Barker’s hypothesis( Reference Barker 1 ) about fetal programming stating that adult diseases are partly attributable to insufficient intra-uterine nutrition is now well accepted( Reference Charles, Delpierre and Breant 2 ). Nutrient deficiencies during pregnancy can induce changes in this critical period that will affect physiology and body composition in both fetal and postnatal development( Reference Charles, Delpierre and Breant 2 ). Among those changes, one of the most widely reported is suboptimal infant growth( Reference Charles, Delpierre and Breant 2 ). A common problem with lower birth weight or being born small-for-gestational-age (SGA) is the catch-up growth that follows in early childhood( Reference Okada, Takahashi and Nagano 3 ). Indeed, children recover their normal fat mass before they recover their normal height, which can lead to elevated adiposity and hormonal dysregulations that can induce metabolic health problems( Reference Okada, Takahashi and Nagano 3 ). Therefore, child growth, adiposity and metabolic health may be partly determined by prenatal nutritional status.
One nutrient of emerging interest is vitamin D, the deficiency of which is a nutritional issue extremely widespread around the globe( Reference Palacios and Gonzalez 4 ). There is no universally accepted definition of vitamin D deficiency. On the basis of circulating 25-hydroxyvitamin D (25(OH)D) concentration, different cut-offs have been used to define vitamin D status. The Institute of Medicine states that a serum 25(OH)D concentration <30 nmol/l is vitamin D deficiency( 5 ), whereas the Endocrine Society defines vitamin D deficiency as 25(OH)D <50 nmol/l( Reference Holick, Binkley and Bischoff-Ferrari 6 ). Vitamin D can be absorbed through alimentation or synthetised in the skin with sun exposure( Reference Naeem 7 ). As cutaneous synthesis under sunlight is responsible for the majority of vitamin D in circulation( Reference Holick and Chen 8 ), the problem is alarming in Canada where almost a third of the population had plasma concentrations of 25(OH)D <50 nmol/l( Reference Janz and Pearson 9 ). Vitamin D deficiency is common during pregnancy; almost 40 % of pregnant women had plasma 25(OH)D concentrations <50 nmol/l in a prospective multi-centre cohort study in Canada( Reference Wei, Audibert and Hidiroglou 10 ), and it represents a common public health problem( Reference McAree, Jacobs and Manickavasagar 11 ).
As vitamin D has an important role in placental metabolism( Reference Shin, Choi and Longtine 12 ) and metabolic health during pregnancy( Reference Palaniswamy, Williams and Jarvelin 13 ), our hypothesis is that low prenatal vitamin D status has an effect on infant growth, adiposity and metabolic health. As child growth and metabolic health are important for childhood well-being and long-term health outcomes, this systematic review and meta-analysis aimed to better understand the role of prenatal vitamin D status in child growth, adiposity and metabolic health.
Methods
Electronic literature search
To evaluate the overall effects of prenatal vitamin D status on child’s growth, adiposity and related metabolic disorders, literature searches on prenatal vitamin D status and newborn anthropometry, SGA, child’s growth and metabolic health disorders were conducted on electronic databases of the human literature in PubMed, up to July 2017 using ‘vitamin D’ and ‘pregnancy’ as keywords. All 2550 studies have been screened by their title and abstract and initially selected if they reported an association between prenatal vitamin D status and newborn anthropometry, more specifically birth weight, length, head circumference and SGA, as well as child’s growth, adiposity and metabolic health, more specifically child weight, length, BMI, fat mass or diabetes type 1. Additional articles have been searched through reviewing the reference lists of relevant articles. Only articles in English have been considered. When only the abstract was available, the author was contacted to provide the data.
Selection of studies
After screening the titles and abstracts, sixty articles were fully read. Twenty-three of them were excluded because they met at least one exclusion criterion: (a) they were reviews, case reports or comments; (b) maternal vitamin D levels were estimated according to their nutrient intake; or (c) the data are incomplete or impossible to merge with other studies. Of the remaining thirty-seven studies, seven were further excluded because they did not meet all the inclusion criteria. The inclusion criteria were as follows: (a) study design was a prospective or retrospective cohort study; (b) there were data on child growth, adiposity or metabolic health, such as weight, length, BMI, head circumstance, SGA, skinfold thickness, fat mass (g or %) and diabetes type 1; (c) study population was pregnant women without pre-existing chronic disease; (d) vitamin D status was indicated by 25(OH)D assays in maternal or cord blood samples taken before or at delivery; and (e) the study met the predefined methodological quality assessment criteria for non-randomised observational studies( Reference Wells 14 ). When data were incomplete, authors were contacted and asked to provide the needed data. Crozier et al.( Reference Crozier, Harvey and Inskip 15 ), Gale et al.( Reference Gale, Robinson and Harvey 16 ), Josefson et al.( Reference Josefson, Reisetter and Scholtens 17 ) and Leffelaar et al.( Reference Leffelaar, Vrijkotte and van Eijsden 18 ) provided us with additional useful data.
