1 Introduction: designing with uncertainty
Engineering design is subject to huge uncertainties as requirements emerge and evolve, the world in which the product operates changes, and teams discover what they can and cannot do as part of the design process. The information that designers work with is fluid, and the representations of the incomplete design are often sketchy, incomplete and sometimes ambiguous. Moreover, the knowledge, experience and expertise of each individual designer is different and is constantly changing through their private and professional lives. Engineering designers have an amazing capacity to use models of designs and information about requirements and decisions that are provisional, incomplete and sometimes downright wrong and still successfully complete their projects. When individuals, groups or states are faced with similar degrees of uncertainty, they struggle and falter, but engineers just handle this routinely.
This paper argues that one way to understand why this is the case is to think of the designers of complex systems as playing games of make-believe, in which they consciously act as though provisional and uncertain information were certain while retaining awareness of its provisional and changing status. It argues that the notion of games of make-believe (derived from the ideas of Walton (Reference Walton1990)) explicates what designers are doing when they make and discard assumptions to handle the complexity of large-scale design projects and, in particular, how these assumptions are coordinated across large design teams. It illustrates some of its points with an example, the development of non-rotary components of a jet engine, drawing on one of the authors’ research on design margins (Eckert, Isaksson & Earl Reference Eckert, Isaksson and Earl2014, Reference Eckert, Isaksson and Earl2019).
Humans tackle complex problems by attempting to decompose them into manageable sub-problems. As Simon (Reference Simon1996) pointed out, most artificial complex systems are ‘almost decomposable’, into components that are relatively loosely coupled but can have complicated interactions. Frequently, these components need to be designed in parallel. Designers need to make assumptions in order to be able to define and work with problems that are simple enough to be tractable (Braha & Bar-Yam Reference Braha and Bar-Yam2004a,Reference Braha and Bar-Yamb) and close enough to reality to be useful. In particular, designers of components of complex systems need to make assumptions about what other components will do that will need to be revised as the whole design develops. As the design is refined, these assumptions are adjusted to reach a set of mutually compatible values and harden into firm decisions.
The aim of the paper is to present an analysis of what engineering designers are doing, rather than an analysis of how they do it. This is a theory of designing at a more abstract and fundamental level than the cognitive processes involved in design thinking, which should explicate the tasks involved in designing as groundwork for understanding designers’ reasoning.
Our analysis is partly motivated by our long-standing interest in models and how they are used in both science and design (Stacey Reference Stacey1992; Eckert & Stacey Reference Eckert, Stacey, Heisig, Clarkson and Vajna2010; Poznic Reference Poznic2016b, Reference Poznic2018; Eckert & Hillerbrand Reference Eckert, Hillerbrand, Vermaas and Vial2018). In our view, the notion of games of make-believe helps to resolve critical conceptual problems to do with how scientists and engineers use models that have varied and changing relationships to target systems that (initially) do not exist, and so have an ontological status that is both slippery and subject to change; however we will not develop this argument in the present paper. We also have a long-standing interest in the design processes of complex products (Eckert, Clarkson & Zanker Reference Eckert, Clarkson and Zanker2004; Eckert & Clarkson Reference Eckert and Clarkson2010; Tahera, Earl & Eckert Reference Tahera, Earl and Eckert2017; Tahera et al. Reference Tahera, Wynn, Earl and Eckert2018).
This includes trying to understand how designers handle imprecise, uncertain and provisional design information (Stacey & Eckert Reference Stacey and Eckert2003). Engineers have a range of methods for quantifying and managing risks and uncertainty. Axiomatic design is an example of a design method intended to enable engineering designers to manage uncertainty (Suh Reference Suh2001). Existing research on uncertainty focuses on classifying uncertainty (for instance, De Weck, Eckert & Clarkson Reference De Weck, Eckert and Clarkson2007), quantifying uncertainty (for instance, Wood & Antonsson Reference Wood and Antonsson1990), designing under conditions of uncertainty (for instance, Yao et al. (Reference Yao, Chen, Luo, van Tooren and Guo2011), on uncertainty-based multidisciplinary design optimisation methods), managing design processes under conditions of uncertainty (Ramasesh & Browning Reference Ramasesh and Browning2014) and risk (for instance, Oehmen et al. Reference Oehmen, Olechowski, Kenley and Ben-Daya2014), on developing decision-making strategies or tools (for instance, Yang & Singh Reference Yang and Singh1994), and on how individuals reason with uncertainty (for instance, Ball & Christensen Reference Ball and Christensen2009; Van Bossuyt et al. Reference Van Bossuyt, Dong, Tumer and Carvalho2013). Design research is often concerned with the assessment of risk (see for example Möller & Hansson (Reference Möller and Hansson2008) for a review of principles of safety engineering) and ensuring that design process (for instance, Wynn, Grebici & Clarkson Reference Wynn, Grebici and Clarkson2011) and products (for instance, Leveson et al. Reference Leveson, Dulac, Zipkin, Cutcher-Gershenfeld, Carroll, Barrett, Hollnagel, Woods and Leveson2006) are resilient to the risks throughout their product life cycles. However, this paper is concerned with how people involved in the design of complex products are able to work with these uncertainties as a routine part of their professional lives.
The paper argues that during the course of the development process, designers play multiple games of make-believe as the assumptions they work with are modified in an orderly way, and their different tasks require them to imagine different things and describe them with increasing degrees of precision. The development process thus comprises a network of games with slightly different rules, with complex but coherent relationships between them. Viewing designing in terms of games of make-believe helps understand how engineers coordinate large-scale projects while the information they share remains uncertain and slippery.
While designing involves a wide range of activities, the core of what designing is, is using information relevant to the design, especially representations of the design and what it needs to do or be like, that are initially incomplete, skeletal, uncertain and vague, to create new or more refined representations of the design that are more complete, detailed and exact (see for instance Visser Reference Visser2006a,Reference Visserb; Cross Reference Cross2008).
This perspective fits with and illuminates experimental and observational studies of design practice. Designers find it difficult to communicate the degree of uncertainty associated with design information (Stacey & Eckert Reference Stacey and Eckert2003) and are on some level acutely aware that the information they work with is uncertain; yet they work systematically with the information that they are provided and confidently take decisions that they know will be undone later. Adopting and modifying Walton’s (Reference Walton1990) make-believe theory allows us to interpret this behaviour of the designers as playing games of make-believe and enables us to see the representations through which designers interact with the emerging design and its design process as props in games of make-believe. When these games are part of organised design processes, they are often stable and widely shared; then the agreed rules of the game give the power of authority to the results of the imagining acts that simply supposing something does not do.
The structure of the paper is as follows. In Section 2, Walton’s make-believe theory is introduced. After that, Section 3 discusses the elements of the make-believe games played by designers. An illustrative example of jet engine design is used to make the case for the application of the make-believe theory to designing in Section 4. Section 5 looks at how make-believe games in design change through time, and puts forwards the argument that the theory has to be expanded because the ‘authorised game’ of design is not a single game but there are rather many interconnected games that have to be changed in an authorised way. Finally, the paper concludes in Section 6 with a summary of how make-believe theory contributes to the multidisciplinary study of design.
2 Games of make-believe in art and science
This paper has adopted the idea of a game of make-believe as a way to conceptualise many design activities from recent work in philosophy of science; the philosophers of science have adopted it from philosophical aesthetics, primarily the work of Walton (Reference Walton1990). Walton’s theory is known in different contexts as make-believe theory, pretence theory and prop theory. The central idea we take from it is that people can and do understand what is or is not true within a fiction by reasoning as though the information in a representation such as a story were true, without committing to it actually being true. This approach has been extended to games by Bateman (Reference Bateman2011).
