1 Introduction
Complex societal problems that underlie challenges such as sustainability call for solutions that are entangled in a manifold of social and technological processes. The processes involved in these problem situations profoundly influence each other in a network of institutions, organisations, phenomena, and stakeholders (DeTombe Reference DeTombe2015a ). In addition, the complexity of societal problems may be dependent on an observer’s knowledge and capacity to act (Murthy Reference Murthy2000). To handle societal problems more effectively, problem solvers from different disciplinary domains have rethought how to employ scientific methods for studying and responding to complexity (Murthy Reference Murthy2000; Espinosa, Harnden & Walker Reference Espinosa, Harnden and Walker2008; Stjepandić, Wognum & Verhagen Reference Stjepandić, Wognum, Verhagen, Stjepandić, Wognum and Verhagen2015). Despite these efforts, the literature shows a significant gap between the complexity of societal problems and problem solvers’ capacity to understand and deal with them (DeTombe Reference DeTombe, Gass and Fu2013). Such a knowledge gap cannot be overcome without a paradigm shift in how we understand and address such issues (Espinosa et al. Reference Espinosa, Harnden and Walker2008).
The science of complexity has been studied in different scientific fields, including the natural, social, and systems sciences. Especially in the social and systems Sciences, the understanding of complexity and problem solving has been consistently linked to social processes. Scholars in these fields have developed and employed a transdisciplinary research approach to address complex, multistakeholder, real-world problems (see Gaziulusoy & Boyle Reference Gaziulusoy and Boyle2013; Jones Reference Jones and Metcalf2014; Gaziulusoy Reference Gaziulusoy2015). This approach, called systems thinking, is underpinned by three central claims. First, systems thinking is a problem-solving approach capable of handling the inherent complexity of societal problems (Ackoff Reference Ackoff1974; Espinosa et al. Reference Espinosa, Harnden and Walker2008; DeTombe Reference DeTombe2015a ,Reference DeTombe b ). Second, it allows designers to adopt a holistic perspective through a specific set of assumptions, premises, and axioms (Clegg Reference Clegg2000; Cardenas et al. Reference Cardenas2010; Blizzard & Klotz Reference Blizzard and Klotz2012; Forlizzi Reference Forlizzi2012; Jones Reference Jones and Metcalf2014). Third, systems thinking has the potential to incorporate differing world views (Jackson & Keys Reference Jackson and Keys1984; Daellenbach Reference Daellenbach2001; Jackson Reference Jackson2003).
In addition, the failure to address societal problems, such as those underlying sustainability challenges, has led problem solvers to express interest in adopting systems thinking in the context of design (Sevaldson, Hensel & Frostell Reference Sevaldson, Hensel, Frostell, Ceschin, Vezzoli and Zhang2010). However, in the design field few contributions touch upon systems thinking and transitions for sustainability (Gaziulusoy Reference Gaziulusoy2015), even though the scope of design has shifted over time from the development of physical objects, to integrated product–services, to complex systems (Joore & Brezet Reference Joore and Brezet2015; Ceschin & Gaziulusoy Reference Ceschin and Gaziulusoy2016). A potential reason for such relative underperformance might be a lack of pragmatism (Lilienfeld Reference Lilienfeld1985). Therefore, some authors have called for integrating systems thinking with design practice (Jones Reference Jones and Metcalf2014; Sevaldson Reference Sevaldson2014). In fact, decades ago systems thinkers like Russell Ackoff (see Ackoff Reference Ackoff1993) and Bela H. Banathy (see Banathy Reference Banathy1996) openly discussed the purposeful design of human social systems and the capacity of problem solvers to empower individuals, groups, and organisations to take part in the design of the system in which they live and work (Metcalf Reference Metcalf2014). In more contemporary systems thinking these discussions have been followed through, and design now sits at the core of the emerging concept of systems methodology (Gharajedaghi Reference Gharajedaghi2011).
The relationship between systems thinking and design can also be observed in recent transdisciplinary research, which points towards the formulation of a systems-oriented design practice, which will be referred to here as a systems design approach (see Charnley, Lemon & Evans Reference Charnley, Lemon and Evans2011; Sevaldson Reference Sevaldson2011; Nelson & Stolterman Reference Nelson and Stolterman2012; Jones Reference Jones and Metcalf2014). Such existing and still evolving approaches integrate systems thinking and design competencies to handle complex systems. Moreover, they aim to deal with problem situations characterised by complexity, uniqueness, value conflict, and ambiguity over objectives and goals (Ryan Reference Ryan2014). Finally, they differ from traditional design approaches in terms of scale, societal complexity, and integration (Jones Reference Jones and Metcalf2014).
In this paper, we aim to contribute to a systems design approach by providing an overview of the developments that are leading to such an approach. We will present an extensive review of systems thinking, drawing insights from a broad body of literature. We will then attempt to gain a better understanding of complex societal problems in the light of systems thinking (as addressed by different systems approaches), and we will relate systems thinking to design in a conceptual framework for a systems design approach. The study builds on the assumption that integrating systems thinking into design is a promising approach for tackling complex societal problems. It further builds on the position that design has the capacity to create holistic solutions to problems, and can potentially develop both fields (Nelson Reference Nelson2008a ; Sevaldson Reference Sevaldson2014). With respect to this last point, an additional and more tentative aim of this paper is to provide an initial exploration of a systems-oriented thinking foundation for design.
2 Literature review
To ensure scientific relevance, we collected relevant publications from multiple disciplinary and interdisciplinary domains. The central questions which guide this literature review are: ‘What are complex societal problems?’; ‘How has systems thinking been developed as a way of handling complex societal problems?’; and ‘To what extent does systems thinking provide the best fit to the design of solutions aimed at complex societal problems?’.
To further integrate insights from a broad body of literature we used a heuristic and reflective tool (based on work by Gaziulusoy & Boyle Reference Gaziulusoy and Boyle2013) to review, evaluate, and report transdisciplinary literature. Hence, the literature review is conducted through an extensive search filtered across four levels: (1) paradigm, (2) problem/solution, (3) context/scope, and (4) knowledge/skills. Paradigm level filters are used to generate criteria based on the visions, norms, and values adopted in the identified research areas. The problem/solution level supports the creation of filters for the specific concerns and solutions shared across the different disciplinary and interdisciplinary domains. The context/scope level helps to create filters that both identify and delimit the broader context of the problem. Finally, the knowledge/skills level guides the generation of filters based on the knowledge base and expertise required to address the central problem of the research.
