Fe deficiency (ID) is the most prevalent micronutrient deficiency worldwide, affecting principally children aged < 5 years and women of childbearing age living in the poorer communities of the developing world( Reference McLean, Cogswell and Egli 1 ). ID has major negative impacts on health and mental development, and in pregnancy, it contributes to the risk of severe anaemia, which is associated with higher maternal morbidity and mortality( Reference Zimmermann and Hurrell 2 , Reference Aikawa, Ngyen and Sasaki 3 ). The aetiology of ID in developing countries is multifactorial, but the major contributors are low dietary Fe bioavailability and intake from monotonous diets based on cereal staple foods such as sorghum( Reference Zimmermann, Chaouki and Hurrell 4 ).
Sorghum (Sorghum bicolor (L.) Moench) is the world's fifth most important cereal in terms of production and a staple food in sub-Saharan Africa and India( Reference Rooney, Waniska, Smith and Frederiksen 5 ), where it is a major source of energy, proteins and micronutrients for millions of people( Reference Godwin, Gray, O'Brien and Henry 6 ). The colour of sorghum grain varies among different varieties. White grains are preferably used for the preparation of foods such as porridge, baked products and couscous. Red and brown grains are also widely used for the preparation of porridges and/or pastes, but, in addition, they are used for the preparation of fermented and non-fermented local beverages( Reference Dicko, Hilhorst and Gruppen 7 – Reference Kayode, Adegbidi and Hounhouigan 9 ). Thin sorghum porridges, which are occasionally fermented, are popular complementary foods throughout sub-Saharan Africa( Reference Badi, Pedersen and Monowar 10 – Reference Kayode, Nout and Bakker 13 ).
The native Fe concentration of sorghum has been reported to be about 3–5 mg/100 g flour( Reference Mitchikpe, Dossa and Ategbo 14 ). Fe bioavailability from sorghum-based diets is expected to be low due to high amounts of phytic acid (PA) in the grains( Reference Kayode, Linnemann and Hounhouigan 15 , Reference Makokha, Oniang'o and Njoroge 16 ) and high amounts of polyphenols (PP) in some coloured varieties( Reference Dicko, Hilhorst and Gruppen 7 , Reference Towo, Svanberg and Ndossi 17 ). Both PA and PP can be potent Fe absorption inhibitors, forming non-absorbable complexes in the gut lumen( Reference Hallberg, Rossander and Skanberg 18 , Reference Hurrell, Reddy and Cook 19 ). The inhibitory effect of PP varies according to their structure( Reference Hurrell, Reddy and Cook 19 – Reference Tuntipopipat, Judprasong and Zeder 21 ). The total PP concentration of sorghum depends not only on genetic factors such as seed colour and pericarp thickness, but also on environmental factors such as growing conditions( Reference Hahn and Rooney 22 ). Sorghum varieties with red and brown colours have higher average PP concentrations (1·35 %) than light-coloured sorghum grains (0·40 %)( Reference Dicko, Hilhorst and Gruppen 7 ); the colour is mainly attributable to PP called deoxyanthocyanidins( Reference Dykes and Rooney 23 , Reference Dykes, Rooney and Waniska 24 ).
Fortification of cereal flours with Fe is the main strategy to combat ID in communities where cereals are the staple food. Unlike wheat flour, sorghum flour is usually not milled centrally and fortification at the community level can be problematic. Therefore, Fe fortification of sorghum flour is still in the early stages, and knowledge on appropriate Fe compounds for sorghum fortification is scarce. Cereals such as sorghum are particularly difficult to fortify with Fe, since they contain significant amounts of both PA and PP( Reference Hurrell, Reddy and Juillerat 25 ). NaFeEDTA is recommended as a fortificant in cereals containing high amounts of PA( Reference Hurrell, Ranum and de Pee 26 ); however, results from previous studies in tea have raised questions about its ability to overcome the inhibitory effect of PP( Reference Hurrell, Reddy and Burri 27 ). The usefulness of NaFeEDTA in the presence of PP from other sources is unknown. Other approaches to enhance Fe bioavailability from sorghum flour would be the addition of ascorbic acid (AA), a well-known Fe absorption enhancer( Reference Hallberg, Brune and Rossander 28 ), and the enzymatic reduction of inhibitory PP by a PP oxidase (PPO). Phytase has been used in a similar way to degrade PA and increase Fe absorption from cereal meals( Reference Hurrell, Reddy and Juillerat 25 ).
