Oxidative stress is defined as an increased generation of reactive oxygen species or a reduced ability to deactivate them. It is well known that oxidative stress is involved in the development of many chronic diseases such as cancer( Reference Reuter, Gupta and Chaturvedi 1 ), CVD( Reference Heitzer, Schlinzig and Krohn 2 ) and diabetes( Reference Ceriello and Motz 3 ). These diseases are considered as public health problems in both developed and developing countries. Many researches have focused on the relationship between oxidative stress and the above-mentioned diseases. One of the main factors involved in oxidative stress reduction is increased antioxidant potential. Numerous studies have investigated the antioxidant properties of some vitamins such as vitamin E( Reference Traber and Atkinson 4 ), vitamin C( Reference Padayatty, Katz and Wang 5 ) and carotenoids( Reference Young and Lowe 6 ) and their effects on human health. One of the neglected antioxidant vitamins is riboflavin, which acts as a coenzyme for redox enzymes in FAD and FMN forms. Many studies have examined the effects of riboflavin on various diseases such as cataract( Reference Cumming, Mitchell and Smith 7 ), night blindness( Reference Graham, Haskell and Pandey 8 ), some cancers (oesophageal, cervical and colorectal)( Reference Siassi and Ghadirian 9 – Reference de Vogel, Dindore and van Engeland 11 ), anaemia( Reference Powers, Hill and Mushtaq 12 ) and CVD( Reference Powers 13 ). One of the roles through which riboflavin can have a potential effect on human health is that as an antioxidant, which has not been investigated completely. Herein, studies that have examined the antioxidant properties of riboflavin and its effect on oxidative stress reduction are reviewed. PubMed and MEDLINE databases were searched for the published studies. The keywords used were riboflavin, oxidative stress, antioxidation, lipid peroxidation, antioxidant enzymes and glutathione peroxidase (GPx). The papers published before August 2012 are reviewed herein.
In this review, two aspects of the antioxidant properties of riboflavin are considered: (1) the role of riboflavin in the prevention of lipid peroxidation and (2) and the effect of riboflavin on the attenuation of reperfusion oxidative injury. Human and animal studies are summarised in Table 1.
Table 1 Summary of the human and animal studies reviewed
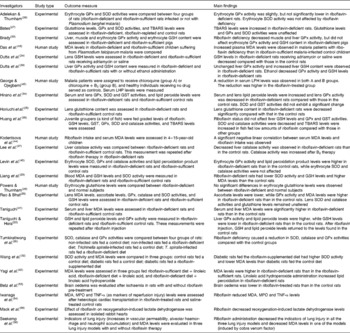
GPx, glutathione peroxidase; SOD, superoxide dismutase; TBARS, thiobarbituric acid-reactive substances; GSH, reduced glutathione; MDA, malondialdehyde; LHP, lipid hydroperoxide; GST, glutathione S-transferase; MPO, myeloperoxidase.
Riboflavin at a glance
Riboflavin was discovered by Blyth in 1872 as a yellow fluorescent pigment in milk( Reference Said, Ross, Shils, Shike, Ross, Caballero and Cousins 14 ), but the vitamin property of this pigment was not established until the early 1930s( Reference Northrop-Clewes and Thurnham 15 ). Riboflavin is essential for nutrient metabolism and also for antioxidant protection( Reference Gallagher, Mahan, Escott-Stump, Raymond and Krause 16 ). Plants and some micro-organisms can synthesise riboflavin; however, it is an essential nutrient for human health and should be provided by the diet( Reference Northrop-Clewes and Thurnham 15 ). The Food and Nutrition Board (FNB) has recommended a daily intake of 0·3–0·4 mg riboflavin/d for infants, 0·5–0·9 mg riboflavin/d for children, 1·3 mg riboflavin/d for adolescents, and 1·4 mg riboflavin/d during pregnancy and 1·6 mg riboflavin/d during lactation for adults( Reference Gallagher, Mahan, Escott-Stump, Raymond and Krause 16 ). Riboflavin-rich foods are eggs, lean meat, milk and leafy vegetables( Reference Said, Ross, Shils, Shike, Ross, Caballero and Cousins 14 , Reference Northrop-Clewes and Thurnham 15 ).
