Next to its intracellular role as an energy carrier, ATP and its metabolites function extracellularly as the main ligands involved in purinergic signalling. Purinergic signalling is important for the regulation of inflammation, muscle contraction, neurotransmission and nociception (see Bours et al. and Burnstock(Reference Bours, Swennen and Di Virgilio1–Reference Burnstock3) for recent reviews). Modulation of purinergic signalling by administration of purine receptor antagonists (e.g. clopidogrel(Reference Raju, Eikelboom and Hirsh4)) or by intravenous administration of ATP or adenosine(Reference Agteresch, Dagnelie and van Der Gaast5, Reference Beijer, Hupperets and van den Borne6) has been shown to effectively modulate human health.
Despite the small number of studies on the effectiveness of oral ATP administration to modulate purinergic signalling, capsules and tablets containing ATP at dosages between 90 and 250 mg/d are being marketed on the Internet as being highly efficacious. Capsules containing 30 mg ATP are presently registered in France (Atépadène®) as an adjunct in the treatment of low back pain of muscular origin. In the late 1980s, two randomised, double-blind, placebo-controlled trials were published in non-indexed medical journals, showing that administration of 90 mg Atépadène® was safe(Reference Mathieu, Richard and Vignon7, Reference de Sezeand and Bordier8). In a recent double-blind trial, the efficacy of Atépadène® for 1 month compared with placebo was investigated in patients with low back pain(Reference Bannwarth, Allaert and Avouac9), while in an open-label trial, the same drug was tested against the advice to stay active(Reference Rossignol, Allaert and Rozenberg10). Both trials indicated that oral ATP had a modest benefit on a secondary end point (the use of rescue analgesics) compared with the non-pharmacological treatment. No differences between ATP and placebo groups were found when considering pain or functional status of the patients. The investigators also noted that in the non-blinded trial, the positive effect might largely be explained by the patients' knowledge of receiving a drug(Reference Bannwarth, Allaert and Avouac9, Reference Rossignol, Allaert and Rozenberg10).
In order to investigate the bioavailability of oral ATP, we previously conducted a study in which an acute oral dose of ATP was administered as an enteric-coated multi-particulate formulation designed to release its contents in the proximal small intestine (further referred to as ‘pellets’)(Reference Coolen, Arts and Bours11). This single dose of ATP pellets did not result in any increase in blood concentrations of ATP or of its metabolites except uric acid. The results remained similar when ATP was administered directly into the duodenum via a naso-duodenal tube. We concluded from this study that a single dose of oral ATP at the given dosage is not effectively taken up. So far, the oral bioavailability of ATP after prolonged administration (as recommended for oral ATP supplements on the Internet) has only been studied in animals. In experimental studies, contrary to expectations, basal plasma ATP levels in animals treated with 5 mg orally administered ATP per kg for 30 d were lower than those in water-treated control animals, suggesting a potential up-regulation of ATP-metabolising enzymes in plasma over the 30 d study. A similar effect was found when oral ATP doses of 1, 5 or 10 mg/kg were given for 20 d. Interestingly, when a single dose of 14C-labelled ATP was administered directly into the lumen of the jejunum of the rats treated with ATP for 30 d, a rapid 1000-fold increase in portal plasma ATP concentration was found compared with the control rats(Reference Kichenin, Seman and Decollogne12). The authors suggested this to be the result of breakdown of ATP to adenosine by intestinal nucleotidases, followed by increased absorption of adenosine by nucleoside transporters present in the intestinal membrane, in combination with an increased efflux of ATP and nucleosides from intestinal cells into the blood stream. Several nucleoside transporters are now known, belonging to two families of concentrative and equilibrative nucleoside transporters(Reference Pastor-Anglada, Errasti-Murugarren and Aymerich13). To test whether such an adaptive metabolic response to chronic ATP administration as observed in animals might also occur in humans, we set out to study this in healthy human volunteers.
The present study had two specific objectives: first, to establish whether whole blood and plasma concentrations of ATP and its degradation products are influenced by oral administration of 0, 250, 1250 or 5000 mg ATP per d for 4 weeks in healthy human subjects; and second, to investigate whether 4 weeks of oral ATP administration leads to adaptations in uptake, metabolism and excretion of a single acute 5000 mg dose of ATP. Adverse events were registered and safety was monitored by measuring a set of liver and kidney blood parameters.
