Several meta-analyses have demonstrated that the consumption of soya protein (SP) reduces the concentration of total cholesterol, especially that of LDL-cholesterol, in humans. In some instances, it increases the concentration of HDL-cholesterol( Reference Anderson, Johnstone and Cook-Newell 1 – Reference Zhan and Ho 3 ). Several mechanisms have been proposed to explain how SP can reduce the concentration of cholesterol( Reference Potter 4 , Reference Torres, Torre-Villalvazo and Tovar 5 ). Among other possible mechanisms, the data obtained from animal studies, mainly using rats and rabbits, suggest that SP increases the excretion of bile acids (BA), lowering the serum concentration of cholesterol( Reference Torres, Torre-Villalvazo and Tovar 5 – Reference Tanaka, Aso and Sugano 7 ).
BA are produced via the neutral (classic) pathway or the acidic (alternative) pathway( Reference Chawla, Saez and Evans 8 ). The neutral pathway is controlled by the rate-limiting enzyme 7α-hydroxylase (also called CYP7A1)( Reference Russell 9 ), whereas the flux through the alternative pathway is regulated by the enzyme 27α-hydroxylase (CYP27)( Reference Repa, Lund and Horton 10 ). The expression of CYP7A1 is regulated by a member of the transcription factor family: liver X receptors or LXRα( Reference Peet, Turley and Ma 11 ). LXR are nuclear hormone receptors that form active heterodimers with retinoid X receptors, which are activated by oxysterols and serve as key sensors of intracellular sterol levels by regulating the expression of the genes that control cholesterol absorption, storage, transport and elimination. It is known that LXRα up-regulates the expression of CYP7A1 by increasing the production of BA( Reference Peet, Turley and Ma 11 ).
In mice, the expression of CYP7A1 is down-regulated by the nuclear receptor farnesoid X receptor (FXR), which is activated by the presence of its natural ligands, BA( Reference Sinal, Tohkin and Miyata 12 ). Thus, when there is an increase in the enterohepatic circulation of BA, these acids activate FXR, which in turn stimulates the transcription of another nuclear receptor, small heterodimer protein (SHP). The expression of SHP represses the transcription of CYP7A1 by competing with the nuclear receptor liver receptor homolog 1 (LRH1), which is a constitutive activator of the CYP7A1 gene( Reference Lu, Makishima and Repa 13 , Reference Goodwin, Jones and Price 14 ). However, it has been demonstrated that CYP27 activity is insensitive to the size of the hepatic BA pool( Reference Xu, Salen and Shefer 15 ). More recent studies have established that the expression of CYP7A1 in mice is also regulated by fibroblast growth factor 15 (FGF15), which is the orthologue of the human FGF19 (fibroblast growth factor 19) and is synthesised in the intestine. The expression of FGF15 is stimulated in the small intestine by the nuclear BA receptor FXR and it is released into the circulation. It is then recognised by a specific receptor in the liver, fibroblast growth factor receptor 4 (FGFR4), leading to an increase in the expression of SHP ( Reference Inagaki, Choi and Moschetta 16 ). As a consequence, there is a reduction in the expression of CYP7A1 and therefore in the synthesis of BA.
On the other hand, the synthesis of FGF15 in the intestine, particularly in the ileal portion, is controlled by the transcription factor FXR. This nuclear receptor also regulates the expression of several important proteins involved in the transport and mobilisation of BA in the enterocytes. These proteins, the ileal BA transporter and the ileal BA-binding protein play key roles in the uptake and mobilisation of BA from the enterocytes into the circulation to transport them into the liver via enterohepatic circulation( Reference Nakahara, Furuya and Takagaki 17 ). In addition, the concentration of BA, as well as that of cholesterol in the enterocytes, is also regulated by the specific transporters that belong to the ATP cassette-binding transporter family ABCG5/8 (ATP-binding cassette, subfamily G, member 5/8)( Reference Kamisako and Ogawa 18 ).
However, there is scarce evidence as to whether SP regulates the synthesis of BA by inducing metabolic adaptations in the liver and intestine. Therefore, the aim of the present study was to determine whether the consumption of SP increases faecal BA excretion by increasing BA synthesis via SHP and/or FGF15.