Walton is interested in how representational arts work. A key question is how readers of fictional works can form an understanding of what is the case, and what is not, within a fictional world defined by such an artwork. Further questions are how the readers agree about this and how statements about what is fictionally true can be correct. Philosophers of science, notably Frigg (Reference Frigg2010) and Toon (Reference Toon2012), have applied Walton’s ideas to understanding how scientists can use models of scientific phenomena to understand these phenomena, when they know that the models are simplifications or are wrong in significant ways; and to trying to understand what scientific models are.
2.1 Walton’s make-believe theory
According to a common perspective on fiction, works of fiction such as novels, as well as paintings and other artworks, define fictional worlds in which certain statements are fictionally true. For example, in the world of Arthur Conan Doyle’s stories, a statement like ‘Sherlock Holmes lived at 221B Baker Street, London’ is fictionally true while a statement like ‘Moriarty was a professor of engineering design’ is not fictionally true.
According to Walton’s (Reference Walton1990) theory, appreciating a representational artwork involves participating in a game of make-believe by imagining that its content were true, and inferring what follows from that, while remaining aware that it is fiction. Objects that contribute to games of make-believe are props, a term he has borrowed from theatre. Props can be objects used in games, like hobby horses or puppets, as well as game elements such as title deed cards in Monopoly; for instance, a broom could be used to play an imagination game involving riding, making ‘Sangeeta is now riding the horse’ a fictionally true statement. Players are supposed to imagine the propositions that are fictionally true. Walton is chiefly concerned with how semantic objects that have content, such as novels and paintings, function as props. The props are used in a game of make-believe to generate the understanding of the fictional world. But props do more than that – they prescribe what can and cannot legitimately be imagined within the game and thus what is fictionally true and what is not. As the two example sentences from above show, Arthur Conan Doyle’s stories as props legitimise only the first of these sentences to be imagined but not the second one. Thus, a statement S is fictional in a work W if the statement is true in the world defined by the work. Players of the game should imagine the set of fictional propositions, and agree that they are fictional, whether or not they actually do imagine these propositions.
Playing the game of make-believe to imagine the fictional statements and knowing what is fictionally true and what is not involves applying principles of generation: these encompass among other things all the knowledge of the world and human society we bring to bear to read stories, but also knowledge of language and genre conventions. In Walton’s terminology, the props and the principles together generate the fictional propositions of a game of make-believe. What these principles in the end are is a difficult question. Walton distinguishes between fictional truths that are directly generated – primary fictional truths – and fictional truths that are implied (cf. Walton Reference Walton1990, 140). The implied fictional truths are implied by primary ones and principles of generation. Two candidates for principles of generation are the Reality Principle and the Mutual Belief Principle. These are widely discussed in the scholarly literature on fiction. Concerning the first, Walton writes:
The basic strategy which the Reality Principle attempts to codify is that of making fictional worlds as much like the real one as the core of primary fictional truths permits. It is because people in the real world have blood in their veins, births, and backsides that fictional characters are presumed to possess these attributes. (Walton Reference Walton1990, 144)
Concerning the second one, he writes:
A storyteller, in a culture in which it is universally and firmly agreed that the earth is flat and that to venture too far out to sea is to risk falling off, invents a yarn about bold mariners who do sail far out to sea. No mention is made in the story of the shape of the earth or the danger. That would be unnecessary, the teller thinks, for he and his audience assume the earth in the story to be shaped as they believe it is in reality. (Walton Reference Walton1990, 150)
Walton problematises both of them. They deliver adequate answers in many cases but not in all cases.
Both will turn out to be seriously inadequate when measured against the subtleties and complexities of actual implications. Nevertheless, many implications do conform to one or the other of them, and recognizable variants are at work in some other cases. (144)
The unruly behavior of the machinery of generation makes life hard for critics. But it is no threat to the theorist; it presents the artist with exciting opportunities; and it is a rich source of fascination for the appreciator. (187)
Bateman (Reference Bateman2011) points out that many stories and games depend on the participants sharing background information and assumptions coming from a larger fictional world or otherwise having the character of make-believe. He terms this the Shared Mythology Principle.
Walton’s theory can be criticised for not telling us the whole story about how we experience literature and film; for instance, New (Reference New1999) pointed out that Walton neglects the role of illusion, into which the viewers knowingly and willingly enter, in the appreciation of art or film. However, this does not stop his ideas being useful for understanding how we actively construct an understanding of hypothetical situations depending on the acceptance of assumptions.
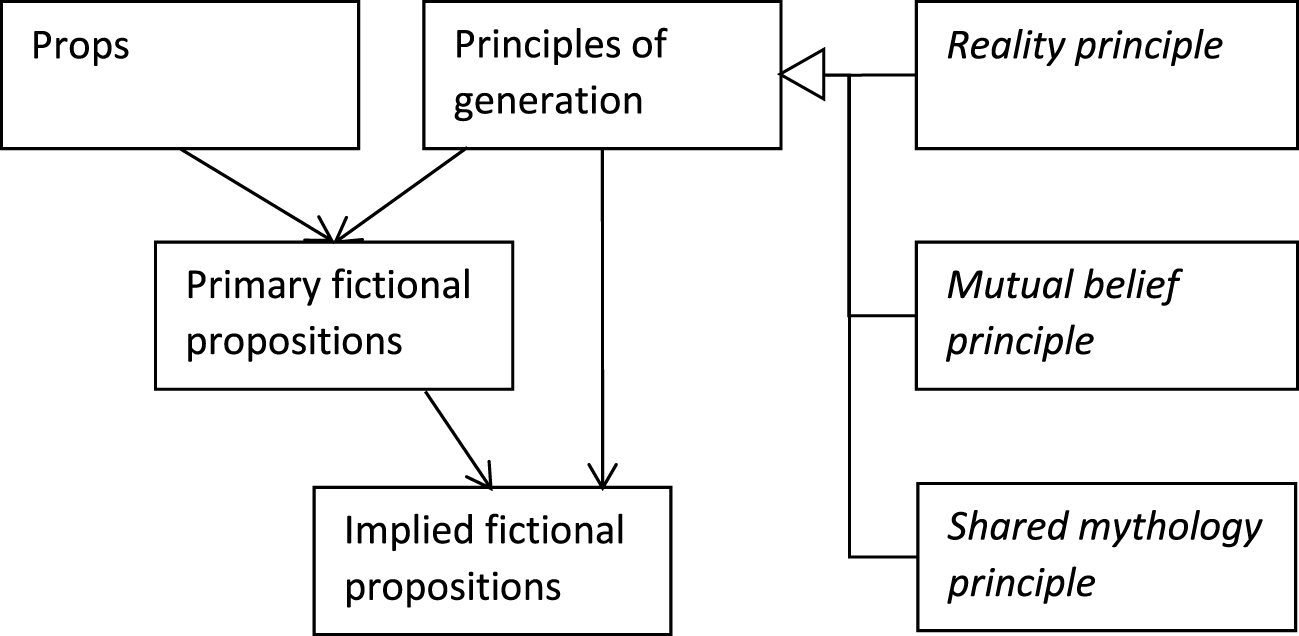
Figure 1. Overview of Waltonian concepts (open arrowheads indicate derivation relationships; triangular arrowheads indicate subtype–supertype relationships).
