced N-methyl-N-nitrosourea (carcinogen)-induced colonic tumours(Reference Raicht, Cohen and Fazzini150). Saponin-rich foods have been shown to inhibit pre-neoplastic lesions caused by azoxymethane in the rat colon(Reference Koratkar and Rao151). Protease inhibitors are also known to suppress carcinogenesis by different mechanisms, but their precise targets are still unknown(Reference Duranti and Gius83, Reference Moy and Bilings152, Reference Kennedy153).
Lycopene, an oxygenated carotenoid present in chickpea seeds, may reduce the risk of prostate cancer(Reference Giovannucci, Ascherio and Rimm154). Though there are association studies suggesting a role for lycopene in protection against prostate cancer, the results from very few RCT conducted are not sufficient either to support or refute the role of lycopene in cancer prevention(Reference Konijeti, Henning and Moro155, Reference Ilic, Forbes and Hassed156). Ziegler(Reference Ziegler157) reported that lower levels of carotenoids either in the diet or body can enhance the risk of certain types of cancer. Studies have shown a direct positive correlation between a carotenoid-rich diet and a decreased incidence of lung and other forms of cancer(Reference Bendich158). The cancer prevention ability of carotenoids could be due to their antioxidant properties(Reference Seis, Stahl and Sundquist159), but the exact mode of action needs to be identified.
Biochanin A, a chickpea isoflavone, inhibits the growth of stomach cancer cells in vitro and reduces tumour growth when the same cells are transferred to mice(Reference Dixon79, Reference Yanagihara, Ito and Toge160). Further, chickpea isoflavone extract specifically inhibited epithelial tumour growth and had no effect on healthy cells(Reference Girón-Calle, Vioque and del Mar Yust161). Murillo et al. (Reference Murillo, Choi and Pan162) have shown a 64 % suppression of azoxymethane-induced aberrant cryptic foci in rats fed with 10 % chickpea flour, and indicated that saponins could be one of the factors for the reduction of lesions. N-Nitrosodiethylamine, a nitrosoamine, has been reported to cause carcinogenesis through DNA mutation(Reference Mittal, Vadhera and Brar163). Inclusion of chickpea seed coat fibre in the diet has been shown to reduce the toxic effects of N-nitrosodiethylamine on lipid peroxidation and antioxidant potential(Reference Mittal, Vadhera and Brar163). The average percentage decrease in lipid peroxidation was about 21 % in the liver and lungs, about 15·50 % in the spleen and kidney and about 12·46 % in the heart. In eighteen rats divided into three groups of six each, a hypercholesterolaemic diet was fed for 4 weeks: group I was fed a control hypercholesterolaemic diet (starch (63 %), oil (10 %), casein (15 %), cellulose (5 %), salt mixture (5 %), yeast powder (1 %) and cholesterol (1 %)); group II fed a hypercholesterolaemic diet plus N-nitrosodiethylamine (100 mg/kg); group III fed a group II diet+5 % chickpea seed coat fibre.
Weight loss/obesity
Intake of foods, which are rich in DF, is associated with a lower BMI(Reference Howarth, Saltzman and Roberts164, Reference Pereira and Ludwig165). Eating of foods with a high fibre content helps in reaching satiety faster (fullness post-meal), and this satiating effect lasts longer as fibre-rich foods require a longer time to chew and digest in the intestinal system(Reference Marlett, McBurney and Slavin103, Reference Burley, Paul and Blundell166). Additionally, consumption of low-GI foods resulted in an increase in cholecystokinin (a gastrointestinal peptide and hunger suppressant) and increased satiety(Reference Swinburn, Caterson and Seidell167–Reference Holt, Brand and Soveny169). Diets with low-GI foods resulted in reduced insulin levels and higher weight loss compared with those with higher-GI foods(Reference Slabber, Barnard and Kuyl170). Since chickpea is considered to be a low-GI food, it may help in weight-loss and obesity reduction.
Chickpea supplementation in the diet prevented increased body weight and the weight of epididymal adipose tissues in rats(Reference Yang, Zhou and Gu136). At the end of the 8-month experimental period, rats fed on a HFD weighed 654 g v. those fed with a HFD plus chickpea (562 g). The epididymal fat pad weight:total body weight ratio was higher in rats fed on a HFD (0·032 g/g) compared with those fed on a HFD plus chickpea diet (0·023 g/g; details of this experiment are explained in the ‘CVD, CHD and cholesterol control’ section)(Reference Yang, Zhou and Gu136). Therefore, chickpea being a low-GI food could be an effective choice in weight-loss programmes. Chickpea has been reported to decrease fat accumulation in obese subjects. This aids in improving fat metabolism and could be helpful in correcting obesity-related disorders(Reference Yang, Zhou and Gu136). Chickpea supplementation in the diet resulted in increased satiation and fullness(Reference Murty, Pittaway and Ball171). In this study, forty-two participants consumed a chickpea-supplemented diet (average 104 g/d) for 12 weeks; this was preceded and succeeded by their habitual diet for 4 weeks each.