Materials and methods
Study design
A total of thirty-three subjects were assigned in a blinded manner to one of four dosage groups by computer-generated randomisation lists with a block size of eight. On days 1–27, subjects were instructed to ingest enteric-coated pellets twice a day, delivering a daily dose of ATP of either (a) 5000, (b) 1250, (c) 250 or (d) 0 mg (placebo). On days 0 and 28, all the subjects received a single dose of 5000 mg ATP as enteric-coated pellets. Subjects ingested the pellets with approximately 200 ml of water that had been acidified to a pH between 1 and 5.
Participants
Healthy male and female volunteers were recruited. Exclusion criteria were: history of lung, heart, intestinal, stomach or liver disease, use of prescription medication (except contraceptives), smoking, drug use, dietary restrictions and pregnancy. Furthermore, only subjects aged 18–60 years could participate. To minimise between-subject variation, the subjects were requested to abstain from products containing alcohol or caffeine as well as from purine-rich foods, such as game, offal, sardines, anchovies and alcohol-free beer for 2 d before test days 0, 7, 14, 21 and 28. Subjects were asked to fast from 22.00 hours the previous day until the end of test days 0 and 28 (16.00 hours) and to refrain from any vigorous physical activity 24 h before each test day. On days 0 and 28, subjects were allowed to drink water starting 30 min after ATP administration. On all intervening days, the subjects were instructed to ingest the pellets at two self-chosen moments during the day, each one either at least half an hour before or 2 h after a meal. The present study was conducted according to the guidelines laid down in the Declaration of Helsinki, and all procedures involving human subjects were approved by the Ethics Committee of Maastricht University Medical Centre, The Netherlands. A written informed consent was obtained from all the subjects.
Materials
ATP disodium salt and adenosine were purchased from Fagron BV, Uitgeest, The Netherlands. ADP disodium salt, AMP Na salt, adenine, inosine, hypoxanthine, uric acid and perchloric acid 70 % solution in water were purchased from Sigma Chemical Company (St Louis, MO, USA). KOH, KH2PO4, K2CO3, K2HPO3·3H2O and NaOH were obtained from Merck (Darmstadt, Germany) and 0·9 % saline was purchased from Braun (Melsungen, Germany).
Production of pellets
Pellets were obtained from the laboratory of Pharmaceutical Technology, Ghent University, Ghent, Belgium. ATP pellets were produced as described by Huyghebaert et al. (Reference Huyghebaert, Vermeire and Remon14), with minor modifications to obtain an ATP content of >40 % (w/w) after coating. Placebo pellets were produced in the same manner, but without ATP. The pellets were coated with 30 % Eudragit® L30D-55 (Röhm Pharma, Darmstadt, Germany) and mixed with anionic copolymers of methacrylic acid and ethyl acrylate (1:1). After coating, the pellets were cured overnight at room temperature at 60 % humidity, packed in sealed double-plastic bags and stored at 4°C. Pellets were used within 3 months after production. To ensure blinding of all personnel involved in the study, a separate person not otherwise involved in the study mixed the ATP and placebo pellets at proportions needed to obtain the different doses (0, 250, 1250 and 5000 mg ATP/d), packaged and sealed the pellets in small plastic bags each containing one daily dose and coded them with subjects' identification numbers according to the randomisation list.
Dissolution testing
The pellets underwent quality control using a dissolution test as described before(Reference Coolen, Arts and Bours11). This test confirmed that the dissolution properties of the pellets were similar to those used in our previous study(Reference Coolen, Arts and Bours11). The release of ATP or degradation products after 120 min in 0·1 m-HCl was < 3 %. Subsequent transfer to a buffer solution of pH 6·5 caused a release of 50 % of the remaining ATP within 10 min, which increased to >90 % after 60 min (data not shown).