Each filter level is applied with the support of reflective questions formulated to assist in the literature review. For instance, to apply the problem/solution filters, we reflect, among others, on the following questions: ‘Which disciplinary and interdisciplinary domains are relevant to the problem?’; and ‘What is already known about the problem?’. By reflecting on these questions, various keywords were generated and used as search terms in the literature search process. This process was applied to limit the scope of the literature review and to prioritise some literature over others (Figure 1).
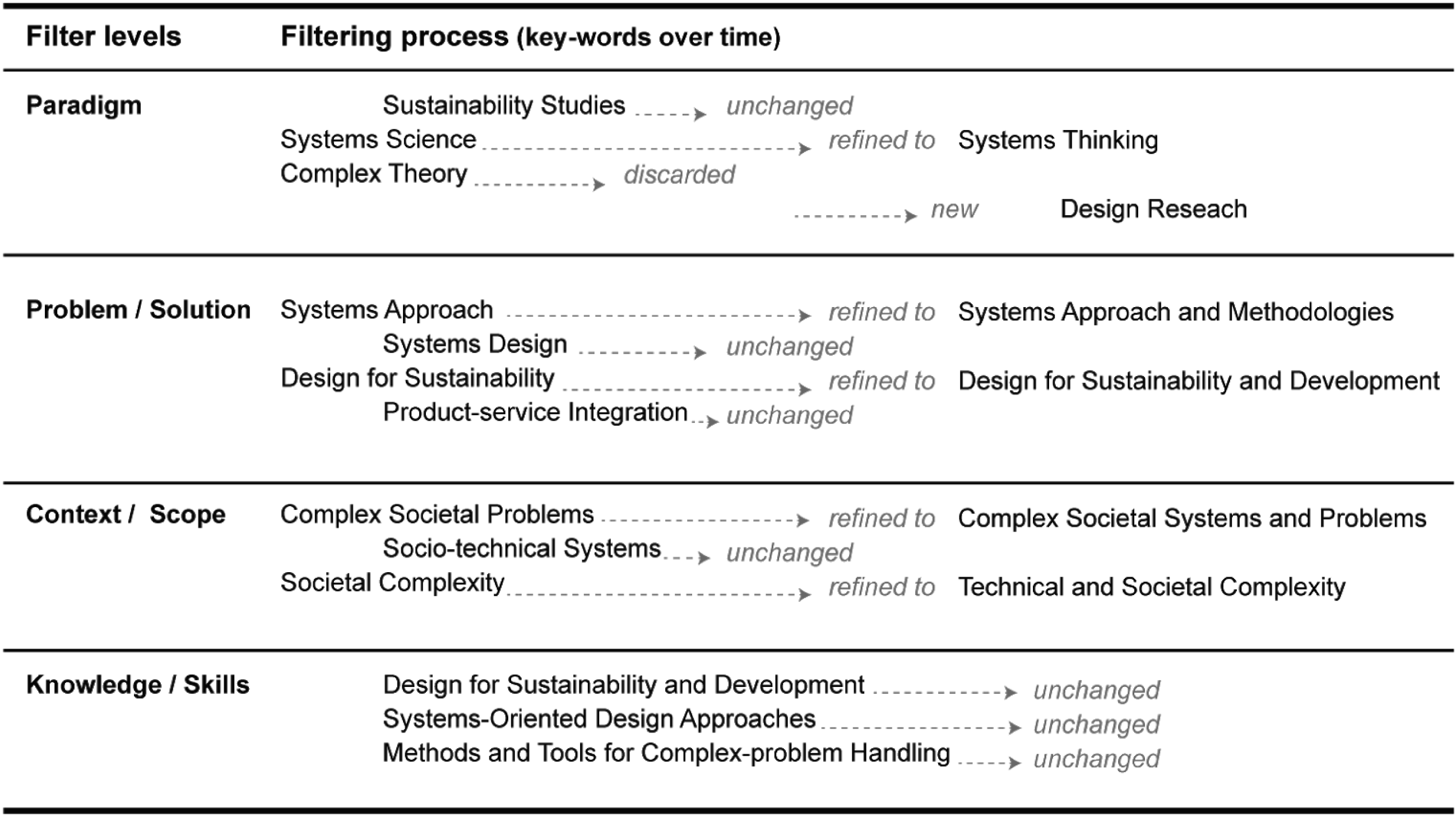
Figure 1. Literature search process. Based on Gaziulusoy & Boyle (Reference Gaziulusoy and Boyle2013).
The search strategy was conducted by first defining the search terms based on the literature review filters, and the relevant data sources and time frame. An initial (preliminary and broad) literature review was conducted using combinations of relevant search terms (e.g., system* design, system* approach*, whole system*, system* thinking, complex societal problem*) in title and keywords searches of the selected databases (ScienceDirect, Scopus, SpringerLink, Emerald, SAGE, SciELO and Google Scholar). The preliminary search selected 351 entries, which represent full peer-reviewed articles and books published in English in the leading academic journals related to the subject. Since the integration of systems thinking into design is a relatively new phenomenon, the focus of this review was, at a first step, on the literature published in or after 1987 until 2017. The preliminary search aimed to create an understanding of cross-disciplinary influences and to identify existing reviews and primary studies relevant to the literature review. Next, to conduct the main literature review, we read the title, abstract, and keywords of the collected publications. In addition, the refined literature review filters were considered (see Figure 1). This process has reduced the list of collected material to 156 publications. Finally, the relevant publications were read in full, and an additional search was conducted based on their reference lists and bibliographies in order to identify additional, relevant studies. This final process has added 20 publications to the literature review portfolio. The reference management software Mendeley DesktopFootnote 1 was used to support the inclusion and exclusion of publications.
The choices made at the different filter levels influenced the overall process of prioritising streams of literature. For instance, an important choice was made at the paradigm level, the concepts of complexity, holism, and sustainability were adopted as major values and norms to generate paradigm filters. As a result, systems theory became the focus over complex theory because it provides a better fit to the initial research intention, which was to focus on inquiry and action aimed at embracing a more radical idea of holism. While conceptual overlap exists, based on the analysis of the preliminary search, the literature review showed that complexity theory and systems theory diverge in their basic premises with which they interpret complex systems (Phelan Reference Phelan1999). The former assumes that the complexity of systems arises from the simple and specific behaviour of a system’s parts (Ibid). On the other hand, systems theory defines systems complexity as a result of the number and type of a system’s parts, as well as the interaction between parts (DeTombe Reference DeTombe2015b ). Another important choice in prioritising streams of literature happened at the problem/solution level. Concerning this, the choice was made to focus the search on problems/solutions in two areas which systems thinking was most developed: the areas of sustainability and development.