Two reviewers (C. S. and W. G. B.) independently searched the electronic literature, screened titles and abstracts and read full-length articles in order to make final inclusion or exclusion decisions. The most complete version of an article was selected when there were two different publications from the same study. Any disagreements were resolved by discussion with a third reviewer (S. Q. W.).
We evaluated the methodological quality of each study using Newcastle–Ottawa Scale (NOS)( Reference Wells 14 ). The NOS is a method recommended by the Cochrane Non-Randomized Studies Methods Working Group to evaluate the quality of the study. Points are assigned based on the selection process of cohorts (0–4 points), the comparability of the cohorts (0–2 points) and the identification of the exposures and the outcomes of research participants (0–3 points). A score of at least 7 out of 9 was defined as high quality.
Data collection and analysis
The primary exposure variable was vitamin D deficiency. The dependent variables were offspring growth and adiposity outcomes. Some outcomes were continuous variables, such as birth weight, birth length, head circumference, BMI and fat mass, and other outcomes were dichotomous variables, such as SGA and diabetes type 1. To merge the results from various studies in meta-analysis, we constructed two-by-two tables of prenatal vitamin D status v. the presence or absence of adverse offspring outcomes for dichotomous outcomes. For continuous outcomes, the mean values and standard deviations and number of subjects were extracted based on prenatal vitamin D status.
No universal consensus exists in the scientific community concerning the definition of vitamin D deficiency. When different cut-offs were presented in a study, we decided to use the lowest cut-off point as we were interested in studying the effects of vitamin D deficiency. In this meta-analysis, for any outcome with different cut-offs, subgroup analysis was performed. All individual studies reporting the same outcome were pooled to calculate the overall effect.
To merge data for continuous outcomes, the mean values and standard deviations and the number of subjects were needed from each group (vitamin D deficiency and vitamin D non-deficiency) in each study. The mean difference (MD) was calculated using the inverse variance method. To merge dichotomous outcomes, the number of patients with the outcome (SGA and diabetes type 1) and the total in each group (vitamin D deficiency v. vitamin D non-deficiency) were needed. The Mantel–Haenszel method was then used to present the effect as OR and 95 % CI. For both continuous and dichotomous outcomes, when the heterogeneity was significant, defined as I 2 of >50 %, the analysis model was changed from fixed effect to random effects. Forest plots were used to illustrate the point estimate with 95 % CI. Data meta-analysis was performed using the Review Manager (RevMan) 5.3( 19 ).
Two independent reviewers (C. S., W. G. B.) went through the process of data extraction and calculation. We followed the Meta-Analysis of Observational Studies in Epidemiology (MOOSE) guidelines( Reference Stroup, Berlin and Morton 20 ).
Results
In all, thirty studies involving a total of 35 032 mother–offspring pairs were included in this meta-analysis. Among the sixty preselected articles, twenty-three were excluded because they had insufficient data. Then, seven of the remaining thirty-seven articles were excluded because they did not meet all inclusion criteria: six of them were not observational studies as they used supplementation( Reference Brekke and Ludvigsson 21 – Reference Sablok, Batra and Thariani 26 ) and one of them had a population of pregnant women with a chronic disease – diabetes type 2( Reference Savvidou, Akolekar and Samaha 27 ). The details of the process of literature search and selection of studies are presented in Fig. 1 – study eligibility flow chart. Table 1 shows characteristics of the included studies: study design, study setting (country), sample size, gestational age when the blood sample was taken, season when the blood sample was taken (percentage of winter), assay method used to determine the 25(OH)D level (including chemiluminescent immunometric assay, electrochemiluminescence immunoassay, ELISA, liquid chromatography-tandem MS, RIA and HPLC), 25 (OH)D cut-off the authors used (when there were more than one, the lowest one was chosen) and child outcomes. Four studies( Reference Josefson, Reisetter and Scholtens 17 , Reference Dalgard, Petersen and Steuerwald 35 , Reference Morgan, Dodds and Langille 43 , Reference Weiler, Fitzpatrick-Wong and Veitch 51 ) measured cord blood 25(OH)D levels and the remaining studies measured maternal blood 25(OH)D levels. Cord blood vitamin D levels were lower than maternal blood levels, but they were highly correlated( Reference Mithal, Wahl and Bonjour 54 ). We also performed sensitivity analysis (excluding the studies with only cord blood data) and similar results were obtained. There was no evidence of publication bias.

Fig. 1 Flow chart of study selection process in a systematic review. NOS, Newcastle–Ottawa Scale.
