Impact statement
Climate change and demographic developments increase societal risk related to floods and droughts globally. Extreme events such as heavy downpours, and prolonged periods of droughts are getting more common, often occurring both in the same area and in different seasons. To improve the resilience of society to both events nature-based solutions (NbS) are often proposed as an alternative or complementary solution in flood and drought management strategies. Not only do NbS provide a solution for the primary goal of flood risk reduction and/or drought risk reduction, they may also provide a net-biodiversity gain and a multitude of other co-benefits to society. Yet water management, climate adaptation and spatial planning strategies often have a strong focus on either flood OR drought risks, rather than assessing their impacts together within the same landscape in an integrated approach. As a result, current knowledge on the effectiveness of NbS is also limited to a single-domain perspective of either flood OR drought.
In this review we address the need for a more integrated approach for evaluating water management solutions, with a focus on the role of NbS in reaching multiple goals: flood resilience AND drought resilience AND biodiversity gain. All three aspects should be evaluated in an integrated manner if we want to truly understand the merits of NbS in comparison to ‘grey’ engineering solutions. We argue that a good evidence base is still lacking in which all three aspects are well recorded within single cases. Studies often lack a proper before-after-control-impact set-up and long-term monitoring to assess changes over time.
We call upon the wider scientific community and water management practitioners to include aspects of flood risks AND drought risks AND biodiversity when selecting measures to improve system functioning.
Introduction
Climate change exacerbates the frequency and intensity of both floods and droughts globally. There is an urgent need for large-scale adjustments in catchments to adapt to these changes. Many catchments currently face an increase in hydrometeorological extreme events, ranging from frequent short intensive peaks in discharge during heavy rain events, to prolonged and more frequent dry periods (Cook et al., Reference Cook, Mankin, Marvel, Williams, Smerdon and Anchukaitis2020; He and Sheffield, Reference He and Sheffield2020; Vicente-Serrano et al., Reference Vicente-Serrano, Peña-Angulo, Beguería, Domínguez-Castro, Tomás-Burguera, Noguera, Gimeno-Sotelo and El Kenawy2022). Combined with increased human impacts on the landscape -such as intensive drainage schemes and unsustainable agricultural practices- this leads to increased flood risks and prolonged periods of low groundwater levels, reduced, or even halted baseflow, soil degradation and desertification.
The compounded impacts of climate change and human-modified landscapes may result in reductions in drinking water availability, water quality, soil water content and productivity, food and energy production and habitat/biodiversity. For example, Duel et al. (Reference Duel, Wolters, Timboe, Ter Maat and Matthews2022) report that at least 1.5 billion people worldwide have been directly affected by droughts this century and the economic cost estimates are above US$124 billion. Toreti et al. (Reference Toreti, Masante, Acosta Navarro, Bavera, Cammalleri, De Jager, Di Ciollo, Hrast Essenfelder, Maetens, Magni, Mazzeschi, Spinoni and De Felice2022) indicated that during the summer of 2022 47% of Europe’s area was in warning conditions, meaning that the soil had dried up and 17% was on alert, with vegetation showing signs of stress. At the same time biodiversity decline is increasing strongly, with both the extent of area of valuable ecosystems, and individual species abundance in decline and many species under threat of extinction (IPBES, Reference Brondizio, Settele, Díaz and Ngo2019). For example, IPBES (Reference Brondizio, Settele, Díaz and Ngo2019) reports that only 13% of the wetlands present in 1700 still existed in 2000, and global forest area now covers only 68% of the pre-industrial forest area. Human impact on individual species presence and abundance is seen in all species groups, but is especially prominent in insect communities, with reports of up to 70% decline in biovolumes in specific locations (Wagner, Reference Wagner2020). Due to climate change, these processes of increased droughts, floods and biodiversity decline will further increase in the future (IPCC, 2021).
The concept of improving the water retention capacity of catchments – here called the ‘sponge functioning of catchments’- is widely recognised as a way to smooth peaky hydrographs, by reducing and delaying peak flows and stimulating infiltration to the groundwater and policies and plans are developed in order to increase this sponge functioning (e.g., Ministerie voor Infrastructuur en Waterstaat/DGWB et al., 2022, UK Gov., 2020). This approach is claimed to decrease overall flood volumes, improving baseflows, soil moisture retention capacity and groundwater levels, especially in smaller catchments. Consequently, investing in the sponge functioning of catchments may reduce the frequency and severity of both flood and drought-related extreme events.
However, in some cases measures that are taken to increase sponge functioning and reduce droughts may actually increase flood peaks due to the reduced storage capacity or reduced discharge rate of areas or the changed timing of discharge peaks in relation to other tributaries in rivers. Therefore, effects of measures on droughts and flood peaks of different sizes (and biodiversity) cannot be analysed in isolation (Fennell et al., Reference Fennell, Soulsby, Wilkinson, Daalmans and Geris2023)
To improve the sponge functioning in catchments, surface water retention and groundwater infiltration solutions are suggested that rely on preserving, restoring or enhancing natural ecosystems and their functioning. These solutions are often referred to as ‘nature-based solutions’ (NbS, following the definition of UNEA 2022)Footnote 1. Other terms such as ‘Green Infrastructure’Footnote 2 (GI), ‘Natural Water Retention Measures’Footnote 3 (NWRM) or ‘Natural Flood Management’ (NFM)4 are also being used to describe these NbS or subsets there of (Cohen-Shacham et al., Reference Cohen-Shacham, Walters, Janzen and Maginnis2016). Reconnection of floodplains, restoration of wetlands and introduction of natural water retention ponds are some examples of the methods that can be used (Hare et al., Reference Hare, McBride, Addy, Pakeman, Donaldson-Selby, Rivington, Gagkas, Jabloun, Wilkinson, Lilly and Akoumianaki2022). These interventions to improve natural water retention capacity also help maintain ecosystem functioning and biodiversity (Collentine and Futter, Reference Collentine and Futter2018) and are context specific: improving sponge functioning must take into account the characteristics of soils and ground- and surface water systems under different land-uses, climatic zones, geology and topographic contexts. The individual measures include improvements of farming practices and soil management strategies (Keesstra et al., Reference Keesstra, Nunes, Novara, Finger, Avelar, Kalantari and Cerdà2018; Sonneveld et al., Reference Sonneveld, Merbis, Alfarra, Ünver and Arnal2018; Miralles-Wilhelm, Reference Miralles-Wilhelm2021), stream- and soil structure to slow down, absorb and reduce runoff, innovative and traditional managed aquifer recharge (MAR) techniques (Martos-Rosillo et al., Reference Martos-Rosillo, Ruiz-Constán, González-Ramón, Mediavilla, Martín-Civantos, Martínez-Moreno, Jódar, Marín-Lechado, Medialdea, Galindo-Zaldívar, Pedrera and Durán2019), conservation and restoration of ecosystems such as forests, peatlands and wetlands, and reconnection of floodplains to provide more room for streams and river flood volumes (Iacob et al., Reference Iacob, Rowan, Brown and Ellis2014).
Many of the smaller-scale individual solutions are ideally implemented at landscape scale as a combination of individual solutions in overarching strategies using an ecosystem-based approach (Cohen-Shacham et al., Reference Cohen-Shacham, Andrade, Dalton, Dudley, Jones, Kumar, Maginnis, Maynard, Nelson, Renaud, Welling and Walters2019). The European Landscape Convention (ETS, 2000) introduced a definition of landscape as “an area, as perceived by people, whose character is the result of the action and interaction of natural and/or human factors”. A landscape scale is therefore a manageable unit of scale that is substantially larger than an individual plot of land, or stream stretch, and normally smaller than an entire river catchment from source to sea, in which relevant ecosystem processes, resource use and policy objectives take place, and that allows the intervention of stakeholders at different levels (local, basin, regional).
However, there is a lack of understanding of NbS effectiveness under normal and extreme hydrometeorological conditions in building resilience to floods AND droughts, and in providing benefits to biodiversity. Sarabi et al. (Reference Sarabi, Han, Romme, de Vries, Valkenburg and den Ouden2020) found that next to political and institutional barriers, this lack of knowledge is an essential barrier that hampers the choice for their implementation. Black et al. (Reference Black, Peskett, MacDonald, Young, Spray, Ball, Thomas and Werritty2021) further conclude based on studies from the United Kingdom that the effectiveness of many of the implemented measures is not properly monitored and evaluated. As a result, it is difficult to determine whether they have improved sponge functioning in terms of, for example, their development over time, response to extreme events, and maintenance. A literature review of drought risk assessments by Hagenlocher et al. (Reference Hagenlocher, Meza, Carl Anderson, Fabrice, Walz and Sebesvari2019) revealed a knowledge gap in our understanding of the role of ecosystems in drought risk and their opportunity for increasing drought resilience. At the same time, the impact of drought measures on the response of the landscapes to floods is normally not reported on. Yet, such measures (such as removal of drains or closing of drainage ditches) will most likely lead to more frequently inundated lands. This lack of understanding also hampers the assessment of NBS effectiveness in comparison with traditional grey-engineered solutions (Lane, Reference Lane2017). Grey solutions are defined as human-engineered traditional approaches to water management such as pipes, hard surfaces, seawalls and related technically engineered and constructed hard features (Kapetas and Fenner, Reference Kapetas and Fenner2020; Singhvi et al., Reference Singhvi, Luijendijk and Van Oudenhoven2022).
We distinguish four main categories of NbS to improve the sponge functioning in catchments:
-
1. NbS focusing on the conservation of ecological functioning of landscapes and preserving the ecosystem services that are there.
