Introduction
Traumatic brain injury (TBI) is a major cause of mortality and morbidity worldwide with the long-term outcome being determined by the severity of the underlying intracranial injury and the ensuing secondary injury.Reference Ghajar 1 The cornerstone of the critical care management of patients with TBI is to prevent secondary ischemic brain injury by optimizing cerebral oxygen delivery. To achieve this, a critical tenant is to ensure adequate cerebral blood flow (CBF) by maintaining appropriate cerebral perfusion pressure (CPP).Reference Czosnyka and Pickard 2
Over the years, there have been numerous, and often conflicting, proposed CPP thresholds.Reference Robertson 3 The Brain Trauma Foundation Guidelines recommend to target a CPP between 60 and 70 mmHg (level IIB).Reference Carney, Totten and OʼReilly 4 In addition, they recommend “avoiding aggressive attempts to maintain CPP>70 mmHg” (level III) due to the risk of acute respiratory distress syndrome (ARDS).Reference Carney, Totten and OʼReilly 4 This recommendation is driven from the increased risk of ARDS seen in a randomized study which compared a CBF strategy (CPP>70 mmHg) with intracranial pressure (ICP) strategy (CPP>50 mmHg).Reference Robertson, Valadka and Hannay 5 Although there was no difference in neurologic outcomes, 15 out of 100 (15%) patients in the CBF strategy developed ARDS compared with 3 out of 89 (3.3%) in the ICP strategy. This association between CPP and ARDS has not been observed in other studies of patients with TBI.Reference Rincon, Ghosh and Dey 6 , Reference Hendrickson, Howard and Kornblith 7
Recently, there has been increased interest in using autoregulation monitoring which may be useful in determining individualized CPP targets in patients with TBI.Reference Czosnyka, Brady, Reinhard, Smielewski and Steiner 8 , Reference Le Roux, Menon and Citerio 9 Using this approach, patients whose actual CPP was within 5 mmHg of their optimal CPP had improved neurologic outcomes.Reference Aries, Czosnyka and Budohoski 10 Interestingly, the actual mean CPP for the study period was 78 mmHg (SD 8), higher than the threshold as recommended by the Brain Trauma Foundation.Reference Carney, Totten and OʼReilly 4
Thus, there appears to be conflicting evidence concerning CPP thresholds in patients with TBI. Whereas targeting individualized CPP thresholds greater than 70 mmHg may be beneficial in some patients, this must be weighed against the risk of developing ARDS. However, the risk of ARDS has not previously been well characterized.
As such, our objective was to conduct a single-center historical cohort study to characterize the incidence, etiology and outcomes of ARDS in patients admitted with severe TBI who underwent multimodal neuromonitoring. We additionally wanted to examine the association between CPP, dose of vasopressors, ventilation parameters (positive end expiratory pressure [PEEP] and driving pressure [ΔP]) and cumulative fluid balance on the risk of developing ARDS.
Material and Methods
We performed an historical cohort study. This study is the same cohort of patients as reported in our prior published quasi-experimental uncontrolled before-and-after study.Reference Sekhon, Gooderham and Toyota 11 We report this results in accordance with the Strengthening the Reporting of Observational Studies in Epidemiology (STROBE) statement.Reference Vandenbroucke, von Elm and Altman 12 This study was approved by both the University of British Columbia and hospital clinical research ethics boards who waived the requirement for written informed consent (H16-01100).
Study population and hospitals
Patients were included in our cohort if they were admitted to the intensive care unit (ICU) at Vancouver General Hospital with a diagnosis of severe TBI, as defined by a post-resuscitation Glasgow Coma Score (GCS)≤8 between February 2010 and May 2016, and in whom an ICP monitor was inserted. Patients were excluded if their pupils were bilaterally dilated and unresponsive to light. The ICU at Vancouver General Hospital is a closed, 34-bed mixed medical-surgical unit that operates on an approximate 1:1.2 nurse-to-patient ratio. Affiliated with the University of British Columbia, the ICU is staffed by fellowship trained subspecialty critical care medicine physicians and specialty residents in attendance.