Herein, we report three stable isotope absorption studies carried out in young women consuming dephytinised Fe-fortified sorghum porridges made from white and brown sorghum flours containing different concentrations of PP. The studies investigated a potential dose-dependent inhibitory effect of sorghum PP on Fe absorption and the ability of NaFeEDTA, AA and laccase to overcome the inhibitory effect.
Methods
Participants
A total of fifty apparently healthy, non-pregnant, non-lactating women aged between 18 and 40 years, with a normal BMI (18·5–25 kg/m2) and a body weight below 65 kg, were recruited from the student and staff population of ETH Zurich, Switzerland. The participants were randomly allocated to three Fe absorption studies, with sixteen or eighteen participants per study. Women with a known metabolic, chronic or gastrointestinal disease, as well as those on long-term medication (except oral contraceptives), were excluded from participating. Intake of vitamin and/or mineral supplements was not allowed during and 2 weeks before the studies. Women who had donated blood or experienced substantial blood loss within 4 months of the start of the study were not recruited. The study was carried out according to the guidelines laid down in the Declaration of Helsinki, and all procedures involving human subjects were approved by the ethical committee at ETH Zurich, Switzerland. All the participants provided written informed consent.
Study design
Using a randomised cross-over design with each participant serving as her own control, three Fe absorption studies were carried out. In study 1, the influence of different amounts of sorghum PP on Fe absorption was investigated using white and brown sorghum flours and a mixture of both. In study 2, the potential of NaFeEDTA to overcome the inhibitory effect of sorghum PP on Fe absorption was investigated, and in study 3, the potential of AA addition and PPO pre-treatment of sorghum meals to overcome the inhibitory effect was investigated. In each study, three differently labelled sorghum test meals were given to the participants on three consecutive days in a randomised fashion (Fig. 1). Fe absorption was determined using a stable isotope technique measuring the incorporation of isotopic Fe labels into erythrocytes 14 d after the consumption of the third test meal( Reference Walczyk, Davidsson and Zavaleta 29 ).
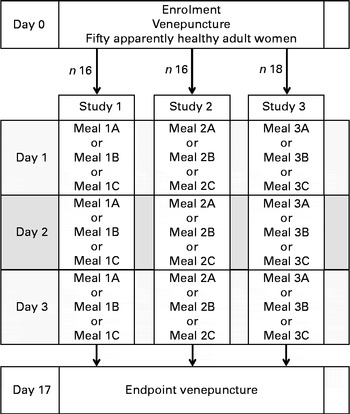
Fig. 1 Schematic diagram of the study protocol. Meals 1A and 2A are low-polyphenol (PP) sorghum porridges fortified with FeSO4; meal 1B is a medium-PP sorghum porridge fortified with FeSO4; meals 1C, 2B and 3A are high-PP sorghum porridges fortified with FeSO4; meal 2C is a high-PP sorghum porridge fortified with NaFeEDTA; meal 3B is a high-PP sorghum porridge fortified with ascorbic acid and FeSO4; meal 3C is a reduced-PP sorghum porridge pre-treated with laccase and fortified with FeSO4.
On day 0, body weight and height were measured, and the first blood sample was collected for Fe status (Hb; plasma ferritin, PF) and inflammation (C-reactive protein, CRP) measurements. On day 1, the first labelled test meal was given in two servings with an interval of minimum 3 h. On days 2 and 3, the second and third test meals were given after an overnight fast following the same procedure. The test meals were given under strictly standardised conditions and under close supervision of the investigators. The complete intake of isotopically labelled meals was ensured by rinsing the test meal bowl twice with 10 ml of high-purity water (18 mol/l) after consumption and by letting the participants consume the rinsing water. No intake of food and fluids was allowed between the two meals and for 3 h after the consumption of the second meal. In all the three studies, the servings of the three different test meals were labelled with 2 mg 54Fe, 57Fe or 58Fe. A second blood sample was drawn for Fe isotopic analysis 14 d after the consumption of the third test meal (day 17).
Test meals
A white sorghum variety (CSM 485; Kossa) and a brown sorghum variety (ICSV 1001; Framida) from Mali and Burkina Faso, respectively, were used to prepare the test meals with low, medium and high PP concentrations. White sorghum was decorticated in Mali using an abrasive dehulling device (TADD; Venable Machine Works). Brown sorghum was decorticated in France using an abrasive laboratory-scale decorticator (DMS 500; Electra). Both varieties were washed before decortication, and an extraction rate of 86–90 % was obtained. After decortication, they were milled in Switzerland using a centrifugal mill (Retsch) equipped with a Ti sieve to avoid Fe contamination. Flours were prepared in bulk and used for the three studies.