Riboflavin deficiency is more prevalent in underdeveloped populations with a low intake of dairy products and meat; however, a higher prevalence of low serum riboflavin levels has also been reported in some developed countries such as the USA and the UK( Reference Powers, Hill and Mushtaq 12 ). People with some cancers, congenital heart disease and excessive alcohol intake are at a greater risk of riboflavin deficiency( Reference Said, Ross, Shils, Shike, Ross, Caballero and Cousins 14 ). As riboflavin is destroyed by UV light exposure, UV therapy in infants with hyperbilirubinaemia could cause riboflavin deficiency( Reference Gallagher, Mahan, Escott-Stump, Raymond and Krause 16 ). When riboflavin supplementation is needed, an amount that is five to ten times the daily recommended amount is appropriate( Reference Said, Ross, Shils, Shike, Ross, Caballero and Cousins 14 ). No toxic or adverse effects of intake of high riboflavin doses by humans have been reported so far( Reference Said, Ross, Shils, Shike, Ross, Caballero and Cousins 14 , Reference Gallagher, Mahan, Escott-Stump, Raymond and Krause 16 ). However, it can be suggested that a high dose of riboflavin could cause an imbalance in the antioxidant state of human body. However, there is no strong evidence in this area, recommending further investigations to clarify the possible adverse effects of riboflavin intake in high amounts.
Role of riboflavin in the prevention of lipid peroxidation
Riboflavin as the glutathione reductase coenzyme
Glutathione reductase (GR) requires riboflavin in the FAD coenzyme form for its activity( Reference Gallagher, Mahan, Escott-Stump, Raymond and Krause 16 ). GR converts oxidised glutathione to the reduced form( Reference Dringen, Gutterer and Hirrlinger 17 ) (Fig. 1). FAD transports hydrogen from NADPH to oxidised glutathione to convert it into the reduced form( Reference Schulz, Schirmer and Pai 18 ) (Fig. 2). Reduced glutathione acts as an endogenous antioxidant in different cell types( Reference Pompella, Visvikis and Paolicchi 19 ) and deactivates reactive oxygen species. Through its action, this peptide is deactivated as it is converted to the oxidised form( Reference Dringen, Gutterer and Hirrlinger 17 ). Therefore, oxidised glutathione should be reduced by GR again to recover its antioxidant properties, the process in which riboflavin has a key role. Consequently, there is a possibility that riboflavin deficiency could affect the antioxidant properties of glutathione and lead to an impaired antioxidant potential of cells. One of the most important antioxidant activities of glutathione is the deactivation of peroxides such as hydroperoxide. This activity of glutathione is mediated by the action of GPx( Reference Hayes and McLellan 20 ). GPx transfers a hydrogen ion from reduced glutathione to lipid peroxide and produces oxidised glutathione and alcohol( Reference Mulherin, Thurnham and Situnayake 21 ). According to the mentioned mechanisms, it is expected that riboflavin deficiency could increase lipid peroxidation.
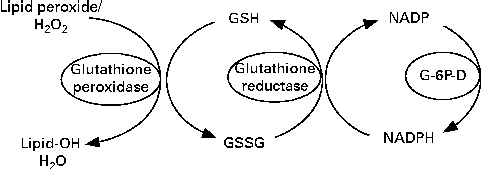
Fig. 1 Conversion of oxidised glutathione (GSSG) to the reduced form (GSH) by glutathione reductase. Glutathione reductase requires riboflavin in the FAD coenzyme form for its activity. G-6P-D, glucose-6-phosphate dehydrogenase.

Fig. 2 Transportation of hydrogen from reduced NADPH to oxidised glutathione (GS–SG; GSSG) by FAD for conversion into the reduced form (GSH). Enz, enzyme. (A colour version of this figure can be found online at http://www.journals.cambridge.org/bjn).
Effect of riboflavin status on glutathione content in tissues
The effect of riboflavin status on reduced glutathione content in tissues has been investigated in a limited number of studies. Taniguchi & Hara( Reference Taniguchi and Hara 22 ) reported that liver reduced glutathione levels are decreased in riboflavin deficiency in rats. In an experimental study, a reduction in reduced glutathione levels was observed in rats fed a riboflavin-deficient diet for over 6 weeks( Reference Liang, Liu and Xu 23 ). Similar results have been reported by some other studies( Reference Dutta, Rivlin and Pinto 24 , Reference Horiuchi, Hirano and Ono 25 ). However, to our knowledge, there are a limited number of human studies that have examined the effect of riboflavin on reduced glutathione status. The only human study published has reported no significant difference in erythrocyte glutathione concentrations between normal and riboflavin-deficient subjects( Reference Powers and Thurnham 26 ). Furthermore, some animal studies also did not report any change in glutathione content in different tissues (such as the liver, lens and erythrocytes) in riboflavin-deficient animals( Reference Bates 27 – Reference Rao and Bhat 29 ). A mechanism for the unchanged tissue glutathione content in riboflavin deficiency could be the increased biosynthesis of glutathione from its precursor amino acids as a compensatory action( Reference Kaplowitz, Aw and Ookhtens 30 ). Another possible mechanism could be the ability of GR to maintain reduced glutathione concentrations in tissues even at low activity levels( Reference Rogers and Augusteyn 31 ).