Sample collection
On days 0 and 28, subjects arrived at the study facility (Maastricht University Medical Centre) at about 08.00 hours. Venous blood was collected from the antecubital vein using a 20-gauge intravenous catheter (Terumo-Europe NV, Leuven, Belgium) connected to a three-way stopcock (Discofix®; Braun Melsungen AG, Melsungen, Germany). Blood was collected into 4 ml EDTA tubes (Venosafe, Terumo-Europe NV) by inserting a 21-gauge multisample needle (Venoject Quick Fit, Terumo-Europe NV) into the membrane of a closing cone (IN-Stopper; Braun Melsungen AG) that was attached directly to the stopcock. The anticoagulant EDTA inhibits the extracellular hydrolysis of ATP by Ca2+- and Mg2+-activated enzymes, such as plasma membrane-bound CD39(Reference Marcus, Broekman and Drosopoulos15). Serum tubes were used to collect the samples for the assessment of safety parameters (see later for details). After blood collection, the tubes were gently inverted three times and put on ice. For whole blood measurements, 500 μl EDTA blood was immediately added to 500 μl ice-cold perchloric acid (8 %, w/v), vortex-mixed and frozen in liquid N2. For plasma ATP determination, every second sample of EDTA blood was diluted 1:2 with FireZyme Dilution Buffer (BioMedica Diagnostics, Inc., Windsor, NS, Canada) and immediately centrifuged at 3000 g for 10 min at 4°C. All the blood samples were stored at − 80°C awaiting analysis.
On days 7, 14 and 21, single samples of venous blood were collected into EDTA tubes for whole blood ATP determination. For the experiments on days 0 and 28, baseline blood was sampled 30, 20 and 10 min before administration of 5000 mg ATP to all thirty-two subjects. Between 30 and 210 min after ATP administration, blood samples were taken every 15 min, and between 210 and 420 min, samples were taken every 30 min. Urine was collected 20 min before and 120, 220 and 400 min after ATP administration, stored on ice before being frozen in − 80°C at the end of the day. The total urine volume at each time point was determined and each sample was stored separately.
ATP measurement in whole blood samples by HPLC
Equipment, sample preparation and measurement conditions for the determination of ATP and its metabolites ADP, AMP, adenosine, adenine, inosine, hypoxanthine and uric acid have been previously described and validated(Reference Coolen, Arts and Swennen16). Briefly, after thawing, the protein fraction was precipitated (12 000 g, 10 min, 4°C) and 40 μl 2 m-K2CO3 in 6 m-KOH was added to 650 μl of the supernatant to neutralise the pH. The resulting insoluble perchlorate was removed by centrifugation (12 000 g, 10 min, 4°C) and 40 μl of supernatant was mixed with 160 μl of 0·05 m-phosphate buffer (pH 6·0) in HPLC vials. The compounds were quantified with HPLC by UV detection at 254 nm.
ATP measurement in plasma samples
The diluted plasma samples were thawed and a CellTiter-Glo® ATP-assay kit (Promega Benelux BV, The Hague, The Netherlands) was used according to the manufacturer's instructions to measure the bioluminescence on a Glomax plate reader.
Uric acid and creatinine measurement in urine samples
Urine samples were thawed and uric acid and creatinine levels were measured using an automated analyser (Beckman-Coulter, Sharon Hill, PA, USA). Uric acid concentration was corrected for creatinine by dividing the uric acid concentration by the creatinine concentration.
Safety parameters
In order to assess the safety of a 4-week period of daily administration of pellets containing up to 5000 mg ATP, concentrations of creatinine, alkaline phosphatase, alanine aminotransferase, lactate dehydrogenase and creatine kinase were measured in undiluted serum samples using a Beckman Synchron CX5 (Beckman Instruments, Inc., Gladesville, CA, USA) automatic analyser.
