Introduction
The inclusion of bentonite in feed by the livestock industry is a readily available method to reduce aflatoxin exposure (Harvey et al., Reference Harvey, Phillips, Ellis, Kubena, Huff and Petersen1991). Aflatoxin B1 (AFB1) is a potent hepatocarcinogen (Busby & Wogan, Reference Busby, Wogan and Searle1984) with genotoxic (Smela et al., Reference Smela, Currier, Bailey and Essigmann2001), immunotoxic (Hinton et al., Reference Hinton, Myers, Raybourne, Francke-Carroll, Sotomayor, Shaddock, Warbritton and Chou2003; Turner et al., Reference Turner, Moore, Hall, Prentice and Wild2003), and antinutritional (Pimpukdee et al., Reference Pimpukdee, Kubena, Bailey, Huebner, Afriyie-Gyawu and Phillips2004) effects. AFB1 is a group 1 carcinogen (International Agency for Research on Cancer [IARC], 2012) produced mainly by Aspergillus flavus and A. parasiticus fungi that commonly contaminate cereals and nuts (Council for Agricultural Science and Technology [CAST], 2003). Although AFB1 is the most toxic and prevalent of the aflatoxin chemical subtypes, the mixture of total aflatoxins (AFs) is also regarded as a group 1 carcinogen (IARC, 2012). Due to their deleterious effects, the FDA established total aflatoxin action levels ranging from 20 μg/kg to 300 μg/kg for different ingredients used in animal nutrition (Food and Drug Administration [FDA], 2019).
Bentonites are montmorillonite-rich clays commonly used as anti-caking agents or flow promoters in feeds. These minerals have GRAS (generally recognized as safe) status when used at levels not exceeding 2% inclusion in feed (Code of Federal Regulations, 2010) and have many potential applications in sorption of toxic compounds, drug delivery, conferring thermal and pH resistance properties to materials, among other useful human health therapeutic applications such as diarrhea control (Uddin, Reference Uddin and Zoveidavianpoor2018). Montmorillonite is regarded as the active ingredient for aflatoxin (AF) binding (Deng et al., Reference Deng, Barrientos-Velázquez, Billes and Dixon2010 Grant & Phillips, Reference Grant and Phillips1998). By adsorbing AFs from the diet, montmorillonite reduces the toxin bioavailability (Phillips, Reference Phillips1999; Phillips et al., Reference Phillips, Afriyie-Gyawu, Williams, Huebner, Ankrah, Ofori-Adjei, Jolly, Johnson, Taylor, Marroquin-Cardona, Xu, Tang and Wang2009) thus protecting animals from toxicity. Calcium bentonites (Ca-bentonites) have been investigated extensively in vitro and in vivo and have been proved to protect animals effectively from the negative effects of AF (Ledoux et al., Reference Ledoux, Rottinghaus, Bermudez and Alonso-Debolt1999; Smith et al., Reference Smith, Phillips, Ellis, Harvey, Kubena, Thompson and Newton1994; Phillips et al., Reference Phillips, Afriyie-Gyawu, Wang, Williams, Huebner, Barug, Bhatnagar, van Egmond, van der Kamp, van Osenbruggen and Visconti2006). In humans, the use of Ca-bentonites (i.e. NovaSil) was shown to be safe and effective in reducing the levels of blood and urine biomarkers of AF exposure (Afriyie-Gyawu, Ankrah et al., Reference Afriyie-Gyawu, Ankrah, Huebner, Ofosuhene, Kumi, Johnson, Tang, Xu, Jolly, Ellis, Ofori-Adjei, Williams, Wang and Phillips2008a; Wang et al., Reference Wang, Afriyie-Gyawu, Tang, Johnson, Xu, Tang, Huebner, Ankrah, Ofori-Adjei, Ellis, Jolly, Williams, Wang and Phillips2008). Similarly, oral sodium bentonite has been shown to protect domestic animals from AF exposure. For instance, bentonite in dairy cattle feed resulted in a reduction in AFM1 (an AFB1 metabolite) excretion in milk (Diaz et al., Reference Diaz, Hagler, Blackwelder, Eve, Hopkins, Anderson, Jones and Whitlow2004). Similarly, New Zealand breed rabbits were protected from aflatoxicosis when dosed at 5 g bentonite/kg of feed (Hassan et al., Reference Hassan, Abu Hafsa, Elghandour, Kanth-Reddy, Cedillo-Monroy and Salem2019). Due to the evident benefits observed from inclusion of these types of minerals in feeds, the European Union and the US state of Texas allow the use of some clays (e.g. bentonites) and other additives as AF adsorbents in feeds (European Food Safety Authority [EFSA], 2010; Office of the Texas State Chemist [OTSC], 2011).
Despite its beneficial effects, the potential interactions of sodium bentonite with anticoccidials, antibiotics (Gray et al., Reference Gray, Ward, Southern and Ingram1998; Shryock et al., Reference Shryock, Klink, Readnour and Tonkinson1994), and vitamin A (Briggs & Spivey-Fox, Reference Briggs and Spivey-Fox1956; Laughland & Phillips, Reference Laughland and Phillips1956) require further studies on the safety of this mineral. Additionally, due to their inherent natural origin and differences in composition, bentonites may contain heavy metals, dioxins, and furans as contaminants. For these reasons, in vivo safety evaluation of any bentonite for use as a toxin binding agent is essential before its inclusion in the diet. The objectives of the present study were to determine the mineral characteristics, sorption properties for AF in vitro, and safety of a selected sodium bentonite (Na-bentonite) in a 3-month rodent study using Sprague-Dawley rats.
Materials and Methods
Na-bentonite was provided by American Colloid’s Wyoming facility, Reserve 2010-5113 (AMCOL International Corporation, Hoffman Estates, Illinois, USA). Ultrapure water (UP-H2O) was obtained by processing distilled water through a PURELAB Ultra filtration system (ELGA Woodridge, Illinois, USA). AFB1 standard (CAS 1162-65-8) was purchased from Sigma Aldrich (St. Louis, Missouri, USA). All other reagents and solvents were of analytical grade.