Table 1 Characteristics of the included studies
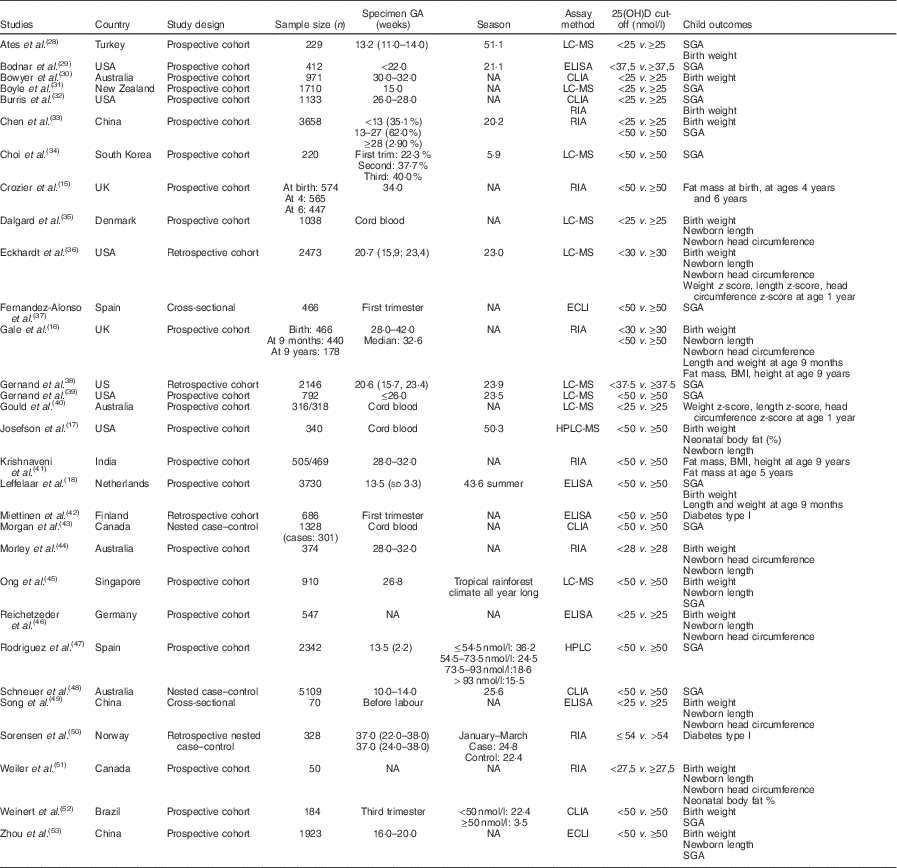
GA, gestational age at the time of specimen sampling; 25(OH)D, 25-hydroxyvitamin D; LC-MS, liquid chromatography–tandem MS; SGA, small-for-gestational-age; NA, not available; CLIA, chemiluminescent immunometric assay; ECLI, electrochemiluminescence immunoassay; season, season of blood sampling (% winter).
Among the finally selected thirty articles, sixteen studies( Reference Gale, Robinson and Harvey 16 – Reference Leffelaar, Vrijkotte and van Eijsden 18 , Reference Ates, Sevket and Ozcan 28 , Reference Bowyer, Catling-Paull and Diamond 30 , Reference Burris, Rifas-Shiman and Camargo 32 , Reference Chen, Fu and Hao 33 , Reference Dalgard, Petersen and Steuerwald 35 , Reference Eckhardt, Gernand and Roth 36 , Reference Morley, Carlin and Pasco 44 – Reference Reichetzeder, Chen and Foller 46 , Reference Song, Si and Liu 49 , Reference Weiler, Fitzpatrick-Wong and Veitch 51 – Reference Zhou, Su and Liu 53 ) involving 18 096 participants reported birth weight. Vitamin D deficiency definition was different in individual studies. We used a 25(OH)D cut-off of 25 nmol/l in seven studies( Reference Ates, Sevket and Ozcan 28 , Reference Bowyer, Catling-Paull and Diamond 30 , Reference Burris, Rifas-Shiman and Camargo 32 , Reference Chen, Fu and Hao 33 , Reference Dalgard, Petersen and Steuerwald 35 , Reference Reichetzeder, Chen and Foller 46 , Reference Song, Si and Liu 49 ), a cut-off of 27·5 nmol/l in one study( Reference Weiler, Fitzpatrick-Wong and Veitch 51 ), a cut-off of 28 nmol/l in one study( Reference Morley, Carlin and Pasco 44 ), a cut-off of 30 nmol/l in three studies( Reference Gale, Robinson and Harvey 16 , Reference Leffelaar, Vrijkotte and van Eijsden 18 , Reference Eckhardt, Gernand and Roth 36 ) and a cut-off of 50 nmol/l in four studies( Reference Josefson, Reisetter and Scholtens 17 , Reference Ong, Quah and Tint 45 , Reference Weinert, Reichelt and Schmitt 52 , Reference Zhou, Su and Liu 53 ). Fig. 2 shows the summary MD of the association between low prenatal 25(OH) D levels and birth weight. The overall summary MD of birth weight (g) was −100·69 (95 % CI −162·25, −39·13) (P=0·001). There was significant heterogeneity across studies (τ²=123 58·50; χ 2=180·67; I 2=92 %; P<0·00001). Subgroup analysis (online Supplementary Fig. S1) shows a significant association between prenatal 25(OH)D<30 nmol/l and a lower birth weight (MD −111·26; 95 % CI −139·60, −82·92; P<0·00001). There was no significant heterogeneity. Subgroup analysis also shows significant association between prenatal 25(OH)D<25 nmol/l and a lower birth weight (g) (MD−212·43; 95 % CI −408·90, −15·96; P=0·03), but this association would become non-significant if accounting for multiple tests. Heterogeneity was significant.