Statistical analysis
Results are reported as means and standard errors. Fasting whole blood ATP and metabolite concentrations were calculated from one sample taken in the morning on days 7, 14 and 21 and from the average of three samples taken at 30, 20 and 10 min before administration on days 0 and 28. The change in area under the curve (ΔAUC) from the fasting concentration on day 0 was calculated using the trapezoidal rule for each subject for the 28 d period. ΔAUC on days 0 and 28 during the 420 min following ATP administration were calculated relative to baseline values on the same day. For uric acid in urine and for plasma ATP, the AUC was calculated using the trapezoidal rule. Differences in ΔAUC or AUC between dosage groups were evaluated with one-way ANOVA, and a least significant difference post hoc test when appropriate. Differences in response to 5000 mg ATP between days 0 and 28 were evaluated with a paired t test. All the analyses were performed using SPSS 17.0 (SPSS, Inc., Chicago, IL, USA), and P < 0·05 was considered statistically significant.
Results
Study population
A total of thirty-two healthy volunteers (thirteen men and nineteen women) completed the 4-week intervention study. Men were on average 28·8 (sem 14·1) years old and weighed 69·4 (sem 9·2) kg with a BMI of 21·8 (sem 1·5) kg/m2 while women were on average 21·7 (sem 4·6) years old and weighed 66·7 (sem 6·5) kg with a BMI of 22·8 (sem 1·6) kg/m2. One female participant (assigned to the placebo group) dropped out of the study because of repeated vomiting after ingesting the pellets. All other subjects tolerated the daily administration generally well. Temporary nausea without vomiting occurred in two women between 120 and 135 min after ingestion of the first 5000 mg dose of ATP on day 0 of the study. Other minor remarks included a bad taste of the pellets (n 1) and a diminished appetite (one female subject from the highest dosage group).
ATP and metabolites in whole blood
No significant change in fasting whole blood ATP concentration occurred over the entire 4-week intervention period, using doses of 0–5000 mg/d of ATP (Table 1). Administration of a single oral dose of 5000 mg ATP on days 0 and 28 of the study also did not lead to increased ATP concentrations in whole blood during the 420 min following administration in any of the groups, and there was no difference in response to ATP between days 0 and 28 (data not shown). The concentrations of adenosine and other metabolites, except uric acid, were not increased after oral administration (data not shown). Uric acid concentrations increased significantly over the 4-week period, and this was only observed in the 5000 mg dosage group v. the 0, 250 and 1250 dosage groups (Table 1). On days 0 and 28, administration of a single dose of 5000 mg ATP increased uric acid concentrations on average up to 40 % above fasting concentrations (Fig. 1). Uric acid concentrations started to increase at approximately 30 min after ATP administration, and levelled off at approximately 150 min until at least 300 min after ATP administration. The ΔAUC for uric acid over the 420 min period following administration of the single dose of 5000 mg ATP did not differ significantly between days 0 and 28 nor between dosage groups (Table 2).
Table 1 Whole blood concentrations of ATP and uric acid in overnight-fasted healthy subjects after oral administration of placebo, 250, 1250 or 5000 mg ATP per d as enteric-coated pellets for 4 weeks
(Mean values with their standard errors)‡
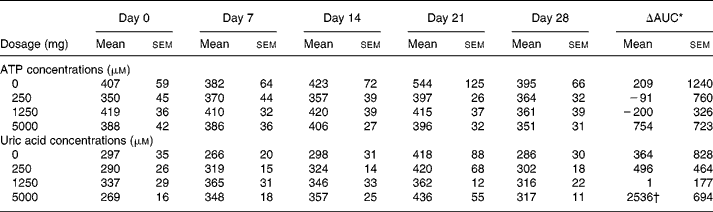
AUC, area under the curve; LSD, least significant difference.
* Mean values were significant overall in AUC between dosage groups for uric acid (P = 0·02), but not for ATP (P = 0·83, ANOVA).
† Mean values were significant for dose of 5000 mg from 0, 250 and 1250 mg dosage groups (P < 0·03, post hoc LSD-test).
‡ Values of eight subjects/dosage group.

Fig. 1 Increase in whole blood uric acid concentration following an acute oral bolus of 5000 mg ATP as enteric-coated pellets to healthy subjects (n 8 for each dosage group). On days 1–27, subjects received placebo (a), 250 mg/d ATP (b), 1250 mg/d ATP (c) or 5000 mg/d ATP (d). , day 0;
, day 28.