Experimental methods
Animals and diets
The animal protocol described below was approved by the Animal Committee of the National Institute of Medical Sciences and Nutrition, Mexico City. A total of forty-eight male Wistar rats (Harlan-Teklad) weighing 170 g each were randomly divided into four groups. The rats were housed individually in stainless-steel cages and placed in temperature- and humidity-controlled rooms under a 12 h light–12 h dark cycle. The rats had free access to water throughout the study. The rats in each group were fed ad libitum for 3 d to acclimatise them to one of the following diets: (1) 20 % casein (C) (Harlan-Teklad Research Diets); (2) 20 % soya protein (S) (Supro 710; Solae); (3) 20 % casein plus 0·2 % cholesterol (CC); (4) 20 % soya protein plus 0·2 % cholesterol (SC). The composition of isoflavones in the SP was as follows (mg/g of protein): genistein 1·38; daidzein 0·71; glycitein 0·19. On the 4th day of the study, each group was subjected to time-restricted feeding (food was available beginning at 09.00 hours and removed at 14.00 hours) with one of the four experimental diets for 1 week. The rats were killed after an overnight fast. Body weight and food intake were recorded daily. The rats were killed by decapitation after CO2 anaesthetisation. Blood was collected in tubes with a gel and clot activator (BD) and centrifuged at 1000 g for 10 min; serum was separated and stored at − 70°C. The liver was removed, weighed and immediately frozen and stored at − 80°C until the extraction of total RNA or total protein. The ileum was quickly removed and immediately frozen and stored at − 80°C until the extraction of total RNA or total protein. Faecal samples were collected for 3 d before the end of the experimental period.
Determination of cholesterol and TAG concentrations
Hepatic and faecal lipids were extracted with chloroform–methanol using the Folch method( Reference Folch, Lees and Sloane Stanley 19 ). Total serum, faecal, and hepatic cholesterol and TAG concentrations were measured using a colorimetric kit. The colorimetric kit for TAG and cholesterol assays was obtained from DiaSys Diagnostic Systems GmbH.
Determination of bile acid concentrations
Total BA from freeze-dried faecal samples were extracted with t-butanol and measured enzymatically with 3α-hydroxysteroid dehydrogenase (Sigma) according to the method of Turley & Dietschy( Reference Turley and Dietschy 20 ).
Real-time PCR
Total RNA was extracted from the liver and ileum samples according to the method described by Chomczynski & Sacchi( Reference Chomczynski and Sacchi 21 ) and pooled. To carry out an integrity analysis, 15 μg of total RNA were separated using 1·0 % agarose/2·2 m-formaldehyde gel electrophoresis. Samples of 300 ng of total RNA were subjected to reverse transcription and PCR amplification using Master Mix (Applied Biosystems). Parallel non-template control reactions were run in the absence of RNA to assess the absence of nucleic acid contamination in the reaction mixtures. TaqMan fluorogenic probes and oligonucleotide primers were obtained from Applied Biosystems (SHP (Rn00589173_ml), LRH1 (Rn00572649_m1), LXR (Rn00581185_m1), sterol regulatory element-binding protein-1c (SREBP1c) (Rn 01495769_m1), CYP7A1 (Rn00564065_m1), CYP27 (Rn00710297_m1), ABCG5 (Rn), ABCG8 (Rn00590367_m1), ATP-binding cassette transporter A1 (ABCA1) (Rn00710172_m1) and ileal bile acid protein (IBAP) (Rn00567314_m1)). TaqMan PCR assays for each target gene were carried out in triplicate in ninety-six-well optical plates with the ABI Prism 7000 Sequence Detection System (Perkin-Elmer Applied Biosystems) following the manufacturer's protocol. The relative amounts of all mRNA were calculated using the comparative CT method (User Bulletin no. 2; PE Applied Biosystems). For the liver and intestine analyses, β-actin was used as the invariant control.