Walton (Reference Walton1990) refers to rules, principles, and principles of generation interchangeably. (Whether the cognitive processes involved in recognising objects and patterns and situations and making inferences about scenes and stories ought to be characterised as applying rules is, of course, debated; see Bateman (Reference Bateman2011, pp. 149–152). Walton interprets the notion of rule very loosely. Wolterstorff (Reference Wolterstorff1980, Reference Wolterstorff1991) prefers to think of making sense of artworks in terms of dispositions to see or conclude. The key point for Walton’s theory, and for us, is that an appropriately trained member of a community of practice should make particular inferences.) This paper sticks as far as possible to ‘principles of generation’ to refer to the knowledge resources used to make use of the props. Figure 1 gives an overview of the Waltonian concepts.
2.2 Authorised games of make-believe
The notion of authorised game is crucial to Walton’s theory. Authorised games are those with an established and socially shared set of props and principles that cannot be arbitrarily changed by the participants, so that what is fictionally true in the imagination game is both well defined and fixed. Different participants in the game have an objectively given basis for what they are allowed and required to imagine, so they should agree on which statements are fictionally true.
A completed artwork such as a novel constitutes a prop of an authorised game. What is fictionally true in such an authorised game cannot arbitrarily be changed by a player who engages in the game. While we can imagine Arthur Conan Doyle making Moriarty a medical doctor and make inferences about what would be the case in this situation, it is unalterably the case that it is fictional in the game of the Sherlock Holmes stories that Moriarty is a professor of mathematics. If we imagine Moriarty as a medical doctor, we are aware that we are considering a situation which is different from the situation that is mandated to be imagined by the Sherlock Holmes stories, and that our inferences about it have a different status from our inferences about the world of the standard Sherlock Holmes game.
Walton seems to be concerned mainly with authorised games after their completion, when the limits of what can be legitimately imagined are set. However, in design, how the limits of what can correctly be imagined – what the game is – are defined and modified through time, and with what authority, is a complicated and crucial issue.
2.3 Games of make-believe in science: Waltonian fictionalism
Walton’s ideas have been adopted by philosophers of science (Frigg Reference Frigg2010; Toon Reference Toon2012) to make sense of scientists speaking of models of scientific phenomena as objects similar to ordinary things that have concrete properties. For example, many models make unrealistic simplifications, like assuming that a sliding object has no friction, yet they are used to reason about ordinary objects with friction. These philosophers suggest that we can think of modelling practices as aspects of science that involve games of make-believe using shared props – model descriptions of scientific phenomena – and shared principles of generation – laws of nature and other scientific principles – to imagine ‘fictional’ propositions. Modellers in science use models in order to study what would be true if the models were correct, and so to reason about what might be true of the world within the limits of the accuracy of the models. In line with Walton’s theory, the scientists imagine certain fictional propositions that they infer from the models, without thereby being committed to the truth of these propositions in the real world. In the philosophy of science, this view is called Waltonian fictionalism. (There are other kinds of fictionalism in the philosophy of science, mostly stemming from the work of Vaihinger (Reference Vaihinger1911); see Fine (Reference Fine1993); see Godfrey-Smith (Reference Godfrey-Smith2006) for a non-Waltonian fictionalist account of modelling in science.) According to some scholars, this term is unfortunate and somewhat dangerous. For example, Giere (Reference Giere and Suárez2009) points out that scientific models and theories are intended as accurate accounts of the real world and are not works of fiction. Although Frigg and Toon’s accounts differ and are both open to criticism (see Weisberg Reference Weisberg2013; Poznic Reference Poznic2016a), the approach enables us to understand some crucial aspects of how scientists use models of natural phenomena. One example that is discussed in the philosophical literature on fictionalism and modelling is the Newtonian model of the earth orbiting around the sun. Here, the earth and the sun are treated as bodies with a homogeneous distribution of mass so that they could be seen as point masses and the shape of the elliptical orbit of the earth can be calculated.
So far, the philosophers of science have been concerned with applying fictionalist ideas to the use of models to understand reality rather than with the construction or modification of the models themselves. Accepting a particular model as given, thus defining an authorised game, is unproblematic for them. Science studies actual physical objects and processes that exist independently of the models, so the idea that the model is a model of some actual physical process that exists, to which it needs to conform (or be wrong), seems to be unproblematic in such a context. However, the distinction that matters here is not between science and engineering, but between analysis and design – between what-if reasoning about whether a model is accurate and what follows if it is, and what-if reasoning to refine and extend a set of models to specify an artefact. (For instance, Weisberg (Reference Weisberg2013) discusses an example that blurs the distinction between science and engineering but remains firmly within the category of analysis, the US Army’s hydraulic model of the Reber plan to dam the San Francisco Bay.) In the context of design, models are used to construct or modify other models. So, the models are often used for modelling things that not only do not exist yet but also have not been fully specified yet.
The nature of scientific models as well as engineering or other models and their relationships to their targets has been the subject of a lot of debate (Suárez Reference Suárez2003; Giere Reference Giere2004; Callender & Cohen Reference Callender and Cohen2006; Frigg Reference Frigg2006, Reference Frigg2010; Nguyen Reference Nguyen2016; Poznic Reference Poznic2016b,Reference Poznicc; Boesch Reference Boesch2017 among many others). Furthermore, there are scholars whose accounts allow for models that do not have targets (e.g., Boon & Knuuttila Reference Boon, Knuuttila and Meijers2009; Toon Reference Toon2012; Weisberg Reference Weisberg2013).
3 The elements of games of make-believe in design
In design, the creation of a new design is played out on the backcloth of scientific laws, rules and principles, and depends on the knowledge and shared interpretation of these by all the designers. The idea of an authorised game with its props and principles of generation gives us a conceptual framework for interpreting the provisional conclusions of design thinking as rational reasoning producing inferences that have well-understood and conceptually sound degrees of belief; as well as for seeing how concepts, data and material artefacts contribute to reasoning about not-yet-existing artefacts. In this section, we present a more or less static view of designing as make-believe at a particular point in the design process; we look at how the game changes through time as the design develops in Section 5.
3.1 Design as a shared game
Many thinking tasks in design involve reasoning from the current state of the design to what follows from it, to rerepresent the design in a different form to make different aspects explicit, to understand and make explicit what other parts of the design need to be like to fit it, how the structure of the design influences its performance, and so on. Many design tasks are purely analytic, that is, they generate new information deductively; but some involve creative synthesis: making new design decisions to refine and add to the design. Individual designers are free to put forward and retract ideas and provisional decisions with varying degrees of commitment and see how they work. We can see this in Waltonian terms as a game of make-believe, but it is not an authorised game.
However collaborative designing by teams requires more structure – agreement on what is needed and on what design choices are taken as given, for the time being. This agreement creates a shared imagination game in which these design elements are accepted and built on by inferring what they require, prohibit and make possible, even though the participants know they are provisional. While individual designers’ ideas can be fluid, the collective game is not: it is fixed and then periodically updated.
3.2 Props in design
The point of designing is that it is the construction of representations of objects that have not yet been brought into being or may never be. In doing so, the designers generate and use a number of props, primarily objects, models, requirements, and documentation of properties and performance. A prop is anything that serves to specify the form of the new design.
Objects. Design is rarely carried out from scratch and most complex designs are based on similar previous designs (see Eckert, Stacey & Earl Reference Eckert, Stacey, Earl, Gero and Bonnardel2005). Jet engine companies have been building jet engines for many decades. In the case of the original equipment manufacturer (OEM) in the example, they have been doing this since the invention of jet engines. They draw on a rich body of experience from past engines. They select an engine of a similar size as a starting point and typically adopt innovative solutions from the previous engines that they have developed.