3 Uncovering the foundations of a systems design approach: selected theoretical roots
System(s) is a word that takes on distinct meanings in different contexts. In the context of design, a system can be defined as an emergent or designed network of interconnected functions that fulfil an intended unit of satisfaction (system outcome) (Jones Reference Jones and Metcalf2014). Additionally, system(s) has been described as a holistic, embodied way of thinking about reality (Nelson Reference Nelson2008a ). Accordingly, the term system(s) represents both a way of inquiry and an object of inquiry (Nelson Reference Nelson2005, Reference Nelson2008a ). In the context of this study, system(s) embodies both a way of designing and an object of design, and the primary contributions of this paper focus on systems as an approach to improve the way of designing.
3.1 Traditional scientific method
Design is a unique form of inquiry and action that aims to create and transform systems to fulfil human needs (Nelson & Vanpatter Reference Nelson and Vanpatter2004; Nelson Reference Nelson2005). Historically, designers have used scientific methods in an attempt to explain, predict, and control social, economic, and environmental transformations that take place in the real world. In general, the scientific method follows certain major steps, which have been summarised by Skyttner (Reference Skyttner2006, p. 16): reduction of complexity through analysis; development of hypotheses; design and replication of experiments; deduction of results; and finally, rejection of hypotheses. Traditionally, scientific method adopts reductionism and analytical thinking to handle problems.
Reductionism contends that explaining phenomena on one level (i.e., fundamental parts) allows the deduction of explanations from a higher level (i.e., entire system). In other words, reductionism believes that everything can be reduced, or disassembled, to its fundamental and independent parts. It provides a foundation for analytical thinking, which from the property of the fundamental parts deduces the behaviour of the whole (Skyttner Reference Skyttner2006). Analytical thinking believes that combining the explanation of the behaviour of these fundamental parts leads to an explanation of the whole. In this paper, we refer to the use of reductionism and analytical thinking to explain and investigate phenomena as the traditional scientific method. In the context of design, the use of traditional scientific method often leads to the following problem-solving process: define a problem; reduce the problem into sub-problems; find solutions for each sub-problem (sub-solutions); aggregate all sub-solutions in an overall solution that addresses the problem as a whole.
3.2 Complementing reductionism and analytical thinking
Aristotle stated that unity relates to things ‘which have several parts and in which the totality is not, as it were, a mere heap, but the whole is something besides the parts’ (Metaphysics, 1045a8–10, from www.plato.stanford.edu). Almost a century ago this idea of a transcendent existence of unitary wholes got translated by Gestalt psychologist Kurt Koffka as the now famous dictum of ‘the whole is other than the sum of the parts’ (Koffka Reference Koffka2013, p. 176). It is a principle that in the literature has come to be known under the term holism. The holistic perspective presumes a synthetic mode of thinking, which ‘[…] is more interested in putting things together rather than in tearing them apart analytically.’ (Misra Reference Misra2008, p. 14). Systems science was among the first to suggest holism as a valuable corrective to reductionism, particularly when employing traditional scientific methods to understand social phenomena (Jackson Reference Jackson2001, Reference Jackson2003; Gharajedaghi Reference Gharajedaghi2011). It has been pointed out, however, that such criticism is not wholly justified because an inquiry which starts from the analysis of the parts still considers their interdependency to the whole through some principles and axioms (Murthy Reference Murthy2000). Nevertheless, the notion of holism also implies approaching problem handling and stakeholder values using methodologies, tools, and techniques that are associated with a systems language.
While it is not the intention here to comment on the limitations of reductionism, this study contends that it is unlikely that the traditional scientific method alone can address the future consequences of present actions (e.g., sustainability issues). At best, it will prove ineffective in handling the vast majority of complex real-world problems (Taket Reference Taket1992; Sterman Reference Sterman2000; DeTombe Reference DeTombe2015a ) due to the lack and uncertainty of the knowledge needed to address the problem, and the lack of understanding of the characteristics of the system in place. At worst, the unanticipated side effects of the proposed solutions may create new problems (Sterman Reference Sterman2000), and a degree of blindness for them. For instance, a personal transportation solution intended to be environmentally friendly by offering technical improvements in energy efficiency may result in side effects, such as an increase in the number of vehicles, an increase in energy consumption, and an increase in miles travelled (see Greening, Greene & Difiglio Reference Greening, Greene and Difiglio2000). To address such a scenario, the integration of systems thinking into design approaches is proposed as a complement to the traditional use of reductionism and analytical thinking.
3.3 Systems thinking
Systems thinking as a mindset comes from systems science, which is an interdisciplinary field that studies simple to complex systems in nature and society. In the context of this study, systems thinking is an approach to problem handling that considers the parts of larger systems as intertwined components rather than independent entities. Such an approach helps to gain an understanding of the relations and interactions between the various components of a system. The adoption of systems thinking can be especially helpful in illustrating the complexity inherent in socio-technical systems through better problem definition processes and visualisations (Sevaldson Reference Sevaldson2013, Reference Sevaldson2015; Dzombak et al. Reference Dzombak2014; DeTombe Reference DeTombe2015a ); synthesising complex wholes, as opposed to breaking them into parts (Nelson Reference Nelson2005); understanding causal relationships between parts (Dzombak et al. Reference Dzombak2014); and putting forward differing world views by creating awareness of the differences in social relations (Phelan Reference Phelan1999; Daellenbach Reference Daellenbach2001; Zheng & Stahl Reference Zheng and Stahl2011). In fact, the integration of systems thinking in design theory and practice has been advocated as a promising approach to address the increasing complexity of societal problems over the years (Vanpatter & Jones Reference Vanpatter and Jones2009; Blizzard & Klotz Reference Blizzard and Klotz2012; Blizzard et al. Reference Blizzard, Klotz, Pradhan and Dukes2012; Sevaldson Reference Sevaldson2013; Jones Reference Jones and Metcalf2014).
By adopting various systems approaches and methodologies, systems thinkers have contributed to tackling complex societal problems, including those underlying sustainability challenges, by offering valuable strategies, tools, and techniques (Espinosa et al. Reference Espinosa, Harnden and Walker2008). For example, System Dynamics (SD) can help to gain a better insight into the role of stakeholders in complex decision-making processes (den Uijl & Bahlmann Reference den Uijl and Bahlmann2002). Another example is seen in the Complex Problem Analysing Method (Compram) developed by DeTombe (Reference DeTombe, Gass and Fu2013, Reference DeTombe2015a ). The Compram methodology offers a multidisciplinary method of handling complex societal problems with the collaborative involvement of policymakers and other stakeholders (DeTombe Reference DeTombe, Gass and Fu2013). The approach is based on the understanding that societal problems are ill-defined and dynamic (i.e., they change over time), and that they involve multiple stakeholders who may have different perceptions regarding the ideal solutions to the problem.