Measurement of Dioxins, Furans, and Heavy Metals in Na-bentonite
As bentonites are naturally occurring minerals, they may contain undesirable toxic compounds that may limit their use as dietary interventions in animals or humans. Hence, data for the 17 most important chlorinated dibenzo-p-dioxins/furans (CDDs/CDFs) found in Na-bentonite were performed by Analytical Perspectives (Wilmington, North Carolina, USA). Procedures followed the US Environmental Protection Agency (USEPA) method 8290 for sample preparation, clean-up, and analysis with high resolution gas chromatography/high resolution mass spectrometry (HRGC/HRMS) (Environmental Protection Agency [EPA], 2007c). Measurements of arsenic (As), cadmium (Cd), lead (Pb), and mercury (Hg) were also performed by the same laboratory. Samples were digested and prepared according to method 3051A using a PerkinElmer Multiwave 3000 Anton Paar Microwave Reaction System (Graz, Styria, Austria) (EPA, 2007a). For Hg analyses, a PerkinElmer FIMS-100 CVAA analyzer was used following EPA method 7470A (EPA, 1994). Other metals were analyzed with a PerkinElmer ELAN 6100 ICP-MS using EPA method 6020A (EPA, 2007b). Elemental composition of Na-bentonite was obtained using X-ray fluorescence (XRF) with a Phillips PW2400 XRF spectrometer. Data were run and provided by AMCOL.
Mineralogical Analyses
Mineralogical analyses were run in the clay mineralogy laboratory at Texas A&M University (TAMU). After size fractionation following the steps described by Soukup et al. (Reference Soukup, Buck, Harris, Ulery and Drees2008), the clay, silt, and sand fractions were analyzed with X-ray diffraction (XRD), Fourier-transform infrared (FTIR) spectroscopy, scanning electron microscopy (SEM), and transmission electron microscopy (TEM). Most mineralogical analyses were performed following standard procedures previously reported (Soukup et al., Reference Soukup, Buck, Harris, Ulery and Drees2008) with minor modifications. The moisture content was determined by subtracting the oven-dry weight (110°C) from the air-dry weight of 5 g of Na-bentonite. For electrical conductivity (EC) measurement, 10 g of sample was weighed into 250 mL plastic bottles; the ratio of deionized water:solid used was 25:1. The sample was shaken for 30 min on a rotary shaker at room temperature and then centrifuged at 1073✕g for 10 min. After centrifugation, the supernatant was used to measure the EC and pH sequentially. To evaluate the presence of sulfates, 2 mL of the supernatant was collected in a glass tube followed by the addition of an equal volume of acetone (sulfate precipitation).
Cation exchange capacity (CEC) and extractable base determination in NH4OAc were performed by the Soil Characterization Laboratory from the Soil and Crop Sciences Department at TAMU, using a mechanically controlled variable-rate leaching device as reported by Holmgren et al. (Reference Holmgren, Juve and Geschwender1977). Soluble bases using DI water were determined by the Soil, Water and Forage Testing Laboratory at TAMU, using a saturated paste extract method based on Rhoades and Clark (Reference Rhoades and Clark1978). 100 g of sample were used for this analysis.
X-ray diffraction (XRD) patterns were recorded using a Bruker D8 ADVANCE X-ray diffractometer (Billerica, Massachusetts, USA). Sand and silt fractions were ground in a mortar, passed through a 140 mesh (105 μm) sieve, and mounted as powder to collect the XRD patterns. Clay fractions were saturated with magnesium (Mg) and potassium (K), and then air-dried on glass discs to obtain oriented films for XRD analysis. Mineral identification was determined using EVA computer software (Bruker, Madison, Wisconsin, USA). Infrared patterns were recorded in a Spectrum 100 FTIR (PerkinElmer, Inc. Waltham, Massachusetts, USA) using the diffuse reflectance infrared Fourier-transform (DRIFT) method and the attenuated total reflectance (ATR) method with a scanning range of 450–7800 cm–1 and a resolution of 2 cm–1. For the DRIFT method, 0.005 g of sample was placed in a holder, using KBr as background material. For the ATR method, 1 mg of the clay fraction was mixed with 300 mg of KBr under a lamp to maintain dryness. Clay sample and KBr were mixed in a steel capsule for 30 s and then placed in a holder for reading. Powder samples were used directly in the ATR analysis with a Universal ATR accessory (PerkinElmer, Inc., Waltham, Massachusetts, USA). Silt samples were examined using a JEOL 6400 scanning electron microscope with energy dispersive X-ray (EDS) recording capability (Akishima, Tokyo, Japan). For TEM analysis, a clay sample was mounted on a holey carbon grid, and images were recorded using a JEOL JEM-2010 microscope with EDS capability (Akishima, Tokyo, Japan).
AFB1 Sorption Analyses
Aflatoxin adsorption isotherms were performed based on methods reported by Grant & Phillips (Reference Grant and Phillips1998), and previously described in detail by Marroquin-Cardona et al. (Reference Marroquin-Cardona, Deng, Taylor, Hallmark, Johnson and Phillips2009). Briefly, a Na-bentonite suspension was prepared in an Erlenmeyer flask by adding 100 mg of clay and 50 mL of water to give a concentration of 2 mg/mL. A toxin parent solution (8 μg/mL) was prepared by dissolving toxin AFB1 crystals (Sigma-Aldrich, Saint Louis, Missouri, USA) in acetonitrile. Then, 11 dilutions of AFB1 ranging from 0.4 to 8 μg/mL were prepared by adding appropriate amounts of parent AFB1 solution and UP-H2O in glass tubes to complete a 5 mL volume; each dilution was done in triplicate. Na-bentonite suspension (50 μL) was added to test tubes (i.e. 0.1 mg clay in each test tube) and tubes were shaken for 2 h at 1000 rpm on an IKA-Vibrax orbital shaker (Wilmington, North Carolina, USA) at 25°C. Isotherms were conducted at pH 2 and 6.5 by adjusting UP-H2O pH. Upon completing the 2 h shaking, tubes were centrifuged at 939✕g for 20 min to collect supernatant for AFB1 measurement at 364 nm wavelength on a Shimadzu UV-Vis spectrophotometer (Columbia, Maryland, USA). Computer-generated equilibrium isotherms were extrapolated from Na-bentonite adsorption data and fitted to the Langmuir model (based on r2 value). The parameters Q max (mol AFB1 kg–1 bentonite) and Kd were estimated to determine sorption capacity and affinity onto Na-bentonite.