Table 2 Change in area under the curve from baseline (ΔAUC) for whole blood uric acid concentrations on days 0 and 28, after a single oral dose of 5000 mg ATP as enteric-coated pellets
(Mean values with their standard errors)*

* Values of eight subjects/dosage group.
† P values are calculated by paired samples t tests across days and by one-way ANOVA across dosages.
Plasma ATP
Fig. 2 shows that plasma ATP concentrations after administration of 5000 mg ATP on days 0 and 28 did not differ significantly between the different dosage groups (P = 0·42 for day 0 and P = 0·47 for day 28), nor between days 0 and 28 (P>0·05).
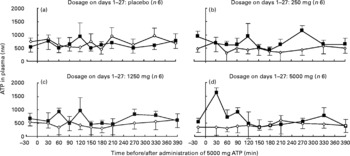
Fig. 2 Plasma ATP concentrations following an acute oral bolus of 5000 mg ATP as enteric-coated pellets to healthy subjects. ATP was administered at t = 0 (n 6 or 4 per dosage group). On days 1–27, subjects received placebo (a), 250 mg/d ATP (b), 1250 mg/d ATP (c) or 5000 mg/d ATP (d). , day 0;
, day 28.
Uric acid and creatinine in urine
Analysis of urine samples collected on days 0 and 28 of the study revealed no clear upward or downward trend in uric acid concentrations relative to creatinine (Fig. 3). There was no significant difference between days 0 and 28 within each dosage group (P>0·10 for all dosage groups). Within days 0 and 28, there were no differences between the dosages of ATP (P = 0·92 on day 0 and P = 0·22 on day 28).
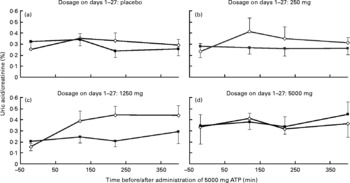
Fig. 3 Concentrations of uric acid relative to creatinine in urine following an acute oral bolus of 5000 mg ATP as enteric-coated pellets to healthy subjects (n 8 for each dosage group). On days 1–27, subjects received placebo (a), 250 mg/d ATP (b), 1250 mg/d ATP (c) or 5000 mg/d ATP (d). Error bars represent standard deviation. , day 0;
, day 28.
Safety parameters
As shown in Table 3, none of the safety parameters changed in response to administration of up to 5000 mg ATP for 4 weeks and all median concentrations remained in the reference range for healthy volunteers. Although we observed some individual values for alkaline phosphatase, lactate dehydrogenase and creatine kinase above the reference range, this occurred to a similar extent at baseline (i.e. before ATP/placebo administration) and after 28 d, and also occurred to a similar extent in the placebo and the highest ATP dose group (Table 3).
Table 3 Serum concentrations of five safety parameters in samples collected 20 min before (t=−20) or 105 min (t=105) after oral administration of 5000 mg ATP as enteric-coated pellets to healthy subjects
(Median values and ranges)*

ALAT, alanine amino transferase; LD, lactate dehydrogenase; CK, creatine kinase.
* Eight subjects/dosage group.
† The reference values are as follows: creatinine 60–115 μmol/l (male), 50–100 μmol/l (female); alkaline phosphatase < 140 IU/l; ALAT < 45 IU/l (male), < 35 IU/l (female); LD < 480 IU/l; creatine kinase < 225 IU/l (male), < 160 IU/l (female) (reference values and full names of the parameters apply to healthy volunteers).
‡ An individual maximum value exceeded the reference value.
§ The number of individuals with higher than maximum values (n 1).
∥ The number of individuals with higher than maximum values (n 2).
¶ The number of individuals with higher than maximum values (n 4).
Discussion
In this randomised, double-blind, placebo-controlled trial, we investigated the effects of a 4-week period of daily oral ATP administration. The first objective of the present study was to assess whether prolonged daily oral administration of ATP would lead to increased blood and plasma concentrations of ATP and its degradation products. Even after 4 weeks of oral administration, we detected no rise in concentrations of ATP or any of its metabolites, except for uric acid, which showed a significant overall increase in the highest ATP dosage group compared with the other dosage groups over the 4-week period.