3.4 Complex problems
Researchers use the term problem to describe a situation in which the actual and future desired states diverge. According to Ackoff (Reference Ackoff1981), a problem is a dilemma that cannot be solved within the current world view. Looking at a problem situation, one can consider two distinct dimensions: problem complexity and diversity of views (Jackson & Keys Reference Jackson and Keys1984). Regarding complexity, problems can be classified as simple, complicated, or complex (Valckenaers & Van Brussel Reference Valckenaers and Van Brussel2016). This classification relies on the number and types of components and characteristics of the problem situation, and on the interactions between them. The classification also refers to how problems can be defined, described, and structured, and to how unpredictable the problem situation is likely to be (DeTombe Reference DeTombe2015a ; Valckenaers & Van Brussel Reference Valckenaers and Van Brussel2016). For example, designing a chair can be considered as a simple problem, while in comparison, designing a bus comprised of thousands of mechanical parts is relatively complicated. Both simple and complicated problems, in these examples, are easier to define than complex problems because they describe, structure, and present relatively more simple and predictable behaviours. Complex problems on the other hand, like designing a sustainable transportation system, follow more unpredictable rules because of the different nature of the components and characteristics, and number of interactions between them (Valckenaers & Van Brussel Reference Valckenaers and Van Brussel2016).
Moreover, a problem situation can result in a diversity of views, due to multiple values and interests among the involved and affected stakeholders, leading to different goals, expectations, and concerns about the problem situation. Another source of diversity can be differences in social relations in terms of power, domination, and alienation (Daellenbach Reference Daellenbach2001), as existing between individuals and groups in different hierarchical, economic, and political positions. Two major types of complexity are considered here: technical complexity and societal complexity. Technical complexity concerns the physical nature of a problem situation. This kind of complexity often arises in technical systems where boundaries are relatively well-defined, well-described, and well-structured, with little diversity in the views of involved stakeholders. The components of systems with high levels of technical complexity often encompass materials, products, machines, and constructed facilities. For example, product complexity comprises factors such as a higher number of functions and physical parts, which contribute to technical complexity. Furthermore, societal complexity is associated with the relationships between the stakeholders within a system. Societal complexity increases in systems where relations between humans and institutions are central to the problem situation, such as sustainability challenges in low-income markets.
3.5 Complex societal problems
In the disciplinary domain of design, little attention has been paid to handling complex problems or systems. What designers have gained in terms of expertise and understanding of complex problems, they have gained through practice rather than education (Siddiqi, Clewlow & Sussman Reference Siddiqi, Clewlow, Sussman, Gheorghe, Masera and Katina2014). In addition, handling complexity in design is often associated with technical systems with well-defined requirements, well-described starting conditions, and well-structured courses of action. Since complex societal problems also have a strong social side to them, most knowledge in design about complex problem handling has limited application. Seen in this light, the expertise of designers, which builds upon traditional design approaches, loses relevance when addressing problem situations with both technical as well as societal complexity, especially since the latter form of complexity is most important for creating successful interventions (Siddiqi et al. Reference Siddiqi, Clewlow, Sussman, Gheorghe, Masera and Katina2014; Moser & Wood Reference Moser and Wood2015). Thus, design approaches to social and societal systems should devote a great deal of attention to social processes, stakeholders relationships and their interrelation with technical factors (Metcalf Reference Metcalf2014).
Complex societal problems are real-world problems, mostly ill-defined, involving multiple stakeholders in an intertwined and dynamic network that may change over time, and that affects multiple aggregation levels of society (DeTombe Reference DeTombe2015a ). Complex societal problems are far from obvious, and solutions for those problems are far from optimal. They are often very hard to define due to limited information about specific problem situation and lack of context-specific knowledge. Nevertheless, they are problems that impact everyday life, as is the case with sustainability issues in developing countries (DeTombe Reference DeTombe, Gass and Fu2013, Reference DeTombe2015a ). For example, the complex societal problem of providing access to affordable, reliable, and sustainable energy in low-income households is hard to define, describe, and structure based on the available resources, infrastructure, and demand (see Costa Junior, Diehl & Secomandi Reference Costa Junior, Diehl and Secomandi2018).
While complex systems or problems may involve a high level of technical complexity, the term complex societal problems adopted in this paper refers to complex problems where technical complexity is entangled with societal complexity, and relations between humans and institutions create additional complexity. Moreover, the concept of complexity adopted in this paper refers to the understanding of the characteristics of the system in place, the characteristics of the problem, and the lack and uncertainty of the knowledge needed to address the problem situation. To this end, equipping future designers to deal with complexity requires further attention to emerging and new approaches to design research and practice.
4 Systems approaches
Over time, systems thinking has followed multiple systems traditions, also referred to by systems thinkers as ‘systems approaches’. A classification of systems approaches is explored, which aims to identify relevant criteria for the adoption of systems thinking into design. Accordingly, based on problem complexity and stakeholders’ diversity of views, we can classify systems thinking along three major systems approaches: Hard Systems Thinking, Soft Systems Thinking, and Critical Systems Thinking (Figure 2).

Figure 2. Systems approaches.
According to Jackson (Reference Jackson1991), systems approaches can be discussed in terms of the appropriate problem-solving approach, the social context within which related methodologies are used, and the consequences of its use. As such, the presented classification allows us to point to the underlying assumptions, the strengths and weaknesses of these different systems approaches. As we shall see, the various systems approaches have certain domains for which their application is most appropriate and effective.
4.1 Hard systems thinking
Hard systems thinking is based on the assumption that a problem situation is best addressed by optimising the performance of the system to achieve clearly defined objectives and goals (Checkland Reference Checkland1978). This approach understands systems as ‘objective’ aspects of reality, comprised of relatively hard (immutable), observable, and real objects. The understanding of systems is therefore considered largely independent of the observer and value-free (Oliga Reference Oliga1988). Hard systems methodologies generate an objective account of the system of concern (Jackson Reference Jackson2003) and aim to generate material well-being by increasing efficiency, improving productivity, and optimising performance.
Hard systems methodologies include, for example, classical (also referred to as traditional) operational research (Churchman Reference Churchman1957), systems engineering (Hall Reference Hall1962) and systems analysis (Optner Reference Optner1973). In general, each involves the use of quantitative models (e.g., spreadsheets, diagrams, and computer simulations) capable of dealing with highly complex physical relationships (Daellenbach Reference Daellenbach2001) and simulating the system’s performance under different conditions (Jackson Reference Jackson1985). These methodologies are often employed in an attempt to predict and control the behaviour of the system. Other systems methodologies question the limits of assumptions underlying hard systems while trying to pursue similar goals (i.e., to determine key aspects that lead to system viability and performance). These systems methodologies, namely: System Dynamics (Forrester Reference Forrester1971; Meadows et al. Reference Meadows1972), Organisational Cybernetics (Beer Reference Beer1972), and Complexity Theory (see Anderson Reference Anderson1999), are classified separately from hard systems thinking by some authors (e.g., Jackson Reference Jackson2003; DeTombe Reference DeTombe2015b ).