Western blot analysis
Extracts of the rat liver and ileum were prepared by homogenising the tissue in a lysis buffer with 50 mm-Tris, pH 7·4, 0·27 m-sucrose, 1 mm-sodium orthovanadate, pH 10, 1 mm-EDTA, 1 mm-ethylene glycol tetraacetic acid, 10 mm-sodium β-glycerophosphate, 50 mm-NaF, 5 mm-sodium pyrophosphate, 1 % (w/v) Triton X-100, 0·1 % (v/v) 2-mercaptoethanol and protease inhibitor cocktail tablets. The samples were stored at − 20°C until analysis. The proteins (40 μg) were separated electrophoretically on a 7 % SDS–polyacrylamide gel and transferred onto polyvinylidene difluoride membranes. The membranes were incubated with specific antibodies against total FGFR4 or total FGF15 and detected with an anti-rabbit secondary antibody. A β-actin antibody was used as a loading control. The labelled bands were detected using chemiluminescence with the visualiser Spray & Glow Western blotting detection system. The chemiluminescence was quantified using optical densitometry and analysed with the ImageJ 144p digital image processing software (National Institutes of Health).
Statistical analysis
Values are expressed as means with their standard errors. All the parameters were evaluated with a two-way ANOVA using the type of protein and the cholesterol content in the diet as independent variables, followed by Fisher's protected least significant difference test. Differences were considered to be statistically significant at P< 0·05 and are indicated by letters in the figures (a>b>c).
Results
Weight gain and food intake
At the beginning of the study, rats from the four experimental groups were not significantly different. However, at the end of the study, rats fed the SC diet tended to gain less weight, although the difference was not significant (P= 0·052). In addition, the average food and energy intake was similar in all the four groups, but as expected, cholesterol intake was higher in the groups that consumed diets containing cholesterol (Table 1).
Table 1 Body and liver weights and serum and hepatic lipid concentrations in rats fed casein or soya protein diets with or without 0·2 % cholesterol (Chol) (Mean values with their standard errors; n 4)
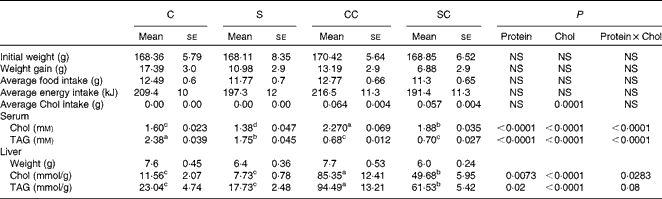
C, 20 % casein; S, 20 % soya protein; CC, 20 % casein plus 0·2 % Chol; SC, 20 % soya protein plus 0·2 % Chol.
a,b,c,dMean values within a row with unlike superscript letters were significantly different (P< 0·05; a>b>c>d).
Serum and hepatic lipid concentrations
Rats fed the CC diet had significantly higher serum cholesterol concentrations than those in the other groups (Table 1). The two-way ANOVA indicated that the type of protein consumed and the cholesterol content in the diet had a significant effect on serum cholesterol concentrations. Rats fed the S or SC diet had a serum cholesterol concentration that was approximately 15 % lower than that of the corresponding groups fed the C or CC diet. Serum TAG content followed the opposite pattern: rats fed the C and S diets had significantly higher concentrations than those fed the CC and SC diets, indicating that the cholesterol content in the diet rather than the type of protein consumed reduced serum TAG concentrations (Table 1).
Interestingly, the liver weight of rats fed the S or SC diet was approximately 10 % lower than that of rats fed the C or CC. As expected, rats fed diets with 0·2 % cholesterol had significantly higher hepatic cholesterol concentrations than those fed diets without cholesterol. However, rats fed the SC diet had 42 % lower hepatic cholesterol concentrations than those fed the CC diet (P< 0·05). Rats fed the SC diet accumulated approximately 6·4 times more cholesterol in their livers than those fed the S diet, and rats fed the CC diet accumulated 7·4 times more cholesterol than those fed the C diet. There was no significant difference between rats fed the C diet and those fed the S diet. Hepatic TAG concentrations followed a similar pattern: rats fed the SC diet had 35 % lower TAG concentrations than those fed the CC diet, and TAG concentrations in the liver of rats fed the CC or SC diet were 4·1 and 3·5 times lower than those in rats fed the C and S diets, respectively (Table 1).