Effect of riboflavin status on the activity of antioxidant enzymes
As expected, some reports have indicated that riboflavin status can affect the activity of antioxidant enzymes including GPx, superoxide dismutase (SOD) and catalase. The results of a study that has investigated the effect of riboflavin therapy on diabetic cardiomyopathy indicated that riboflavin can increase SOD activity in the heart tissue( Reference Wang, Li and Lu 32 ). A reduction in liver and muscle GPx activity in riboflavin-deficient pigs was reported by Brady et al. ( Reference Brady, Brady and Parsons 33 ). However, erythrocyte GPx activity was not found to be affected by riboflavin deficiency in this study. Another study that has investigated the effect of dietary riboflavin on the antioxidant defence mechanism of fish has reported a significant reduction in the SOD and catalase activities of riboflavin-deficient (12 weeks) fish compared with those of the control fish( Reference Huang, Tian and Wu 28 ). Moreover, some other animal studies have reported a similar effect of riboflavin on SOD( Reference Liang, Liu and Xu 23 , Reference Hirano, Hamajima and Horiuchi 34 , Reference Tumkiratiwong, Tungtrongchitr and Migasena 35 ), GPx( Reference Tumkiratiwong, Tungtrongchitr and Migasena 35 , Reference Wang, Mei and Chen 36 ) and catalase( Reference Tumkiratiwong, Tungtrongchitr and Migasena 35 , Reference Lee, Ye and Jones 37 ) activities. However, some animal studies did not observe any association between riboflavin status and antioxidant enzyme activity( Reference Adelekan and Thurnham 38 , Reference Dutta, Seirafi and Halpin 39 ). The results of the study carried out by Adelekan & Thurnham( Reference Adelekan and Thurnham 38 ) indicated that the mean erythrocyte GPx activity in riboflavin-deficient rats was slightly, but not significantly lower than that in the control rats. In a study carried out by Dutta et al. ( Reference Dutta, Seirafi and Halpin 39 ), although riboflavin deficiency alone did not affect the activities of GPx and GR and increased the content of glutathione in the liver, ethanol administration (which can exacerbate oxidative stress) significantly decreased the activities of these enzymes and the content of glutathione in the liver of riboflavin-deficient rats compared with those in the liver of the riboflavin-sufficient control rats. However, there is a controversy regarding the results of different studies. In these studies, an increased GPx activity in the liver( Reference Taniguchi and Hara 22 ), lens( Reference Rao and Bhat 29 ) and blood cells( Reference Levin, Cogan and Levy 40 ) due to riboflavin deficiency has been reported. Increased lipid peroxidation has also been observed concurrent with an increased GPx activity. Therefore, it has been suggested that increased GPx activity could be a response to increased lipid peroxidation, resulting from riboflavin deficiency( Reference Rao and Bhat 29 ). The possible allosteric effect of reactive oxygen species on GPx enzyme can lead to an increased activity through increased lipid peroxidation( Reference Levin, Cogan and Levy 40 ).
Finally, results indicate that riboflavin status could affect the activity of antioxidant enzymes, but some studies do not agree with this. Furthermore, all studies in this area have been limited to animals, recommending further investigations in human populations.