4.2 Soft systems thinking
Aware of the limitations of functionalist hard systems approaches, systems thinkers have developed systems methodologies which assume that problem situations can be socially complex in nature (Checkland Reference Checkland1978, Reference Checkland1981; Ackoff Reference Ackoff1979; Oliga Reference Oliga1988). This concern suggests that very few real-world problems manifest themselves in terms of systems with clearly defined goals and objectives. Hence, to extend the application of systems thinking to ill-defined problem situations, systems thinkers (e.g., Churchman (Reference Churchman1971), Ackoff (Reference Ackoff1972), and Checkland (Reference Checkland1981)) have developed systems methodologies which assume that problem situations have to be handled, rather than solved. Soft systems thinking adopts a ‘subjectivist’ perspective to systems thinking, in which the problem situations reflect a social world of subjective meaning and intention (Oliga Reference Oliga1988).
Soft systems methodologies (SSMs) seek to understand particular interpretations of the social world, to create shared understanding and consensus so that mutual agreement can emerge about action to be taken (Oliga Reference Oliga1988; Jackson Reference Jackson1991). Soft Systems Methodology (Checkland Reference Checkland1981), Inquiring Systems Design (Churchman Reference Churchman1971) and Social Systems Design (Gharajedaghi Reference Gharajedaghi2011) illustrate systems methodologies that put stakeholders’ values and interests at the core of their purpose. For instance, soft systems methodology (SSM) is a methodology for systems development that accounts for the social system into which a technical system must integrate (Baskerville, Pries-Heje & Venable Reference Baskerville, Pries-Heje and Venable2009).
4.3 Critical systems thinking
Critical systems thinking emerged as a response to the limitations of hard and soft systems thinking. A major shortcoming of hard systems thinking is that the decision-making process can enforce the development and implementation of an ‘ideal’ problem solution to the detriment of other opportunities (Bausch Reference Bausch2014). In addition, as Jackson (Reference Jackson1982) observes, the interpretative assumptions underlying soft systems thinking constrain the ability of SSMs to ensure a fair debate among stakeholders in many problems situations. Notably, the co-participative debate that is key to the success of soft systems thinking cannot be achieved when problem situations are dominated by coercive relationships (Jackson Reference Jackson1991).
Critical systems methodologies aim at the prevention of technical and social (political) influences in communication, which can interfere with the achievement of an open and free debate during the design and implementation of a system. For instance, Critical Systems Heuristics (Ulrich Reference Ulrich1983) provides guidelines for action in coercive problem situations, to promote open debate between those involved in the design of the system and those affected by the designed system. According to Jackson (Reference Jackson1991, p. 142), critical systems thinking ‘is about putting all the different systems approaches to work, according to their strengths and weaknesses and the social conditions prevailing’, to result in a more general emancipatory design.
5 Systems methodologies
In previous sections, we described how a classification based on complexity and social processes is useful for the assessment of the relative strengths and weaknesses of systems approaches, and the consequences of employing different systems methodologies. Hence, the systems methodology of choice depends crucially on the type of problem situation (Jackson & Keys Reference Jackson and Keys1984). When systems thinkers attempt to address a problem situation, they systematically use various systems skills, techniques, and tools, and by doing so employ a systems methodology (Jackson Reference Jackson2003). The different characteristics of systems approaches imply various forms of inquiry and action that underwrite different systems methodologies. A designed system can be developed from many points of view, which can be seen as complementary rather than competitive (Skyttner Reference Skyttner2006). Building on the analysis of the three systems approaches seen in Section 4, this section reflects on the linkages between the various systems methodologies and the field of design.
5.1 Reflections on hard systems methodologies: model and simulation
Within hard systems, the problem-solving process is closest to that traditionally used in design. Notably, problem solutions are deliberated, preferred solutions are selected, and a ‘final’ chosen solution is further developed, implemented, and evaluated (Bausch Reference Bausch2014). This approach works well to optimise results when starting conditions are known, and the problem is well-defined. On the other hand, a major drawback is the implication that a problem solution is put forward at the expense of other possibilities (Bausch Reference Bausch2014). Nevertheless, research on modularisation and customisation of engineering products and systems have contributed to the development of methods to prioritise the development of components for modularisation, predict change propagation and design for customisation (see Koh et al. Reference Koh, Förg, Kreimeyer and Lienkamp2015; Clarkson, Simons & Eckert Reference Clarkson, Simons and Eckert2001).
The technical nature of hard systems thinking assumes that the real world comprises systems that can be ‘designed’. Therefore, it implies that models of those systems can be made and their behaviour can be simulated (Checkland Reference Checkland1985). A model is a tool used to gain insights into phenomena and stakeholders, and the relations between them (DeTombe Reference DeTombe2015b ). Modelling and simulation tools represent a significant contribution from hard systems methodologies to design and engineering. For example, System Engineering (SE) is an established hard systems methodology for handling complexity and tackling the challenges of product development (Biahmou Reference Biahmou, Stjepandić, Wognum and Verhagen2015). System Engineering applies development models, such as product life cycle models, functional flow block diagrams, and data flow diagrams to synthesise data as a basis for better decision-making processes.
Previous research in design has explored the relevance of hard systems methodologies to handle complexity and develop product–service combinations. Cavalieri & Pezzotta (Reference Cavalieri and Pezzotta2012) provide an up-to-date review of the literature on SE, paying particular attention to how SE can support the design and development of services either as a system or as a product–service combination. Afshar & Wang (Reference Afshar and Wang2010) use SD to develop models and simulate system behaviour quantitatively, allowing designers to handle the structural complexity of product–service systems (PSSs). The authors have employed SD tools to represent systemic relationships among stakeholders, economic activities, and material flows, as well as to simulate cause and effect relationships among those components.
5.2 Reflections on soft systems methodologies: participatory design
The paradigm shift towards social orientation in design has slowly moved stakeholders from their traditional role as a ‘passive audience’ to become ‘co-creators of value’ (Prahalad & Ramaswamy Reference Prahalad and Ramaswamy2000, p. 80). Other authors share similar presumptions about participation in design (see Cross Reference Cross2001; Nelson Reference Nelson2008a ). Design as a social process takes places in a conglomerate of interactions and negotiations between stakeholders who bring with them their individual world views, comprised of their specific knowledge and awareness of aspects of the systems under design (Metcalf Reference Metcalf2014). Therefore, there is a need for collaboration among those who design systems, those affected by the designed systems, and those invested in the outcome of the system but who are not directly served by the outcome (Nelson Reference Nelson2008a ).