Hepatic gene expression of SHP, liver receptor homolog 1, liver X receptors, sterol regulatory element-binding protein-1c, 7α-hydroxylase and 27α-hydroxylase
To explore BA synthesis, we determined the relative expression of SHP, LRH1, LXR, SREBP1c, CYP7A1 and CYP27 mRNA in the liver of rats in the four groups. The results revealed that the expression of SHP and LRH1 mRNA was higher in rats fed the SP diet regardless of the cholesterol content in the diet. As expected, the presence of cholesterol in the diet reduced the expression of SHP and LRH1 (Fig. 1(A) and (B)). The expression of CYP7A1 mRNA was significantly higher in the liver of rats fed diets with cholesterol than in that of rats fed diets without cholesterol (Fig. 1(C)). Interestingly, rats fed the SC diet had significantly higher expression of CYP7A1 than those fed the CC diet (Fig. 1(C)). There were no significant differences in the expression of CYP27 among the groups (Fig. 1(D)). On the other hand, the expression of LXR and SREBP1c mRNA was significantly lower in the liver of rats fed the SP diet, regardless of the cholesterol content in the diet (Fig. 1(E) and (F)).
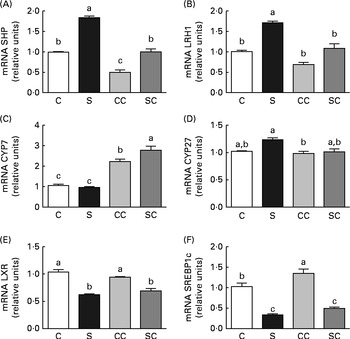
Fig. 1 Effects of the consumption of a soya protein diet with 0·2 % cholesterol (SC) and without 0·2 % cholesterol (S) for 9 d on the expression of (A) SHP, (B) liver receptor homolog 1 (LRH1), (C) 7α-hydroxylase (CYP7A1), (D) 27α-hydroxylase (CYP27), (E) liver X receptor (LXR) and (F) sterol regulatory element-binding protein-1c (SREBP1c) in the liver of rats (n 12 rats per group). Values are means, with their standard errors represented by vertical bars. a,b,cMean values with unlike letters were significantly different (P< 0·05). C, 20 % casein; CC, 20 % casein plus 0·2 % cholesterol.
Concentrations of ileal fibroblast growth factor 15, hepatic fibroblast growth factor receptor 4 and faecal bile acid
To determine whether SP consumption has an independent mechanism of SHP action, we measured the concentrations of FGF15 and FGFR4 in the ileum and liver, respectively. The results indicated that the consumption of SP increased FGF15 concentrations, but FGF15 was not detected in rats fed diets without cholesterol (Fig. 2(A)). Interestingly, rats fed the CC diet had FGFR4 concentrations that were 0·22 times lower than those of rats fed the C diet, and rats fed the SC diet had FGFR4 concentrations that were 1·0 times lower than those of rats fed the S diet (Fig. 2(B)).
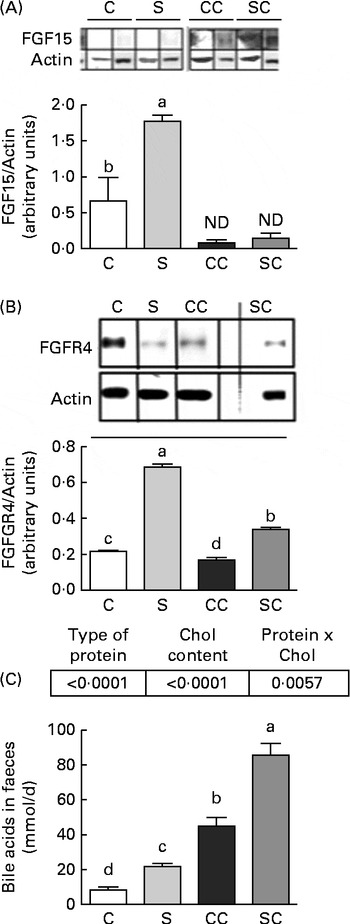
Fig. 2 Effects of the consumption of a soya protein diet with 0·2 % cholesterol (SC) and without 0·2 % cholesterol (S) for 9 d on (A) fibroblast growth factor 15 (FGF15) concentrations in the ileum, (B) fibroblast growth factor receptor 4 (FGFR4) concentrations in the liver and (C) total bile acid concentrations in the faeces (n 12 rats per group). P values for protein ( < 0·0001), cholesterol ( < 0·0001) and protien × cholesterol (0·0057). Values are means, with their standard errors represented by vertical bars. a,b,c,dMean values with unlike letters were significantly different (P< 0·05). C, 20 % casein; CC, 20 % casein plus 0·2 % cholesterol; ND, not detected.