These reference designs provide valuable knowledge about the behaviour of the new design through the results of the design analysis and importantly through performance data in action. Shared knowledge of past designs also provides a common frame of reference for design discussion. A statement like ‘should we have the same configuration as in the [reference engine]’ makes perfect sense to those who know this design, but is totally impenetrable to those who do not. The new design inherits properties from the design being used as a reference point that are not spelled out, and problems might only emerge when parts are integrated.
In the case of engine components, past designs can act as physical props. Designers carry components with them and use them to illustrate points that they are trying to make. As the example illustrates, the performance of the design depends on intricate geometry, which can be difficult to describe verbally, while being communicated instantly by reference to the physical object.
However, objects that are referred to do not need to be physically present. It is often enough to refer to them by name. For example, engine designers refer to competitors’ engines to make specific points about system architecture.
The object also does not need to be of the same kind. In many fields, designers draw on ideas very widely and use them as sources of inspiration or to frame the space of possible designs in discussion (see Eckert et al. Reference Eckert, Stacey, Earl, Gero and Bonnardel2005; Stacey, Eckert & Earl Reference Stacey, Eckert, Earl, McDonnell and Lloyd2009). This might be less the case for jet engine components, where the problems are tightly constrained and plausible solutions have precedents. However, jet engine designers look at other industry sectors for materials or manufacturing processes.
Models. Designing proceeds through the construction of models of non-existent artefacts (see Visser Reference Visser2006a,Reference Visserb) to the final realisation of a product. These models, along with less coherent descriptions of aspects of the design, define the current state of the design, and so what can be added to the design to make it more complete, without retracting previous decisions.
Requirements. In nearly all technical designing, specifications of the requirements the design needs to meet play an important role. By stating what the design must do or be like, they contribute to determining what the designer is or is not allowed to imagine within the rules of the games of make-believe designers play. However, often a lot of what is required is implicit in the designers’ understanding of the game they need to play, and thus part of its principles of generation, rather than explicitly specified in the requirement documentation.
Documentation. Designers do not just construct models; they also produce documentation for others or their future selves specifying the design, recording results of tests and simulations, explaining the design process and documenting their activities. In the aerospace industry, much documentation is mandatory and will therefore be produced according to requirements governing content and format. However, not all the information that exists and is useful is ever recorded. Convincing designers to document their rationales for decisions has been notoriously difficult.
3.3 The network of models
In engineering design, it is critical for the designers to understand and to be able to predict all aspects of the product’s behaviour and properties under all circumstances before they commit to the product entering the market. Although capturing absolutely all aspects of a product might be an unobtainable goal, engineers need to try to be complete within reason. Engineers create many different models for different aspects of the design. Many of these models are in defined relationships to other models that they are used to generate, which makes different aspects of the design explicit and fill in details, so the models comprise a structured network where the different models constrain each other’s interpretations. Much of the time, the individual models or documents are not complete in themselves, requiring only background knowledge to interpret them, but have meaning assigned to them by the designer in the context of other props and the state of the design itself and the development process. Similarly, physical objects sometimes function as symbols in design discourse, serving to indicate features of the new design; their intended meanings depend on the context. The relationships between these various props, connecting the information they convey about the new design, are sometimes communicated in words or by reference to other objects but are often implied.
Although each of the models separately is an abstraction that only shows a selected aspect of the product, leaving other aspects unspecified or uncertain, the network of models collectively needs to cover all aspects of the final product precisely enough to manufacture it and predict its behaviour before the company can be confident to release the product into the market.
In the earlier stages of the development process, when known constraints and hard decisions underspecify the design, aspects of the design (whether structural elements or performance characteristics) need to be conjectured and proposed as well as inferred from previous decisions. These aspects, expressed in models and other documentation, vary in how firm or provisional the decisions are, how precise shapes and other parameter values are, or whether the design features are exact or rough or merely placeholders for qualitative categories (see Stacey & Eckert Reference Stacey and Eckert2003). Many of these models appear to be – and formally are – exact specifications; the uncertainty associated with a model or group of models is often extrinsic to the model, implied by other models or specifications, or contained in the understanding of individuals. Through the development process, the uncertainty diminishes as design decisions are first made provisionally and then become firm and exact, and the design the models specify collectively becomes closer to the final product. The example described in Section 4 illustrates this process of convergence to a final product, and how we can see it as a series of games of make-believe.
3.4 The principles of generation of the game
For us to make sense of a fictional world, we require an implicit understanding of the principles of generation of the game that is related to the work of fiction. We must pick up, for example, on the clues that the author gives us about the characters and the plot. These are rarely spelled out, but often implied by details. Therefore, we must imagine this implied world in which the story unfolds. Usually we make the assumption that the characters and events in the story are bound by the laws of nature and the events of the time they are set in, unless something else is powerfully flagged up by the author (see the discussion of the Reality Principle and the Mutual Belief Principle in Section 2.1).
The principles of generation involved in understanding models encompass all the cognitive and physical resources that can be used to draw inferences from the props. Mapping out the rules is made problematic by the situatedness of much procedural knowledge, in engineering as in all other fields (see Clancey Reference Clancey1997). A variety of types of knowledge are involved in problem solving in general and engineering in particular (see Vincenti Reference Vincenti1993; Kant & Kerr Reference Kant and Kerr2018), but unpacking them is unnecessary for the argument of this paper. We can draw a conceptual distinction between declarative factual knowledge and the procedural reasoning knowledge used to make inferences from it, but making this distinction is difficult in practice as much of our knowledge of how to act and solve problems is tightly bound to specific situations.
Being a competent member of the designers’ community of practice involves possessing shared sets of concepts as well as knowledge of how to find and use pertinent general information, and knowledge of how to read diagrams of models and make inferences about the form and behaviour of the design – what Bucciarelli (Reference Bucciarelli1994) terms an object world. As well as laws of nature, properties of materials, principles of mechanics, and the like, principles of generation in engineering include exogenous factors, like regulatory frameworks that the company cannot influence; and endogenous factors like constraints, manufacturing parameters or time scales, which are not props specifying the design but are pertinent to the design. Considering the context in which design takes place and picking up on clues is also an important aspect of engineering interactions. This context can be defined explicitly, for example in the regulations that the engine needs to comply with, but importantly includes understanding background information that is not documented about capabilities of the companies involved.
Much of what designers know about how to design takes the form of heuristics – inference rules that are frequently useful but not guaranteed to produce correct or optimal outcomes, embodying standard solutions to common problems and best practice. This may blur the distinction between what designers are required to infer about the current state of the design (by the props and the principles of generation) and what this entails for the further development of the design.
3.5 The aims of the game
What the players of the game are supposed to achieve is not a concern for Walton’s make-believe theory; nor is it a crucial issue for its application to science. What matters is what follows from an artwork or a scientific model – what is fictionally true in the world it defines. In design, where the current set of models and other props underspecifies the product, the job of the designers is to create a more detailed and complete design within the space of what the current set of props allow – in Waltonian terms, within the limits of what can be fictionally true in the game – unless and until the time comes to try to do something else.
Designing, like other problem solving, is driven by goals and awareness of what constitutes success. In design, as in many kinds of games, the principles of generation for the make-believe games the designers play with models and other props include success criteria. Many of the success criteria are made explicit in the games, and some are written down in the props, but a lot are tacit. They are implicit and taken for granted. Explicit requirements are expressed in the props; that there will be requirements and how the designers should deal with particular kinds of requirements are tacit but crucial elements of the principles of generation.