In study 1, test meals were based on the white sorghum flour labelled with 2 mg 54Fe as FeSO4 (1A), brown sorghum flour labelled with 2 mg 58Fe as FeSO4 (1C) and a 1:1 mixture of the two flours labelled with 2 mg 57Fe as FeSO4 (1B), respectively. In study 2, one test meal was based on the white sorghum flour labelled with 2 mg 54Fe as FeSO4 (2A) and the other two test meals were based on the brown sorghum flour labelled with 2 mg 57Fe as FeSO4 (2B) or with 2 mg 58Fe as NaFeEDTA (2C). In study 3, test meals were based on the brown sorghum flour labelled with 2 mg 58Fe FeSO4 (3A), labelled with 2 mg 57Fe as FeSO4 and containing 40 mg AA per serving (3B) or pre-treated with laccase and labelled with 2 mg 54Fe as FeSO4 (3C).
In all the three studies, test meal servings (235 g) were based on 50 g sorghum flour, 15 g sugar and 170 g water. Except for the test meal pre-treated with laccase, test meals were prepared by adding sorghum flour and sugar to previously heated water (about 55°C), and the pH of the resulting slurry was adjusted to 5·0–5·2 using 0·5 m-hydrochloric acid (Sigma-Aldrich). Approximately 3000 phytase units (FTU)/100 g flour were added to the slurry using the DSM phytase 5000 liquid provided by DSM Nutritional Products, and the slurry was held at about 55°C for 1 h in a water-bath to allow for complete PA degradation. The slurry was then heated to 80°C to inactivate phytase. After cooling, water was added to adjust to the ratio of water:sorghum flour of 3·4:1, and portions corresponding to 50 g dry weight of sorghum flour were weighed as test meal servings.
The test meal pre-treated with laccase was prepared by adding sorghum flour and sugar to previously heated water (about 40°C) without adjustment of pH. To the slurry, 7–10 ml of phytase and 120 ml of laccase (NS 33 136; Novozymes) were added, and the slurry was held at about 40°C for 4 h under continuous stirring in a water-bath to allow for complete PA and partial PP degradation. The slurry was then heated to 80°C to inactivate enzymes and processed in the same way as the other test meals mentioned above.
The test meals were prepared in batches, and servings were stored frozen until the day of the consumption. To each test meal serving, shortly before test meal consumption, 2 mg of Fe label in solution were added. With each test meal serving, 250 g of deionised water were consumed.
Test meal analysis
The Fe concentrations of the sorghum flours and test meals were determined using graphite-furnace atomic absorption spectrophotometry (AA240Z; Varian) after mineralisation by microwave digestion (MLS ETHOSplus; MLS GmbH). For the estimation of soil contamination, Al and Ti levels in the sorghum flours were determined by X-ray fluorescence analysis using Spectro X-Lab 2000 (Spectro Analytical Instruments GmbH). A matrix correction for food samples was applied. PA concentrations were measured using a modification of the Makower method( Reference Makower 30 ), in which Fe was replaced by cerium in the precipitation step. After the mineralisation of the precipitates, inorganic phosphate concentrations were determined according to the method of Van Veldhoven & Mannaerts( Reference Van Veldhoven and Mannaerts 31 ) and converted into PA concentrations. The total PP concentrations of the sorghum flours and test meals were determined using a modified Folin–Ciocalteu method( Reference Singleton and Rossi 32 ), and they are expressed as gallic acid equivalents. Wheat bran (PA assay) and milled beans (PP assay), flushed with Ar to avoid PP oxidation, were analysed together with each series of samples and were used as an internal control material to monitor reproducibility. In vitro accessible Fe concentrations were determined using the method described by Luten et al. ( Reference Luten, Crews and Flynn 33 ). This method includes a gastric stage, where the sample is incubated in a HCl–pepsin solution at pH 2 and 37°C for 2 h, followed by an intestinal stage determining dialysability.