Our findings partly agree with previous studies conducted in rats (5 mg/kg per d for 30 d) and rabbits (20 mg/kg per d for 14 d) by Kichenin et al. (Reference Kichenin, Seman and Decollogne12, Reference Kichenin, Decollogne and Angignard17), who found no increase in erythrocyte ATP and adenosine concentrations. However, these authors even reported a decrease in basal plasma ATP concentrations in rats receiving 5 mg/kg per d for 30 d(Reference Kichenin, Seman and Decollogne12). High-dose oral ATP administration trials in human subjects have not been reported before. In one previous trial, healthy males ingested much lower doses of ATP (0, 150 or 225 mg/d) for 14 d. No significant increase in ATP levels was observed and no other metabolites were measured in that human trial(Reference Jordan, Jurca and Abraham18).
It is known that ATP is quickly metabolised in the small intestine. First, ecto-nucleotidase triphosphatase diphosphohydrolases present on the luminal side of enterocytes dephosphorylate ATP via ADP to AMP(Reference Yegutkin19). Secondly, AMP is also not likely to stay intact in the small intestine, since ecto-5′-nucleotidase (CD73) and alkaline phosphatases degrade it to adenosine(Reference Strohmeier, Lencer and Patapoff20). Adenosine can be transported to the intestinal villi and into erythrocytes by nucleoside transporters(Reference Molina-Arcas, Casado and Pastor-Anglada21). We suggest that the enzymes adenosine deaminase (present in endothelial cells) and xanthine oxidase (present in the liver) efficiently degraded adenosine to uric acid(Reference Fox22). This could explain our observation that exclusively uric acid was found to be increased during ATP administration. This increase in uric acid, but not in ATP or other metabolites, upon administration of a single dose of 5000 mg ATP is in accordance with our earlier findings(Reference Coolen, Arts and Bours11). Uric acid has an elimination half-life of approximately 20 h(Reference Geren, Bendich and Bodansky23). Indeed, the uric acid concentration we observed in the present study did not decline much during 6 h after administration of 5000 mg ATP. Since pellets were ingested twice a day from days 1 to 27, the periods between ATP ingestion were close to 12 h; therefore, partial transfer of uric acid from the last dosage from the previous day may have contributed to the observed rise in basal uric acid concentrations on days 7, 14, 21 and 28 (Table 1). The implications of this increase in uric acid concentration are discussed later.
Our second objective was to investigate whether the uptake, metabolism and excretion of an acute oral ATP bolus would be influenced by prolonged administration (4 weeks) of 250, 1250 or 5000 mg/d of oral ATP. For this purpose, we performed an experiment with a single acute dose of 5000 mg ATP on day 0 and repeated this experiment in all subjects on day 28. Results showed that, again, only the concentrations of uric acid, and not of ATP or any of the other metabolites, increased immediately after the single dose of 5000 mg ATP. Furthermore, there were no significant differences in concentration changes between days 0 and 28 for any of the measured compounds. Although NS, the data presented in Table 2 and Fig. 1(d) suggest that in the highest dosage group (5000 mg ATP), the rise in uric acid concentrations in response to administration of 5000 mg ATP on day 28 was smaller than on day 0. Since we found no increase in uric acid excretion in the urine samples collected on day 28 (Fig. 3), a more efficient excretion of uric acid is not likely to be the cause.
Previous studies in animals showed that, while systemic concentrations did not change, concentrations of ATP and its metabolites in the portal vein increased after prolonged ATP administration. Clearly, in our healthy volunteers, we could not sample portal blood to clarify the intestinal fate of ATP(Reference Kichenin, Seman and Decollogne12).