Fig. 2 Forest plots of summary mean difference (MD) (g) of birth weight between prenatal vitamin D deficiency and vitamin D non-deficiency.
In all, ten studies( Reference Gale, Robinson and Harvey 16 , Reference Josefson, Reisetter and Scholtens 17 , Reference Dalgard, Petersen and Steuerwald 35 , Reference Eckhardt, Gernand and Roth 36 , Reference Morley, Carlin and Pasco 44 – Reference Reichetzeder, Chen and Foller 46 , Reference Song, Si and Liu 49 , Reference Weiler, Fitzpatrick-Wong and Veitch 51 , Reference Zhou, Su and Liu 53 ) involving 8191 mother–newborn pairs reported the relationship between prenatal vitamin D and newborn length/height. As some studies had data on the length at birth( Reference Gale, Robinson and Harvey 16 , Reference Josefson, Reisetter and Scholtens 17 , Reference Eckhardt, Gernand and Roth 36 , Reference Morley, Carlin and Pasco 44 – Reference Reichetzeder, Chen and Foller 46 , Reference Song, Si and Liu 49 , Reference Zhou, Su and Liu 53 ) while others had data on the length at approximately 2 weeks after birth( Reference Dalgard, Petersen and Steuerwald 35 , Reference Weiler, Fitzpatrick-Wong and Veitch 51 ), the outcome of interest is newborn (0–28 d) length instead of birth length. There was no association between prenatal vitamin D status and newborn length (MD −0·00; 95 % CI −0·32, 0·31; P=0·98). There was significant heterogeneity (τ 2=0·18; χ 2=48·89; I 2=82 %; P<0·00001).
Seven studies( Reference Gale, Robinson and Harvey 16 , Reference Dalgard, Petersen and Steuerwald 35 , Reference Eckhardt, Gernand and Roth 36 , Reference Morley, Carlin and Pasco 44 , Reference Reichetzeder, Chen and Foller 46 , Reference Song, Si and Liu 49 , Reference Weiler, Fitzpatrick-Wong and Veitch 51 ) on newborn head circumference involving 5018 participants were included in this meta-analysis (vitamin D deficiency cut-off of 30 nmol/l( Reference Gale, Robinson and Harvey 16 , Reference Eckhardt, Gernand and Roth 36 ), 28 nmol/l( Reference Morley, Carlin and Pasco 44 ), 27·5 nmol/l( Reference Weiler, Fitzpatrick-Wong and Veitch 51 ) or 25 nmol/l( Reference Dalgard, Petersen and Steuerwald 35 , Reference Reichetzeder, Chen and Foller 46 , Reference Song, Si and Liu 49 )). No association between prenatal vitamin D status and newborn head circumference was found (MD −0·11; 95 % CI −0·30, 0·09; P=0·27). There was significant heterogeneity (τ 2=0·04; χ 2=17·37; I 2=65 %; P=0·008).
The association between prenatal vitamin D status and risk of SGA is presented in Fig. 3. There were a total of sixteen studies( Reference Leffelaar, Vrijkotte and van Eijsden 18 , Reference Ates, Sevket and Ozcan 28 , Reference Bodnar, Catov and Zmuda 29 , Reference Boyle, Thorstensen and Mourath 31 – Reference Choi, Kim and Yoo 34 , Reference Fernandez-Alonso, Dionis-Sanchez and Chedraui 37 – Reference Gernand, Simhan and Caritis 39 , Reference Morgan, Dodds and Langille 43 , Reference Ong, Quah and Tint 45 , Reference Rodriguez, Garcia-Esteban and Basterretxea 47 , Reference Schneuer, Roberts and Guilbert 48 , Reference Weinert, Reichelt and Schmitt 52 , Reference Zhou, Su and Liu 53 ) involving 26 292 patients. Vitamin D deficiency was defined using circulating 25(OH)D level of 50 nmol/l as the cut-off in eight studies( Reference Chen, Fu and Hao 33 , Reference Choi, Kim and Yoo 34 , Reference Fernandez-Alonso, Dionis-Sanchez and Chedraui 37 , Reference Morgan, Dodds and Langille 43 , Reference Ong, Quah and Tint 45 , Reference Rodriguez, Garcia-Esteban and Basterretxea 47 , Reference Weinert, Reichelt and Schmitt 52 , Reference Zhou, Su and Liu 53 ), 37·5 nmol/l in two studies( Reference Bodnar, Catov and Zmuda 29 , Reference Gernand, Simhan and Klebanoff 38 ), 30 nmol/l( Reference Leffelaar, Vrijkotte and van Eijsden 18 , Reference Gernand, Simhan and Caritis 39 ) in two studies and of 25 nmol/l in four studies( Reference Ates, Sevket and Ozcan 28 , Reference Boyle, Thorstensen and Mourath 31 , Reference Burris, Rifas-Shiman and Camargo 32 , Reference Schneuer, Roberts and Guilbert 48 ). Vitamin D deficiency was associated with an increased risk of SGA with an overall OR of 1·55 (95 % CI 1·16, 2·07) (P=0·003). There was significant heterogeneity (τ 2=0·26; χ 2=102·22; I 2=85 %, P<0·00001).