Preparation of isotopically labelled iron
Isotopically labelled 54FeSO4, 57FeSO4 and 58FeSO4 were prepared from isotopically enriched elemental Fe (54Fe metal: 99·9 % enriched; 57Fe metal: 97·1 % enriched; 58Fe metal: 99·9 % enriched; all obtained from Chemgas) by dissolution in 0·1 m-sulphuric acid. The solutions were flushed with Ar to keep the Fe in the +II oxidation state. The prepared Fe tracer solutions were analysed for Fe isotopic composition and tracer Fe concentration using reverse isotope dilution MS. Na58FeEDTA was prepared in solution from 58Fe-enriched elemental Fe (58Fe metal: 99·9 % enriched; Chemgas). The metal was dissolved in 2 ml of HCl and diluted with water. The resulting FeCl3 solution was mixed with a freshly prepared aqueous Na2EDTA solution (Na2EDTA.H2O2; Sigma Chemical Company) at a molar ratio of 1:1 (Fe:EDTA). The resulting NaFeEDTA solution was added to the test meals.
Blood analysis
Hb concentrations were measured using a Coulter Counter (AcT8 Counter; Beckman Coulter); anaemia was defined as Hb concentrations < 120 g/l( 34 ). PF and CRP concentrations were measured using an IMMULITE automatic system (Siemens). ID was defined as PF concentrations < 15 μg/l and ID anaemia as Hb concentrations < 120 g/l and PF concentrations < 15 μg/l( 34 ). High-sensitivity CRP concentrations in healthy individuals were < 5 mg/l.
Each isotopically enriched blood sample was analysed in duplicate for its isotopic composition. Whole blood was mineralised by microwave digestion, and Fe was separated by anion-exchange chromatography and a subsequent precipitation step with ammonium hydroxide( Reference Hotz, Krayenbuehl and Walczyk 35 ). Fe isotope ratios were determined using a multicollector inductively coupled plasma-MS instrument (NEPTUNE; Thermo Finnigan).
Calculation of the percentage of iron absorption
The percentages of 54Fe, 57Fe and 58Fe labels in the blood were calculated based on the dose of administered isotopes (4 mg/type of test meal), the shift in Fe isotope ratios and the estimated amount of Fe circulating in the body. The amount of circulating Fe was calculated based on the blood volume estimated from height and weight and measured Hb concentrations( Reference Brown, Hopper and Hodges 36 ). The calculations were based on the principles of isotope dilution, taking into account that Fe isotopic labels were not monoisotopic using the method described by Turnlund et al. ( Reference Turnlund, Keyes and Peiffer 37 ). The calculation of Fe absorption is shown in detail in Fig. S1 (available online). For the calculation of the percentage of absorption, 80 % incorporation of the absorbed Fe into erythrocytes was assumed( Reference Barrett, Whittaker and Williams 38 ).
Statistical analyses
Analyses were carried out using the SPSS statistical software (SPSS 19.0; SPSS, Inc.). All values were converted to their logarithms for statistical analyses and reconverted for reporting. The results of the test meal analyses are reported as means and standard deviations. The results of Fe absorption analysis are presented as geometric means with the 95 % CI in parentheses. The results of Fe status and inflammation measures are presented as means and standard deviations if normally distributed. Medians with 25th–75th percentile ranges in parentheses are used to present results that were not normally distributed after log conversion (CRP and PF). Fe absorption from the different test meals in the same participant was compared using a linear mixed model with repeated measures followed by a post hoc Bonferroni test for multiple comparisons. In the linear mixed model, types of test meal and study day were the fixed effects and individual participant was the random effect. The dependent variable was Fe absorption, and study day was treated as the repeated measure assuming an unstructured covariance type. For the between-study comparison, the same linear mixed model with repeated measures was applied, but study as a fixed effect and PF as a covariate were added. A one-way ANOVA followed by a post hoc Bonferroni test for multiple comparisons was used for the analysis of participants' baseline characteristics as well as test meal composition (total Fe and PP). Differences were considered significant at P< 0·05. The studies were powered to detect an intra-subject difference of 30 % in Fe absorption with an α-level of 0·05.
Results
Participant characteristics
Among the participants, six (two in study 1, three in study 2 and one in study 3) had ID and three were anaemic (one in each study). ID anaemia was observed in two participants (one each in study 1 and study 2). A slightly elevated CRP concentration (>3 mg/l) was observed in three women, but none had a CRP concentration >5 mg/l. None of the variables given in Table 1, except age, differed significantly between the study groups.