Soft systems methodologies can assist in bringing about accommodation between distinct value positions and can generate commitment among stakeholders to implement agreed objectives (Jackson Reference Jackson2003). Soft systems thinkers such as Churchman, Ackoff, and Checkland, through their systems methodologies, advocate respect for the world views, goals, and objectives of all the stakeholders involved in the problem situation and affected by the problem solution (Jackson Reference Jackson1985). Another major aspect of soft systems is their attempt to avoid formulating problems according to one particular perspective to the exclusion of others. Using open-framed problem definitions and open-ended solutions equip designers with the opportunity to adapt and reconfigure solutions to better fit the needs of the system during the project development. Moreover, such adoption allows designers to deal with higher levels of uncertainty and unpredictability.
Soft systems move from the idea of ‘optimising’ to the concept of ‘learning’ (Checkland Reference Checkland1985). Such methodologies are also influenced by research fields like action research and participatory action research. These provide human-centred approaches like Participatory Learning and Systems Learning (see Ison, Maiteny & Carr Reference Ison, Maiteny and Carr1997; Flood Reference Flood2010). Influences of this type of research can be seen in emerging design approaches, such as human-centred design (ISO 2010), customer-centred design (Beyer & Holtzblatt Reference Beyer and Holtzblatt1997), people-centred design (Wakeford Reference Wakeford2004), user-centred design (Vredenburg, Isensee & Righi Reference Vredenburg, Isensee and Righi2002), and Participative Ecodesign (Ison Reference Ison1993).
5.3 Reflections on critical systems methodologies: design ethics
The integration of critical systems methodologies in design practice involves two major aspects: design ethics and implications of design practice. Scholars have raised concerns about the design of social and societal systems and the underlying ethical choices of designers (see Banathy Reference Banathy1996; Manzini Reference Manzini2006). According to Manzini (Reference Manzini2006), when a solution is dictated by coercive relationships and technical constraints, there is no design in place. Manzini challenges the idea of well-being socially constructed over time in design, which is based on the democratisation of access to products (product-based well-being). Instead, he proposes the conception and development of systems that consider and enable people’s capabilities and promote sustainable well-being. Similarly, others advocate that people in the system should become the experts, rather than the design being brought from experts (Metcalf Reference Metcalf2014).
The second aspect, which concerns the implications of design practice, refers to the social consequences of design action and choices concerning specific methods, tools, and techniques. Choices made by designers with strong systems-oriented thinking are guided with respect to the appropriate way to engage the problem regardless of the situation (Bausch Reference Bausch2014). Critical systems thinking provides valuable insights into criteria for complex problem-solving methods, tools, and techniques (Murthy Reference Murthy2000). For example, some authors have provided insight to increase understanding of the strengths, weaknesses, and theoretical underpinnings of available systems methodologies (critical awareness) (Oliga Reference Oliga1988); to make explicit the social consequences of using different systems methodologies (social awareness) (Jackson Reference Jackson1985); to promote human emancipation (Jackson Reference Jackson2001; Ulrich Reference Ulrich1983, Reference Ulrich2013); and to support systems practice (pragmatism) (Jackson & Keys Reference Jackson and Keys1984).
6 A selection of systems-oriented design approaches
This paper builds on central and relevant theories and practices that contribute to the debate of a systems-oriented perspective and sustainability issues in design, referred to here, for the sake of simplicity, as systems design approaches. It aims to foster designers’ understanding of systems thinking in order to contribute, through design, to the handling of complex societal problems. Systems design approaches differ from systems approaches, as the latter is concerned with the different traditions of systems thinking and imply different ways of thinking about how systems approaches relate to each other and the application of distinct sets of methodologies, knowledge, skills, and tools. In this paper, a systems design approach refers to the mental model through which designers can frame the world, sometimes referred to as a perspective or paradigm. Such an approach guides designers as they incorporate systems theory into design practice and develop design theory grounded in systems theory (Figure 3). In other words, it guides designers in their interpretation of systems approaches and methodologies to handle complex problem situations and design better systems.
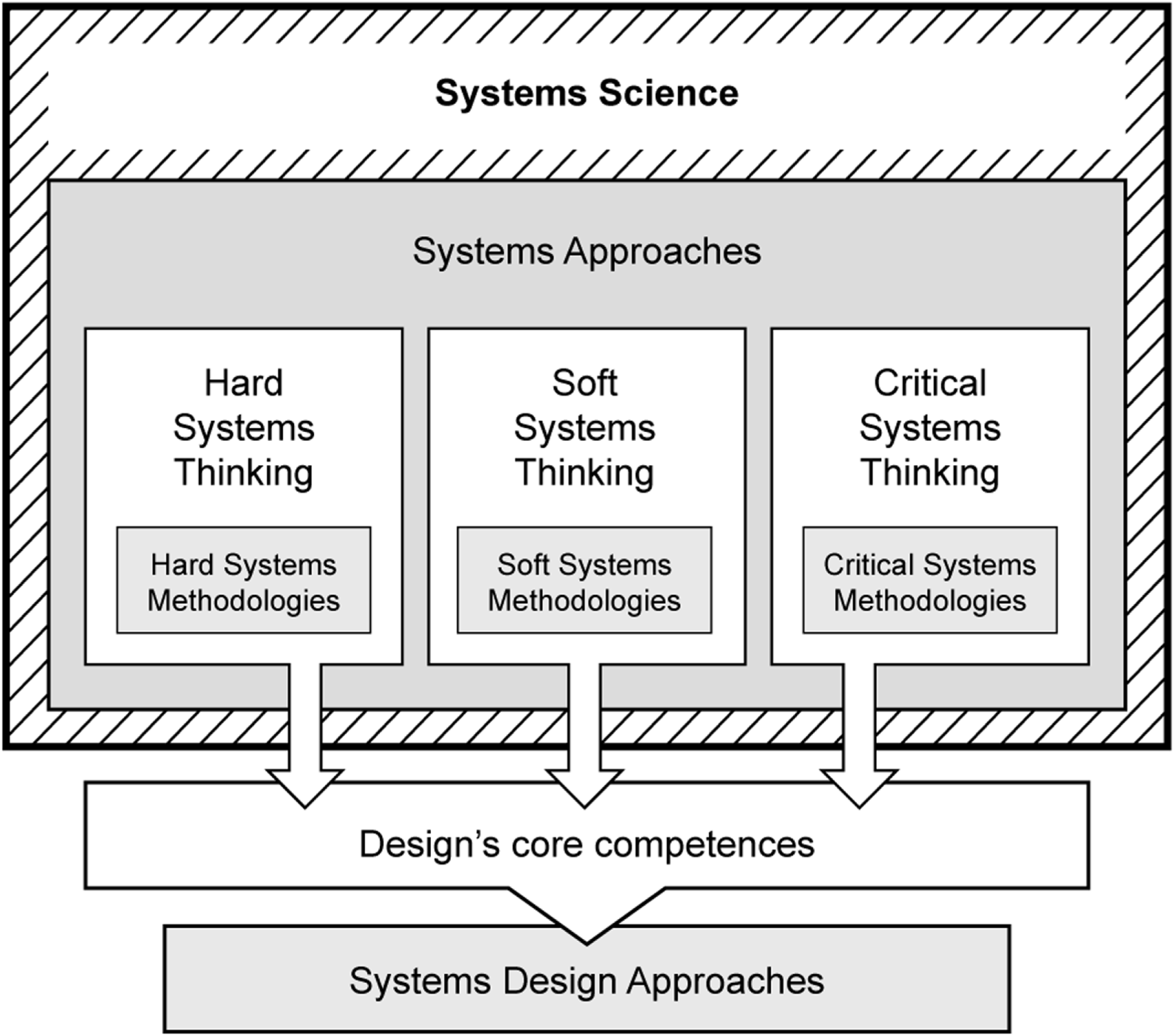
Figure 3. Systems design approaches.