Data collection
Patients were identified using the Provincial ICU database. Data were abstracted from the medical record into a standardized case-report form in Microsoft Excel 2013® (Redmond, Washington, DC, USA). In addition to demographic data, the following baseline data were abstracted: post-resuscitation GCS, pupillary abnormalities (one pupil dilated, both pupils dilated), pre-hospital hypoxia (SpO2<92%) or hypotension (systolic blood pressure<90 mmHg), and Rotterdam score on the initial computerized tomography head exam.Reference Maas, Hukkelhoven, Marshall and Steyerberg 13 We collected the following variables for the first 7 days: temperature (oC), medications administered (propofol, norepinephrine, neuromuscular blockers, barbiturates, hydrocortisone, fludrocortisone and midazolam), intravenous fluids per day (ml) (crystalloids, colloids, red blood cells and mannitol), laboratory values (hemoglobin, troponin and serum sodium) and arterial blood gases (pH, partial pressure of oxygen and carbon dioxide). We also collected the following daily mean ventilation data: peak and plateau pressure, minute ventilation and PEEP. Driving pressure was calculated by subtracting the PEEP from the plateau pressure. Daily chest radiographs were read independently by two authors (ST and MS) who were unaware of the other clinical parameters. The chest radiographs were read documenting the presence or absence of bilateral infiltrates in keeping with ARDS. Neurophysiologic data (mean arterial pressure [MAP], ICP, CPP, PbO2 and pressure reactivity index [PRx]) were recorded in real-time and continuously at 10-second intervals from our bedside monitors directly onto the ICM+® brain monitoring software program (Division of Neurosurgery, Cambridge University). These variables were then averaged for each day. We also collected procedural data, including: surgical interventions (decompressive craniectomy, craniotomy, external ventricular drain, tracheostomy), medical interventions (therapeutic hypothermia, barbiturate use, days of intravenous sedation) and invasive neuromonitoring (days of monitoring, jugular venous oximetry, intra-parenchymal ICP, PbO2 and PRx). The following outcome data were obtained from the ICU database: intensive care and hospital days, days of mechanical ventilation and hospital mortality. Neurologic outcomes were assessed using the Glasgow Outcome Scale and were determined at 6 months by telephone interview. Glasgow Outcome Scale is an ordinal scale between 1 and 5, where: 1—death, 2—permanently vegetative state, 3—severe disability (requires help with activities of daily living), 4—moderate disability and 5—good recovery.
Pressure Reactivity Index and Optimal Cerebral Perfusion Pressure-Guided Management
Pressure reactivity index, is the moving correlational coefficient between MAP and ICP. Pressure reactivity index is a measure of autoregulation that can be monitored in real time using the ICM+ monitoring software program. Mean arterial pressure and ICP numerical data are streamed into a laptop from our bedside monitors (Carescape; General Electric, New York, NY, USA) via a USB-RS232 to serial adapter cable. Pressure reactivity index is generated from 30 consecutive 10-second averaged values of ICP and MAP. This methodology has been described in previous studies as a valid measure of autoregulation after severe TBI.Reference Aries, Czosnyka and Budohoski 14 , Reference Steiner, Czosnyka and Piechnik 15
By plotting the entire CPP range in 5 mmHg bins (x axis) versus the absolute PRx (y axis), U-shaped curves can be generated with the nadir reflecting the optimal CPP. Identification of the optimal CPP is conducted on a daily basis and a 10 mmHg CPP range is prescribed every 24 hours while multimodal monitoring is place. Optimal CPP-guided management based on PRx is used in our unit on the basis of previous published studies and guideline statements.Reference Le Roux, Menon and Citerio 9 , Reference Aries, Czosnyka and Budohoski 14 In the setting of complete dysfunctional autoregulation (i.e., PRx>0.3), we individualize CPP targets based on the minimum CPP required to achieve adequate brain oxygenation (PbO2>20 mmHg), while weighing the risks of excessive iatrogenic complications (fluid administration and vasopressor doses).
Definition of Acute Respiratory Distress Syndrome
We used the Berlin criteria to define ARDS.Reference Maas, Hukkelhoven, Marshall and Steyerberg 13 A patient was considered to have ARDS if they had an acute onset of bilateral opacities consistent with pulmonary edema (on either chest X-ray or CT scan) with a PaO2 to FiO2 (PF) ratio ≤300 mmHg that is not fully explainable by heart failure or volume overload. Acute respiratory distress syndrome was characterized as either mild (200 mmHg<PF≤300 mmHg), moderate (100 mmHg<PF≤200 mmHg) or severe (PF≤100 mmHg). The underlying etiology of ARDS was adjudicated by two authors (ST and MS).