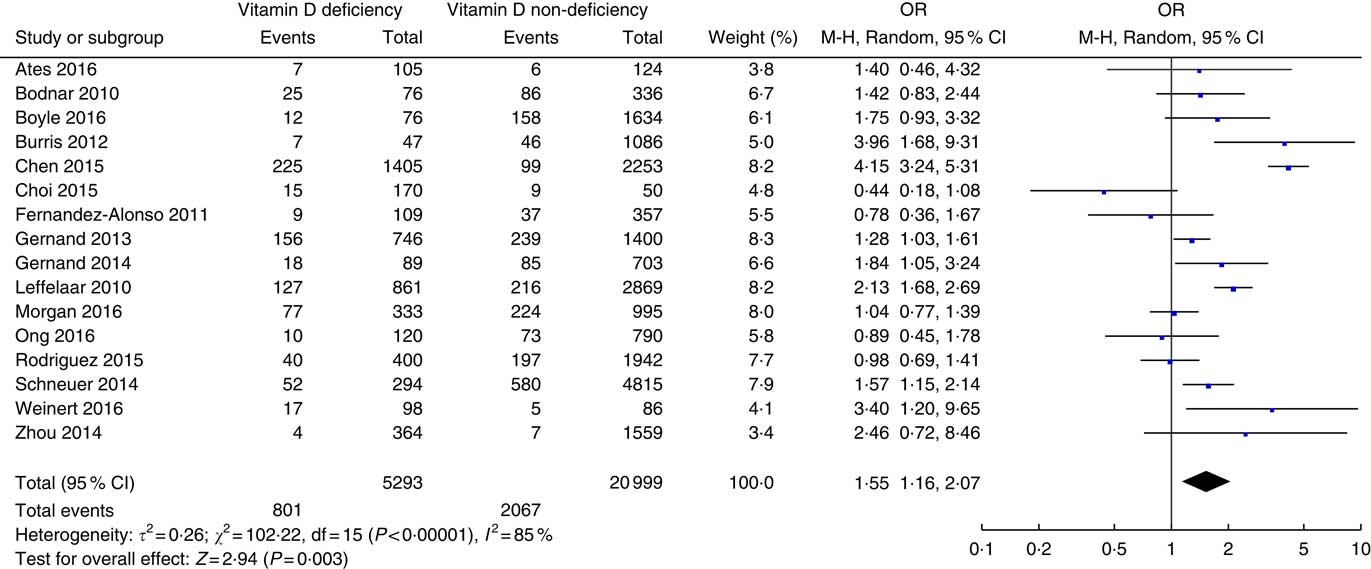
Fig. 3 Forest plots of summary crude OR of the association between prenatal low 25-hydroxyvitamin D levels and small-for-gestational-age.
Two studies( Reference Gale, Robinson and Harvey 16 , Reference Leffelaar, Vrijkotte and van Eijsden 18 ) involving 4170 infants reported the association between prenatal vitamin D status and infant growth at the age of 9 months (Fig. 4). Compared with prenatal adequate vitamin D levels, infants with low prenatal vitamin D status had heavier weight (g) (MD 119·75; 95 % CI 32·97, 206·52; P=0·007). There was no association between prenatal vitamin D status and length (cm) at 9 months (MD −0·09; 95 % CI −0·30, 0·13; P=0·43). No significant heterogeneity was detected across studies.