Rodent Study Experimental Design
Authorization for the study was obtained by Texas A&M University, according to animal use protocol (AUP) 2008-39. Sprague Dawley®™ (SD) rats were obtained from Harlan (Houston, Texas, USA). Animals in each group came from different litters to assure independence of samples. Four-week-old female and male SD rats were maintained on rodent feed meal (8604) from Teklad Harlan (Madison, Wisconsin, USA) and water ad libitum. After a brief acclimation period (5 days), the rats (1 rat per cage) were allocated to each group, including one control and two Na-bentonite treatment groups. Each group consisted of 10 male and 10 female rats, with average weights at the beginning of the study of 107–133 g and 79–105 g, respectively. The control group received basal rodent diet throughout the study while the treatment groups received formulations containing basal rodent diet with low (0.25%) and high (2%) inclusion of Na-bentonite. Dietary clay concentrations were based on the minimum (0.25% w/w) and maximum (2%) doses previously tested in similar safety studies (Afriyie-Gyawu et al., Reference Afriyie-Gyawu, Mackie, Dash, Wiles, Taylor, Huebner, Tang, Guan, Wang and Phillips2005).
Animals were housed in a climate-controlled environment (temperature 22–25°C) with artificial illumination (12 h dark/12 h light). General appearance, behavior, and signs of morbidity and mortality were inspected daily. Body weights were measured initially and every three days throughout the study (30 time points). Feed consumption was also recorded every three days (29 time points). Before termination, blood was drawn via cardiac puncture under isoflurane anesthesia. Following euthanasia, organs and tissues of interest were removed and evaluated for gross anatomical abnormalities. Wet weights of liver, kidneys, heart, lungs, brain, spleen, tibia, and uterus plus ovaries were recorded.
Hematological and Serum Analysis
Hematology and serum biochemical parameters were analyzed by the Clinical Pathology Lab, Texas Veterinary Medical Diagnostics Lab (TVMDL) (College Station, Texas, USA). Hematological analysis of whole blood samples was conducted using an Abbott CELL-DYN 3700 Hematology Analyzer (Abbott Laboratories, Abbott Park, Illinois, USA) and included hemoglobin (Hb) concentration, mean corpuscular hemoglobin (MCH), mean corpuscular hemoglobin concentration (MCHC), mean corpuscular volume (MCV), percent corpuscular volume (PCV), platelets, and red blood cell (RBC) and white blood cell (WBC) counts. Serum biochemical parameters were assessed using an automated analyzer Modular P (Roche Diagnostics, Indianapolis, Indiana, USA) and included albumin/globulin ratio (A/G), albumin (ALB), alkaline phosphatase (ALP), alanine aminotransferase (ALT), amylase (AMYL), aspartate aminotransferase (AST), blood urea nitrogen (BUN), calcium (Ca), cholesterol (CHOL), creatine kinase (CK), chloride (Cl), creatinine (CRT), gamma glutamyl-transferase (GGT), globulins (GLOB), glucose (GLUC), potassium (K), sodium (Na), Na/K ratio, phosphorous (P), total bilirubin (T-BIL), and total serum protein (TSP). Serum micronutrients iron (Fe) and zinc (Zn) were measured with a Hitachi 911 chemistry analyzer (Roche Laboratories, Indianapolis, Indiana, USA) and a PerkinElmer Analyst 100 (Shelton, Connecticut, USA), respectively. Vitamin A and E were measured by HPLC according to previously reported methods by Weinmann et al. (Reference Weinmann, Oliveira, Jorge and Martins1999) and Rupérez et al. (Reference Rupérez, Mach and Barbas2004).
Statistical Analysis
To investigate the weight gained among the control group and the low dose (0.25 %) and high dose (2%) Na-bentonite groups, a linear mixed model was constructed with the fixed effect treatment, sex, and treatment-sex interaction. Time was included as a continuous variable and tested for interactions with treatment and sex. Differences in final body weight (FBW) were investigated with a similar model that included the fixed effect treatment and the covariates initial body weight (IBW) and total feed consumption (TFC) as potential explanatory variables. Backward selection was done by removing one term at a time, starting with non-significant interactions (p > 0.05). To investigate the differences in biochemical parameters, organ weights, and selected vitamins and minerals among the control and the two doses of Na-bentonite for males and females separately, a linear or a generalized linear mixed model was constructed with the fixed effect treatment as the only independent variable. The MIXED and GLIMMIX SAS procedures were used for all statistical analyses using SAS 9.2 with Enterprise Guide 4.1. In all of these analyses, subject (i.e. rat) was considered as a random effect. All post-test comparisons were adjusted by the method of Tukey-Kramer (Tukey, Reference Tukey1977). Total bilirubin data were analyzed with non-parametric tests, data were classified into categories before analysis with a Kruskal-Wallis test followed by the Mann-Whitney test (Mann & Whitney, Reference Mann and Whitney1947; Kruskal & Wallis, Reference Kruskal and Wallis1952).