Effect of riboflavin status on lipid peroxidation
Animal studies
The results of several animal studies indicate not only the adverse effects of riboflavin deficiency on lipid peroxidation but also the desirable effects of riboflavin administration on it( Reference Taniguchi and Hara 22 , Reference Liang, Liu and Xu 23 , Reference Bates 27 – Reference Rao and Bhat 29 , Reference Wang, Li and Lu 32 , Reference Hirano, Hamajima and Horiuchi 34 , Reference Levin, Cogan and Levy 40 – Reference Yagi, Komur and Yoshino 42 ). In studies in which riboflavin deficiency was induced in animals using a riboflavin-deficient diet, lipid peroxidation in different tissues was found to be significantly increased compared with that in the control groups( Reference Taniguchi and Hara 22 , Reference Liang, Liu and Xu 23 , Reference Bates 27 – Reference Rao and Bhat 29 , Reference Hirano, Hamajima and Horiuchi 34 , Reference Levin, Cogan and Levy 40 – Reference Yagi, Komur and Yoshino 42 ). In another study, it was shown that riboflavin administration could reduce the production of lipid peroxides (such as malondialdehyde (MDA)) or/and protein carbonyls in diabetic rats( Reference Wang, Li and Lu 32 ). However, only one study( Reference Bates 27 ) has reported that riboflavin deficiency increased MDA levels in the lens but did not affect MDA content in the liver in rats.
Human studies
A limited number of human studies have investigated the effect of riboflavin status on lipid peroxidation and confirmed the mentioned effect of riboflavin on lipid peroxidation. In a case–control study( Reference Das, Thurnham and Patnaik 43 ) on Indian children, it was found that plasma MDA levels of malaria patients with riboflavin deficiency were significantly higher than those of malaria-infected children with normal riboflavin status. However, in healthy children without malaria infection, no difference in MDA levels was observed between riboflavin-deficient and riboflavin-sufficient children. Furthermore, in this study, riboflavin status was found to be inversely correlated with plasma MDA levels in malaria patients but not with those in healthy subjects. A possible mechanism explained by the researchers is the increased oxidative stress in malaria-infected patients, which causes a reduction in glutathione levels( Reference Das, Thurnham and Patnaik 43 ). In a cross-sectional study carried out in Moscow, it was shown that there is a significant negative linear correlation between serum MDA levels and riboflavin intake( Reference Kodentsova, Vrzhesinskaia and Beketova 44 ). The results of the study carried out by George & Ojegbemi( Reference George and Ojegbemi 45 ) confirm these findings.
According to the findings of animal and human studies, riboflavin status appears to have an effect on the oxidative state of the body, in particular, on lipid peroxidation. However, it seems that the mechanism through which riboflavin can exert its antioxidant effect cannot be limited to the glutathione redox cycle and its relevant antioxidant enzymes. Although in most of the reviewed studies riboflavin status was found to be inversely related to lipid peroxidation, only in some of these studies, this relationship could be attributed to the role of riboflavin in the activities of glutathione reductase and related antioxidant enzymes. In these cases, a change in lipid peroxidation was found to occur simultaneously with an inverse change in the activity of antioxidant enzymes( Reference Liang, Liu and Xu 23 , Reference Wang, Li and Lu 32 , Reference Hirano, Hamajima and Horiuchi 34 ). In spite of increased lipid peroxidation due to riboflavin deficiency, any change in the activity of antioxidant enzymes was not observed in the study carried out by Huang et al. ( Reference Huang, Tian and Wu 28 ). In this study, increased liver MDA levels were observed in riboflavin-deficient fish without any change in liver GR and GPx activities and glutathione content. However, in this study, riboflavin deficiency was found to lead to reduced SOD and catalase activities. As H2O2 and superoxide (O2 −) ions have been reported to have an inhibitory effect on SOD and catalase activities, respectively, the authors have suggested that riboflavin deficiency may lead to an overproduction of reactive oxygen species (obviously through a mechanism independent of the glutathione redox cycle) beyond the scavenging ability of SOD and catalase. Therefore, SOD and catalase could be inhibited by these free radicals. Bate et al. ( Reference Bates 27 ) also reported elevated lipid peroxidation in riboflavin deficiency with no change in glutathione levels and GPx activity. Moreover, in some studies, an increased GPx activity was observed simultaneously with increased lipid peroxidation in riboflavin deficiency( Reference Taniguchi and Hara 22 , Reference Rao and Bhat 29 , Reference Levin, Cogan and Levy 40 ). As has been mentioned previously, this increased GPx activity, most probably, is a compensatory response to increased lipid peroxidation occurring in riboflavin deficiency.