-
2. NbSaiming to restore the ecological functioning where it was lost. For example, rewetting of wetlands, reconnecting floodplains, and afforestation are commonly suggested measures to do so.
-
3. Nbs which enhance the ecological functioning through deliberate management to allow the landscape to better perform under floods and/or droughts (such as implementation of natural water retention measures, buffer strips and soil improvements);
-
4. NbS which apply Ecosystem-based adaptation (EbA) practices that focus on sustainable use of ecosystem services to reduce vulnerability and build resilience to climate change.
EbA promotes using practices that focus on sustainable and climate-smart agriculture (Vignola et al., Reference Vignola, Harvey, Bautista-Solis, Avelino, Rapidel, Donatti and Martinez2015; Chandra et al., Reference Chandra, McNamara and Dargusch2018), integrated water resource management and sustainable forest management. EbA takes into account impact of expected climatic change, for example, through improvement of soil organic carbon content in agricultural practices or change of crops to better fit within the conditions the landscape provides: paludiculture in very wet zones, and drought resistant crops in areas prone to low groundwater levels.
This paper aims to address the question of whether currently reported NbS to improve sponge functioning are being sufficiently well evaluated on their multiple benefits for flood risk reduction, drought risk reduction and biodiversity. It addresses the need for a more integrated approach to evaluate the effectiveness of NbS for water management in reaching multiple goals, namely flood resilience AND drought resilience AND biodiversity gain. This is required to support well-informed decisions on designing, implementing and upscaling NbS strategies. This paper focuses predominantly on rural areas at catchment scale in regions where both floods and droughts can occur. Although ecosystems may contribute to alleviating the sharp peaks of these events and recharge surface and groundwater systems, these ecosystems are also vulnerable to flood and drought hazards, which may affect their functioning and the overall biodiversity values that they represent.
The remainder of this paper is structured as follows. In the next section, we first provide an overview of the functioning of NbS for the individual aspects of reducing flood risk, drought risk or biodiversity gain. We then evaluate the available evidence on the combined evaluation of NbS for floods AND droughts AND biodiversity as reported in literature and in available databases with case study examples of NbS projects worldwide. Based on this analysis we present a way forward and draw conclusions on the combined evaluation of NbS for improving sponge functioning at landscape scale.
NbS for reducing flood risks
Flood management may apply NbS to alleviate flood risks, for example, in the NFM (Lane, Reference Lane2017), Natural Water Retention Measures (Collentine and Futter, Reference Collentine and Futter2018) and large-scale river management programs such as Room for the River (Klijn et al., Reference Klijn, Asselman and Wagenaar2018) and the German Blue Belt (BMVI and BMU, 2020). These programs involve allocating more space to the water system, enabling it to retain a greater volume and consequently reduce the velocity and height of the peak discharge or river water levels. For example, Black et al. (Reference Black, Peskett, MacDonald, Young, Spray, Ball, Thomas and Werritty2021) show NbS in the Eddleston catchment slow down peaks between 2–7 hours compared to the baseline. Precipitation surpluses or river discharges can be stored in the soil (groundwater), in surface waters or above ground. Such storage capacity can have a dual purpose for both flood control and water conservation. The latter preserves water during periods with a water surplus to have this available during periods with water shortage.
However, when storage is limited to confined areas, water retention may bring about unnatural fluctuations of the water level, and sometimes a change in the chemical water quality. Important factors determining the impact of water retention are the (biophysical) storage place characteristics, the storage time (duration and period) and water volume. For example, rainwater can be retained where it falls or can be stored in dedicated places (areas assigned within the landscape). Often more natural areas, such as forests and wetlands, are designated to retain and store large volumes of water. In case water is let into a natural area with a high sensitivity to droughts (such as peatlands and wetlands) this can also help prevent drought damage to the natural values in these areas. Taking these characteristics into account it is possible to subdivide the above-mentioned examples in four types of water retention and storage (based on Penning and Duel (Reference Penning and Duel2003)), see Table 1.
Table 1. Overview of water retention types
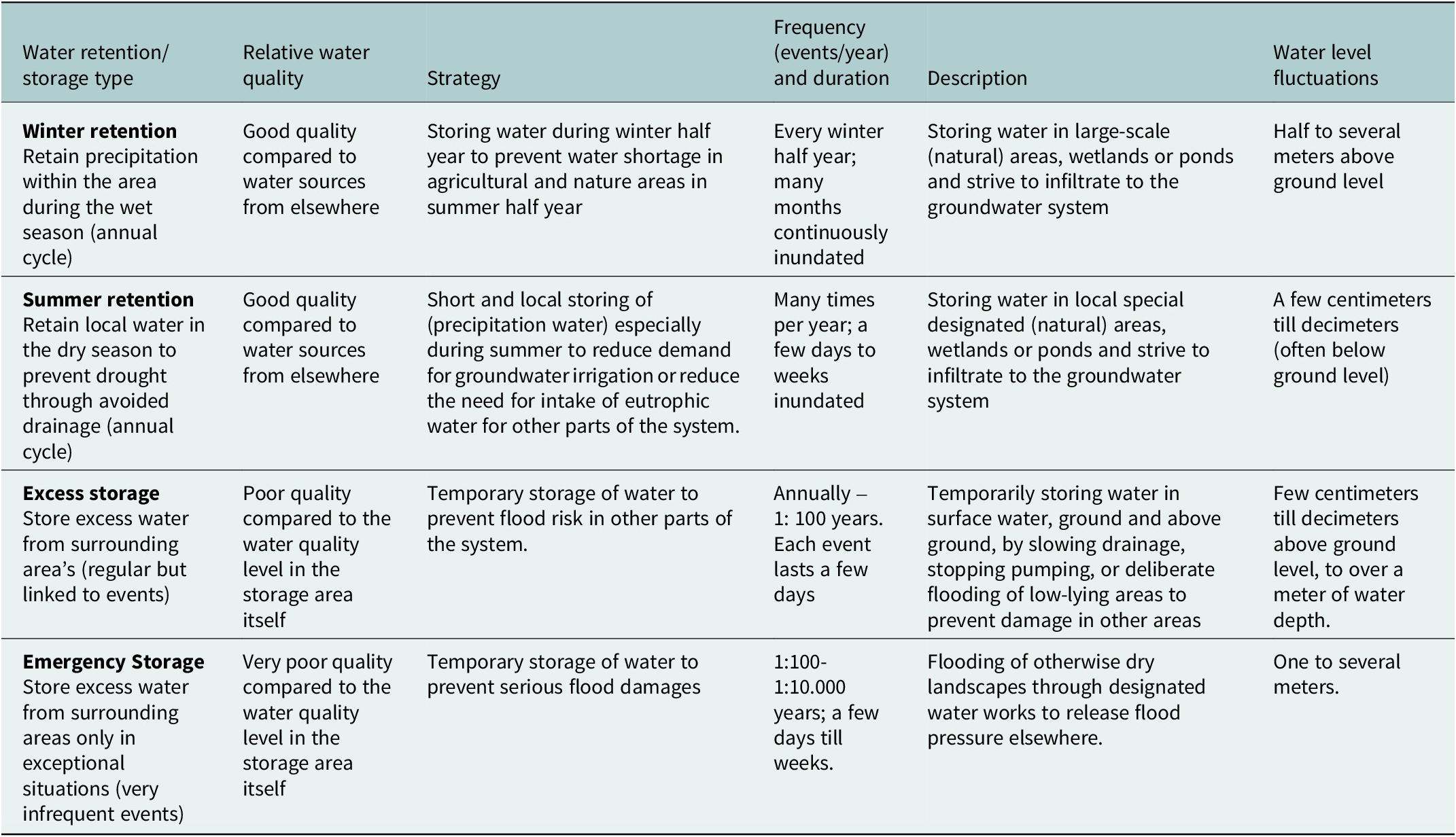
Measured evidence of the functioning, effectiveness, and benefits of NbS for floods is rarely published (Black et al., Reference Black, Peskett, MacDonald, Young, Spray, Ball, Thomas and Werritty2021). Kay et al. (Reference Kay, Old, Bell, Davies and Trill2019) estimate that fewer than 25% of NFM projects in the UK give evidence of effectiveness based on observational data. Most cases only measured reduction in flood peak levels, recorded as a percentage reduction or delay in hydrographs. The results of this study also show that NFM measures are much less likely to offset the impacts of climate change for later time-slices and higher emissions scenarios, and that the chance of offsetting the impacts of climate change in any individual catchment will depend on its type (how sensitive it is to climatic changes) and its location (due to spatial variation in climatic changes) (Kay et al., Reference Kay, Old, Bell, Davies and Trill2019).
Evidence related to NWRM/NFM for flood peak reduction is frequently reported in studies originating from the United Kingdom, where this topic has received considerable attention. The Environment Agency set up a dedicated program on NFM from 2017–2021, using 60 pilots across England allowing for this evidence base to grow and be better evaluated (EA, 2017; Hare et al., Reference Hare, McBride, Addy, Pakeman, Donaldson-Selby, Rivington, Gagkas, Jabloun, Wilkinson, Lilly and Akoumianaki2022). The recent evaluation from the Environment Agency on this project concluded that NFM can reduce flood risk and contribute to nature recovery and climate resilience (EA, 2022). Data from other countries and climatic zones is less systematically reported and not frequently reported in scientific journals as operational managers do not consider such publications as a necessity for their regular practice. Information on the experience resulting from essential maintenance over time to support the longevity of these measures is therefore also lacking in scientific literature, nor is there information on how the measures deal with extreme events beyond those they were designed to cater for, such as multi-year droughts, 1:1000-year floods, or exceptional rainfall such as the summer 2021 event in Germany/Belgium/Netherlands (Fekete and Sandholz, Reference Fekete and Sandholz2021).