With the present study, we intended to explore whether oral administration of ATP by enteric-coated pellets could be used as an alternative to other means of ATP administration. Intravenous administration of ATP has been described to elicit marked health benefits and promote survival in cancer patients(Reference Agteresch, Dagnelie and van Der Gaast5, Reference Beijer, Hupperets and van den Borne6, Reference Beijer, Wijckmans and van Rossum24–Reference Haskell, Mendoza and Pisters31). Although it is feasible to intravenously administer ATP at home, some drawbacks include the increased risk of side effects, difficulty of achieving venous access and the need for medical supervision(Reference Agteresch, Dagnelie and van Der Gaast5, Reference Beijer, Hupperets and van den Borne6, Reference Beijer, Wijckmans and van Rossum24–Reference Haskell, Mendoza and Pisters31). Previous studies with oral administration of ATP have employed either ATP in solution or relatively large enteric-coated capsules. The latter has the drawback of being difficult to target a specific area of the small intestine due to gastric retention and unreliable enteric coating(Reference Jordan, Jurca and Abraham18). In the present study, we prevented these problems by using small enteric-coated pellets with a well-defined release profile, which in addition was tested in pilot experiments. Although the pellets were well tolerated and did release their contents, we could not demonstrate any increase in systemic ATP levels in contrast with intravenous ATP administration.
The present results have important implications. First, the reported efficacy of oral ATP supplements that are available through web stores or as registered drugs should be reconsidered. As we find no change in whole blood ATP concentrations at dosages several times exceeding the dosages administered via currently used oral ATP supplements, the present results justify the strong doubts regarding the claims on these supplements as being effective in providing ATP to the body. Secondly, the observed increases in uric acid concentrations in the present study may well explain some of the physiological effects reported after oral ATP administration in previous studies. Uric acid has a controversial reputation: it is at the same time thought to be (1) an important physiological antioxidant that reduces oxidative stress, (2) a normally innocent metabolic waste product that only causes gout or kidney stones if present in excessively high concentrations and (3) a causal factor for hypertension, obesity and vascular and renal diseases(Reference Krishnan, Kwoh and Schumacher32–Reference Masuo, Kawaguchi and Mikami35). With regard to the first hypothesis, there are indications that uric acid may have anti-inflammatory activities in conditions such as multiple sclerosis, Parkinson's disease and acute stroke(Reference Amaro, Planas and Chamorro36–Reference Rentzos, Nikolaou and Anagnostouli38). These may be partly due to the strong antioxidant capacity of uric acid. The use of oral ATP-containing supplements to increase uric acid concentrations might be of therapeutic value for these diseases. Secondly, the pro-inflammatory properties of uric acid crystals have long been known to be involved in gout. The uric acid deposits that occur predominately in joints after prolonged periods of excessively high concentrations of uric acid can eventually trigger an inflammatory response(Reference Cronsteinand and Terkeltaub39). Uric acid is also known to act as a ‘danger signal’, when it is released from necrotic cells(Reference Shi, Evans and Rock40). Hyperuricaemia is often defined as uric acid >71 mg/l (420 μmol/l) in men and >61 mg/l (360 μmol/l) in women(Reference Armstrong, Johnson and Campbell41, Reference Johnson, Kang and Feig42). In the present study, the blood concentrations of uric acid stayed below these values. Our data on creatinine, alkaline phosphatase, alanine aminotransferase, lactate dehydrogenase and creatine kinase also demonstrate that daily administration of 5000 mg ATP is safe. As for the third hypothesis, given the complex relationship of uric acid with other established cardiovascular risk factors, such as obesity, the metabolic syndrome, diabetes and especially hypertension, and due to the lack of prospective studies, it remains unclear whether uric acid is indeed a causal factor for these conditions(Reference Wheeler, Juzwishin and Eiriksdottir43, Reference Culleton, Larson and Kannel44).
In conclusion, the present study shows that a 4-week period of daily administration of ATP as enteric-coated pellets does not lead to increased plasma or blood ATP concentrations, nor is there any evidence of a metabolic adaptation in the uptake or metabolism of ATP. The present results cast serious doubt on the health claims of oral ATP supplements.
Acknowledgements
All the authors have contributed significantly to the work. To specify the authors' contribution to the supplied manuscript, E. J. C. M. C. was involved in writing, laboratory analyses, medical approval and the volunteer study; I. C. W. A. in drafting the manuscript; O. B. in measurements; C. V. in advice on and production of the pellets used; A. B. in manuscript preparation and advice; P. C. D. in manuscript preparation, drafting and advice. All the authors state that there were no conflicts of interest. The present research received no specific grant from any funding agency in the public, commercial or not-for-profit sectors.