Results and Discussion
Levels of Dioxins, Furans, and Heavy Metals in Na-bentonite
Detectable dioxins/furans in Na-bentonite included tetrachlorodibenzo-p-dioxin, hexachlorodibenzo-p-dioxin, heptachlorodibenzo-p-dioxin, tetrachlorodibenzo-p-furan, and pentachlorodibenzo-p-furan. The average calculated dioxin/furan toxic equivalents (TEQs) were 0.0811 pg/g. The Joint FAO/WHO Expert Committee on Food Additives (JECFA) has placed a provisional tolerable daily intake (PTDI) value for dioxins at 2.3 pg/kg body weight/day (Joint FAO/WHO Expert Committee on Food Additives [JECFA], 2002) for humans. To reach the PTDI level, and assuming bioavailability of the dioxins in the gastrointestinal tract, an average 70 kg person would have to consume approximately 2 kg of Na-bentonite per day. Under realistic conditions, consumption of bentonite will be at much lower levels that confer a very low risk of dioxin exposure. The metal levels found in Na-bentonite were 0.01 mg/g for As (metalloid), 0.003 mg/g for Cd, <0.00003 mg/g for Hg, and 0.03 mg/g for Pb. Using the tolerable daily intake (TDI) levels reported by the JECFA (JECFA, 1989, 2007, 2011), the TDIs calculated for a 70 kg person are 0.14 mg for As, 0.07 mg for Cd, 0.01 mg for Hg, and 0.21 mg for Pb. Even ingesting 3 g of Na-bentonite, which is the maximum level of calcium clays given in capsules to humans for reduction of AF bioavailability (Afriyie-Gyawu, Ankrah, et al., Reference Afriyie-Gyawu, Ankrah, Huebner, Ofosuhene, Kumi, Johnson, Tang, Xu, Jolly, Ellis, Ofori-Adjei, Williams, Wang and Phillips2008a), the exposure of these metals through ingestion would be very low.
Mineralogical Analyses
The mineral characteristics and elemental composition of Na-bentonite according to XRF data are summarized in Table 1. Insignificant reaction followed HCl application and trace amounts of calcite were detected by XRD (Fig. 1) suggesting that carbonate minerals were present in small amounts in Na-bentonite. Calcite could be associated with beneficial effects as some forms of calcium carbonate can act as a calcium supplement (Palacios et al., Reference Palacios, Ramirez and Lilue2020). Similarly, little or no reaction following H2O2 treatment suggested that Na-bentonite does not contain significant amounts of oxidizable or reducible components (e.g. manganese oxides, siderite, etc.) or organic matter. The pH of the sample was 8.3, a value within ranges reported for clay samples containing smectites (Kannewischer et al., Reference Kannewischer, Tenorio-Arvide, White and Dixon2006; Marroquin-Cardona et al., Reference Marroquin-Cardona, Deng, Taylor, Hallmark, Johnson and Phillips2009). The EC values observed suggested soluble salts were present in this sample. The presence of sulfates (i.e. gypsum) was suggested by the acetone test precipitation and was confirmed by XRD (Fig. 1). Gypsum is a mineral form of calcium sulfate, which is used commonly as a food additive (General Standard for Food Additives, [GSFA], 2019), hence its presence in Na-bentonite does not represent a risk. The percentages calculated for each fraction (sand, silt, and clay) showed that Na-bentonite was composed mainly of <2 μm clay particles (90%) (Table 1).
Table 1 Mineral characteristics and XRF elemental composition of Na-bentonite

aExtractable bases were measured in DI-water vacuum extracts from a paste made with 100 g of clay according to Rhoades and Clark (Reference Rhoades and Clark1978); ND not detected

Fig. 1 Powder mount XRD patterns of Na-bentonite. Minerals identified included montmorillonite (12.5, 5.1, 4.48, 2.56, 1.49 Å), gypsum (7.6 Å), quartz (3.3 Å), calcite (3.0 Å), orthoclase feldspars (3.7 Å), and andesine (3.24 and 3.20 Å)
The major minerals identified in the powder mount XRD were montmorillonite (12.5 Å), gypsum (7.6 Å), and quartz (3.3 Å) (Fig. 1). Peaks for quartz, mica (10.0 Å), and cristobalite (4.04 Å), as well as peaks of feldspars from the plagioclase group (e.g. anorthite) and alkali feldspars (sanidine and albite) were observed in the sand and silt fractions. The presence of montmorillonite was confirmed after XRD analysis of the clay fraction exchanged with Mg and K. After treatment with Mg and glycerol solvation, the d-spacing of the most intense montmorillonite peak increased from 15 Å to 18 Å. Samples exchanged with K and then air-dried, showed a peak of 12 Å, which is in the range expected for montmorillonites (10–14 Å) (Hawkins & Egelstaff, Reference Hawkins and Egelstaff1980; Oueslati et al., Reference Oueslati, Ammar and Chorfi2015). After heating the samples at 330°C and 550°C, the ~12 Å peak changed to ~10 Å and 9 Å, respectively. The XRD analysis also suggested the presence of quartz and opal CT in the clay fraction. The presence of montmorillonite was confirmed by the FTIR analysis. The specific identification of FTIR peaks is shown in Table 2. The SEM examination revealed quartz and feldspars with a variety of particle shapes and compositions. SEM also revealed traces of other minerals such as monazite particles containing phosphorus (P), lanthanides, and actinide elements such as cerium (Ce), neodymium (Nd), samarium (Sm), and protactinium (Pa) (Fig. 2a), and a particle composed of calcium phosphate compatible with hydroxylapatite (Ca5(PO4)3(OH) (Fig. 2b). Hydroxylapatite is a mineral that showed no adverse effects on mice and rabbits under acute and sub-chronic oral toxicity studies (Hanh et al., Reference Hanh, Ngoc-Bich and Thanh-Thao2019). The TEM images revealed the common folding tendency of the montmorillonite particles (Fig. 2c). The particles are shown as thin films of considerable length (3–5 μm). Moiré patterns were observed in some particles, and this effect was probably due to the overlap of plates with different orientation (Fig. 2d).