Reporting evidence on flood peak reduction is most common in the documented literature, yet often information on the type of peak that was reduced is missing. Iacob et al. (Reference Iacob, Rowan, Brown and Ellis2014) do show there is a significant difference in the effectiveness of flood peak reduction depending on the type of event, with the studied NBS being more effective for a 1 in 2-year event than for a 1 in 50–200-years flood event. Therefore, without understanding if the peak was reduced for a 1 in 2-year event or a 1 in 50-year event the impact of the NBS remains vague in terms of effectiveness in relation to set management goals. Reporting both expected and monitored effects is thus best done in relation to the type of event or design discharge.
NBS for reducing drought risks
NbS implementation cases for drought risk management are still limited and mainly focused on pilot studies rather than on large-scale implementation. Additionally, most of these implementations are reported in the Global South (Kalantari et al., Reference Kalantari, Santos Ferreira, Keesstra and Destouni2018; www.justdiggit.nl, www.theweathermakers.nl). Sahani et al. (Reference Sahani, Kumar, Debele, Spyrou, Loupis, Aragão, Porcù, Aminur Rahman Shah and Di Sabatino2019) reported that in an analysis of 205 case studies of NbS only 3.4% of the cases NbS were used for drought risk management. Model-based case studies exist that explore the potential benefits of NbS to reduce the societal costs of droughts, for example, in Sao Paulo (Ciasca et al., Reference Ciasca, Klemz, Raepple, Kroeger, Acosta, Cho, Barreto, Bracale and Cesário2023). A review of drought vulnerability and risk assessments, however, showed that most of them focus on the social dimension and impact on societies and not specify sufficiently what type of drought hazard is assessed or how the impact of the drought was related to the type and quality of the local ecosystems (Hagenlocher et al., Reference Hagenlocher, Meza, Carl Anderson, Fabrice, Walz and Sebesvari2019).
Three types of droughts are commonly distinguished (Wilhite et al., Reference Wilhite, Sivakumar and Pulwarty2014; Van Loon et al., Reference Van Loon, Stahl, Di Baldassarre, Clark, Rangecroft, Wanders, Gleeson, Van Dijk, Tallaksen, Hannaford, Uijlenhoet, Teuling, Hannah, Sheffield, Svoboda, Verbeiren, Wagener and Van Lanen2016; Duel et al., Reference Duel, Wolters, Timboe, Ter Maat and Matthews2022): meteorological drought (precipitation deficiency over an extended period of time), soil moisture/agricultural drought (lack of availability of soil water to support crop and forage growth) and hydrological drought (when surface and subsurface water supplies start falling below a threshold), resulting in ecological and socio-economic impacts. Socio-economic drought is sometimes added as a fourth drought type that results from the other three and is associated with the supply of some commodity or economic good (and its dependence on water supply). Drought risk is understood as the integration of all socio-economic and ecological consequences of drought over a wide range of conditions. Drought impacts include crop losses and shipping delays, indirect effects on the socio-economic system (such as food insecurity, income losses and unemployment), and intangible effects such as deteriorated water quality and biodiversity loss (Logar and van den Bergh, Reference Logar and Van den Bergh2013; Mens et al., Reference Mens, Van Rhee, Schasfoort and Kielen2022).
NbS have the potential to reduce the frequency and severity of hydrological drought as well as the ecological consequences by enhancing groundwater recharge, improving base flows during dry periods, increasing the storage capacity of soils, and improving water quality through sustainable land management. For example, reforestation with trees that match the environmental conditions may increase groundwater recharge, providing springs and rivers with sufficient base flow during dry periods (Price, Reference Price2011). Other examples to increase natural (groundwater) recharge areas include floodplains, natural water retention ponds and upstream wetland areas. NbS influence the propagation of the drought through the hydrological system by changing the hydrological regime of surface and groundwater within a catchment, potentially increasing water availability for ecosystems as well as humans.
However, changing vegetation (either in response to climate change or human-induced) may also increase the frequency and severity of hydrological droughts. Bouaziz et al. (Reference Bouaziz, Aalbers, Weerts, Hegnauer, Buiteveld, Lammersen, Stam, Sprokkereef, Savenije and Hrachowitz2022) found that the larger root zone storage capacities (+34 %) in response to a more pronounced climatic seasonality with warmer summers under 2 K global warming result in strong seasonal changes in the hydrological response of the Meuse River in Europe, leading to a decline of groundwater storage and reduced summer flows. Also, reforestation of agricultural land influences various aspects of the hydrological cycle (evaporation, infiltration, runoff) in positive as well as negative ways, but the long-term effects on water yield, including streamflow in the dry season, often remain unclear (Cunningham et al., Reference Cunningham, Mac Nally, Baker, Cavagnaro, Beringer, Thomson and Thompson2015).
NbS relevant for drought risk management must be considered in relation to the context-specific climatic zone and type of drought. Figure 1 shows that in a survey using references collected by the Oxford Evidence Base Database there is only a limited number of NBS cases for drought risk management (42). When split per climate zones and/or by the type of drought which they mitigate, the number of studies reduces even further. Most of the published NBS cases with positive effects on droughts, reduce water shortage and/or desertification and can be found in the temperate climate zone followed by cases in the tropical climate zone.
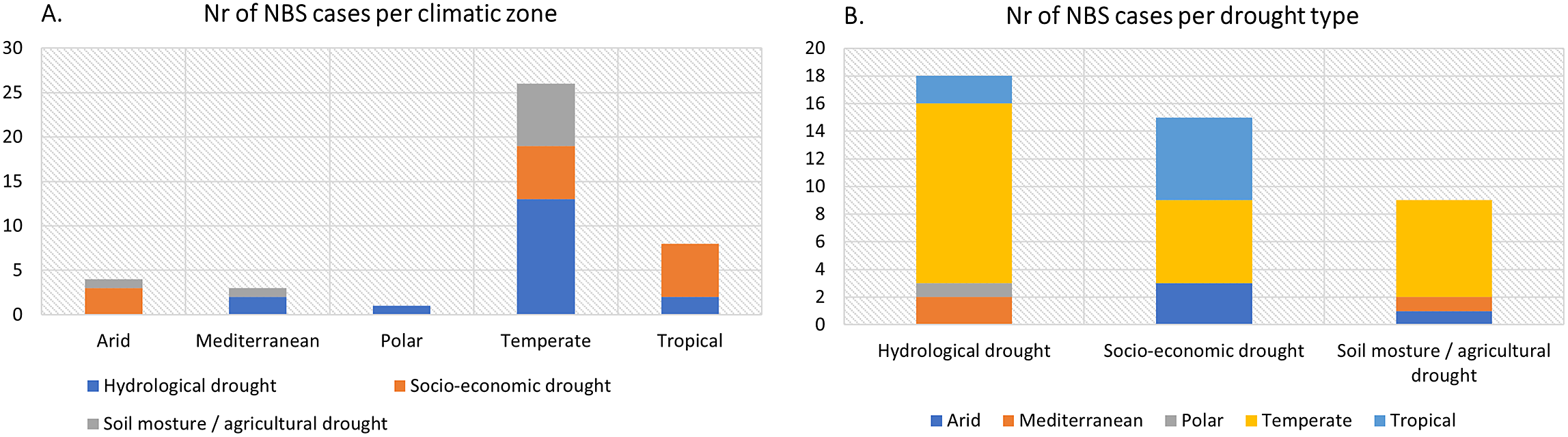
Figure 1. (a) Number of published NbS cases with positive effects on climate change impacts (reduced water availability, drought, desertification) implemented in different major climate zones (N = 42 cases). (b) Number of published NbS cases with positive effects on climate change impacts per drought category (N = 42 cases). Data obtained from the Nature-based Solutions Evidence Platform of the University of Oxford https://www.naturebasedsolutionsevidence.info/.
NbS that may contribute to drought resilience focus on retaining water and avoiding unnecessary loss through inbalanced evapotranspiration. These NbS should therefore always be evaluated within a larger strategy in which individual measures are combined. Drought risk reduction through NbS include actions to protect, restore, conserve, and sustainably use and manage ecosystems as pastoral and agricultural land, wetlands, forest and natural parks, watersheds, floodplains, and setback levees. Groundwater recharge can be enhanced by maintaining natural recharge areas, such as floodplains, natural water retention ponds and traditional recharge channels) and upstream wetland areas (Browder et al., Reference Browder, Ozment, Rehberger Bescos, Gartner and Lange2019; Kupika et al., Reference Kupika, Gandiwa, Nhamo and Kativu2019; Jódar et al., Reference Jódar, Martos-Rosillo, Custodio, Mateos, Cabello, Casas, Salinas-Bonillo, Martín-Civantos, González Ramón, Zakaluk, Herrera-Lameli, Urrutia and Lambán2022). Native forests can also contribute to increased water availability by increasing storage capacity, improving base flows during dry periods, and enhancing water quality (Woldie and Tadesse, Reference Woldie and Tadesse2019). Bypass floods can hold a volume bigger than the volume of the river and convey a high percentage of its floodwaters during large events (Browder et al., Reference Browder, Ozment, Rehberger Bescos, Gartner and Lange2019).