Management of Traumatic Brain Injury Patients
The management of patients admitted with a TBI at our institution has previously been reported.Reference Sekhon, Gooderham and Toyota 11 Our TBI management protocol is modeled after other published protocols.Reference Patel, Menon, Tebbs, Hawker, Hutchinson and Kirkpatrick 16 Patients are managed in a tiered fashion depending on the degree of intervention required to control their ICP below 20-25 mmHg. During the time of this study (November 2014), we transitioned our neuromonitoring from solely the use of external ventricular drains with jugular venous oximetry, to the Camino® parenchymal ICP and Licox® PbO2 monitors (Integra® Life Sciences, Plainsboro, NJ, USA). Individualized CPP thresholds are set using PbO2 and autoregulation monitoring with PRx.Reference Smielewski, Aries and Lavinio 17
Statistical Analysis
All analyses were performed using Stata 10.0 (StataCorp, TX, USA). All tests of hypothesis tests were two-sided and we considered a p-value of less than 0.05 to be statistically significant. Normally distributed, non-normally distributed and categorical data were described with mean (SD), median (interquartile range [IQR]) and proportion (percent), respectively. Univariable comparisons of continuous variables were performed using independent t-tests for normally distributed data and Wilcoxon rank-sum test for non-normally distributed data. The sample size was one of convenience. A complete-case analysis was performed and missing data are presented when applicable. We sought to determine the association between ventilation parameters (ΔP and PEEP), cumulative fluid balance, CPP and use of norepinephrine on the risk of ARDS. Owing to the repeated measures nature of the data structure, we performed a generalized linear regression to model the association of these variables and the risk of ARDS.
Results
Between February 2010 and May 2016, there were 115 patients identified using the ICU database. We excluded two patients who presented with unreactive and dilated pupils, leaving 113 patients included in the final analysis. The cohort had a mean age of 39 years (SD 17) and 26 out of 113 (23%) were female. The median GCS motor score was 3 (IQR 1-4). The demographic and admission characteristics are presented in Table 1. The clinical interventions and patient outcomes are presented in Table 2. Of note, there were no missing data.
Table 1 Baseline characteristics of cohort

ARDS=acute respiratory distress syndrome; IQR=interquartile range.
Table 2 Clinical interventions and outcomes stratified by patients who developed acute respiratory distress syndomre (ARDS)

ICP=intracranial pressure; EVD=external ventricular drain; ICU=intensive care unit; IQR=interquartile range; GOS=Glasgow Outcome Scale.
Characteristics of patients developing Acute Respiratory Distress Syndrome
Overall, 16 out of 113 (14%) patients developed ARDS in the first 7 days of admission. There was a total of 46 out of 694 (6.6%) patient days where the criteria of ARDS were met. Seven out of the 16 patients met ARDS criteria on the 1st day of admission with two and three patients developing ARDS on admission days 2 and 3, respectively. Four out of 16 patients developed ARDS between days 5 and 7. Regarding the severity of ARDS, 10 out of 16 (68%) were mild and 6 out of 16 (38%) were moderate. There were no cases of severe ARDS.
The etiology of ARDS were as follows: aspiration pneumonia (8 out of 16), pulmonary contusion (4 out of 16) and sepsis (4 out of 16). The median duration of ARDS was 4 days (IQR 2-7.5). Comparing patients who developed ARDS to those who did not develop ARDS, there was no difference in mechanical ventilation-free days, sedation-free days, ICU-free days or duration of hospitalization in days (Table 2).
Relationship of Acute Respiratory Distress Syndrome to Cerebral Perfusion Pressure, ventilation parameters, cumulative fluid balance
There was no difference in CPP during the first 7 days between those patients who developed ARDS (74 mmHg [15]) compared with those patients who did not develop ARDS (73 mmHg [18]). Ventilation parameters (ΔP and PEEP), PF ratio, CPP and cumulative fluid balance stratified by day of admission and ARDS are presented in Table 3 and Figure 1. Patients who developed ARDS had a higher ΔP during the first 7 days (15 mmHg [5]) compared with those patients who did not develop ARDS (12 mmHg [4]) (p=0.016). Compliance was also lower over the first 7 days comparing those patients who had ARDS (35 ml/cmH2O [10]) to those who did not have ARDS (49 ml/cmH2O [18]) (p=0.024). There was no difference in PEEP, PaCO2, cumulative fluid balance or norepinephrine dose.

Figure 1 Depicted are differences in cerebral perfusion pressure (CPP), ventilation parameters (PEEP and ΔP), norepinephrine doses (mcg/min) and cumulative fluid balance (ml) in patients who developed acute respiratory distress syndrome versus those who did not. ΔP=driving pressure; PEEP=positive end expiratory pressure; ICU=intensive care unit; ARDS=acute respiratory distress syndrome.