Table 1 Age, anthropometric features and Hb, plasma ferritin (PF) and C-reactive protein (CRP) concentrations of the participating healthy adult women at baseline (Mean values and standard deviations; medians and 25th and 75th percentiles)
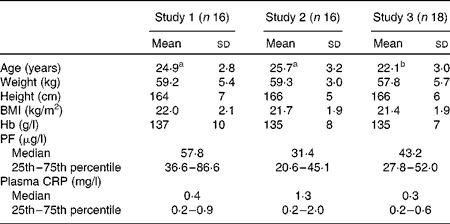
a,bMean values within a row with unlike superscript letters were significantly different (P< 0·05).
Test meal composition
Uncooked flours from decorticated white and brown sorghum had PP concentrations (expressed as gallic acid equivalents) of 21 (sd 1) mg and 553 (sd 16) mg/100 g, respectively. The PP concentration of the white sorghum porridge (low PP) was approximately ten and four times lower than that of the brown sorghum porridge (high PP) and that of the porridge based on a mixture of the white and brown sorghum flours (medium PP), respectively (P< 0·0001) (Table 2). Incubation with laccase reduced PP concentrations of the brown sorghum test meals by about 75 % (P< 0·0001). However, laccase did not completely degrade PP, and we were not able to attain a further PP reduction, as measured by the Folin–Ciocalteu method, by modifying the incubation conditions (time, temperature and pH). PA concentrations were below the detection limit in all the test meals ( < 8 mg/test meal serving).
Table 2 Composition of different sorghum test meals based on 50 g of flour and consumed by the healthy adult women (Mean values and standard deviations)
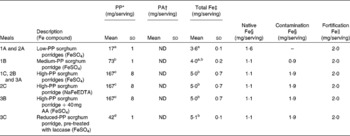
PP, polyphenol; PA, phytic acid; ND, not detectable; AA, ascorbic acid.
a,b,c,dMean values within a column with unlike superscript letters were significantly different (PP: P< 0·0001; Fe: P< 0·05).
* n 3 independent analyses. Expressed as gallic acid equivalents.
† ND, below the limit of detection ( < 8 mg/serving), n 3 independent analyses.
‡ n 3 independent analyses for the prepared sorghum porridges.
§ The calculation of native and contamination Fe concentrations is based on the measured Fe concentrations of the flours of washed (non-contaminated) and unwashed (contaminated) brown sorghum grains: 2·1 (sd 0·1) mg/100 g and 5·8 (sd 0·4) mg/100 g, respectively.
∥ 2 mg Fe added as 54Fe, 57Fe or 58Fe in the form of FeSO4 or NaFeEDTA.
The Fe concentration of the white sorghum flour was 2·6 (sd 0·3) mg/100 g. The total Fe concentration of the brown sorghum flour used for the preparation of the test meals was 5·8 (sd 0·4) mg/100 g, whereas native Fe concentration was 2·1 (sd 0·1) mg/100 g and contamination Fe concentration was about 3·7 mg/100 g. The native Fe concentration of the brown sorghum flour was obtained by analysing the Fe concentration of brown sorghum grains that, in contrast to the grains used to obtain the bulk of flour, were carefully washed after decortication. Fe contamination of the brown sorghum flour was confirmed by the small silver spots detected on the magnetic stirrer after the incubation of the flour with laccase. The amount of contamination Fe was about 0·9 mg/portion in the test meals based on the white and brown sorghum flours (meal 1B), and it was about 1·9 mg/portion in the test meals based on the brown sorghum flour (meals 1C, 2B, 2C, 3A, 3B and 3C). The total Fe concentrations and the proportion of different Fe sources in the different test meals are given in Table 2.
The Al concentrations of the non-contaminated and contaminated grains were 4·9 (sd 1·4) mg/100 g and 3·0 (sd 1·6 mg)/100 g, respectively, and Ti concentrations of the non-contaminated and contaminated grains were below the detection limit (0·5 mg/100 g). The in vitro accessible Fe concentrations of the non-contaminated and contaminated flours after in vitro gastric digestion followed by a dialysability procedure were 578 (sd 87) μg/100 g and 608 (sd 30) μg/100 g, respectively, indicating that the contamination Fe was not soluble during simulated gastric digestion and/or dialysable.