Additionally, NbS related to landslides, land use changes and reforestation may also decrease drought risk (Fedele et al., Reference Fedele, Locatelli, Djoudi and Colloff2018). Hillsides with radical terraces have shown an increase of the soil and water conservation rate, improving environmental resilience, soil erosion, crop yields, and water loss, but also improving livelihood of farmers (Mupenzi et al., Reference Mupenzi, Li, Jiwen, Habumugisha, Dieu, Habiyaremye, Jean and Innocent2012). Fedele et al. (Reference Fedele, Locatelli, Djoudi and Colloff2018) showed that local people directly benefited from nature-inclusive land use changes that promote resilience to climate change such as reforestation of low productive agricultural fields and planting trees in gardens. These actions affected the perceptions of the wider stakeholder community, facilitating scaling up of these solutions to landscape scale, thereby improving the wider hydrological functioning in the area. Reforestation using trees that match the environmental conditions has a potential role in increasing groundwater recharge, providing springs and rivers with base-flow discharge during the dry period (Woldie and Tadesse, Reference Woldie and Tadesse2019).
NBS for biodiversity gain
Both terrestrial and aquatic biodiversity values can be maintained, preserved or improved through optimal water management and many methods and definitions exist to express what biodiversity encompasses (Moss et al., Reference Moss, Hering, Green, Adoud, Becares, Beklioglu, Bennion, Boix, Brucet, Carvalho, Clement, Davidson, Declerck, Dobson, Van Donk, Dudley, Feuchtmayr, Friberg, Grenouillet, Hillebrand, Hobaek, Irvine, Jeppesen, Johnson, Jones, Kernan, Lauridsen, Manca, Meerhoff, Olafson, Ormerod, Papastergiadou, Penning, Ptacnik, Quintana, Sandin, Seferlis, Simpson, Trigal, Verdonschot, Verschoor and Weyhenmeyer2009). In general, the diversity in genetic resources, species, habitats and regional characteristics all contribute to an overarching expression of biodiversity (Whittaker, Reference Whittaker1972) and biodiversity is directly linked to the ecological functioning of ecosystems and responds to drivers and pressures affecting these ecosystems. In freshwater systems concepts of, for example, environmental flow requirements (Tharme, Reference Tharme2003; Acreman and Dunbar, Reference Acreman and Dunbar2004) or functional flow requirements (Yarnell et al., Reference Yarnell, Petts, Schmidt, Whipple, Beller, Dahm, Goodwin and Viers2015) are defined to support the overarching biodiversity values in the river systems. The role of anthropogenic influence on these flow regimes is evident and linked to both surface-water and groundwater management. For example, Hendriks et al. (Reference Hendriks, Kuijper and Van Ek2014) show for two temperate sandy catchments that anthropogenic alterations (drainage) caused 25–40% reduced baseflows and groundwater abstraction caused 5–28% of reduction in baseflow. Predictions of the response of these systems under climate change resulted in an additional baseflow reduction of 33–70% by 2050.
Large-scale floods are part of the natural dynamics of riverine landscapes and create much-needed disturbance to set back vegetation development and rejuvenate freshwater habitats, thereby creating space for pioneer species and enhancing the overall biodiversity at a landscape scale (Baptist et al., Reference Baptist, Penning, Duel, Smits, Geerling, Van der Lee and Van Alphen2004; Stoffers et al., Reference Stoffers, Collas, Buijse, Geerling, Jans, Van Kessel, Verreth and Nagelkerke2021). At the same time, floods may temporarily affect existing biodiversity values. For example, Zhang et al. (Reference Zhang, Li, Ge, Chen, Xu and Guan2021) evaluate the role of extreme floods on terrestrial animals and conclude that the extent of the impacts on animals in the flooded areas depends on four main factors: (i) the ratio of flood duration to the survival time of animals in the flood, (ii) the ratio of flood depth to plant height, (iii) the migration ability of animals and (iv) temperature at the time of the event. In addition to these four indicators, it is however essential that indicators related to resilience, the potential for recovery, recovery time, susceptibility to the invasion of allochthonous species more adapted to floods/drought, recolonisation speed, connectivity, and the area of newly created habitats after a flood should also be included in such evaluations.
Large-scale NbS projects that are focused on conservation and restoration of the ecological functioning in landscapes will yield substantial benefits towards enhancing biodiversity. For instance, large-scale re-meandering of streams will improve instream habitat diversity and thereby the expected number of species present in the stream (Lorenz et al., Reference Lorenz, Jähnig and Hering2009; Garcia et al., Reference Garcia, Schnauder and Pusch2012) and riparian zones increase the regional species richness by harbouring different -not more- species (Sabo et al., Reference Sabo, Sponseller, Dixon, Gade, Harms, Heffernan, Jani, Katz, Soykan, Watts and Welter2005). Also, smaller-scaled NbS (e.g., related to wetland creation in agricultural landscapes, environmentally friendly and climate-smart agricultural practices) may have a positive effect on biodiversity (Thiere et al., Reference Thiere, Milenkovski, Lindgren, Sahlén, Berglund and Weisner2009; Tumwesigye et al., Reference Tumwesigye, Aschalew, Wilber, Atwongyire, Nagawa and Ndizihiwe2019), but as the overarching impact of an NbS on biodiversity is dependent on the size and location of the NbS within an otherwise altered landscape, the impact will be according to that scale of implementation. In addition, Seddon et al. (Reference Seddon, Chausson, Berry, Girardin, Smith and Turner2020) point out that NbS for climate mitigation through afforestation with non-native monocultures can reduce biodiversity values and result in maladaptation. Such proposed measures to capture carbon should therefore not be called true NbS following the Global Standard as defined by IUCN (2020).
On the contrary, projects that primarily aim to restore ecosystems from a biodiversity standpoint may overlook the fact that they can also contribute to achieving multiple objectives. For example, these ecosystem restoration projects can contribute to climate mitigation or other Green Deal Goals and these projects can further optimise their design to contribute to more of these goals (Buijse et al., Reference Buijse, Penning, Alatalo, Andrzejewska, Anlauf, Anton, Baattrup-Pedersen, Bañanres, Birk, Boets, Brandao, Cerqueira Correia, Colls, de Lange, Dias, Drexler, Ecke, Elosegi, Ferreira, Forio, Fuchs, Funk, Geerling, Gerisch, Gerner, Goethals, Grossman, Gruber, Hein, Henriques, Hering, Hershkovitz, Hunter, Jägrud, Jarak, Kajner, Karnatz, Katz, Kempter, Mateljak, Meissner, Nørgaard, Pickard, Pietilä, Portela-Pereira, Puiu, Rankinen, Ratner, Rodríguez-González, Ronkainen, Ronkanen, Rudziński, Sá, Samu, Schneider, Scrieciu, Sime, Sommerhäuser, Streater, Tögel, Trandziuk, Udklit, Unzurrunzaga, Wijnen and Wilińska2022). An example of this is the Dutch Room for the Rivers where flood mitigation and strengthening of the natural values in the floodplains were combined in one single large-scale strategy (Klijn et al., Reference Klijn, Asselman and Wagenaar2018), where cyclic floodplain rejuvenation was suggested as a way to also deal with the developing nature of the NbS based strategy (Baptist et al., Reference Baptist, Penning, Duel, Smits, Geerling, Van der Lee and Van Alphen2004). Yet this program was not designed to also think about the impact of increased droughts due to climate change (Buijse et al., Reference Buijse, Penning, Alatalo, Andrzejewska, Anlauf, Anton, Baattrup-Pedersen, Bañanres, Birk, Boets, Brandao, Cerqueira Correia, Colls, de Lange, Dias, Drexler, Ecke, Elosegi, Ferreira, Forio, Fuchs, Funk, Geerling, Gerisch, Gerner, Goethals, Grossman, Gruber, Hein, Henriques, Hering, Hershkovitz, Hunter, Jägrud, Jarak, Kajner, Karnatz, Katz, Kempter, Mateljak, Meissner, Nørgaard, Pickard, Pietilä, Portela-Pereira, Puiu, Rankinen, Ratner, Rodríguez-González, Ronkainen, Ronkanen, Rudziński, Sá, Samu, Schneider, Scrieciu, Sime, Sommerhäuser, Streater, Tögel, Trandziuk, Udklit, Unzurrunzaga, Wijnen and Wilińska2022). Environmental protection laws and strategies can be a great aid in choosing an NbS, for instance, both the European Green Deal (EC, 2019) and the European Water Framework Directive (WFD) (2000/60/EC, European Commission, 2000) can be of great help in stimulating the restoration of the natural functioning of ecosystems and selecting appropriate strategies to do so.
Available evidence on combined effects
We evaluated the existing evidence base for combined evaluation of the performance of NBS for floods AND droughts AND biodiversity by reviewing both existing online databases with example cases throughout the world and specifically searching additional literature on evaluated combined effects of NbS with a focus on NbS at landscape scale outside the urban domain.
Databases on NBS examples
There are many successfully implemented NbS reports, in many different environments globally. We identified nine web-based datasets that provide access to case studies of implemented NbS and that give a certain level of classification to which risk-reducing goals the NbS is related to (e.g., flood, drought, erosion, water quality) and whether biodiversity, social benefits and carbon sequestration are also mentioned as goals. In these web-based datasets (Table 2), a total of 4.289 documented NbS case studies are collected, with most cases reported from Europe and the Global South. In the majority of these cases multiple goals are indicated as relevant, sometimes with a split between a primary benefit and associated co-benefits (world-bank database). A total of 695 cases specifically report that the project has a type of flood risk reduction as prime interest, and 439 mention drought-related challenges. In these projects biodiversity is sometimes mentioned as a primary or co-benefit, but not necessarily. Where biodiversity is a primary goal there are often links with social relevance and a generic restoration of the hydrological cycle without specific focus on flood or drought challenges that are clearly documented (especially in www.nwrm.eu).