Total faecal BA excretion was measured in the faecal samples collected for 3 d before the end of the experimental period. As shown in Fig. 2(C), rats fed the SC diet excreted significantly more BA during the study period than those fed the CC diet. At the end of the study, rats fed the SC diet excreted 0·94 times more BA than those fed the CC diet (P< 0·05). Interestingly, rats fed the S diet also excreted 1·5 times more BA than those fed the C diet. The two-way ANOVA revealed that the type of protein consumed and the cholesterol content in the diet had a significant effect on faecal BA excretion. In addition, the interaction between the type of protein consumed and the cholesterol content in the diet was highly significant.
Hepatic gene expression of ATP-binding cassette transporter A1 and ATP-binding cassette, subfamily G, member 5 and serum HDL and LDL concentrations
To evaluate reverse cholesterol transport (RCT), we measured the expression of ABCA1 and ABGC5 in the liver. The expression of ABCA1 was significantly increased only in rats fed diets containing 0·2 % cholesterol (Fig. 3(A)). There were no significant differences in the abundance of ABCG5 mRNA among the four groups (Fig. 3(B)).
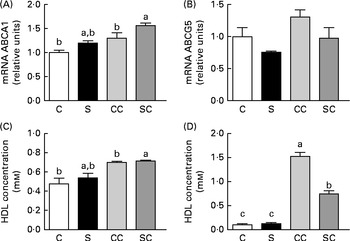
Fig. 3 Effects of the consumption of a soya protein diet with 0·2 % cholesterol (SC) and without 0·2 % cholesterol (S) for 9 d on the expression of (A) ATP-binding cassette transporter A1 (ABCA1) and (B) ATP-binding cassette, subfamily G, member 5 (ABCG5) in the liver of rats, (C) HDL concentrations in serum and (D) LDL concentrations in serum (n 12 rats per group). Values are means, with their standard errors represented by vertical bars. a,b,cMean values with unlike letters were significantly different (P< 0·05). C, 20 % casein; CC, 20 % casein plus 0·2 % cholesterol.
To determine whether the consumption of SP regulates HDL and/or LDL concentrations, we measured the concentrations. The results indicated that the consumption of SP does not alter HDL concentrations (Fig. 3(C)). However, LDL concentrations in rats fed the SC diet were 2·0 times lower than those in rats fed the CC diet (Fig. 3(D)).
Intestinal gene expression of ATP-binding cassette, subfamily G, member 5, ATP-binding cassette, subfamily G, member 8, and ileal bile acid-binding protein and total faecal cholesterol concentrations
We studied the effect of SP on the expression of several genes in the ileal portion of the intestine. The expression of the ABCG5 and ABCG8 transporters was significantly stimulated by the cholesterol and SP content in the diet, and the effect was additive, indicating that the up-regulation of the expression of these transporter genes by SP was enhanced when rats consumed diets containing cholesterol (Fig. 4(A) and (B)). There were no significant differences in the expression of the ileal BA-binding protein (IBABP) among the groups (Fig. 4(C)). Interestingly, rats fed the SP diet had significantly higher faecal cholesterol concentrations than those fed the C diet (Fig. 4(D)).
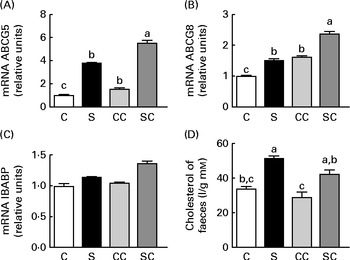
Fig. 4 Effects of the consumption of a soya protein diet with 0·2 % cholesterol (SC) and without 0·2 % cholesterol (S) for 9 d on the expression of (A) ATP-binding cassette, subfamily G, member 5 (ABCG5), (B) ATP-binding cassette, subfamily G, member 8 (ABCG8) and (C) ileal bile acid-binding protein in the ileum of rats and the concentrations of (D) total faecal cholesterol (n 12 rats per group). Values are means, with their standard errors represented by vertical bars. a,b,cMean values with unlike letters were significantly different (P< 0·05). C, 20 % casein; CC, 20 % casein plus 0·2 % cholesterol.