Gut health and laxation
A significant increase (18 %) in DF intake was recorded when 140 g/d of chickpea and chickpea flour were consumed by nineteen healthy individuals for 6 weeks(Reference Nestel, Cehun and Chronopoulos172). Similarly, Murty et al. (Reference Murty, Pittaway and Ball171) reported a 15 % increase in DF intake in forty-two volunteers (age 52·17 (sd 6·30) years old). These studies revealed an overall improvement in bowel health accompanied by an increased frequency of defecation, ease of defecation and softer stool consistency while on a chickpea diet compared with a habitual diet. DF promote laxation/bowel function by aiding in the movement of material through the digestive system.
Other health benefits
Chickpea seed oil contains different sterols, tocopherols and tocotrienols(Reference Akihisa, Yasukawa and Yamaura173–Reference Akihisa, Nishismura and Nakamura175). These phytosterols have been reported to exhibit anti-ulcerative, anti-bacterial, anti-fungal, anti-tumour and anti-inflammatory properties coupled with a lowering effect on cholesterol levels(Reference Murty, Pittaway and Ball171, Reference Arisawa, Kinghorn and Cordell176). Δ7-Avenasterol and Δ5-avenasterol, phytosterols present in chickpea oil, have antioxidant properties even at frying temperatures(Reference Wang, Hicks and Moreau177). Carotenoids such as lutein and zeaxanthin, the major carotenoids in chickpea seeds, are speculated to play a role in senile or age-related macular degeneration. Though there are some epidemiological and association studies suggesting a beneficial effect of lutein and zeaxanthin on age-related macular degeneration, evidence from RCT on the effect of carotenoids on age-related macular degeneration is not presently available(Reference Mozaffarieh, Sacu and Wedrich178). Carotenoids have been reported to increase natural killer cell activity(Reference Santos, Leka and Ribaya179). Vitamin A, a derivative of β-carotene, is important in several developmental processes in humans such as bone growth, cell division/differentiation and, most importantly, vision. It has been reported that at least three million children develop xerophthalmia (damage to cornea) and about 250 000–500 000 children become blind due to vitamin A deficiency(Reference Reifen180). Chickpea has been reported to have higher levels of carotenoids (explained above) than ‘golden rice’, and it could be potentially used as a source of dietary carotenoids.
Chickpea seeds have been used in traditional medicine as tonics, stimulants and aphrodisiacs(Reference Pandey and Enumeratio181). Further, they are used to expel parasitic worms from the body (anthelmintic property), as appetizers, for thirst quenching and reducing burning sensation in the stomach(Reference Zia-Ul-Haq, Iqbal and Ahmad35). In the Ayurvedic system of medicine, chickpea preparations are used to treat a variety of ailments such as throat problems, blood disorders, bronchitis, skin diseases and liver- or gall bladder-related problems (biliousness)(Reference Sastry and Kavathekar182). In addition to these applications, chickpea seeds are also used for blood enrichment, treating skin ailments, ear infections, and liver and spleen disorders(Reference Warner, Nambiar and Remankutty183). Uygur people of China have used chickpea in herbal medicine for treating hypertension and diabetes for over 2500 years(Reference Li, Jiang and Zhang184–Reference Zhang, Jiang and Wang186).
Conclusions
The information presented in this review shows the potential nutritional importance of chickpea and its role in improved nutrition and health. It is an affordable source of protein, carbohydrates, minerals and vitamins, DF, folate, β-carotene and health-promoting fatty acids. Scientific studies have provided some evidence to support the potential beneficial effects of chickpea components in lowering the risk of various chronic diseases, although information pertaining to the role of individual chickpea components in disease prevention and the mechanisms of action are limited to date. This is due to the complex nature of disease aetiology and various factors having an impact on their occurrence. It is imperative that the scientific community continues to unravel the mechanisms involved in disease prevention and determine how food bioactives from foods such as chickpea can influence human health. Further research, especially well-conducted RCT, needs to be performed to provide compelling evidence for the direct health benefits of chickpea consumption.
Acknowledgements
We would like to acknowledge the ICRISAT library staff and other researchers for their help in this review. The authors have no conflict of interests to declare. A. K. J. acquired the necessary material and wrote most of the sections. P. M. G. and R. N. C. contributed to the writing of the nutritional aspects of the manuscript. P. M. G. also corresponded with the other authors. C. L. L. G. helped in writing the introduction part.