Table 2. Overview of case studies reported in databases on NbS. Database No. refers to the list of visited databases below the table.
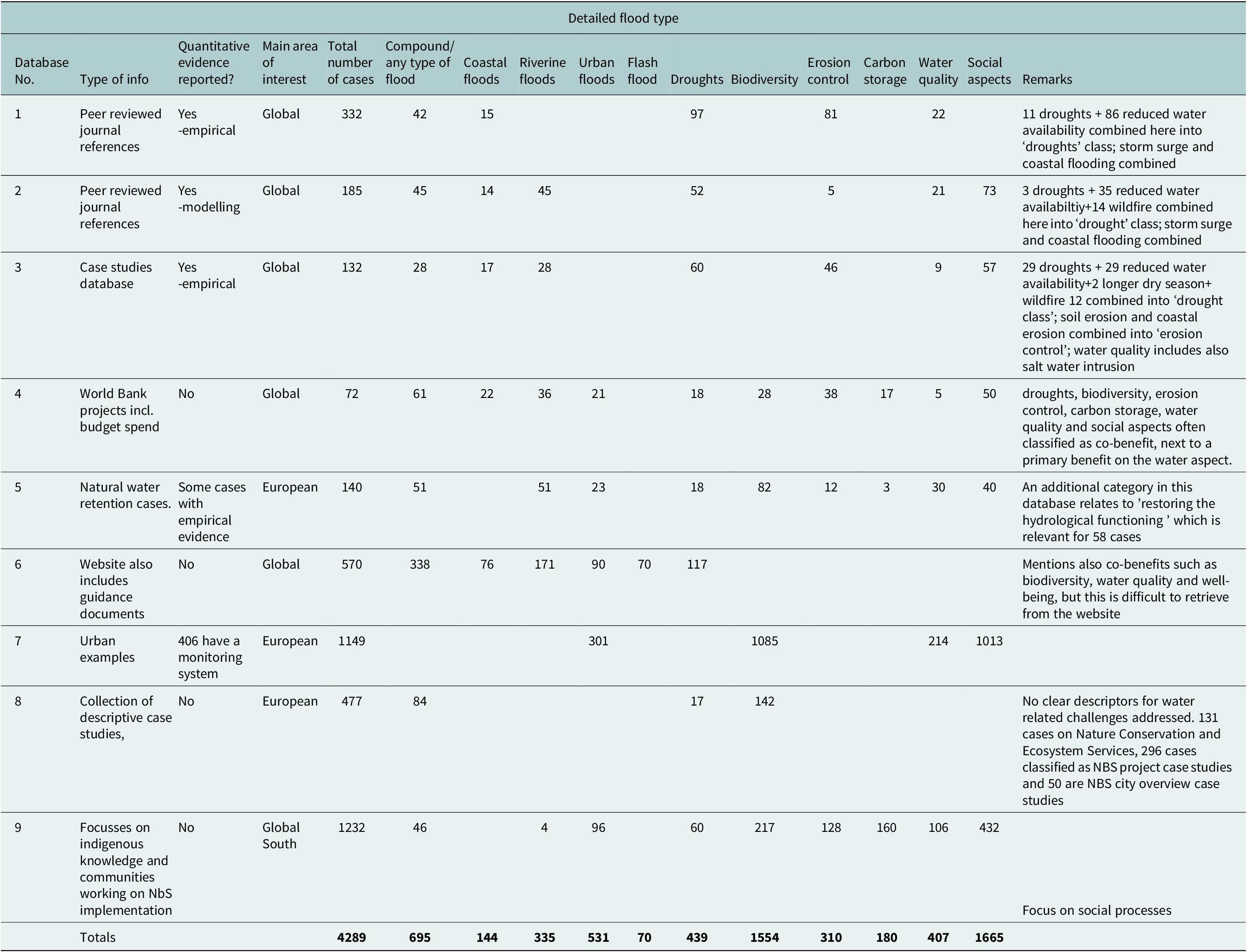
Database No.: Numbers of Used databases in Table 1.
1. https://www.naturebasedsolutionsevidence.info/evidence-tool/reports.
2. https://www.naturebasedsolutionsevidence.info/evidence-tool/.
3. https://casestudies.naturebasedsolutionsinitiative.org/case-search/.
4. https://naturebasedsolutions.org/projects.
5. http://nwrm.eu/.
6. https://geoikp.operandum-project.eu/NbS/explorer.
7. https://naturvation.eu/atlas -=> transferred to https://una.city/.
8. http://oppla.eu/case-study-finder.
9. https://www.equatorinitiative.org/knowledge-center/nature-based-solutions-database/.
Non-included but visited databases:
https://platform.think-nature.eu/case-studies (powered by oppla) selecting flood and biodiversity results in 41 cases, with no quantitative evidence easily retrievable (flood alone 3 cases).
https://www.urbangreenbluegrids.com/ case study hub focused predominantly on Dutch urban NBS cases with little evidence base, and often very small scale examples.
The various databases differ in the goals they document, the classifications used for expressing the objectives and the level of detail with which this is done, making an overall comparison challenging. The evidence base reported in the databases also fluctuates. For example, the Oxford evidence platform lists and evaluates peer-reviewed journal articles that report both empirical and modelling-based evidence related to specific NBS studies in very many different ecosystems globally and reports on 132 case studies (in three different parts of the database). The GeoIKP-Operandum database focusses on reporting relevant hazards in relation to the NbS. In contrast the Equator-Initiative database focusses on showing the potential of local communities in realising NbS with an emphasis on social and biodiversity benefits. The World Bank database is the only database that clearly distinguishes primary goals and co-benefits of projects but does not contain easily retrievable monitored evidence of the outcomes of the project.
Most of the studied databases contain links to qualitative summary descriptions of the projects and contact details for further enquiry with the case study owners. However, they often do not state if there is quantified empirical data collected through monitoring to support the perceived success of the cases. Only for the Una.city database a total of 406 urban NbS cases report that a monitoring system is in place. Out of the 1.149 cases in this database this is a mere 35%. Almost all of the listed cases appear to have a certain level of success despite it is not being clear what the long-term efficiency of the projects is and how maintenance and finance influences the longevity. Many case studies report a role for local communities, farmers and land-owners in implementation and maintenance, but lack descriptors for how long-term management is arranged and carried out. One exception to this a Latvian case in the nwrm.eu database that reports that the effectiveness of the NbS (sediment-capturing ponds in the Latvian State forests) is dependent on the skills and quality of the work carried out by the professionals constructing the ponds.
The two Engineering with Nature Atlases (Bridges et al., Reference Bridges, Bourne, King, Kuzmitski, Moynihan and Suedel2018; Bridges et al., Reference Bridges, Bourne, Suedel, Moynihan and King2021) together provide the narratives of 119 examples of successful NbS. These examples are not arranged by categorised classes of functioning they address, but by landscape type. Despite the word biodiversity only being mentioned sparsely in the text (0 times in Atlas1, 21 times in Atlas2) the generic values of the natural ecosystem are well expressed (natural used 302/412 times, respectively; ecosystem 51/93 times, respectively). Most cases focus on flood protection and the word drought is only mentioned 3 times in Atlas2 and not at all in Atlas1. No quantified evidence is available in these publications, as they are primarily meant to tell the overarching story for policy makers.
Most cases reported in the studied databases lack quantified, long-term scientific evidence base in their response to combined impact for floods AND droughts AND biodiversity. Two databases (nrwm.eu and the World Bank database) explicitly state multiple goals per case (Table 3). In the nwrm.eu database only 3% (5 out of 140) and in the World Bank database only 9% combine all three objectives. Despite the lack of monitoring many of the NbS cases, especially for flood mitigation through flood peak reduction, or slowing down the peaks, also report in more qualitative – even anecdotical – terms an increase in biodiversity, for instance through the mentioning of observations on increased bird numbers (GWP-CEE, 2015a; 2015b), which is suggesting that also the underlying food web is sufficiently strong to support these bird communities.
Table 3. Overview of recorded cases (#cases) with combined goals for floods (F); droughts (D); and biodiversity (B).

Studies that quantitatively evaluate sponge functioning at landscape scale during both floods and droughts are consistently lacking, and additional processes, such as the potential role of soils in providing a buffering function in relation to hydrometeorological events, are often neglected. Similarly, the link between co-benefits and trade-offs, and the role of stakeholder opinions are very rarely reported in a quantified manner, rather qualitative remarks are often made. For example, GWP-CEE (2015b) gives an overview of 14 cases in central Europe of various small water retention measures and reports on an increase in water retention and a visible increase in biodiversity values, especially in bird fauna. Such down-to-earth cases are often reported in grey literature (in this case GWP- CEE, 2015b) and don’t necessarily make it to overarching evidence data-bases such as https://www.naturebasedsolutionsevidence.info/evidence-tool/ or even the www.nwrm.eu
Combined goals and interaction between NbS for floods and droughts and biodiversity
Hydrological processes are at the heart of the functioning of natural systems at landscape scale and changes in these processes due to external pressures such as climate change and societal developments affect not only that natural system but also the socio-economic aspects related to that functioning. Flood risk, drought risk and biodiversity gain or loss are linked via the cycle of hydrological processes. The four categories of NbS (conservation based, restoration based, enhancing ecosystem functioning based and EbA-based NBS) all influence these hydrological processes in their own distinct manner, being it through conservation of existing values or the restoration thereof, or the enhancement of these processes for optimal performance for the related risk. Where needed adaptation of land-use practices and water use also help stimulate a balance between the natural and socio-economic system (Figure 2).