Table 2 ATR and KBr analysis of Na-bentonite

aThe 622 cm-1 band of Na-bentonite may also be referring to cristobalite, although it was not found in the SEM. Infrared bands identification was based on Madejová and Komadel (Reference Madejová and Komadel2001), Paluszkiewicz et al. (Reference Paluszkiewicz, Holtzer and Bobrowski2008) and Alabarse et al. (Reference Alabarse, Conceição, Balzaretti, Schenato and Xavier2011)
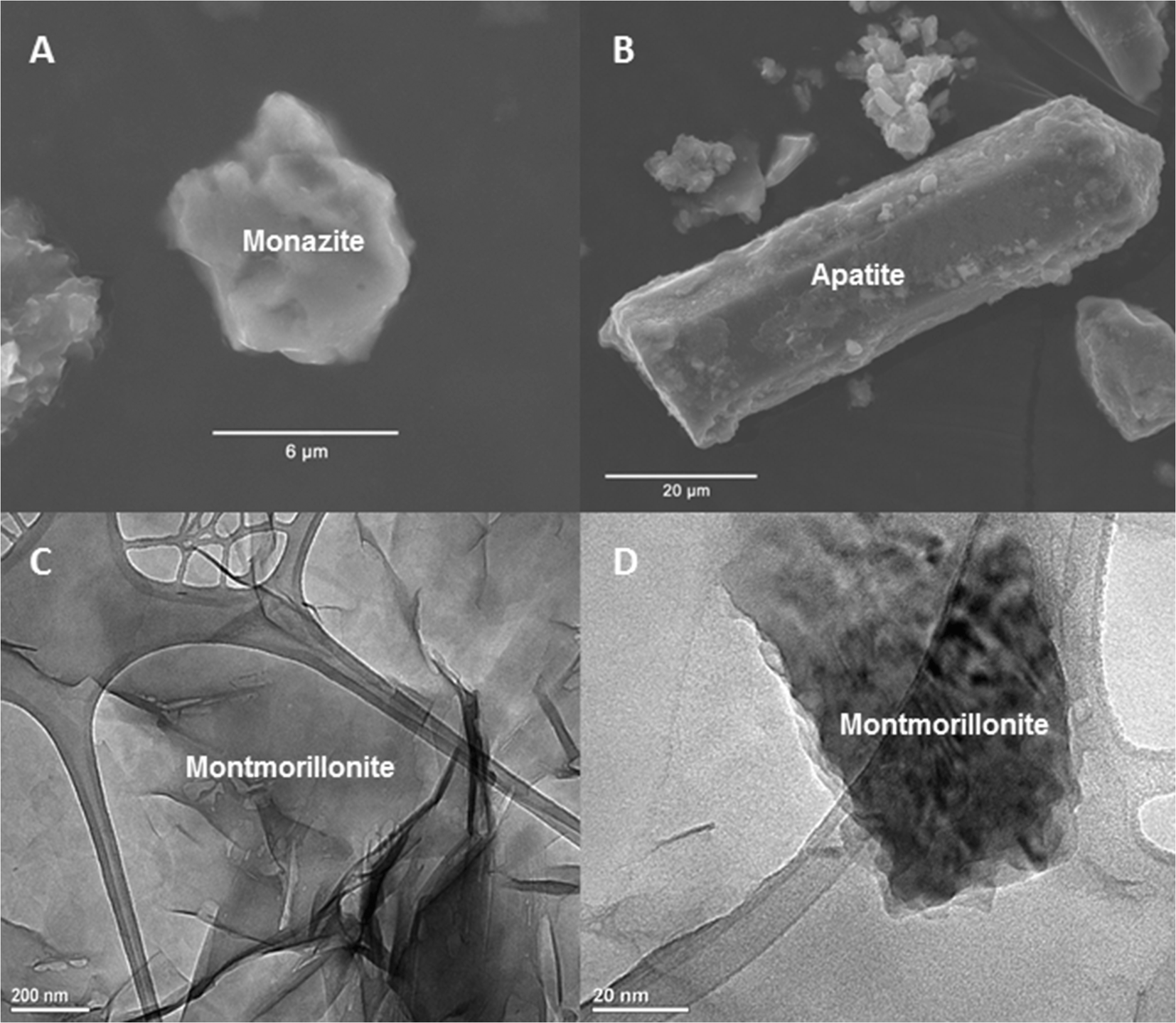
Fig. 2 A SEM image of monazite particle in Na-bentonite sample. B Hydoxylapatite particle in Na-bentonite. C Smectite particles in sheets and rolls with approximate length >1 μm, according to the scale bar. This morphology is commonly observed for effective aflatoxin adsorbents, according to Mulder et al. (Reference Mulder, Tenorio Arvide, White and Dixon2008). D Moiré patterns observed in a platelet of Na-bentonite
AFB1 Sorption Analyses
The parameters Q max and Kd calculated for Na-bentonite demonstrated the AF adsorption effectiveness of the clay in vitro. A favorable AF sorption pattern characterized as an L-shape curve at both pH levels (Fig. 3) was depicted for Na-bentonite. Specifically, an L2-shape was documented at pH 6.5 with a calculated Q max value of 0.42±0.02 mol AFB1/kg of clay (Langmuir fit r2 = 0.95). A slightly lower Q max of 0.39±0.01 mol AFB1/kg of clay (Langmuir fit r2 = 0.99) was calculated at pH 2.0, for which an L1 pattern was observed. The L1 shaped pattern is indicative of sorption processes in approximation of a plateau, while an L2 pattern suggests that saturation has been reached (Giles et al., Reference Giles, MacEwan, Nakhwa and Smith1960; Grant & Phillips, Reference Grant and Phillips1998). The differences in patterns may account for the different Q max values at pH 6.5 versus pH 2. The Kd values obtained at pH 6.5 and 2 were 5.45·105 and 2.86·105, respectively. According to the sorption capacity for AFB1 (Q max > 0.3 mol/kg of clay) and the mineral characteristics of Na-bentonite (pH of 8.3, high smectite composition, CEC value of 90.5, and FTIR evidence of framework Mg and/or Fe) this material could be considered for inclusion in feeds as an aflatoxin binder, according to the classification system proposed by Dixon et al. (Reference Dixon, Kannewischer, Tenorio-Arvide and Barrientos-Velazquez2008).