Table 3 Cerebral perfusion pressure (CPP), intracranial pressure, ΔP, PEEP and cumulative fluid balance stratified by acute respiratory distress syndomre (ARDS) for the first 7 days of intensive care unit admission
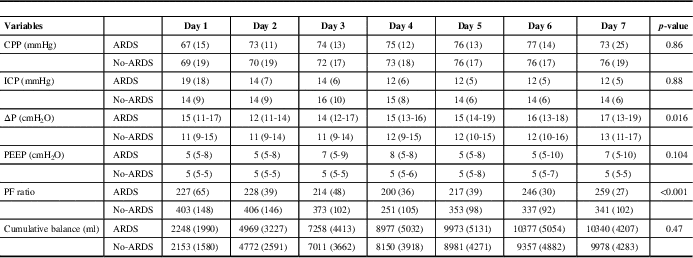
ICP=intracranial pressure; ΔP=driving/delta pressure; PEEP=positive end expiratory pressure; PF ratio=PaO2 to FiO2 ratio.
Standard deviations are recorded next to absolute mean values in parentheses.
Discussion
The goal of our historical cohort study was to characterize the incidence, characteristics and etiology of patients admitted with a severe TBI requiring multimodal monitoring, who fulfilled the Berlin criteria for ARDS. ARDS occurred in 16 out of 113 patients. The vast majority of cases of ARDS were due to aspiration pneumonia and was mild in severity. There was no difference in CPP, cumulative fluid balance or norepinephrine dose between patients who developed ARDS and those who did not. Patients who developed ARDS had higher ΔP and lower respiratory system compliance. Finally, there was no difference in the two groups in mechanical ventilation-free days, sedation-free days, ICU-free days and duration of hospitalization.
The incidence of ARDS in patients with severe TBI varies between 7.7% and 30%, with rates being similar between both the Berlin and previous American European Consensus Conference (AECC) definitions.Reference Ranieri and Rubenfeld 18 , Reference Aisiku, Yamal and Doshi 19 Robertson et al.Reference Robertson, Valadka and Hannay 5 performed a randomized trial comparing a CBF-target protocol to an ICP-targeted protocol. The major difference between these two protocols was that CPP was maintained >70 mmHg in the CBF-targeted group, compared with >50 mmHg in the ICP-targeted group. Overall, ARDS occurred in 18 out of 189 patients (9.5%): 15 out of 100 (15%) of patients in the CBF strategy compared with 3 out of 89 (3.3%) in the ICP strategy. In a subsequent analysis of these 18 patients who developed ARDS, the identified risk factors were: administration of epinephrine (odds ratio [OR] 5.7, 95% confidence interval [CI]: 1.0-34.3), administration of dopamine (OR 10.8, 95% CI: 1.4-488) and a history of drug abuse (OR 3.1, 95% CI: 0.93-11.2).Reference Contant, Valadka, Gopinath, Hannay and Robertson 20 In the study by Robertson et al., the PF ratio used to define ARDS was ≤200 mmHg. Using the same PF ratio, the incidence of ARDS in our study was much lower, only 6 of 113 (5%). In contrast to the studies by Robertson et al., we did not demonstrate an association between either CPP or vasopressor dose and the development of ARDS.
Using the Berlin criteria for ARDS, Hendrickson et al.Reference Hendrickson, Howard and Kornblith 7 observed an incidence of ARDS within the first 8 days in patients admitted with a severe TBI of 30%. Of the 62 cases of ARDS in their study, 32% were mild, 49% were moderate and 19% were severe. Following multivariable adjustment, male sex and platelet transfusion in the first 12 hours were associated with the risk of developing ARDS. Furthermore, despite no difference in mortality, those patients who developed ARDS had increased length of stay and fewer ventilator-free days. However, despite a higher incidence of ARDS in their study, there was no description of the etiology of ventilator parameters of those patients who developed ARDS.