Iron absorption measurements
In study 1, the mean percentage of Fe absorption from the medium-PP and high-PP sorghum meals was approximately three times lower (P< 0·001) than that from the low-PP sorghum meals (Table 3). There was no difference in Fe absorption (P= 0·9) between the medium-PP and high-PP sorghum meals. In study 2, Fe absorption from the FeSO4-fortified low-PP sorghum meals was four and 2·3 times higher (P< 0·001) than that from the FeSO4- or NaFeEDTA-fortified high-PP sorghum meals, respectively. However, Fe absorption from the high-PP sorghum meals fortified with NaFeEDTA was almost 2-fold higher than that from the high-PP sorghum meals fortified with FeSO4 (P< 0·001). In study 3, the mean percentage of Fe absorption from the high-PP sorghum meals with added AA was almost 3-fold higher than that from the high-PP sorghum meals without AA (P< 0·001). Decreasing the concentrations of PP of high-PP sorghum meals by laccase pre-treatment did not increase Fe absorption compared with that from the high-PP sorghum meals without laccase pre-treatment (P= 0·4). Regarding the between-study comparison (Fig. 2), Fe absorption from meals containing 167 mg PP per serving fortified either with FeSO4 (meals 1C, 2B and 3A) or with NaFeEDTA (meal 2C) was different (P< 0·05), but absorption from the latter was not different from that from the FeSO4-fortified meals containing 72 mg PP (P= 0·8) or the laccase pre-treated meals (42 mg PP; P= 0·06). The between-study comparison of meals based on the white sorghum flour (meals 1A and 2A) and the AA-fortified meal based on the brown sorghum (meal 3B) showed no difference in Fe absorption (P= 1·0).
Table 3 Percentage of iron absorption from different sorghum test meals fortified with ferrous sulphate or sodium iron EDTA in the healthy adult women (Geometric means and 95 % CI)
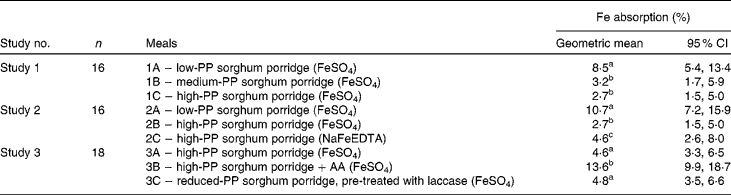
PP, polyphenol; AA, ascorbic acid.
a,b,cGeometric mean values in studies 1, 2 and 3 with unlike superscript letters were significantly different at P< 0·001 (except meal 2B v. meal 2C, P< 0·05).
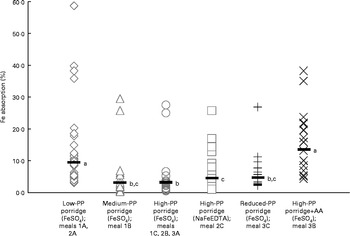
Fig. 2 Between-study comparison of iron absorption from the different types of sorghum porridges consumed by healthy adult women. Values are individual data points, with the horizontal bar representing the geometric mean. Absorption data from the same type of meals given in the different studies (meals 1A and 2A, n 32; meals 1C, 2B and 3A, n 50) were combined for the statistical analyses. a,b,cValues with unlike letters were significantly different (P< 0·05). PP, polyphenol; AA, ascorbic acid.
Discussion
In study 1, we observed that 73 mg and 167 mg of PP in brown sorghum, expressed as gallic acid equivalents, significantly decreased the absorption of fortification Fe from standardised dephytinised sorghum porridges. These results indicate that Fe bioavailability from traditional Western African porridges based on red or brown sorghum, containing 80–160 mg of PP( Reference Kayode, Adegbidi and Hounhouigan 9 ), would be expected to be impaired even if most of the PA is degraded by fermentation. However, it should be noted that we used standardised dephytinised test meals, and the effect of PP on Fe absorption might be differently pronounced in composite, non-dephytinised, sorghum-based meals. In a study with beans, the inhibitory effect of PP has only been observed in standardised bean meals but not in composite bean meals containing different enhancers and inhibitors( Reference Petry, Egli and Gahutu 39 ).