Discussion
SP is known to lead to significant reductions in serum and hepatic cholesterol concentrations in animal models and in humans. In the present study, as others have established in previous studies, we determined that one potential mechanism for the elimination of cholesterol by SP consumption is the elimination of BA( Reference Potter 4 , Reference Lin, Meijer and Vermeer 6 , Reference Huff and Carroll 22 ). There is evidence that multiple mechanisms are involved in the inhibition of CYP7A1 gene transcription via BA feedback. It is generally recognised that the FXR/SHP cascade mechanism mediates BA feedback inhibition of CYP7A1 in the liver( Reference Lu, Makishima and Repa 13 , Reference Goodwin, Jones and Price 14 ). SHP is a negative factor that interacts with many nuclear receptors, including LRH1. SHP inhibits the transactivating activity of LRH1, which binds to the CYP7A1 gene. We observed that rats fed the SP diet exhibited greater expression of SHP. However, the expression of CYP7A1 was higher only in the presence of 0·2 % cholesterol and SP. When CYP7A1 is deficient( Reference Schwarz, Lund and Setchell 23 ), the secondary acid pathway of BA synthesis (CPY27A) compensates by generating and disposing of potentially hepatotoxic oxysterols derived from cholesterol( Reference Bjorkhem 24 , Reference Schwarz, Lund and Lathe 25 ). We examined the expression of cholesterol 27α-hydroxylase (CYP27A) and found no differences among the experimental groups. Furthermore, a SHP-independent mechanism has been described, involving FGF15 as a BA/FXR-induced intestinal hormone that inhibits CYP7A1 gene transcription in the liver( Reference Inagaki, Choi and Moschetta 16 ). FGF15 in the intestine is secreted into the circulatory system and then transported to the liver to activate the FGFR4 signalling pathway and inhibit CYP7A1( Reference Yu, Wang and Kan 26 ). We detected high concentrations of FGF15 only in rats fed the SP diet. Interestingly, in the experimental groups fed diets containing 0·2 % cholesterol, FGF15 was not detected. However, when we measured FGFR4 concentrations in the liver of rats fed high-cholesterol diets, the concentration of the FGF15 receptor was found to be low. As a result, there was an increase in the concentration of faecal BA. These findings suggest that SP may regulate cholesterol homeostasis through a SHP-directed mechanism that decreases the expression of SHP and LRH1 and also through an indirect mechanism that decreases the secretion of FGF15 from the intestine and reduces the concentration of FGFR4 in the liver. In both instances, an increase in the expression of CYP7A1, as well as in the synthesis and elimination of BA, led to a decrease in serum cholesterol concentrations.
RCT is generally described as the process by which cholesterol is removed from extrahepatic tissues and returned to the liver for conversion into BA and excretion into bile. Nonetheless, in the intestine, RCT plays a key role in the transport of excess cholesterol in the enterocytes into the intestinal lumen. Several ATP cassette-binding transporters, including ABCA1, ABCG5 and ABCG8, among others, are involved in RCT. It has been established that RCT is an important mechanism by which HDL protects against atherosclerosis( Reference Fielding and Fielding 27 ). Although ABCA1 facilitates the cellular efflux of cholesterol and phospholipids to lipid-poor apoAI or HDL( Reference Oram and Vaughan 28 ), we did not observe a significant change in HDL-cholesterol concentrations in rats fed the SP diet despite the modest increase in the expression of ABCA1 mRNA. On the other hand, there was a small but significant increase in the expression of the ABCG5/ABCG8 transporters involved in the secretion of sterols from the liver and the efflux of dietary sterols from the gut( Reference Berge, Tian and Graf 29 ) to the liver; however, there was a significant increase in the mRNA abundance of both transporters in the intestine of rats fed the SP diet. Interestingly, this difference was magnified in rats fed the SC diet, suggesting that ABCG5 and ABCG8, as well as ABCA1, are regulated by the LXR/retinoid X receptor heterodimer because this nuclear receptor is activated by natural oxysterols( Reference Lee, Lu and Hazard 30 ).