Many theoretical accounts of designing view it as a cycle of problem formulation, synthesis, and evaluation, a perspective that is traced back to Asimow (Reference Asimow1962). To describe this in Waltonian terms: In synthesis activities, the task is to extend the design; that is, to imagine and record new propositions describing or elaborating parts of the design that are consistent with each other and with the existing props (describing both the design and the requirements), and success consists in doing this. The new propositions can then be incorporated into the props for further activities. In evaluation activities, the task is to evaluate the current state of the design; that is, to derive other propositions (for example, about performance properties) that follow from recently proposed propositions (whether or not recorded in props), and check whether the new propositions are indeed consistent with each other and the existing props. In problem formulation activities, the task is to make decisions about how to alter the props and sometimes the principles of generation governing further designing activities.
Of course, success criteria in design are socially negotiated, are often multiple and varied, and can conflict. What designers imagine, what thoughts they choose to pursue, and what provisional decisions they make – where they go in the space of possibilities allowed by the game – are influenced by their goals and preferences. Only a small part of the complex network of goals that drive large-scale design processes are shared by all the participants. Engineering design is hierarchically organised, with designers focusing on particular components and tasks. Parts of the design, and individual designers’ contributions to them, can be successful and recognised as such, whether or not the whole project delivers something or yields a successful product. The goals and success criteria that drive designing are often local or personal, but they are connected, and the sharing of goals is essential for coordinating the design process.
The goals for design tasks and their corresponding success criteria often go beyond making the current design successful. For example, jet engine companies use parallel development of solution alternatives to gain knowledge about high-risk solutions such as innovative materials with the view of using them at a later date in different engines. The teams developing these high-risk solution alternatives are well aware of this and have multiple goals from the outset.
The goals of the design process are influenced by soft requirements and preferences beyond what is explicitly documented in the props. The contextual knowledge designers employ can include awareness of the strategies and agendas that the different companies pursue. For example, the supplier might be aware that the OEM might aim for a big push in combustion temperature; or the supplier might want to push the development of a particular material because they would like to use it on other projects. While formal negotiation takes place at the end of iteration rounds when new official values are issued, the teams often know each other well and therefore can pick up on comments and interpret descriptions of each other’s activities and thereby pick up clues.
3.6 Authorised games coordinate design activities
Design is in most cases a shared process, where a range of different people have different roles in this shared process and need to work towards a shared but potentially evolving goal. They all need to use models and other props to understand the current state of the design and how it should develop so that the different contributions they make fit together. Drawing inferences from shared props both depends on and helps to create shared goals and shared frames of reference. In our illustrative example of jet engine component design – discussed in the next section – all the engineers share common models of the overall system architecture, but individual teams also develop their own models to generate or evaluate particular designs; for instance, a team concerned with a cooled component design has models of the airflow within the component, which is not relevant for a non-cooled alternative design.
While models serve a range of purposes in designing, one crucial role is enabling the participants to share an understanding of aspects of the design. In large-scale design projects, people separated in space and time communicate by giving each other documents, diagrams, CAD models and sometimes other props (see for instance Henderson Reference Henderson1998; Eckert & Stacey Reference Eckert and Stacey2000). In meetings, designers often communicate with a mixture of talking (including references to props), sketching and gesturing, where each of these is unintelligible without the others (see Tang Reference Tang1991; Harrison & Minneman Reference Harrison, Minneman, Cross, Christiaans and Dorst1996). As with reading novels and using scientific models to reason about the nature of reality, this communication through props involves sharing principles of generation for drawing inferences from the props to generate shared beliefs about their implications.
However, situations where competent players of the make-believe games employ different principles of generation and make different inferences are both normal and important in engineering design. The models often serve as boundary objects (Star & Griesemer Reference Star and Griesemer1989; see for instance Carlile Reference Carlile2002; Subrahmanian et al. Reference Subrahmanian, Monarch, Konda, Granger, Milliken and Westerberg2003), mediating communication between colleagues with different expertise, conceptual frameworks and concerns – different object worlds (Bucciarelli Reference Bucciarelli1994) – who are responsible for different aspects of the design. Their shared understanding does not need to be complete – perhaps it cannot be complete – but there needs to be a degree of alignment of interpretations to ensure that different designers do not make incompatible inferences. In Waltonian terms, that they do not actively disagree on what is fictionally true within the game.
There is a danger that props can be over-interpreted as specifying aspects of the design more exactly than intended, or possibly under-interpreted as specifying the design more loosely, by designers importing assumptions about what the design is and how it will develop that are not warranted either by the props or by the knowledge of the community of practice that constitutes the principles of generation. We view this as a failure of the Shared Mythology Principle. Moreover, designers can suffer from what psychologists call fixation, the inability to free themselves from past ideas even if they are explicitly told to do so (see Jansson & Smith Reference Jansson and Smith1991; Purcell & Gero Reference Purcell and Gero1996; Crilly Reference Crilly2015).
Developing the design further involves making tentative decisions, choosing to accept them as given for the time being, exploring and discarding alternatives and analysing their implications, before new decisions are accepted and contribute to the props. Decisions narrow the space of possible design options and therefore reduce the uncertainty for the design teams. While designers can explore any ideas they want, collaboration with their colleagues requires them to know what is and is not within the scope of agreed possibilities, and to be clear about when they are stepping beyond those boundaries; and most of the time, they need to stay within them to make useful progress.
For different designers’ beliefs about the state of the design to remain in alignment, it is essential that the games of make-believe designers play when interpreting the state of the design are authorised games, in that the props embodying the current set of decisions and assumptions, and thus the specification of what is and what is possible, are agreed, and modified only in a controlled manner. We will come back to this in Section 5.
4 Engineering as a game of make-believe: jet engine design as an illustrative example
Complex products, like cars, aeroplanes or engines are typically designed by hundreds or even thousands of people organised into teams, sometimes working for different companies and coming from different disciplinary backgrounds. Complex engineering products are usually designed incrementally based on previous versions of the products (Eckert et al. Reference Eckert, Clarkson and Zanker2004). They are typically overconstrained, by the predecessor design as well the requirements arising from their own business as well as customers (Stacey & Eckert Reference Stacey and Eckert2010). Developing this type of product like simpler consumer products is subject to endogenous uncertainties, like changing consumer requirements and regulations, but also considerable uncertainty arising from the interaction of different teams (Yassine & Braha Reference Yassine and Braha2003). In large-scale engineering projects, different teams often work in parallel with assumptions that need to be revised as different parts of the design converge towards something feasible, supposing at each point that the current assumptions and parameter values are correct while knowing that they are likely not. For example, design is often carried out in parallel with testing so that designs are tested that have already been modified by other teams. This mutual information dependency can lead to costly iteration (Wynn & Eckert Reference Wynn and Eckert2017) and to what is called churn, i.e., unproductive work by some teams while others advance the design (Yassine et al. Reference Yassine, Joglekar, Braha, Eppinger and Whitney2003). Companies try to handle this by mapping information dependencies (Browning Reference Browning2001) and taking a set-based approach for aspects of the problem that can be clearly parameterised, where both the requirements and the solution spaces are kept flexible to avoid distinct rounds of iteration (Sobek, Ward & Liker Reference Sobek, Ward and Liker1999). Although these approaches were proposed 20 years ago, they are still not widely used and require a considerable understanding of the problem and the potential solution before they can be used.