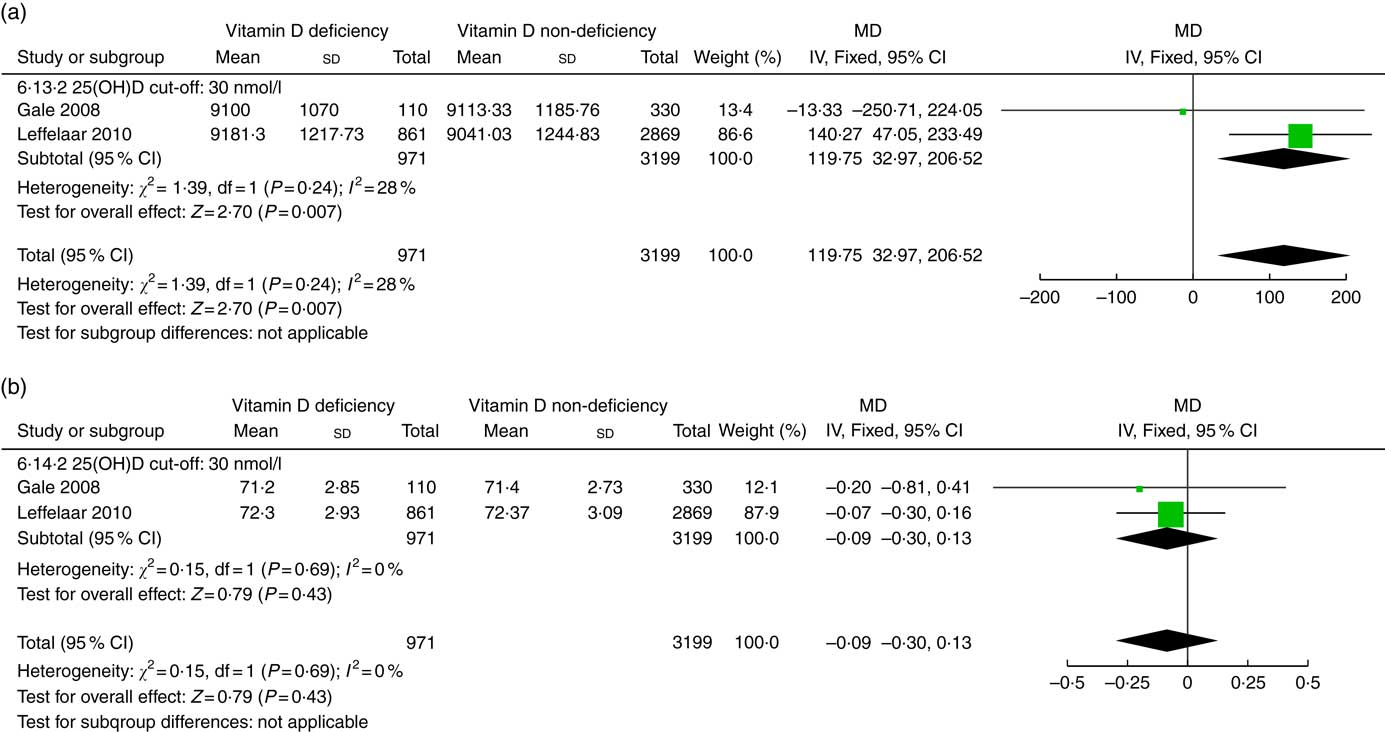
Fig. 4 Forest plots of summary mean difference (MD) of anthropometry in infants at the age of 9 months between prenatal vitamin D deficiency and vitamin D non-deficiency. (a). The association between prenatal vitamin D deficiency and infant weight (g) at the age of 9 months. (b). The association between prenatal vitamin D deficiency and infant length (cm) at the age of 9 months. 25(OH)D, 25-hydroxyvitamin D.
Two studies( Reference Eckhardt, Gernand and Roth 36 , Reference Gould, Anderson and Yelland 40 ) examined the relationship between prenatal vitamin D status and infant growth at the age of 1 year, including weight-for-age z score, length-for-age z score and head-circumference-for-age z score. The sample size was 2791 for length and head circumference, and 2789 for weight. Prenatal vitamin D deficiency was associated with lower head-circumference-for-age z score at the age of 1 year (MD −0·27; 95 % CI −0·37, −0·17; P<0·00001). There was no significant association between prenatal vitamin D status and weight-for-age z score (MD −0·09; 95 % CI −0·18, 0·01; P=0·06) or the length-for-age z score (MD −0·05; 95 % CI −0·38, 0·27; P=0·75). The heterogeneity is significant for length-for-age (τ 2=0·04; χ 2=3·23; I 2=69 %; P=0·07), whereas there was no significant heterogeneity for weight-for-age z score or head-circumference-for-age z score.
Two studies( Reference Crozier, Harvey and Inskip 15 , Reference Krishnaveni, Veena and Winder 41 ) examined maternal vitamin D status in pregnancy and adiposity in the offspring at birth and 4–6 years of age. Krishnaveni et al.( Reference Krishnaveni, Veena and Winder 41 ) measured fat mass at the age of 5 years and Crozier et al. measured fat mass at birth and at ages 4 and 6 years. Crozier et al.( Reference Crozier, Harvey and Inskip 15 ) reported that low maternal vitamin D status was associated with lower fat mass at birth and higher fat mass at the age of 6 years. No significant association was found at the age of 4( Reference Crozier, Harvey and Inskip 15 ) or 5 years( Reference Krishnaveni, Veena and Winder 41 ). The data could not be pooled as adiposity was not measured at the same age.