So far, we have attempted to gain a better understanding of systems thinking. In the next sections, we capture examples from the literature that illustrate how the design community has interpreted and employed systems methodologies to tackle complex societal problems. In particular, we strive to identify critical factors that contribute to enhancing integration.
6.1 Whole system(s) design
Whole system(s) design is a collaborative and integrative approach that aims to enhance the collective response to complex problems, such as sustainability challenges (Pittman Reference Pittman2004; Stasinopoulos et al. Reference Stasinopoulos2009). It uses learning principles based on a holistic approach to systems inquiry and design practice (Nelson & Vanpatter Reference Nelson and Vanpatter2004; Nelson Reference Nelson2005). While systems thinking provides a base for synthesising knowledge, design practice supports innovative inquiry and creative action (Nelson Reference Nelson2005). Through this approach, designers make decisions and choices on what systems methodologies and design tools to employ based on their understanding of each problem situation and their expertise (Nelson Reference Nelson2004).
Whole system(s) design exploits SSMs like Inquiring Systems Design (Churchman Reference Churchman1971), and Social Systems Design (Churchman Reference Churchman1971; Ulrich Reference Ulrich1983). This systems design approach contends a co-participatory approach to the problem situation, where solutions should not be imposed. Rather, stakeholders should be empowered in the functioning of the system. Moreover, stakeholders actively participate in the conceptualisation and implementation of the newly designed system (Nelson Reference Nelson2004).
6.2 Systems-oriented design
Systems-oriented design (SOD) is a skill-based approach intended to develop better designs, visualisations, and systems practices (Sevaldson et al. Reference Sevaldson, Hensel, Frostell, Ceschin, Vezzoli and Zhang2010; Sevaldson Reference Sevaldson2011, Reference Sevaldson2013). This holistic approach was developed in project-based education with the intention of creating a new generation of designers who can cope with enhanced complexity (Sevaldson et al. Reference Sevaldson, Hensel, Frostell, Ceschin, Vezzoli and Zhang2010; Sevaldson Reference Sevaldson2011, Reference Sevaldson2013). It considers different hierarchies and boundaries within a particular socio-technical system to increase the capacity of the system to address its function and achieve sustainability (Sevaldson et al. Reference Sevaldson, Hensel, Frostell, Ceschin, Vezzoli and Zhang2010; Reinders, Diehl & Brezet Reference Reinders, Diehl and Brezet2012). As such, it proposes the design of a coherent combination of processes and product–services combinations that together can fulfil the function of the system.
According to Sevaldson (Reference Sevaldson and Menges2008, Reference Sevaldson2015), early systems thinking research followed a mechanistic approach which regards systems as mechanical, cause–effect driven networks, and therefore, offers a limited fit for the scope of SOD. Hence, SOD adopted a pragmatic view on modern systems thinking rooted in soft and critical systems thinking which ‘deals with the dynamic complexity of real-world problems in a pragmatic way’ (Sevaldson Reference Sevaldson2013, p. 3). From Systems Architecture (SA), SOD explores the capability to synthesise complex problem situations (Sevaldson Reference Sevaldson and Berg2009). Moreover, SSM offers helpful tools like the Rich Picture (Checkland Reference Checkland1981). The concept of Rich Picture was reformulated to create a generative mapping tool called GIGA-mapping (Sevaldson Reference Sevaldson2011). GIGA-mappings are large and information-dense diagrams that act as a bridge between inquiry and design. Such visualisation maps are used to synthesise and interrelate knowledge, and they promote a shared understanding of the system among stakeholders (Sevaldson Reference Sevaldson2013, Reference Sevaldson2015).
6.3 Product–service systems
In the literature, the integration of product and services is most often called a PSS. However, the fundamental assumption underlying PSS, i.e., to provide functions which fulfil human needs through product–service combinations, is found across different disciplines, such as Operational Research, Information Systems, Systems Engineering, Cyber-Physical Systems, Innovation and Business Management, and Marketing. While there is no consensus regarding the roots of PSS in Systems Science, many authors provide corroboration that this approach fundamentally follows a holistic mindset (Briceno & Stagl Reference Briceno and Stagl2006; Lindahl et al. Reference Lindahl, Takata and Umeda2007; Coley & Lemon Reference Coley and Lemon2009; Geum & Park Reference Geum and Park2011; Mukaze & Velásquez Reference Mukaze and Velásquez2012).
For many scholars, a systems thinking perspective on PSS is fundamental for a proper conceptualisation and in-depth understanding of the system in place (Afshar & Wang Reference Afshar and Wang2010; Cavalieri & Pezzotta Reference Cavalieri and Pezzotta2012). Several authors (e.g., UNEP 2001, 2002; Manzini & Vezzoli Reference Manzini and Vezzoli2003; Tukker & Tischner Reference Tukker and Tischner2006) agree that PSS can stimulate major changes in current production and consumption patterns for an environmentally sound path to socioeconomic development. According to these authors, PSS can promote higher system efficiency leading to higher levels of well-being at lower cost in complex societal contexts.