Fig. 3 Aflatoxin B1 adsorption isotherm for Na-bentonite at pH 6.5 and 2. An L-shaped pattern was observed at both pH levels. The bend in the pattern indicated that the sorbent reached saturation as the concentration of the solute was increased. The Q max (mol AFB1/kg) values were similar at both pH levels. Q max was calculated according to the formula: Q max = q/((Kd C w/(1+Kd C w)), with q being the concentration of AFB1 adsorbed (mol/kg), Q max the maximum capacity (mol/kg), Kd the distribution constant, and C w the equilibrium concentration of AFB1 (Grant & Phillips, Reference Grant and Phillips1998)
Rodent Study Outcomes
Consumption of Na-bentonite at either low or high dose did not cause any observable toxicity throughout the study. Importantly, the EFSA Panel on Additives and Products or Substances used in Animal Feed (EFSA-FEEDAP) recently concluded that bentonite at inclusion levels of 2% w/w in complete feed is safe for all animal species (EFSA FEEDAP Panel, 2017). In the current study, all rats remained healthy and active. Males gained more weight than the females (p ≤ 0.0001), as expected (Fig. 4). Weight-gain over time was different in at least one of the treatment groups (p ≤ 0.0001), but the interaction between treatment and sex was not significant (p = 0.6780). Rats in the control group gained more weight than rats in the 2% Na-bentonite group by time 5 (day 13) (p = 0.0019) and consecutively up to time 30 (day 88) (p ≤ 0.0001). Similarly, animals in the control group gained more weight than the 0.25% Na-bentonite group by time 20 (day 58) (p = 0.0131) and consecutively at time 30 (day 88) (p = 0.0002). In the females, a significant difference was observed for FBW between the control and the Na-bentonite treatment groups (Table 3). The TFC and IBW were not significant factors for explaining the difference in FBW. Similarly, in males, IBW was not a significant factor for explaining the difference in FBW. However, in males a linear relationship was observed between TFC and FBW in both treatment groups (p = 0.0278). Differences in growth parameters between male and female rats were explained by Slob and Van Der Werff Ten Bosch (Reference Slob and van der Werff Ten Bosch1975), revealing that neonatal androgens play the most important part in increasing growth in males while ovarian secretions starting in puberty suppress growth rate in females.

Fig. 4 Growth charts of SD rats ingesting Na-bentonite. Mean body weight (g) of males and females are plotted versus time. In total, the body weights were recorded 30 times
Table 3 Growth parameters and organ weights of SD rats receiving the control diet and a diet with 0.25 and 2.0% Na-bentonite for 3 months

aMeans with asterisk are significantly different from the control at the 0.05 level
bSignificantly different between Na-bentonite groups at the 0.05 level
FBW Final body weight, FCE Feed conversion efficiency, IBW Initial body weight, TBWG Total body weight gain, TFC Total feed consumption. Data are reported as mean values ± standard deviation
Hematological and Serum Analysis
Some differences between the control and at least one of the Na-bentonite treatment groups were observed for hematological, serum vitamin, and micronutrient parameters (Tables 4 and 5). Relevant findings included increased vitamin E levels observed in both females and males of Na-bentonite treatment groups suggesting a potential protective effect of the clay on the vitamin, or the ability of the clay to enhance vitamin E bioavailability; however, more research is needed to delineate the specific mechanisms involved with this observation. With regards to vitamin A, male rats ingesting 2% Na-bentonite had significantly lower serum levels of this vitamin than the control group. Interactions of vitamin A and bentonites have been observed previously in rats and chickens at high dietary inclusion rates of bentonite (i.e. >3% w/w) (Briggs & Spivey, Reference Briggs and Spivey1954; Laughland & Phillips, Reference Laughland and Phillips1954; ). However, more recent studies involving SD rats, using 2% inclusion rates of a similar bentonite, have shown no significant changes in serum vitamin A levels, when compared to the control (Afriyie-Gyawu et al., Reference Afriyie-Gyawu, Mackie, Dash, Wiles, Taylor, Huebner, Tang, Guan, Wang and Phillips2005; Marroquin-Cardona et al., Reference Marroquin-Cardona, Deng, Garcia-Mazcorro, Johnson, Mitchell, Tang, Robinson II, Taylor, Wang and Phillips2011). The difference between the effects of Na vs. Ca bentonites on vitamin A may be attributed to differences in particle size and/or swelling characteristics.