Two studies, Gale 2008( Reference Gale, Robinson and Harvey 16 ) and Krishnaveni 2011( Reference Krishnaveni, Veena and Winder 41 ), compared height, BMI and fat mass at the age of 9 years according to prenatal vitamin D status (deficiency v. non-deficiency). The total sample size for these three measures was 647. There were no associations between prenatal vitamin D status and height, BMI and fat mass at 9 years of age. The summary MD were −0·11 (95 % CI −1·07, 0·84) (P=0·81) for height, −0·34 (95 % CI −0·78, 0·10) (P=0·13) for BMI and −0·40 (95 % CI −1·36, 0·57) (P=0·42) for fat mass. There was no evidence of heterogeneity for height. The heterogeneity was significant for BMI (τ 2=0·07; χ 2=2·92; I 2=66 %; P=0·09) and fat mass (τ 2=0·41; χ 2=7·01; I 2=86 %; P=0·008).
The risk of diabetes type 1 in prenatal vitamin D-deficient mother–child pairs has been compared with vitamin D-non-deficient mother–child pairs in two studies( Reference Miettinen, Reinert and Kinnunen 42 , Reference Sorensen, Joner and Jenum 50 ), including 1014 mother–child pairs. Sorensen et al.( Reference Sorensen, Joner and Jenum 50 ) showed that low maternal 25(OH)D level was associated with increased risk of diabetes type 1 in children (the mean age at diabetes diagnosis was 9 years), whereas Miettinen et al. ( Reference Miettinen, Reinert and Kinnunen 42 ) did not observe any difference between 25(OH)D levels of the case and control mothers (the mean age at diabetes diagnosis was 3 years). The meta-analysis results show that low maternal vitamin D status during pregnancy was not associated with risk of diabetes type 1 in the offspring. The summary crude OR was 1·25 (95 % CI 0·78, 2·02). There was significant heterogeneity (τ 2=0·08; χ 2=2·65; I 2=62 %; P=0·10).
Discussion
The main findings of this systematic review and meta-analysis was that low prenatal vitamin D status was associated with lower birth weight and increased risk of SGA. Interestingly, low prenatal vitamin D status was associated with greater weight in infants at the age of 9 months, but no significant difference was observed in length, indicating that low prenatal vitamin D status may be linked to accelerated growth and adiposity in early postnatal life. However, prenatal vitamin D deficiency was not related with risk of childhood diabetes type 1.
Previous systematic reviews of observational studies mainly focused on vitamin D during pregnancy and maternal and neonatal outcomes. Aghajafari’s meta-analysis found an association between prenatal vitamin D deficiency and lower birth weight and elevated risks of SGA, as well as other maternal outcomes( Reference Aghajafari, Nagulesapillai and Ronksley 55 ). Nassar’s review also showed similar results with an increased risk of SGA associated with maternal vitamin D deficiency( Reference Nassar, Halligan and Roberts 56 ). Our previous systematic review found that maternal low vitamin D status was associated with increased risk of adverse pregnancy outcomes including preeclampsia, gestational diabetes, preterm birth and SGA( Reference Wei, Qi and Luo 57 ). The present review focuses on the impact of low prenatal vitamin D status on offspring growth, adiposity and metabolic health in the literature up to 2017/07.
The results of this meta-analysis show that low maternal vitamin D status was associated with higher weight at the age of 9 months, indicating that maternal low vitamin D status may be associated with an accelerated postnatal growth. Accelerated growth during infancy has been reported to be associated with childhood adiposity( Reference Ong, Emmett and Northstone 58 ) and the risk of subsequent overweight( Reference Ong, Emmett and Northstone 58 ) or obesity( Reference Baird, Fisher and Lucas 59 ). However, results have to be interpreted cautiously. The mean weight difference in infants at the age of 9 months was only 119·75 g (1·3 % of infant weight), which may not be clinically relevant. Although there is a lack of data on what percentage of weight difference during infancy can increase odds of overweight or obesity in later life, further research is needed in this area. In addition, confounding factors such as dietary intake and breast-feeding during infancy may have affected this relationship. Adiposity was observed to be elevated at child at age 6 years in prenatally vitamin D-deficient mother–offspring pairs( Reference Crozier, Harvey and Inskip 15 ).
An association between maternal vitamin D deficiency and risk of diabetes type 1 in children was not observed in this meta-analysis. However, there were only two studies that examined the link between maternal vitamin D status and diabetes in offspring. A nested case–control study( Reference Sorensen, Joner and Jenum 50 ) showed the association, whereas another study( Reference Miettinen, Reinert and Kinnunen 42 ) did not. However, the mean age at diagnosis for diabetes was too young (only about 3·4 years) in the Miettinen study( Reference Miettinen, Reinert and Kinnunen 42 ), whereas the peak incidence for diabetes in children is at age 10–14 years, and thus this study cannot exclude the possibility that maternal vitamin D deficiency may contribute to the risk of diabetes type 1. More longitudinal cohort studies with longer follow-up of children (age 10–14 years) are needed to clarify this association.