Brown sorghum PP seem to be slightly less inhibitory than black tea PP, since Hurrell et al. ( Reference Hurrell, Reddy and Cook 19 ) reported that 100 and 200 mg gallic acid equivalents of black tea PP reduced Fe absorption from an Fe-fortified bread meal by about 80 %. The inhibitory effect of sorghum PP in the present study was similar to that of herb tea PP( Reference Hurrell, Reddy and Cook 19 ), but markedly greater than the inhibitory effect of common bean PP( Reference Petry, Egli and Zeder 40 ). The reason for these differences in the inhibitory potential of PP from different food sources is the different PP structures, which lead to different Fe-binding properties( Reference Brune, Rossander and Hallberg 20 , Reference Tuntipopipat, Judprasong and Zeder 21 ). The structures responsible for the inhibitory effect observed in the present study are most probably catechol and galloyl groups of condensed tannins, which represent 72 % of the PP compounds found in the brown sorghum variety used in the present study (Framida)( Reference Dicko, Hilhorst and Gruppen 7 ). Red or brown sorghum has been reported to contain high amounts of catechols and galloyls (1350 and 550 mg/100 g grain, respectively)( Reference Towo, Svanberg and Ndossi 17 ), which can form strong, non-absorbable complexes with Fe in the intestinal tract( Reference Hurrell, Reddy and Cook 19 , Reference Brune, Rossander and Hallberg 20 ). The fact that Fe absorption from the medium-PP sorghum meals was as low as that from the high-PP sorghum meals suggests that relatively low amounts of these condensed tannins in Framida sorghum can strongly inhibit Fe absorption. The white sorghum contained very little PP and did not have a pigmented testa, indicating that it does not contain condensed tannins. We assume that there was little or no significant interference with Fe absorption from white sorghum porridges.
The results of the present study are in agreement with those of a previous radioisotope study reporting 2-fold higher Fe absorption from a low-PP sorghum variety than from a high-PP sorghum variety( Reference Gillooly, Bothwell and Charlton 41 ), but contradictory to those of an early radioisotope study reporting similar Fe absorption from a low- and a high-tannin sorghum variety( Reference Radhakrishnan and Sivaprasad 42 ). In both studies, the different PA concentrations of the two sorghum varieties make interpretation difficult. More recently, Hurrell et al. ( Reference Hurrell, Reddy and Juillerat 25 ) have reported that dephytinisation of low-tannin sorghum meals increases Fe absorption by 2-fold, but that no improvement in Fe absorption can be observed after dephytinisation of high-tannin sorghum meals. In all the three previous studies, the exact concentrations of PP in the test meals are unknown because PP measurements were made before cooking, which is known to significantly decrease PP concentrations( Reference Awika, Dykes and Gu 43 , Reference Kayode, Linnemann and Nout 44 ).
The results of study 2 using NaFeEDTA confirmed the earlier reports that EDTA cannot completely protect Fe from PP binding( Reference Hurrell, Reddy and Burri 27 , Reference Macphail, Bothwell and Torrance 45 ). Presumably at the pH of the stomach, PP (but not PA) can still strongly compete with EDTA for Fe binding. Sorghum foods are usually not completely dephytinised and contain considerable amounts of PA( Reference Kayode, Linnemann and Nout 44 ). NaFeEDTA is known to overcome the inhibitory effect of PA( Reference Hurrell, Reddy and Burri 27 ); thus, it seems to be a suitable compound for the fortification of sorghum flour containing both inhibitors. A previous study using NaFeEDTA as a fortificant for fermented red sorghum flour with moderate concentrations of PP and PA has reported high Fe absorption of about 18 % in women treated for malaria( Reference Cercamondi, Egli and Ahouandjinou 46 ).
The enhancing effect of AA on Fe absorption in study 3, completely overcoming the inhibitory effect of PP, can be attributed to its ability to reduce ferric Fe to ferrous Fe in the stomach and duodenum and to form soluble complexes with ferric and ferrous Fe at a higher pH in the intestine( Reference Rossander-Hulthén, Hallberg and Hallberg 47 ). Fe that is bound to AA can no longer bind to PP, and ferrous Fe forms much weaker complexes with PP compared with ferric Fe( Reference Perron and Brumaghim 48 ). The present results are in agreement with those of a previous study, which showed that AA prevents the dose-dependent inhibitory effect of the PP compound tannic acid( Reference Siegenberg, Baynes and Bothwell 49 ). The addition of AA to high-PP sorghum foods fortified with Fe would appear to be a useful approach to increase Fe bioavailability, but the heat and oxidation sensitivity of AA is a disadvantage and has to be considered.