Table 4 Blood and serum parameters of female SD rats receiving dietary Na-bentonite at 0.25 and 2% for 3 months

aMeans with asterisk are significantly different from the control at the 0.05 level
bSignificantly different between NaB groups at the 0.05 level
cData are reported as mean values ± standard deviation
Hb hemoglobin, MCH mean cell hemoglobin, MCHC mean corpuscular hemoglobin concentration, MCV mean corpuscular volume, PCV packed cell volume, RBC red blood cell, WBC white blood cell. ALB albumin, ALP alkaline phosphatase, ALT alanine aminotransferase, AMYL amylase, AST aspartate aminotransferase, BUN blood urea nitrogen, CHOL cholesterol, CK creatine kinase, CTR creatinine, GGT gamma-glutamyl transferase, GLOB globulin, GLUC glucose, T-BIL total bilirubin, TP total protein. References: (1) Wolford et al. (Reference Wolford, Schroer, Gohs, Gallo, Brodeck, Falk and Ruhren1986); (2) Lillie et al. (Reference Lillie, Temple and Florence1996); (3) Almodovar-Cuevas et al. (Reference Almodovar-Cuevas, Navarro-Ruiz, Bastidas-Ramirez, Mora-Navarro and Garzon1985; (4) Kohn & Clifford, (Reference Kohn, Clifford, Fox, Anderson, Loew and Quimby2002); (5) Afriyie-Gyawu et al. Reference Afriyie-Gyawu, Mackie, Dash, Wiles, Taylor, Huebner, Tang, Guan, Wang and Phillips2005
Table 5 Blood and serum parameters of male SD rats receiving dietary Na-bentonite at 0.25 and 2% for 3 months
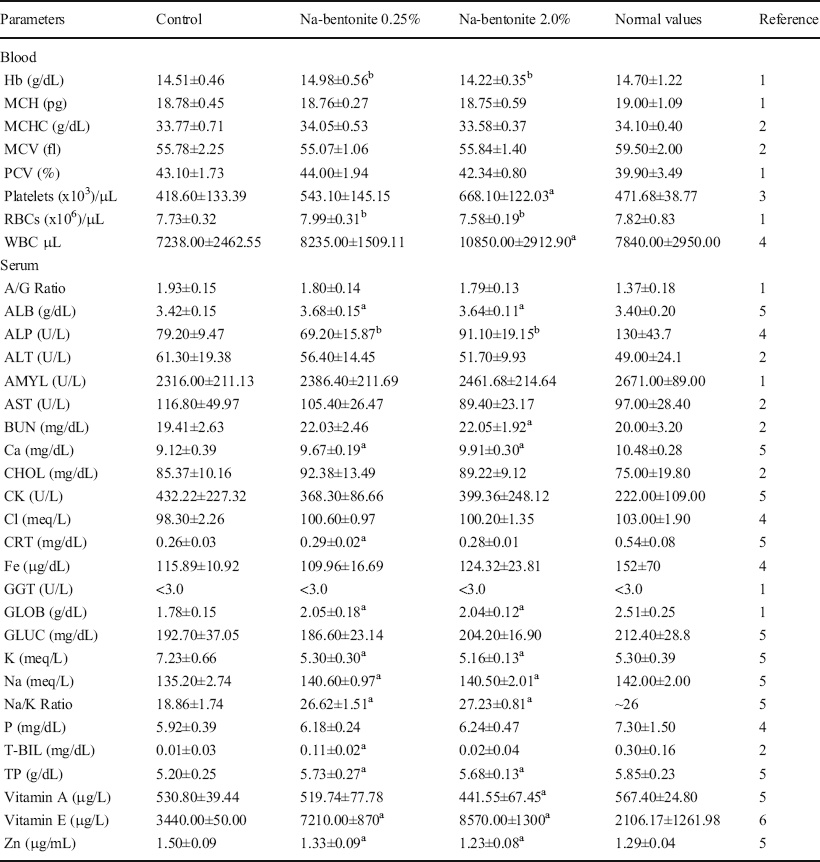
aMeans are significantly different from the control at the 0.05 level
bSignificantly different between Na-bentonite groups at the 0.05 level
cData are reported as mean values ± standard deviation
Hb hemoglobin, MCH mean cell hemoglobin, MCHC mean corpuscular hemoglobin concentration, MCV mean corpuscular volume, PCV packed cell volume, RBC red blood cell, WBC white blood cell, ALB albumin, ALP alkaline phosphatase, ALT alanine aminotransferase, AMYL amylase, AST aspartate aminotransferase, BUN blood urea nitrogen, CHOL cholesterol, CK creatine kinase, CTR creatinine, GGT gamma-glutamyl transferase, GLOB globulin, GLUC glucose, T-BIL total bilirubin, TP total protein. References: (1) Wolford et al., (Reference Wolford, Schroer, Gohs, Gallo, Brodeck, Falk and Ruhren1986); (2) Lillie et al. (Reference Lillie, Temple and Florence1996); (3) Almodovar-Cuevas et al. (Reference Almodovar-Cuevas, Navarro-Ruiz, Bastidas-Ramirez, Mora-Navarro and Garzon1985); (4) Kohn & Clifford, Reference Kohn, Clifford, Fox, Anderson, Loew and Quimby2002; (5) Afriyie-Gyawu et al. (Reference Afriyie-Gyawu, Mackie, Dash, Wiles, Taylor, Huebner, Tang, Guan, Wang and Phillips2005); 6) Seifi et al. (Reference Seifi, Kadkhodaee, Zahmatkesh, Golab and Bakhshi2009)
Concerning serum cations, an interesting finding was the significant reduction in serum K of both males and females from Na-bentonite treatment groups when compared to control groups. Limited information on the effects of these types of clays on serum K is available though some clinical reports have linked the consumption of soils or clay to hypokalemia in pregnant and non-pregnant women (McKenna, Reference McKenna2006; Severance Jr. et al., Reference Severance, Holt, Patrone and Chapman1988; Trivedi et al., Reference Trivedi, Daga and Yeolekar2005; Ukaonu et al., Reference Ukaonu, Hill and Christensen2003). Nevertheless, these reports lack specific information regarding the mineral identification of the ingested material. Other studies using ionic K-concentrated groundwater revealed the ability of bentonite to adsorb K from the water over a period of 9 months, while findings for Na were opposite, for which an increase was observed over time (Melamed & Pitkänen, Reference Melamed and Pitkänen1996). This may be related to inherent cation exchange abilities of different types of bentonites. Interestingly, a study using a Ca-bentonite has shown similar effects on serum K levels in SD rats (Marroquin-Cardona et al., Reference Marroquin-Cardona, Deng, Garcia-Mazcorro, Johnson, Mitchell, Tang, Robinson II, Taylor, Wang and Phillips2011). Importantly, in both studies the serum K levels were within normal physiological ranges for SD rats. Moreover, no effect of Ca-bentonite clay was observed in serum K level of humans ingesting up to 3 g a day for up to 3 months (Afriyie et al., Reference Afriyie-Gyawu, Wang, Ankrah, Xu, Johnson, Tang, Guan, Huebner, Jolly, Ellis, Taylor, Brattin, Ofori-Adjei, Williams, Wang and Phillips2008b).