The underlying mechanisms in the association between maternal vitamin D during pregnancy and infant growth and adiposity are biologically plausible. Indeed, vitamin D promotes Ca and P absorption, which is essential for the mineralisation process during fetal bone formation( Reference DeLuca 60 ). During pregnancy, the placenta synthesises vitamin D to help achieve a higher concentration in circulation( Reference Shin, Choi and Longtine 12 ). As Ca reaches fetal circulation by crossing the placental barrier, the mineralisation of fetus bones depends on the mother’s Ca absorption. Therefore, vitamin D deficiency in the mother can induce Ca deficiency in the fetus, which may affect bone formation in the fetus( Reference Viljakainen, Saarnio and Hytinantti 61 ). In addition, intra-uterine exposure to low vitamin D status negatively affects fetal skeletal muscle( Reference Hines, Coffey and Starkey 62 ) and adiposity development( Reference Crozier, Harvey and Inskip 15 ). The adverse effects of prenatal vitamin D deficiency on fetal bone( Reference Viljakainen, Saarnio and Hytinantti 61 ), muscle( Reference Hines, Coffey and Starkey 62 ) and fat( Reference Crozier, Harvey and Inskip 15 ) development might be responsible for lower birth weight or SGA. Vitamin D also helps to reduce inflammatory response during pregnancy and contributes to embryo implantation( Reference Shin, Choi and Longtine 12 ). Moreover, it is widely accepted that vitamin D is mainly stored in adipose tissue( Reference Abbas 63 ). Numerous studies have reported a correlation between vitamin D deficiency and obesity( Reference Pereira-Santos, Costa and Assis 64 ). However, the causal relationship between vitamin D status and obesity remains unclear. Vitamin D deficiency might play a role in the development of obesity because decreased circulating 25(OH)D levels could result from a modification in vitamin D metabolism occurring during obesity development( Reference Landrier, Karkeni and Marcotorchino 65 ). Indeed, modifications in the expression of genes encoding key enzymes of vitamin D metabolism have been reported in the adipose tissue of obese people( Reference Wamberg, Christiansen and Paulsen 66 ). Accelerated postnatal growth may promote adiposity( Reference Okada, Takahashi and Nagano 3 ) and thus an elevated fat mass. Our finding that prenatally vitamin D-deficient infants had lower weight at birth but heavier weight at 9 months of age is consistent with a recent study from the animal model( Reference Belenchia, Johnson and Ellersieck 67 ), indicating that prenatal vitamin D status may affect adiposity in offspring.
Some limitations of this review should be noted. First, there is no universal definition of vitamin D deficiency, and various definitions were used in individual studies. However, we performed overall analysis based on vitamin D deficiency and non-deficiency, as well as subgroup analysis according to the specific cut-off of 25(OH)D level, and the findings were similar. Second, the observed associations between prenatal vitamin D status and offspring outcomes could be affected by potential confounding factors. However, most adjusted OR were similar to the crude estimates. Third, the variability in the assay methods for 25(OH)D may have affected the results. Finally, loss to follow-up may be an issue for cohort studies on child long-term health outcomes.
This systematic review has strengths. It was the first to summarise the evidence on prenatal vitamin D status on offspring growth, adiposity and metabolic health. It included the most recent studies and followed the MOOSE guidelines( Reference Stroup, Berlin and Morton 20 ). The quality of the review data in this review depends on the methodological quality of the included studies. Each study was evaluated using the NOS, and only high-quality observational studies were included in the meta-analysis.
The evidence from this systematic review indicates that low prenatal 25(OH)D status has an impact on fetal growth and low prenatal vitamin D status may be associated with infant adiposity. Further confirmation of these findings in large longitudinal cohorts is warranted. Carefully defined large multi-centre randomised clinical trials of vitamin D supplementation in pregnancy for improving infant growth need to be implemented to better define the risks and benefits. Given the high prevalence of vitamin D deficiency in pregnant women, the findings could have substantial public health implications.
Acknowledgements
Dr S. Q. W. was supported by an award from the Fonds de Recherche en Sante du Quebec.
The authors’ contributions were as follows – S. Q. W.: designed and supervised the study (conception and design; analysis and interpretation of data; revising and finalising the paper). C. S.: acquisition, analysis and interpretation of data, and writing and revising the paper. W. G. B. acquisition, analysis and interpretation of data, and revising the article. L. L., N. T., P. J., Z.-C. L., F. A. and A. M. N.: revisions for intellectual content of the manuscript. All authors contributed to the interpretation of data and read and approved the submitted manuscript.
The authors declare that there are no conflicts of interest.
Supplementary Material
For supplementary material/s referred to in this article, please visit https://doi.org/10.1017/S0007114517003646