One interesting finding in our study was the association of ΔP and the development of ARDS. Driving pressure (ΔP=tidal volume/respiratory system compliance) essentially normalizes the delivered tidal volume to the respiratory system compliance. In a recent large observational study of 3,562 patients enrolled into nine randomized trials of patients with ARDS, ΔP, rather than PEEP or tidal volume, was found to be the respiratory parameter most strongly associated with survival.Reference Amato, Meade and Slutsky 21 Guerin et al.Reference Guérin, Papazian and Reignier 22 found a 5% increase in the risk of death for each cmH2O increase in ΔP. The association of ΔP and ARDS has also been demonstrated in a recent multicenter observational study in neurocritically ill patients.Reference Tejerina, Pelosi and Muriel 23
Physiologically, the development of ARDS may pose significant concerns for patients with TBI. First, ARDS can cause profound arterial hypoxemia which is associated with concomitant brain hypoxia and secondary injury.Reference Oddo, Nduom and Frangos 24 Second, permissive hypercapnia with low tidal volume ventilation, a mainstay in the ventilation management of ARDS, may be poorly tolerated in patients with TBI.Reference Go and Singh 25 Carbon dioxide, a potent cerebral vasodilator, increases CBF and volume, which can cause dangerous elevations in ICP in patients with limited intracranial compliance.Reference Go and Singh 25 , Reference Rangel-Castilla, Lara, Gopinath, Swank, Valadka and Robertson 26 Furthermore, hypercapnia can distort cerebral autoregulation by narrowing the intact zone of autoregulation and resulting in a rightward shirt of the lower limit of intact autoregulation, rendering the injured brain susceptible to ischemia.Reference Meng and Gelb 27 Finally, mechanical ventilation, specifically application of positive expiratory pressure has been associated with increased intrathoracic pleural pressure and subsequent increased ICP.Reference Burchiel, Steege and Wyler 28 - Reference Videtta, Villarejo and Cohen 30 However, this latter relationship may not hold in the setting of poor lung compliance.Reference Caricato, Conti and Della Corte 31
The obvious conflict here is that the modifiable variables used to maintain an optimal CPP, namely vasopressors and fluids, have previously been associated with the risk of developing ARDS, which itself carries morbidity to the patient. This is reflected in the Brain Trauma Foundation Guidelines which specifically recommend “avoiding aggressive attempts to maintain CPP>70 mmHg” (level III) due to the risk of ARDS.Reference Carney, Totten and OʼReilly 4 In contrast, the international multidisciplinary consensus conference on multimodality monitoring in neurocritical care does not specify specific CPP targets. Rather, they recommend that individualized CPP targets and that autoregulation monitoring may be useful in determining optimal CPP.Reference Le Roux, Menon and Citerio 9 There is an association with improved neurologic outcomes when patients actual CPP is close to their optimal CPP.Reference Aries, Czosnyka and Budohoski 10 Although associated with better neurologic outcomes, this individualized approach to CPP management often results in higher CPP than recommended by the Brain Trauma Foundation. In fact, the mean CPP in patients who achieved good neurologic outcomes was 79 mmHg (SD 7). Sorrentino et al.Reference Sorrentino, Diedler and Kasprowicz 32 identified thresholds for values for ICP, CPP and pressure reactivity using a database of 459 patients with TBI. They found that for patients with impaired autoregulation, those with CPP<70 mmHg had significantly worse outcomes.Reference Sorrentino, Diedler and Kasprowicz 32 Thus, given the increasingly clinical evidence supporting individualized CPP, which is often higher than 70 mmHg, the risk of developing ARDS, with its associated outcomes, needs to be characterized.
There are several significant limitations to our study that require consideration when interpreting our results. The most obvious limitation is small sample size as we only had 16 cases of ARDS in our cohort using the Berlin criteria. We believe this incidence to be correct and unlike prior studies in patients with TBI, we determined the underlying etiology of ARDS. However, the small sample size greatly limits the inference of associations with the variables of interest, namely: CPP, use of vasopressors and cumulative fluid balance. Furthermore, only nine patients developed “late” ARDS whereby the development of ARDS occurred after the first 48 hours of admission which would more accurately reflect the effect of increased CPP, vasopressor dose or fluid administration pm the occurrence of ARDS. Future logical next steps include further interrogating the potential associations between vasopressor doses and increased CPP in a larger cohort. In addition, because of the observational nature of the study, we are unable to clarify the direction of association between ΔP and ARDS. In other words, it may be that clinicians used higher pressures in those patients who developed ARDS, rather than ΔP leading to ARDS. Because of the low event rate of ARDS, we were unable to perform an adjusted analysis. Thus, confounding is clearly an alternate explanation for our findings. The strengths of our study include a detailed characterization of the etiology of ARDS, and a repeated measures analysis for longitudinal data structure.
Conclusion
In conclusion, the risk of ARDS in our cohort of TBI is lower than previously reported. Patients who developed ARDS had similar outcomes to those who did not develop ARDS. Although we have few outcomes events, there was no association with the development of ARDS and CPP, fluid balance or vasopressor dose. Finally, we demonstrated an association between ΔP and the risk of developing ARDS.
Acknowledgment
The authors acknowledge the Vancouver general Hospital ICU staff for their support.
Disclosures
ST, DEG, WRH, and MSS have nothing to disclose.
Statement of Authorship
ST is the primary author and contributed the majority of the manuscript preparation and background research. DEG contributed to data analysis and manuscript preparation. WRH contributed to manuscript preparation. MSS contributed is the senior author, contributed to data collection, analysis and manuscript preparation. All authors read and approved the final manuscript.