Serum Zn was another serum cation for which significant differences from the control were observed, although this only occurred in the males of the Na-bentonite groups. Interactions of similar bentonite clays (e.g. hydrated sodium calcium aluminosilicate; HSCAS) with Zn have been reported by Chung et al. (Reference Chung, Erdman and Baker1990). In that study, the authors observed significantly (p < 0.05) lower Zn levels in the tibia of chickens fed 1% HSCAS in the diet. Tibial Zn concentration is a more reliable indicator of Zn levels in the body than serum (Wedekind & Baker, Reference Wedekind and Baker1990); however, serum and plasma Zn have both been reported as useful indicators of short-term changes in dietary zinc for SD rats (Baltaci et al., Reference Baltaci, Mogulkoc and Halifeoglu2005; Sunar et al., Reference Sunar, Baltaci, Ergene and Mogulkoc2009). Normal clinical ranges of serum Zn for 3-month-old SD rats are not available in the literature, but the levels shown in the present study were within the normal clinical ranges reported for 6-month-old SD rats (Afriyie-Gyawu et al., Reference Afriyie-Gyawu, Mackie, Dash, Wiles, Taylor, Huebner, Tang, Guan, Wang and Phillips2005). Results from the present study also agree with data from a Ca-bentonite study (Marroquin-Cardona et al., Reference Marroquin-Cardona, Deng, Taylor, Hallmark, Johnson and Phillips2009). The main differences between these types of bentonites (Ca and Na) are their cation saturation and swelling capacity upon hydration. Both bentonites have high CEC values, and these types of clays may retain cation exchange abilities in the gastrointestinal tract. These chemical properties may potentially explain the observed reduction in serum Zn in the males (see Table 4), in agreement with the suggestion that some geophagic clays may interfere with the absorption of cations (Young et al., Reference Young, Sherman, Lucks and Pelto2011). Nonetheless, the Na-bentonite effects on Zn were observed only in males (not in females) and further work is needed to confirm this finding. The supplementation of some cations by soils has also been proposed (Wiley & Katz, Reference Wiley and Katz1998) and this may be related to the observed increase in serum Na (see Table 5) in both males and females of Na-bentonite groups. Serum Ca was also significantly increased in males from Na-bentonite treated groups. Similar studies using SD rats have found significant increases in serum Ca levels in females ingesting 0.5% and 2.0% of Ca-bentonite (Afriyie-Gyawu et al., Reference Afriyie-Gyawu, Mackie, Dash, Wiles, Taylor, Huebner, Tang, Guan, Wang and Phillips2005), and in females and males ingesting 0.25 and 2% of a refined Ca-bentonite (Marroquin-Cardona et al., Reference Marroquin-Cardona, Deng, Garcia-Mazcorro, Johnson, Mitchell, Tang, Robinson II, Taylor, Wang and Phillips2011). In the present study, the females from the 2% Na-bentonite treatment had significantly increased levels of Ca, but this did not occur at the lower dose (0.25% Na-bentonite). Calcium sources in Na-bentonite may include calcite, gypsum, and montmorillonite as evidenced on the XRD patterns (Fig. 1), and the potential supplementation of Ca by clays has been discussed previously (Wiley & Katz, Reference Wiley and Katz1998; Wilson, Reference Wilson2003). Other notable outcomes, in males and females of both Na-bentonite groups, included increased serum Na/K ratio (Tables 4 and 5). The interactions of clays with cations may not always lead to deficiencies, but, depending on the chemistry of the cation, in some cases supplementation may occur. Importantly, in the present study, no effects of the clay were observed for serum P as other studies have suggested. For instance, reduction of serum P in chickens was attributed to diets containing 0.5% inclusion of bentonite (Santurio et al., Reference Santurio, Mallmann, Rosa, Appel, Heer, Dageforde and Bottcher1999). In the current research, serum P levels in rats from Na-bentonite groups were not significantly different from the control.
The rest of the parameters measured in blood and serum were either not significantly different from the control group or, if different from the control, they fell within normal clinical ranges and showed no evidence of dose dependency (Tables 4 and 5).
Organ Weights
Isolated differences were observed for some of the organ weights for males (Table 3). Specifically, liver and brain weights of males of both Na-bentonite groups were different when compared to the control. However, this was not observed in the females and no indication of a dose dependent effect was found.
Conclusions
Na-bentonite is a material with a large montmorillonite content and with favorable sorption patterns for AF. In general, no adverse health effects resulted from the ingestion of Na-bentonite at 0.25% and 2% inclusion in diet for up to 3 months in SD rats. The consumption of Na-bentonite was associated with increased serum Na and vitamin E, and decreased serum K in males and females, at both inclusion levels. Calcium serum levels were increased in males of both Na-bentonite groups and in females from the high dose group. Reduction of nutrients such as serum vitamin A and Zn were noted in the 2% Na-bentonite group (males-females) and in the males of both 0.25% and 2% inclusion levels, a trend previously observed for other bentonites. Besides the differences observed when comparing Na-bentonite treatments with control groups, no evidence of dose dependency was found, and all parameters fell within normal clinical ranges reported for SD rats. Nonetheless, due to the inherent cation exchange capacity of these types of clays, the current findings with Ca, Na, K, and Zn warrant further research.
Acknowledgments
The authors thank AMCOL International Corporation (Hoffman Estates, Illinois) for funding this research and for providing the Na-bentonite used in the present study.
Code Availability
Not applicable
Authors' Contributions
Alicia G. Marroquin-Cardona: experimental work of animal study, samples collection, writing, submission.
Youjun Deng: supervision of mineral analyses, revision of data and manuscript.
Jose F. Garcia-Mazcorro: statistical analysis.
Natalie M. Johnson: experimental work in animal study, sample collection.
Nicolle J. Mitchell: experimental work in animal study, sample collection.
Lili Tang: vitamin analyses.
Jia-Sheng Wang: vitamin analyses.
Roger B. Harvey: co-advisor, revision of documents.
Timothy D. Phillips: graduate advisor and sponsor of this research.
Funding
Funding sources are as stated in the Acknowledgments.
Data Availability
Not applicable
Declarations
Ethics Approval
The animal use protocol (AUP) 2008-39 was reviewed and approved by the Institutional Animal Care and Use Committee (IACUC) at Texas A&M University.
Consent to Participate
Not applicable
Consent for Publication
All authors give consent for publication.
Conflict of Interest
The authors declare that they have no conflict of interest.