It has been suggested that riboflavin may have antioxidant properties independent of its action in the glutathione redox cycle. Durusoy et al. ( Reference Durusoy, Karagoz and Ozturk 46 ) reported that the antimutagenic effect of riboflavin is independent of antioxidant enzyme activity. They suggested that the antimutagenic effect of riboflavin can, at least in part, result from its direct scavenging activity on free radicals produced by mutagens. In vitro studies have also indicated that riboflavin itself has an antioxidant nature, independent of its action as the GR coenzyme( Reference Toyosaki and Mineshita 47 ). The suggested mechanism could be the deactivation of hydroperoxide through the reversion of riboflavin from the reduced form (dihydroriboflavin) to the oxidised form (Fig. 3) ( Reference Toyosaki 48 ). It is also possible that riboflavin exerts its antioxidant effect by reinforcing the effect of other antioxidants such as vitamin C( Reference Rao and Bhat 29 ). In this study carried out by Rao & Bhat( Reference Rao and Bhat 29 ), a reduced concentration of vitamin C in the lens of riboflavin-deficient rats was reported, which is concurrent with increased lipid peroxidation. However, there is no strong evidence about the effect of riboflavin on other dietary antioxidants, suggesting further investigations.
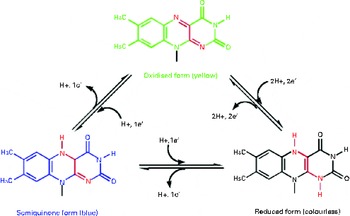
Fig. 3 Conversion of reduced riboflavin to the oxidised form – a possible mechanism for its antioxidant nature. (A colour version of this figure can be found online at http://www.journals.cambridge.org/bjn).
Effect of riboflavin on the attenuation of reperfusion oxidative injury
Reperfusion injury is the tissue damage that occurs when blood flows into the tissue after a period of ischaemia( Reference Carden and Granger 49 ). It has been shown that free radicals( Reference Ambrosio, Flaherty and Duilio 50 , Reference Bolli, Zughaib and Li 51 ) and inflammatory cytokines( Reference Kukielka, Smith and Manning 52 ) have a key role in reperfusion injury process. Some reports( Reference Betz, Ren and Ennis 53 – Reference Seekamp, Hultquist and Till 56 ) have indicated that riboflavin can alleviate oxidative injuries in these situations, probably through its ability to scavenge free radicals. Mack et al. ( Reference Mack, Hultquist and Shlafer 55 ) reported that riboflavin decreases reoxygenation injury in the heart of rabbits. It has also been shown that riboflavin could decrease oedema and brain injury after cerebral ischaemia( Reference Betz, Ren and Ennis 53 ). The protective effect of riboflavin against reperfusion oxidative injury in other organs such as the lung and after cardiac allotransplantation in animal models has also been reported( Reference Iwanaga, Hasegawa and Hultquist 54 , Reference Seekamp, Hultquist and Till 56 ).
This protective effect of riboflavin could be attributed to dihydroriboflavin, produced by the flavin reductase activity of NADPH-dependent methaemoglobin reductase( Reference Hultquist, Xu and Quandt 57 ). Dihydroriboflavin has been indicated to have the ability to reduce oxidised Fe in haemoproteins( Reference Matsuki, Yubisui and Tomoda 58 , Reference Xu and Hultquist 59 ), which have been implicated in the oxidative damage of cells including reperfusion oxidative injury( Reference Hultquist, Xu and Quandt 57 ). Although studies that have investigated the effect of riboflavin on reperfusion injury have obtained consistent results from experimental models, there are no firm data about its action in human body. Therefore, this needs to be clarified. Aside from this possible therapeutic effect of riboflavin, these findings could also introduce the riboflavin-dependent mechanism by which cells could protect themselves from oxidative damage in normal conditions( Reference Christensen 60 ) and provide further support for the antioxidant nature of riboflavin.
It is concluded that riboflavin could act as an antioxidant against oxidative stress, especially lipid peroxidation and reperfusion oxidative injury. The mechanisms by which riboflavin protects the body against oxidative stress may be attributed to the glutathione redox cycle and also to other possible mechanisms such as conversion of reduced riboflavin to the oxidised form. However, most of the investigations in this area are limited to experimental studies and, therefore, further investigations should examine this effect of riboflavin through observational and interventional studies in human populations.
Acknowledgements
This work was supported by the Tehran University of Medical Sciences, Deputy of Research. This work did not receive any financial support.
All authors contributed to the work and have approved the content of the submitted manuscript.
None of the authors has any conflicts of interest to declare.