Figure 2. Central role of hydrological processes in evaluation of NbS functioning for flood and drought risk and resulting impact on biodiversity under the influence of climate change and societal developments.
Yet, few peer-reviewed articles explicitly report on quantified evidence of NbS in their performance for floods AND droughts AND biodiversity and the use of a predefined set of consistent indicators or key performance indicators is often missing (Angelopoulos et al., Reference Angelopoulos, Cowx and Buijse2017). For several large-scale and widely mentioned NbS types, we therefore link a selection of examples to the broader understanding of functioning of ecosystems and the role of NbS in strengthening that functioning.
Reconnecting floodplains and side channels are an important and large-scale type of NbS with a primary function of providing space to store and retain surface water during peak discharges. Giving room to the river (Klijn et al., Reference Klijn, Asselman and Wagenaar2018) also aids natural values in these areas as they are being re-exposed to the natural flood dynamics of the river, which is an essential aspect for their natural functioning. River restoration practices that often primarily served the improvement of biological, morphological and physico-chemical functioning are also only seldom evaluated on those three aspects together. Angelopoulos et al. (Reference Angelopoulos, Cowx and Buijse2017) show that for 671 European case studies on river restoration the majority do not have information on how successful these initiatives have been. Only 20 cases recorded success on the physio-chemical components, 52 on the morphological components and 112 on the biological component. Especially the inadequate monitoring and appraisal of outcomes is a factor hampering the understanding of the success of the intervention.
Reforestation of upstream catchment to slow down rainfall peak discharges is often suggested as a suitable NbS for flood risk reduction. Yet, caution should be exercised in the execution of reforestation efforts and their impact on base flow conditions. Ford et al. (Reference Ford, Laseter, Swank and Vose2011) showed that both the forestry management practice and the type of vegetation planted (native vs. non-native) affect the response of stream flow during wet and dry years, and how this changes under climate change. The authors report that managed pine forests generate lower base flows in both wet and dry years compared to a reference situation of unmanaged catchments. Also, Iacob et al. (Reference Iacob, Rowan, Brown and Ellis2014) note that although there are clear benefits in increasing woodland cover related to peak flow reduction, biodiversity and carbon storage, there may also occur disbenefits such as reduced food production and synchronisation of peaks from different sub-catchments within an overarching catchment. Fennell et al. (Reference Fennell, Soulsby, Wilkinson, Daalmans and Geris2023) report for a Scottish catchment of 1 km2 that tree planting reduced low flows and recharged groundwater, whereas ‘Runoff Attenuation Features’ – being soft engineered measures designed to store and attenuate rapid runoff by controlling the discharge rate, that is, ‘leakinesss’ – had a positive but smaller effect on these two processes. Both types of NbS did attenuate high and medium flows but design and influence of antecedent wetness impacted the effect. These results were based on detailed physical-based hydrological modelling informed by empirical data, including data collected through extreme conditions.
Stimulating infiltration to the groundwater can be done by both stimulating natural water retention areas, or via MAR techniques and can be implemented with both technical and more natural methods. Natural water retention areas and restoration of wetlands are often classified as aids to restore the overall hydrological functioning of the landscape and provide clear benefits to biodiversity as wetlands are among the most diverse habitats available. The more technical MAR techniques make use of the presence of ponds and depressions in the landscape to aid groundwater infiltration by installing dedicated systems to enhance this infiltration. Alam et al. (Reference Alam, Pavelic, Sharma and Sikka2020) evaluated the effectiveness of one of such MAR-techniques in a rural Indian village where the process is known as Underground Transfer of Floods for Irrigation (UTFI). They found that the small-scale UFTI influenced the groundwater levels to some extend positively, but that overarching geology, topography, scale, water quality and maintenance need to be considered in the successful use of these techniques.
Way forward
Flood and drought-related risks are increasing globally, and biodiversity is in strong decline due to climate change and demographic developments. These developments may lead to poverty, food shortages, lack of access to clean and sufficient drinking water and general decline in many ecosystem services. NbS that improve the sponge functioning of landscapes to mitigate flood and drought-related risks have a potential to protect and improve the livelihoods of communities and at the same time add to improving biodiversity.
We argue that four components are needed to facilitate planning, design, implementation, and monitoring of NbS that improve sponge functioning for floods and droughts:
-
1. Better understanding of how NbS affect hydrological processes of both extremes along the full range of potential conditions (normal, dry, extremely dry, wet and extremely wet)
-
2. Work at landscape scale
-
3. Improve integrated modelling and upscaling techniques
-
4. Use a consistent and socially relevant set of indicators to evaluate the NbS and communicate with stakeholders
Improve process understanding
There are many processes related to the functioning of NbS in relation to the propagation of floods and droughts on a landscape scale that are not well described or need additional attention during the planning, design and implementation phase of an NbS. For example, rainfall interception (and evaporation) for different types of vegetation and canopy structures (Muzylo et al., Reference Muzylo, Llorens, Valente, Keizer, Domingo and Gash2009), seasonality and pre-event effects (Fennell et al., Reference Fennell, Soulsby, Wilkinson, Daalmans and Geris2023), the impact of climatic zone and local conditions (Burek et al., Reference Burek, Mubareka, Rojas, de Roo, Bianchi, Baranzelli, Lavalle and Vandecasteele2012), the role of soil carbon content (Fennell et al., Reference Fennell, Soulsby, Wilkinson, Daalmans and Geris2022), and linkage between the unsaturated zone and the deeper ground water (Szabó et al., Reference Szabó, Szijártó, Tóth and Mádl-Szőnyi2023) all play a role in defining the overall functioning of a NbS (Kumar et al., Reference Kumar, Debele, Sahani, Rawat, Marti-Cardona, Alfieri, Basu, Basu, Bowyer, Charizopoulos, Gallotti, Jaakko, Leo, Loupis, Menenti, Mickovski, Mun, Gonzalez-Ollauri, Pfeiffer, Pilla, Pröll, Rutzinger, Santo, Sannigrahi, Spyrou, Tuomenvirta and Zieher2021a). In addition, the linkage with the impact of selected measures for floods and droughts on changes in water quality and biodiversity must be improved using easy-to-translate hydrological boundary condition indicators (e.g., minimum flow velocities for rheophilic fish communities and desired ground-water levels in summer seasons for groundwater dependent on vegetation communities).
Such process understanding can be obtained via long-term monitoring, experiments in the lab or in the field, and via modelling to facilitate understanding of the development of the NBS over time in wet, normal or dry years. For monitoring of NBS ideally a good Before-After-Control-Impact set-up is implemented, to allow for good comparison with a reference situation where the NBS is not implemented. Where this set-up is difficult to achieve a Before-After set-up will show how parameters change due to the implemented NBS. Both in-situ field monitoring and Earth Observation (EO) methods can be applied for monitoring, depending on the scale of the NBS: for small-scale NBS satellite-based EO might not be able to provide the required resolution and for soil- and groundwater parameters field monitoring might be used in unison with EO to generate the overarching process understanding of the hydrological mechanisms in the area (Kumar et al., Reference Kumar, Debele, Sahani, Rawat, Marti-Cardona, Alfieri, Basu, Sarkar Basu, Bowyer, Charizopoulos, Jaakko, Loupis, Menenti, Mickovski, Pfeiffer, Pilla, Pröll, Pulvirenti, Rutzinger, Sannigrahi, Spyrou, Tuomenvirta, Vojinovic and Zieher2021b). Experimental set-ups in the lab may help in providing an understanding of the response of NBS under extreme conditions that may not be expected during normal field-conditions. For example, the woods-versus-waves experiment provided insight in the wave-dampening capacity of willow forest under extreme conditions, to validate numerical assessment methods using live trees that are used in flood risk assessments (Van Wesenbeeck et al., Reference Van Wesenbeeck, Wolters, Antolínez, Kalloe, Hofland, de Boer, Çete and Bouma2022). This way scaling effects that may occur in indoor labs were avoided, enhancing the overall trustworthiness of the NBS.
Work at landscape scale
Planning, designing, implementing and upscaling NbS to build resilience to floods and droughts requires a proper approach that needs to pay special attention to the landscape scale, climatic zones and the type of hydrometeorological event (Duel et al., Reference Duel, Wolters, Timboe, Ter Maat and Matthews2022). The landscape scale addresses the way relevant natural and human processes are measured according to spatial characteristics and may include both the rural and urban domain. Ecosystem health and integrity depend on landscape-level processes (i.e., environmental processes that are influenced by anthropogenic activities and climate change) (Reed et al., Reference Reed, Deakin and Sunderland2015), which in turn will determine the effectiveness of the NbS intervention. Therefore, the size and extension of NbS or combinations of NbS into an overarching strategy affects their ability to deliver expected outcomes. For example, the implementation of upstream water retention areas or restoration of wetlands may affect the direct surrounding landscape and a shorter section of river catchment downstream of this intervention, while the measure does not contribute significantly to changes in the overarching discharge response on the full catchment scale of very large rivers such as the Rhine river (Waterloo et al., Reference Waterloo, Gevaert, Kersbergen, Hegnauer, Becker and de Roover2019). In some cases, the NbS implementation location and the location of the expected effects are not the same: measures taken in upstream areas will benefit predominantly stakeholders in downstream areas. Balancing these interests requires cooperation between stakeholders in the different areas (Seher and Löschner, Reference Seher and Löschner2018; Collentine and Futter, Reference Collentine and Futter2018).