The increase in the excretion of faecal BA, due in part to the increase in BA synthesis promoted by an increase in the expression of CYP7A1 as well as an increase in the expression of the reverse cholesterol transporters in the intestine, could explain the well-established ability of SP to reduce serum cholesterol concentrations. In fact, our data revealed that rats fed a SP diet rich in cholesterol had significantly lower total and LDL-cholesterol concentrations in serum.
On the other hand, it is known that LRH1 interacts with LXR to regulate the expression of SREBP1c, which is a master transcriptional factor that regulates lipogenesis. We observed that SP consumption reduced the expression of LXR and SREBP1c, independently of the presence of cholesterol. These data may explain the reduction in hepatic TAG content observed in rats fed the SP diet. Furthermore, the reduction of SREBP1c expression in the liver of rats fed the SP diet independent of the presence or absence of cholesterol in the diet can be due to a decrease in insulin secretion, as the amino acid profile in the serum of rats fed SP diminishes the release of pancreatic insulin as has been reported previously, a hormone involved in the up-regulation of SREBP1c expression( Reference Noriega-Lopez, Tovar and Gonzalez-Granillo 31 ). Interestingly, the addition of cholesterol to the diets increased the concentrations of hepatic TAG. It has been demonstrated that cholesterol can increase the expression of SREBP1c via LXR ( Reference Lin, Yang and Tarr 32 ), and we observed a significant increase in SREBP1c expression in rats fed the CC diet, although rats fed the SC diet exhibited a modest increase.
In conclusion, there are two possible mechanisms by which SP regulates cholesterol homeostasis: (1) by increasing BA synthesis via a direct SHP mechanism, by decreasing the expression of SHP and LRH1 and consequently increasing the expression of CYP7A1, and by decreasing the secretion of FGF15 and the hepatic receptor FGFR4, which favours an increase in CYP7A1 expression, or (2) by increasing the activity of LXR, leading to lower serum LDL concentrations by increasing the expression of ABCA1 in the liver while promoting RCT in the intestine by increasing the expression of the ABCG5/ABCG8 transporter (Fig. 5). Further studies are needed to understand how isoflavones are involved in these mechanisms.
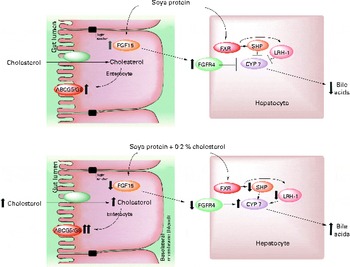
Fig. 5 Proposed model for the mechanism by which soya protein regulates reverse cholesterol transport and bile acid synthesis via fibroblast growth factor 15 (FGF15) and/or SHP after the consumption of soya protein (A) and soya protein plus 0·2 % cholesterol (B) compared with that in the control groups fed casein or casein plus 0·2 % cholesterol, respectively. In the absence of cholesterol in the diet, soya protein stimulates the expression of intestinal FGF15 as well as its hepatic receptor fibroblast growth factor receptor 4 (FGFR4). In addition, soya protein also induces the expression of SHP and liver receptor homolog 1 (LRH1). Both mechanisms decrease the expression of 7α-hydroxylase (CYP7A1) and hence the excretion of bile acids. The addition of cholesterol to a soya protein diet reverses this process by increasing the excretion of faecal bile acids and cholesterol via the up-regulation of the expression of intestinal reverse cholesterol transporters ATP-binding cassette, subfamily G, member 5/8 (ABCG5/ABCG8). FXR, farnesoid X receptor.
Acknowledgements
The present study was supported by the CONACYT grant 154939 to A. R. T. The funder contributed to the study design and conduct and manuscript preparation.
The authors' contributions are as follows: G. L. A.-M. was responsible for the experimental design, analysed the data and wrote the manuscript; N. T. was responsible for the experimental design and data analysis; O. G. wrote the manuscript and analysed the data; B. P.-G. wrote the manuscript and analysed the data; I. M.-V. analysed the data; A. R. T. contributed to the experimental design and discussion and wrote the manuscript.