This section discusses designing components of jet engines at a second-tier aerospace supplier in terms of games of make-believe. This example draws on research on how design margins (the amounts by which design parameters exceed their requirements) can drive the dynamics of design iteration, carried out by one of the authors of this paper with a co-author who has worked for many years as a jet engine engineer; the research included interviews with a number of working engineers on margins in jet engine design (Eckert et al. Reference Eckert, Isaksson and Earl2014, Reference Eckert, Isaksson and Earl2019). It is therefore an illustrative reinterpretation of an existing case study. This is a complex and realistic illustration of how designers of complex products work during an iteration round with the values of parameters that they agreed on, even though they are aware that these will be changed, because they see the benefit of the learning and insights they gain by doing so. For example, we observed in an empirical study of diesel engine design that companies run expensive physical tests on versions of a design that have been superseded to learn more about what can be achieved reliably with that design (Tahera et al. Reference Tahera, Earl and Eckert2017). This learning is a means for them to handle and reduce uncertainty about the performance of the eventual final design. In Waltonian terms, this is using the inferences made by playing one version of the game to develop more precise situation-specific principles of generation for playing later versions of the game with different parameter values specified by different props – revised documents or CAD models.
Jet engines are highly complex products that involve the collaboration of hundreds of engineers across multi-tier supply chains. Typically, the engine OEM, such as Rolls Royce, General Electric or Pratt & Whitney, that sits at the top of the supply chain, defines the system architecture and controls the main combustion process. Their suppliers are specialised in developing specific components, in the case of this example the non-rotary components.
The efficiency of a jet engine depends on the combustion temperature: the higher the temperature in the combustion chamber the more efficiently the jet engine can operate. So, a task for designers is to develop a feasible solution to the problem of maximising the combustion temperature of a jet engine. The components have to withstand high temperatures in order to increase the efficiency of the engine. However, very few materials can operate reliably under these temperatures. The melting point of a particular metal may be the ultimate upper bound of the temperature that the engine is designed to. Those materials that exist and can withstand high temperature are expensive, and many of those materials are relatively new developments that have not been tested in action. The geometry of the combustion chamber also has a vital influence on the temperature the non-rotary components are exposed to. The main parameters of the geometry are the angles and distances between different elements. Designers at the OEM aim to optimise these parameters in order to maximise the resulting combustion temperature. If the component does not withstand a particular combustion temperature, it is possible to cool the components with air or fuel. As these parameters are refined through the design process, they remain uncertain for others who have to base their decisions on them. These changes in the values of the parameters can be considerable. The engineers issue updated analysis models through the design process, which then constitute new props.
In jet engine design, the emphasis lies on meticulous analysis of small changes to existing designs. The modified designs are expressed in a range of models and later physical prototypes that are analysed through multiple calculations, numerical approximations, simulations and physical tests. To carry out these analyses, the engineers need to work with assumed values and geometrical configurations. They possess a lot of specialist knowledge of physics, material properties, possible solution principles with their advantages and disadvantages, available components, and so on, which constitute the principles of generation of their make-believe games; changes in these parameter values are what distinguish different versions of the game of creating designs for particular jet engine components.
The team designing the combustion chamber at the OEM and the teams designing the non-rotary components work in parallel. As it is difficult to predict which temperature can be achieved at the beginning of the design process, each team starts with an assumed combustion temperature issued by the OEM and some key geometric parameters. Before the design analysis work has been carried out, the combustion temperature is hard to predict. It might need to be adjusted down, if an ambitious target cannot be achieved, which means that the supplier might use an unnecessarily expensive design option. It might also be adjusted up if it turns out to be higher than predicted, which might mean that the supplier needs to adopt another solution. In any case, the suppliers have to make an estimate of the temperature that will be achieved, beforehand, and then they have to work towards a design in light of this estimated temperature.
While the combustion teams at the OEM work on the details of the combustion, the suppliers design components to the estimated final temperature. They might have multiple teams working in parallel on different solutions that could meet different combustion temperatures, at different costs, which have different levels of maturity. For example, the first team works with an established material that meets the assumed temperature. The second one works with an experimental material that gives them a buffer for a potential temperature increase but would require high testing costs. And the third one looks at an air-cooled solution that can withstand a higher temperature but is more expensive to manufacture. Developing solutions in parallel is a common strategy for such design tasks and it is an effective means of learning about innovative solutions and increasing their maturity level. Learning as much as they can about a new material or geometry is very important to aerospace companies; this is one reason why the companies often carry on working on design solutions they are fairly sure they will not deploy.
For safety reasons, aerospace companies aim to deploy tried and tested technology, which has been successfully tested in flight. The trustworthiness of the technology, and therefore its maturity or readiness for use, is measured in terms of technology readiness levels (Mankins Reference Mankins1995). What the principles of generation are differs according to what the maturity of the technology is. Tacit assumptions about how to develop the design can belong to the principles of generation as well as agreements about what is acceptable. These agreements can be spoken ones that are not written down, or come from documentation that exists elsewhere, or can be tacit and never articulated.
During the design process, the teams may communicate with other teams in the organisation and across the supply chain. Yet, each team keeps mainly working on its own on its design. The OEM issues updates on the predicted combustion temperature. The supplier assesses whether their preferred design options can meet the new requirements and enters into negotiations with the OEM if this poses a significant problem or increases the cost or risk associated with the design. This might exclude some of the solution alternatives or stop some that are no longer considered necessary to pursue.
In the next round of iteration, the geometry may have to be amended, and clearer estimates of the temperature and airflow are emerging. The teams work individually on their expanded solutions and, after a while, they meet again. Typically, there will be several of these iteration cycles until the supplier company settles on one solution and starts to optimise it. This form of convergent iteration (Wynn & Eckert Reference Wynn and Eckert2017) can go through multiple rounds, and run the risk of so-called churn, where a team works on modifying a solution without achieving a practical improvement.
Jet engine design is in some ways an extreme case of design, because it has well established classes of solutions, which can be fully described with values for a fixed set of parameters. Uncertainty arises from three main sources:
(i) The science of high-temperature physics and material science as established solution principles are pushed to their limits with new materials.
(ii) The changing requirements from the end customers; for example, around 2000, with the introduction of on-board entertainment systems, the amount of electrical power required by aircrafts increased dramatically so that it affected both the configuration of jet engines and the power for thrust they could provide.
(iii) The activities of other teams and their ability to meet or exceed targets.
Both the case study company and the OEM are beginning to explore the use of set-based design to develop generic designs for a class of engines, but a lot of work needs to be done in order to make it feasible.
We can think of each of the parallel designs as a separate game of make-believe, where each team pushes their design as far as they can and in as much detail as they can to learn as much as possible about the solution they are pursuing. The engineers know and accept that their work might not directly contribute to the final product so that the propositions they are imagining remain fictional propositions that are fictionally true but not literally true. They understand the value of the collective learning that can be gained from engaging in the game. For example, if a candidate material shows up a failure mode in an extreme use condition, it is valuable to know so that the company can avoid using the material in that use context in the future. Throughout the development process, the engineers generate multiple models, for instance describing the geometry, the temperature flows, and the stress patterns, typically in the form of computer models, which are passed on to other teams and also recorded to be reused by the company in the future. At present, the models are still generated in different software packages and model different aspects of the system. The engineers have to piece the models together to build up a complete picture of the engine. Often, they require feedback from others on the models that they have generated. This is an analogous case to the broom standing for a horse. There is an implicit request to ‘assume for now that the values of $X$ and
$Y$ are correct and tell me what it means for
$Y$’. Understanding can only be gained if all play the game.
As the OEMs demand changes or ask for tests, which reveal that certain options need to be changed, the games themselves change. Assumptions need to be revised and new assumptions need to be made. The game needs to change.