Additionally, different types of solutions (both NbS and grey-engineered solutions) may need to be combined at a spatial scale to mitigate the risks of floods and droughts. Therefore the design and implementation of NbS in both a flood and drought context require the application of a landscape scale and assessments need to be carried out taking responses throughout the year into account. For example, a landscape approach can support the combination of options of water storage during the wet season (such as wetlands restoration or natural aquifer recharge) with measures to reduce water demand during dry seasons (such as landscape restoration, agriculture conservation or mulching). In this, the linkage between groundwater and surface water processes is to be well-understood (Fennell et al., Reference Fennell, Soulsby, Wilkinson, Daalmans and Geris2023; Szabó et al., Reference Szabó, Szijártó, Tóth and Mádl-Szőnyi2023). Ecosystems such as wetlands and floodplains can help reduce peak flows and increase baseflows and groundwater levels, thereby reducing risks of both floods and droughts. Yet understanding the geological features and soil characteristics can be of utmost importance to establish the infiltration capacity of, for example, retention ponds and the ease with which they drain after a rain event. If the ponds are located on impermeable soil their capacity to drain and be ready for a next event may be limited (EA, 2022).
Improve integrated modelling and upscaling techniques
Models are useful and often essential tools to facilitate decision-making and communication between stakeholders for design and evaluation of the performance of NbS at larger landscape scales. Conceptual models such as the Framework of Analysis (Van Beek et al., Reference Van Beek, Nolte, Ter Maat, Faneca-Sanchez, Asselman and Gehrels2022) can provide guidance to inclusive decision-making processes in integrated water management. As part of this overarching process numerical biophysical and socio-economic models help in the scientific underpinning of describing and quantifying the performance of NbS under different settings and scenario’s (e.g., Crespo et al., Reference Crespo, Albiac, Dinar, Esteban and Kahil2022; Fennell et al., Reference Fennell, Soulsby, Wilkinson, Daalmans and Geris2022). Over the last decade, significant improvements have been made to allow for inclusion of ecohydraulic processes in many of the standard hydro-morphodynamic software packages with a focus on processes related to instream flow-vegetation-sediment interaction (e.g., Van Oorschot et al., Reference Van Oorschot, Kleinhans, Geerling and Middelkoop2016; Willemsen et al., Reference Willemsen, Smits, Borsje, Herman, Dijkstra, Bouma and Hulscher2022). Yet, further numerical model improvements are still strongly needed to correctly quantify NbS in catchments as a step to overcome the current barriers blocking upscaled implementation (Ruangpan et al., Reference Ruangpan, Vojinovic, Di Sabatino, Leo, Capobianco, Oen, McClain and Lopez-Gunn2020).
Including all relevant processes in numerical models
Quantification of NbS related to water retention in catchments is hampered by challenges in linking the groundwater and surface water domains, due to the often-large differences in time scales and time steps used in these models and spatial scales these models run on (Banerjee and Ganguly (Reference Banerjee and Ganguly2023). However, for a full understanding of the effect of NbS on the whole hydrological regime, on both droughts and floods of different severity and on biodiversity these links between domains are crucial. For example, infiltration to the deeper groundwater of stagnant water in retention areas or on agricultural plots during rain events is not necessarily well represented in rainfall-runoff modelling and depends strongly on the level of saturation of the soil (Bronstert et al., Reference Bronstert, Niehoff and Schiffler2023). Representing such processes in hydrological models is challenging and model sensitivity related to parameter settings can be critical in producing reliable outputs of the model (Brauman et al., Reference Brauman, Bremer, Hamel, Ochoa-Tocachi, Roman-Dañobeytia, Bonnesoeur, Arapa and Gammie2021). Additionally, the models that are currently used for quantifying NbS in the hydrological domain do not link well with (ground-)water quality and biodiversity and do not assess all potential effects. Next to intended effects on the hydrological behaviour of catchments or landscapes and biodiversity, the NbS also may have unintended side effects such as increased risks of vector-borne diseases (Gingrich et al., Reference Gingrich, Anderson, Williams, O’Conner and Harking2006) or augmented ground water flooding in vulnerable catchments (Hughes et al., Reference Hughes, Vounaki, Peach, Ireson, Jackson, Butler, Bloomfield, Finch and Wheater2011) and these should therefore also be considered.
Upscaling in time and space
Defining appropriate strategies for improving sponge function of landscapes depends on the given local geology, topography, land use types, soil types and climatic zone (Raška et al., Reference Raška, Bezak, Ferreira, Kalantari, Banasik, Bertola, Bourke, Cerdà, Davids, Madruga de Brito, Evans, Finger, Halbac-Cotoara-Zamfir, Housh, Hysa, Jakubínský, Kapović Solomun, Kaufmann, Keesstra, Keles, Kohnová, Pezzagno, Potočki, Rufat, Seifollahi-Aghmiuni, Schindelegger, Šraj, Stankunavicius, Stolte, Stričević, Szolgay, Zupanc, Slavíková and Hartmann2022). The modelling must consider the right scale or be done in such a way that upscaling is possible. What is considered the right scale strongly differs per user and per decision: Information for land users is most beneficial at plot scale, while for water managers this information needs to be upscaled to (sub-) basin scale to assess effectiveness for a larger area. Ground water and hydrological models may be used for this purpose but need monitoring data for correct parameterisation of processes and validation of outcomes. Such monitoring data is scarce at the scale of single implemented measures (Zabret and Šraj, Reference Zabret and Šraj2015) let alone collecting monitoring data to validate processes on the scale of an entire catchment. However, for watershed management and planning, it is essential to understand how individual locally implemented NbS interact at the wider landscape scale (Spray et al., Reference Spray, Black, Bradley, Bromley, Caithness, Dodd, Hunt, MacDonald, Martinez Romero, McDermott, Moir, Quinn, Reid and Robertson2022). Specifically, how can NbS for improved sponge functioning jointly contribute to the overall reduction and delay in flood peaks and volumes during wet seasons as well as the improvement of base flows and ground water levels during dry seasons.
Kumar et al. (Reference Kumar, Debele, Sahani, Rawat, Marti-Cardona, Alfieri, Basu, Basu, Bowyer, Charizopoulos, Gallotti, Jaakko, Leo, Loupis, Menenti, Mickovski, Mun, Gonzalez-Ollauri, Pfeiffer, Pilla, Pröll, Rutzinger, Santo, Sannigrahi, Spyrou, Tuomenvirta and Zieher2021a) evaluated available models to quantify the effects of NbS on floods, droughts, heat waves, landslides and storm surges/coastal erosion and concluded that many of these models are still in the development stage when it comes to assessing their response for different scenarios of change. For this, numerical modelling of NbS needs to include a good process definition of the interaction between vegetation, hydrodynamics and morphodynamics over time, using the appropriate time steps to allow for feedback between these parameters
The next-generation approach to modelling landscape-scale water management strategies should consist of methods that combine floods and droughts and biodiversity into one single assessment strategy rather than treating these as separate goals to be achieved without acknowledging the interaction and dependencies between them. Many modelling studies still lack integration of all these relevant processes at the right scale which hampers not only good quantification of individual measures but also overall assessments at landscape scale. In upscaling the information from individual measures to landscape scale the size of a model’s spatial resolution automatically becomes coarser. With grid sizes greater than 0.5 km frequently being used for large basin modelling, small-scale processes such as the role of buffer strips, small preferential pathways of surface runoff through forests and wetlands, and different ploughing strategies cannot easily be represented.
Therefore, more attention to the correct upscaling of technical fine-scale processes to coarser scale process parameterisation in catchment models to increase the reliability of assessments at landscape scale is needed. In addition, new methods for linking groundwater models used for droughts (normally with timesteps of 1 day for long periods) with hydrological models for flood assessments (often with intra-hourly timesteps and event based) are to be developed and made commercially available to provide decision-makers with a good understanding of seasonal, annual and multi-year functioning of the individual measures within larger strategies. Detailed models for individual measures are often too complex, or too computationally heavy to upscale the results to larger (sub-)basins. Successful upscaling starts with good landscape data such as land-use or soil data and methods exist to incorporate fine-scale processes in these larger-scale landscape analyses, for example, using Pseudo Transfer Functions (Imhoff et al., Reference Imhoff, van Verseveld, van Osnabrugge and Weerts2020) or sophisticated upscaling rules for hydrographic data (Eilander et al., Reference Eilander, Van Verseveld, Yamazaki, Weerts, Winsemius and Ward2021).
Use a consistent set of indicators to evaluate and communicate
We suggest a straightforward framework to co-design and co-evaluate NbS for floods AND droughts AND biodiversity in a conscious manner together with all relevant stakeholder in the area of interest. In this framework NbS are not only evaluated for their primary functioning now and in the future, but also evaluated for their secondary co-benefits and trade-offs taking into account the enabling environment affecting implementation and necessary stakeholder engagement in all steps of the process (Figure 3). Evaluations can be made for individual NbS but should also be evaluated on a wider landscape scale for which an overarching strategy consisting of combinations of various individual measures (both NbS and grey solutions where needed). This framework is in line with many related guidelines available from a wide range of organisations promoting the evaluation and implementation of NbS as part of overarching water management strategies at landscape scale (EC, 2021a; EC, Reference Dumitru and Wendling2021b; Green-Grey Community of Practice, 2020, Castellari et al., Reference Castellari, Zandersen, Davis, Veerkamp, Förster, Marttunen, Mysiak, Vandewalle, Medri and Picatoste2021; Somarakis et al., Reference Somarakis, Stagakis and Chrysoulakis2019). It emphasises the need to acknowledge the primary functioning (flood, droughts, biodiversity – expressed through performance indicators) both now and in the future and also assesses various logical co-benefits and trade-offs. In its handbook for practitioners for evaluation of NbS, the EC (2021a) emphasises the need to evaluate on multiple goals for NbS and provides guidance for relevant indicators of their performance across this range of goals. This implies that even evaluating NbS ‘only’ for floods, droughts and biodiversity is not enough: evaluations should also strive to include economic and social aspects.