It was somewhat surprising that the enzymatic reduction of total PP in sorghum porridges by laccase did not increase Fe absorption. Enhanced absorption was predicted by an in vitro study, which reported increased in vitro accessible Fe concentrations after PP reduction in dephytinised high-tannin sorghum( Reference Matuschek, Towo and Svanberg 50 ). The in vitro study, however, used tyrosinase instead of laccase as the PPO and whole red sorghum grains with a much higher initial PP concentration than that of the decorticated sorghum in the present study. We used laccase as the PPO because, in terms of PP degradation, tyrosinase did not perform well in preliminary trials. Furthermore, tyrosinase is not suitable for human food applications, as there is currently no food-grade product available. Food-grade laccase is an enzyme that catalyses the oxidation of a wide range of phenolic substrates including ortho- and para-diphenols and triphenols. Tyrosinase preferably oxidises monophenols and a few diphenols( Reference Aniszewski, Lieberei and Gulewicz 51 ). We had assumed that laccase would oxidise the catechol groups and, as these groups are suspected to bind to Fe, increase absorption. However, the present results indicate that laccase did not degrade or only partly degraded the inhibitory PP in the brown sorghum porridge. It seems that the remaining unchanged PP (42 mg/serving) are still strongly inhibitory and/or that the transformed PP can still bind to Fe. Although the present study did not find laccase to be useful to improve Fe absorption, more systematic studies with laccase and other PPO are needed to evaluate further possibilities.
Some test meals used in the present study contained contamination Fe (about 23 % and about 38 % of total Fe). We can rule out that this Fe came from soil contamination because the grains were carefully washed before decortication and because soil-contaminated grains would have higher Al and Ti concentrations than those found in the grains used in the present study( 52 ). Contamination from milling can also be excluded because the centrifugal mill was constructed with Ti. Therefore, iron contamination might have resulted from the decorticator made of stainless steel. Based on the present results of simulated gastric digestion followed by dialysis, which showed that the quantity of in vitro accessible Fe was identical for non-contaminated and contaminated sorghum grains, we conclude that the Fe in stainless steel is not bioavailable or at best poorly bioavailable. Nevertheless, if significant amounts of the contaminant steel Fe have dissolved and entered the common Fe pool in the three Fe absorption studies, our data for the percentage of Fe absorption would slightly underestimate the inhibitory effect of PP because the additional bioavailable Fe from steel would lead to a lower molar ratio of PP:Fe, which could result in a higher percentage of absorption from the isotopic tag. Therefore, we conclude that the contamination Fe present in some sorghum meals would not be expected to greatly influence the measured percentage of absorption values or change the conclusion on the extent to which sorghum PP inhibit Fe absorption.
In conclusion, the three Fe absorption studies show that brown sorghum PP are strong inhibitors of Fe absorption. They appear to be more inhibitory than bean PP and similar to herb tea PP. This means that dephytinisation of meals, based on coloured sorghum varieties, is unlikely to greatly increase Fe absorption as does dephytinisation of other cereals( Reference Hurrell, Reddy and Juillerat 25 ). AA is the preferred enhancer of Fe absorption from sorghum meals as this overcomes the inhibition of both PP and PA, whereas the use of NaFeEDTA is much more effective against PA than against PP. Reduction of PP by PPO appears to be possible, and this treatment merits more investigation.
Supplementary material
To view supplementary material for this article, please visit http://dx.doi.org/10.1017/S0007114513002705
Acknowledgements
The authors thank Claire Monquet-Rivier (Institute de Recherche pour le Développement, Montpellier, France), Laurencia Toulsoumdé Ouattara (Centre National de la Recherche Scientifique et Technologique, Ouagadougou, Burkina Faso) and Eva Weltzien (International Crops Research Institute for the Semi-Arid Tropics, Bamako, Mali) for providing the sorghum varieties and for the decortication of sorghum. They also thank DSM Nutritional Products (Basel, Switzerland) and Novozymes (Dittingen, Switzerland) for donating the phytase and laccase, respectively.
The present study was supported by the INSTAPA project, which receives funding from the European Community's Seventh Framework Programme [FP7/2007–2013] under grant agreement no. 211484. The Seventh Framework Programme of the European Community had no role in the design and analysis of the study or in the writing of this article.
The authors' contributions are as follows: C. I. C., I. M. E., C. Z. and R. F. H. designed the research; C. I. C., I. M. E. and C. Z. conducted the research; C. I. C. and C. Z. analysed the data; C. I. C., I. M. E. and R. F. H. wrote the paper; C. I. C., I. M. E. and R. F. H. had primary responsibility for the final content. All authors read and approved the final version of the paper. None of the authors declares any conflict of interest.