Compared with other branches of engineering, jet engine design is at the formal extreme, largely comprising problems where both the requirements and the characteristics of the solutions can be defined with sets of parameters, and a large part of the work is mathematical and computational analysis of the performance of proposed designs specified in computational models. Nonetheless, jet engine designers need to apply imagination to see where potential solutions might go wrong and make sure to test for anticipated failure modes. In branches of engineering with more open-ended problems, the key challenge can be imagining what is possible or how a requirement can be met given the current set of constraints – that is, the currently agreed set of props specifying the requirements and the current set of decisions about the design.
5 Design processes as networks of authorised games
A large design project comprises a huge number of different activities, in which different people use models and descriptions of the future artefact in different ways for different purposes. Even small-scale designing by one or a handful of people involves a range of different activities.
The different design activities generate outputs that feed into new activities carried out by the same designers or by others. They constitute a highly structured and tightly connected network (see Browning & Ramasesh (Reference Browning and Ramasesh2007) for a review of the literature on models of design processes as networks of activities). Some activities might generate additional props for new make-believe games involving different sets of goals and concepts. In each sub-game, designers share common goals and common principles of generation and also make assumptions about each other’s behaviour and strategies. Sometimes planned iteration or backtracking is needed to repeat the same game – reasoning about the same goals and concepts – with different props. In our jet engine design illustration, as in many large-scale design processes, tasks depend on each other for input so that they need to be repeated with better estimates of the parameters provided by the other tasks.
So what is the game? Whether we think of a design project as a network of related games of make-believe or as one game that is progressively modified is a matter of perspective. For example, one could think of the entire design of the jet engine as one game or the activities of the different suppliers and the OEM as networks of games. We do not propose a definitive view here. However, there are two natural levels at which we can see a game as divided into sub-games or a sequence of related games. One is at the points where the project makes significant changes to the requirements or target parameter values, or to design decisions that are treated as settled. The other is the very large number of tasks that have their own distinct goals and require different kinds of thinking from the other tasks that surround them.
5.1 Changing the game
There are numerous ways in which the games of make-believe can change. We can draw a useful distinction between two classes of changes. (1) Games can be changed by extension – accepting new decisions or other information as part of the props that define the space of possibilities, thus adding to the existing props, to make the currently accepted design more complete or detailed, making the game more tightly specified, and so increasing the number and precision of the inferences that can be drawn from the props, which narrows the space of possibilities that are compatible with the props. (2) Games can be changed by modification – altering the existing props, and thus what is true within the game, and hence moving to a different space of possibilities.
Five important ways in which the game can be altered, making different propositions required to be imagined or not within the game, and shifting the space of possibilities, are these. (i) Changes to requirements. (ii) Iteration loops in convergent iteration, where new parameter values are negotiated between player representatives and then released to the wider game. (iii) Catastrophic events either internally or externally that falsify major assumptions. In extreme cases, a new insight or revelation changes a design process, for example, when a key test fails or a key parameter changes. External events can include issues like the cancellation of orders. (iv) Changes to the design in response to problems, which can sometimes have complicated knock-on effects (see Eckert et al. Reference Eckert, Clarkson and Zanker2004). Such changes are typically assessed and bundled for new releases of the props. (v) Changes to understanding, for instance about material properties, that change the principles of generation that are applied to the props.
The jet engine design process includes iteration loops in situation (ii); however, it is imaginable that surprising test results could either push it into situation (iii), for example when an unexpected failure mode occurs, or situation (v), for example if a test reveals higher melting temperatures than expected.
Changes to the degree of commitment to decisions about aspects of the design are crucially important to the process of managing uncertainty and controlling the exploration of alternatives, revision and backtracking in the course of developing a complete and settled design. However, they do not affect what follows from those decisions (and so what is fictionally true within the make-believe game that assumes those decisions).
The propositions that designers regard as following from the current state of the design (in Waltonian terms, fictional within the game), and so the spaces of possibilities that these propositions allow for the further development of the design, depend, in practice, on what inferences they are able to draw from the props. Infrequently, but sometimes importantly, conceptual breakthroughs come from rerepresenting what is already known in a different form, to make other features and relationships explicit that were previously implicit. Creating new sub-games by reframing – formulating new goals for new tasks and representing the information differently – does not change what follows from the props, and so does not change what is fictionally true within the game, but profoundly influences what the players of the game actually do imagine to be true or possible.
5.2 Authorised changes to authorised games
Walton’s make-believe theory depends on the notion of an authorised game, which cannot be altered. However, the static notion of an authorised game of make-believe put forward by Walton does not reflect the dynamic nature of design. The idea of an authorised game defining agreed limits on what ought to be imagined within the game is essential to the view of designing we present here, but we need to extend it. Walton’s perspective is to look at the problem from the viewpoint of how an existing work of fiction can be understood in a shared way by different people and at different times. In design, we are confronted with the process of creating an object. In this process, the features and properties of the design and the requirements it must meet change many times, and this requires changes to the props. However, designers know (or should know) the limits of what they can and cannot change within the scope of their current tasks, and on a higher level the changes that are allowable are themselves authorised. In practice, this is problematic, as the boundaries of what can and cannot be done within the scope of the current partial design are often unclear, and this makes engineers conservative, trying to stay within what they know is possible (see Eckert et al. Reference Eckert, Clarkson and Zanker2004).
Only certain people can authorise a change to the game. Design managers can change processes; technical experts or designers can generate aspects of the emerging product. The formality of the process of changing the game varies between design processes. In some development processes, sets of assumptions are changed at review points according to set procedures, and changes to the design need to be agreed through a formal process. In engineering, the word ‘change’ refers to altering something that was considered to be finished, and typically needs to be authorised by a change board (Jarratt et al. Reference Jarratt, Eckert, Caldwell and Clarkson2011); this constitutes changing the game by modification. Decisions on whether a change is or is not carried out are usually made by change boards, which meet periodically and bundle changes so that a whole set of change requests are released at once. In the jet engine design example, the change board authorising change requests is one means of changing authorised games. Suppliers of components get releases of new requirements or performance parameters from the OEM; conversely, OEMs may be told by suppliers that performance parameters are impossible or that particular requirements need to be met. The example company, like most other large engineering companies, employs a stage gate process with periodic reviews of the design during project gateways, where updates are decided on or in extreme cases projects are cancelled.
However, hierarchy is not enough to explain all the changes. Facts also change the game. For example, newly emerging properties of the product that are discovered might require a change to the design and often a divergent interpretation of the models. All members of a design team have a duty to alert their colleagues to anticipated problems; if there is agreement about the problem, again the game is changed.
5.3 Repeating parts of the game: iteration loops
We can think of the overall jet engine design process as one game of make-believe. The laws of physics, like the combustion temperature that the designers have to adhere to, or the regulations the engine must meet, can be thought of as principles of generation. The requirement specification and the various models can be thought of as the props, as well as the objects the designers refer to and the other documentation they employ.
As we have described, parts of the jet engine design process need to be repeated with different assumptions and target parameters. We propose to think of each of the iteration loops in these convergent iteration cycles as part of an overarching game of make-believe in which the authorised game is amended. The specification of materials, the assumed temperature and the geometry comprise the main props of the overarching engine design game. Everybody works towards the given target parameters, which might or might not be achieved by the engine. At the decision point that starts a new iteration, a decision is taken on which principles have to be changed and how to play the new game. For example, the teams could decide together that designing the combustion chamber so that the temperature is lower might be better than redesigning the surrounding components.
The iteration loop with modified props forms new versions of the game that are interconnected by shared principles of generation and by shared props. We can think of different teams pursuing alternative solutions with different requirements as playing parallel games.