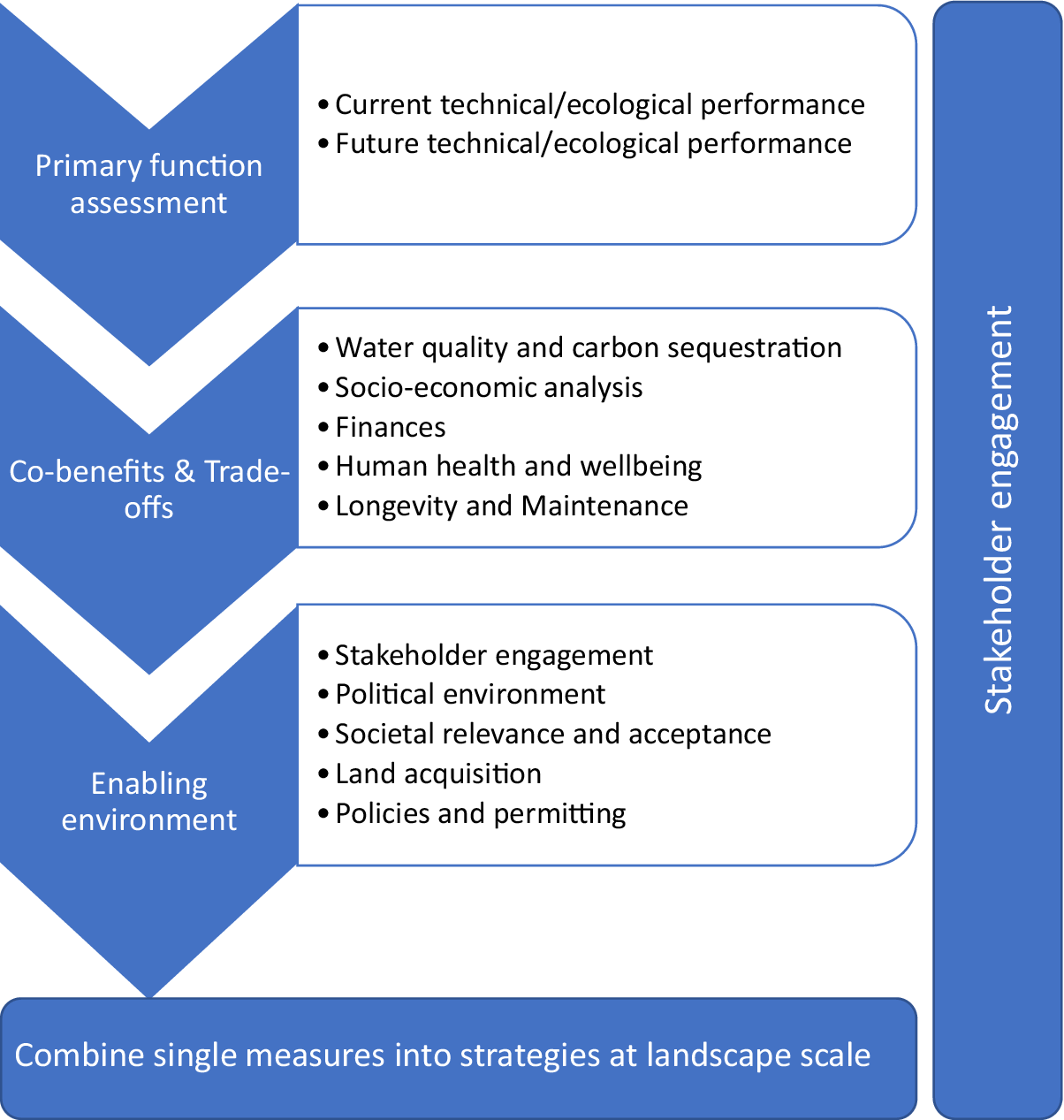
Figure 3. Suggested approach to evaluating NbS for their primary functioning and secondary co-benefits and trade-offs taking into account the enabling environment affecting implementation and necessary stakeholder engagement in all steps of the process.
Next to evaluating the primary benefits and co-benefits, it should be acknowledged that the successful implementation of NBS also depends on a wide range of enabling factors (IUCN, 2020; OECD, 2020). We call this the ‘enabling environment’ in which social relevance and acceptance – both in the political environment and the wider stakeholder community is key (IUCN, 2020; OECD, 2020). Additional enabling factors lay in the land-ownership and related policies and permitting that might be needed to facilitate implementation (IUCN, 2020; OECD, 2020). If all these three steps have been assessed for single NbS measures, they need to be combined into overarching strategies at landscape scale, which may require additional upscaling through modelling. Note that in many landscape-scale strategies combinations of green, hybrid and grey solutions may go hand in hand to bring out the best. For instance, the Dutch Room for the Rivers program is a good example where multiple individual solutions together provide the desired flood risk reduction effect and increased natural values in the floodplains of the rivers (Klijn et al., Reference Klijn, Asselman and Wagenaar2018).
The use of relevant indicators to communicate assessment results to stakeholders may consist of both technical indicators and demand-indicators (Righetti et al., Reference Righetti, Masiero, Bottaro and Pettenella2022). EC (Reference Dumitru and Wendling2021b) provides a full list of relevant indicators and methods to quantify these. Technical indicators are, for example, peak flow reduction, alterations of baseflow, ground water levels and seepage fluxes, storage capacity, or changes in peak timing and synchronicity with other (sub-)catchments, but also relate to aspects of co-benefits and trade-offs such as costs, water quality impact, etc.). Demand indicators translate technical indicators to meaningful indicators for stakeholders (such as a change in agricultural productivity, ecosystem services). Table 4 gives an example overview of suggested indicators for NbS suitable for landscapes with lowland streams in temperate climate (not extensive, based on experiences in Dutch NbS studies (Reeze et al., Reference Reeze, Schep, Slob, Querner and Van der Kooij2021). Such a list of indicators can be further amended based on the local situation, preferably in dialogue with relevant stakeholders to include aspects specifically of interest to those affected by/influencing the current and future water and soil management in the area.
Table 4. Overview of suggested indicators for NbS suitable for landscapes with lowland streams in temperate climate (not extensive)

Concluding remarks
Flood and drought-related risks are increasing worldwide due to climate change and socio-economic and demographic developments, leading to societal disruption, poverty, food shortages and lack of access to clean and sufficient drinking water. NbS that aim to improve the sponge functioning of landscapes and thereby mitigate flood and drought-related risks can be a potential cost-effective method to protect and improve the livelihoods of local communities and at the same time enhance biodiversity.
In this paper, we demonstrated that the quantified evaluation of NbS is still fragmented and not standard practice in many projects. We found that especially the implementation of large-scale NbS for mitigating droughts is still limited and that most cases reported in the studied databases lack quantified, long-term scientific evidence based on their response to combined impact of floods AND droughts AND biodiversity.
As hydrological and ecosystem processes and services take place on a larger spatial scale than at the scale at which a single NbS may be implemented it is necessary to integrate these NbS into overarching sustainable management strategies of ecosystems at a landscape scale. In this, the context-specific climatic zone and type of flood and drought events and characteristics must be considered. We provided a simple framework to carry out such evaluations, acknowledging both the primary function of the NbS and the potential co-benefits and trade-offs in relation to the enabling environment needed for implementation. We suggested the use of specific technical and demand indicators to quantify this functioning of these NbS and call for further development of modelling tools that help provide insights at the landscape scale. Various individual NbS examples have shown that the implementation of NbS options can be effective in mitigating flood and drought-related risks and at the same time do contribute positively to biodiversity values in the area. When assessing the current and future impact of NbS for flood and drought risk mitigation, large-scale technical analysis tools using global data are already available to give first estimates, but for gaining relevance these datasets must be combined with validation data including field measurements, remote sensing, surveys, interviews, focus groups, and participatory mapping. Only then a more in-depth assessment in the context of local project-level technical analysis can be fulfilled and serve as successful examples to inspire others in selecting NbS within overarching sustainable water management strategies.
Open peer review
To view the open peer review materials for this article, please visit http://doi.org/10.1017/wat.2023.12.
Data availability statement
The data presented in this publication (Table 2) is available as xls from the corresponding author. No other new data was generated for this study.
Acknowledgements
The landscape figures used in the graphical abstract are reproduced with permission and derived from the STOWA discussion pictures that are used to communicate the needs to develop from heavily modified to more naturally managed catchments and stream landscapes. Source: https://www.stowa.nl/publicaties/praatplaat-klimaatbestendig-beekdallandschap. STOWA is the Foundation for Applied Water Research in the Netherlands.
Author contribution
All authors contributed substantially to conceptualisation and drafting the manuscript. Material preparation, data collection and the design of the figures was performed by EP, MM and RP. The first draft of the manuscript was written by EP. All authors read and approved the final manuscript
Financial support
This paper was funded by the Deltares IN-SITO strategic research program ‘Sustainability’.
Competing interest
The authors do not have any conflicts of interest.
Comments
No accompanying comment.