5.4 Dissolving games of make-believe in physical reality
Unlike literary books and other works of fiction, most design processes converge towards a final product that is made, which has measurable and perceivable properties. The games of make-believe get closer to reality as both the games and reality change. At the end of the design process of a jet engine component, there is a physical component. It has the properties that the models predict unless the engineers have overlooked some important aspect of the design problem. In cases where reality does not correspond to the designers’ predictions, designers often blame wrong assumptions in their use scenarios.
At the end of the collective design process, once the product has actually been manufactured, the outcome is not ‘fiction’ but ‘reality’. The eventual description of the product and the prediction of its behaviour that the design process arrives at are accurate with regard to the final product and its behaviour unless the company has made a mistake. At this point, one can view the design as an accurate specification of the product (subject to the variability of manufacturing), no longer requiring make-believe.
The change in the games of make-believe through time and the production of an actual artefact alter both the relationship between models and descriptions of the product and their target system, and the nature of the reasoning about them. Neither change is as abrupt as it appears at first glance.
The game of make-believe moves towards the specification of a design through a series of decisions. Through the development process, the uncertainty and the space of alternative possibilities diminish as design decisions are first made provisionally and then become firm and exact. Elements of the design are frozen, and then regarded as certain. This increasingly turns ill-defined problems to be explored through if-it-were-the-case make-believe into well-defined problems that can be solved. Thus the design the models specify collectively becomes closer to the final product. At the same time, the design is prototyped and tested and models are validated by external evidence that the performance properties they predict are correct (see Tahera et al. Reference Tahera, Wynn, Earl and Eckert2018). As the inferences from the props, to which one has the attitude of make-believe, become more exact and complete, the corresponding but separate attitude of belief that the product matches the props becomes stronger. Ultimately, the props become expressions of reliable knowledge about the product.
In practice, we are not entirely done with games of make-believe at the end of the design process. Engineers still make use of models to understand the product. Reasoning about how the product should behave if a model were accurate still has the character of make-believe, but when belief in the accuracy of the model is solidly grounded the propositions about the final product should not only be imagined but also believed. Due to the complexity of most engineering products, engineers can only look at parts of a whole system. Therefore, they need to make assumptions about parts of the system they are not associated with; in particular, they assume that these parts are working as specified, taking on trust that the work of their colleagues is correct.
A similar but alternative take is delivered by C–K Theory. Here the focus is on the truth values of the statements at hand. According to C–K Theory (Hatchuel & Weil Reference Hatchuel and Weil2009; Hatchuel, Weil & Masson Reference Hatchuel, Weil and Masson2013), the end of the design process is when the definition of the design has transitioned from Concept Space, where propositions do not have truth values, to Knowledge Space, where propositions are established and do have truth values.
6 Discussion
This paper presents a new lens for thinking about how designers design – one that helps us understand how they deal with assumptions and uncertainties. Our purpose is to make sense of what designers are doing, at a more fundamental level than cognitive analyses of how they think. Working out what models are and what they do in design processes, and what the components of design problems are, concerns philosophers and cognitive scientists far more than working engineers, but these issues matter if we want to understand what is really happening in designing.
The paper employs and adapts Walton’s (Reference Walton1990) make-believe theory to argue that designers participate in games of make-believe, imagining what would be the case if certain propositions were true. This enables them to make effective use of models and other design information (the props, according to make-believe theory) that they know are too simple, or unreliable, or outright wrong; to cope with complexity by making simplifying assumptions and using placeholders for future decisions; and to cope with uncertainty by treating suggestions and provisional decisions as certain (for the time being) while retaining the awareness that they are not. It enables them to treat assumptions, estimates and provisional decisions in the same way as certain and accurate information when thinking about the design, without losing track of the uncertain and shifting epistemological status of the different kinds of assertions they work with, as they are proposed or calculated, retracted, or confirmed and committed to.
Participating in games of make-believe with agreed rules, in the form of provisionally agreed sets of requirements, constraints and previous decisions, enables designers to communicate and cooperate efficiently with each other (most of the time) about design information whose accuracy, certainty and epistemological status is unclear and changing. It also helps explain how a team can work together without requiring a very high degree of shared understanding or shared goals (beyond the overall goal of the project).
Drawing on make-believe theory to look at designing in these terms forces us to modify one of the theory’s key concepts, the idea of an authorised game of make-believe, where what is to be imagined to be true within the game is fixed and mandated by one or more props that need to be accepted as given. We need to stretch the idea of an authorised game to deal with changing props as the design is developed and sometimes revised, and sometimes with changing principles of generation, when the designers’ background knowledge is revised to accommodate new techniques or new scientific information. Achieving agreement and acceptance of which design decisions are firmly accepted, provisionally accepted or treated as negotiable is an essential element of the active management and the dynamic self-organisation of collaborative design processes (see Minneman Reference Minneman1991). Observation of design practice shows that the rigid notion of ‘authorised’ that fits the interpretation of completed literary works in Walton’s make-believe theory does not cover all aspects of design. Because of that, we propose to extend the notion of authorisation from authorised games of make-believe to include authorised changes to such games. We also go beyond Walton’s treatment of the interpretation of representational artworks by looking again at the notion of game, and what the game is. Walton’s games of make-believe do not have objectives. But many games involve winning or losing, or at least doing well or less well according to some criteria for success; the make-believe games in designing are directed at achieving goals and are judged by success criteria.
Complex design processes seem to be open to many solutions, but there are constraints. Shared understanding and awareness of the limits of possibilities are crucial to effective designing, and in complex design processes, the limits are actively managed. By applying the idea of make-believe games to designing, we are able to explain why engineering processes do not end up in chaos by describing how the active management of possibilities works. The proposed lens supports a convincing story about how engineers are able to handle problems that look overpowering at first glance, without being troubled by the slippery and changing ontological status of the emerging design or by the complicated epistemological relationships between the design and the skeletal, provisional and uncertain information the designers possess about it. This makes clear that designers do not act irrationally. If what the designers did were really irrational and conceptually incoherent, it would not work.
A question that we have left aside is the authorship of designed artefacts, in contrast to the predefined authorship of the representational artworks that define Walton’s games of make-believe. Although engineering design is almost always collective, defining authorship in terms of responsibility for particular authorised changes to the games of make-believe ‘should’ be unproblematic; we would caution that in practice the origins of particular design choices are often cloudy and not easily traced to identifiable decision points. Another question we have left aside is the relationship between the make-believe view of designing and how engineers themselves view what they do. The methodology of ethnography adopts the principle that the key validation for accounts of social activities is that the ethnographers’ accounts should ring true to the participants themselves (see Hammersley & Atkinson Reference Hammersley and Atkinson2007). The engineers with whom we have discussed our application of make-believe theory to design have liked it.
Our extension of make-believe theory to design offers a way to connect a philosophical approach to understanding what models are and what they do in design, to a cognitive view of how designers use provisional and uncertain information to imagine a design, to a sociological analysis of how teams of designers socially negotiate the parameters of their shared designing activities. Mapping this out in detail is an area for further research.
Acknowledgments
Claudia Eckert and Martin Stacey were visiting researchers at ITAS, Karlsruhe Institute of Technology, in summer 2017. Our work has benefited from discussions of jet engine design and many other aspects of engineering with Ola Isaksson of Chalmers University of Technology. Michael Poznic gained from discussions of this work at the Forum on Philosophy, Engineering and Technology held at the University of Maryland in 2018. We wish to thank the Design Science reviewers for comments that were insightful, challenging and constructive.