Previous studies have attempted to combine PSS and the field of systems thinking (Vezzoli, Ceschin & Kemp Reference Vezzoli, Ceschin and Kemp2008; Afshar & Wang Reference Afshar and Wang2010; Ceschin Reference Ceschin2012; Cavalieri & Pezzotta Reference Cavalieri and Pezzotta2012; Joore & Brezet Reference Joore and Brezet2015). For instance, Afshar & Wang (Reference Afshar and Wang2010) propose employing SD in PSS as a tool for analysing/synthesising causal loops (e.g., systemic relationships among stakeholders, economic activities, and material/energy resources) and simulating the dynamic behaviour of systems quantitatively (simulate system’s behaviour). Also, Joore (Reference Joore2010) proposes a multilevel approach that takes into consideration the development of PSS in relationship to the changes that happen within its socio-technical systems.
6.4 Design for development
Both the need to support increasing changes in the scale of the challenges facing the development of society’s infrastructure and resource limitations, have led to the emergence of new fields of design. The implementation of a systems perspective in design is perhaps most fruitful, and therefore, most needed in the context of developing economies, where almost no formal systems are in place and where there is a lack of socio-technical networks and infrastructures (Sklar & Madsen Reference Sklar and Madsen2010). Design for Development (DfD) includes design approaches aimed at marginalised groups, where problem solutions assist social, human, and economic development (Donaldson Reference Donaldson2002). Those emerging approaches recognise the need for a societal perspective which considers the capacity of design to improve stakeholders’ well-being by meeting currently unmet basic needs of existing generations while fostering sustainable production and consumption for future generations.
For instance, some approaches aim to improve social and economic sustainability performance, such as Design for the Base of the Pyramid (DfBoP) (Crul & Diehl Reference Crul and Diehl2006). Other approaches focus on assisting stakeholders to employ personal resources (individual capabilities), and problem solutions to which they have access, like the Capability Approach (CA) (Nussbaum & Sen Reference Nussbaum and Sen1993; Mink, Diehl & Kandachar Reference Mink, Diehl and Kandachar2018). According to Oosterlaken (Reference Oosterlaken2009), DfD approaches have raised awareness about how problem solvers practice design. For the author, to expand human capacities of marginalised groups with the help of design, systems thinking needs to be integrated into design, as in approaches like Whole System Design (WSD) and PSS.
From a systems thinking perspective, problem solvers should empower communities to solve their own problem situations (Meadows Reference Meadows2008). Oosterlaken (Reference Oosterlaken2013) and Mink (Reference Mink2016) discuss at length the relationship between design and the CA and highlights the differences in social relations of power as a major issue in the expansion of human capabilities and agency by design. Furthermore, Zheng & Stahl (Reference Zheng and Stahl2011, Reference Zheng and Stahl2012) argue that CA fails to consider issues of distribution of power and conclude that Critical Theory, as applied by Information Systems and Science and Technology Studies, can be beneficial. Critical Theory follows two major approaches: Critical research in information systems, and Critical theory of technology. Similar to Critical Systems Thinking, these approaches aim to reveal the social structure of power, control, domination, and oppression, and thereby promote emancipatory social practices (Zheng & Stahl Reference Zheng and Stahl2011).
7 Results: conceptual framework for systems design approach
Design is an approach to inquiry and action not limited to the creation of physical products or structures. Rather, it is ‘an approach to human agency in a complex world’ based on foundational ideas inclusive of systems thinking (Nelson Reference Nelson2008a , p. 2). According to Checkland (Reference Checkland2000), systems practice, or when a problem solver knowledgeably applies a systems methodology to improve a perceived problem situation, involves three fundamental elements: (1) the underlying methodology, (2) the perceived problem situation, and (3) the stakeholders involved and affected by the use of the approach. Systems practice is helpful to generate an understanding of how a systems design approach (A), which is the application of different systems methodologies and design methodologies (M) supports users (U), whether those users are involved or affected stakeholders, to handling a problem situation (P).
For instance, SOD (A) (Sevaldson Reference Sevaldson2011, Reference Sevaldson2013) builds on two systems methodologies, SSM and Systems Architecture (M) (Sevaldson Reference Sevaldson2011). This systems design approach relies heavily on the technique of Giga-mapping, which is a holistic mapping tool for boundary critique (e.g., boundary judgements concerned to what observations are to be considered relevant or not) to the conception and framing of complex systems (Sevaldson Reference Sevaldson2011). Developed using SOD, the project ECO CAP (ecological capsule for cloning trees) proposes a holistic evaluation of economic and social factors in rural communities to engage in the local production of seedlings and the planting of trees as an alternative to public–private partnerships (Sevaldson Reference Sevaldson and Berg2009). The Institute of Industrial Design at the Oslo School of Architecture (U) has used this approach to address complex societal problems (P) in small communities in Oslo (U).
Existing systems design approaches, such as those presented in Section 6 have provided a significant contribution to the transition from a traditional design approach to a systems-oriented perspective in design. Based on these theoretical insights the authors emphasise the need for developing new and strengthening existing systems design approaches. Therefore, the authors propose a conceptual framework that aims to facilitate new and existing approaches to fully realise the resources of systems thinking from which they can draw. The following sections present the main discussions involving the five major clusters or set of elements of the framework and demonstrate how they influence each other in an iterative process: mindset, methodology set, knowledge set, skill set and tool set.
Mindset concerns the understanding of the assumptions underpinning systems thinking. It supports designers to reflect on the need to complement traditional design approaches with a system-oriented perspective when addressing complex societal problems. The methodology set deals with identifying a dominant way of thinking adopted by a systems methodology and creating alignment with the designer’s problem-handling approach. By identifying the dominant stance of the systems methodologies adopted, a better understanding of the appropriate knowledge required to address a problem situation can be created. Based on the result of this stage, through knowledge set is possible to determine the appropriate knowledge that needs to be generated to address the problem. The skill set covers the complementary skills to design’s core competencies required to support the production of knowledge required for handling the problem or system. Finally, tool set covers tools and techniques that can be used to explore competencies in order to mediate and facilitate reasoning, visualising, modelling, sense-making and sense-sharing.
Whichever systems approach is taken, it is important to acknowledge that each approach has different strengths and weaknesses. Therefore, any systems approach is better introduced into design through a combination of aspects from different systems methodologies. This makes it interesting to reflect on the overlaps and distinctions between the various systems approaches and methodologies which were presented in previous sections. The conceptual framework that emerged from the literature review offers criteria for complementary and informed exploration of systems thinking. It is proposed and discussed largely in terms of Habermas’ (Reference Habermas1972), Jackson & Keys’ (Reference Jackson and Keys1984), Banathy’s (Reference Banathy1987), Nelson’s (Reference Nelson2004, Reference Nelson2005) and Ryan’s (Reference Ryan2014) formulations. This framework supports new thinking through the cross-fertilisation of knowledge and perspectives focusing on systems practice.
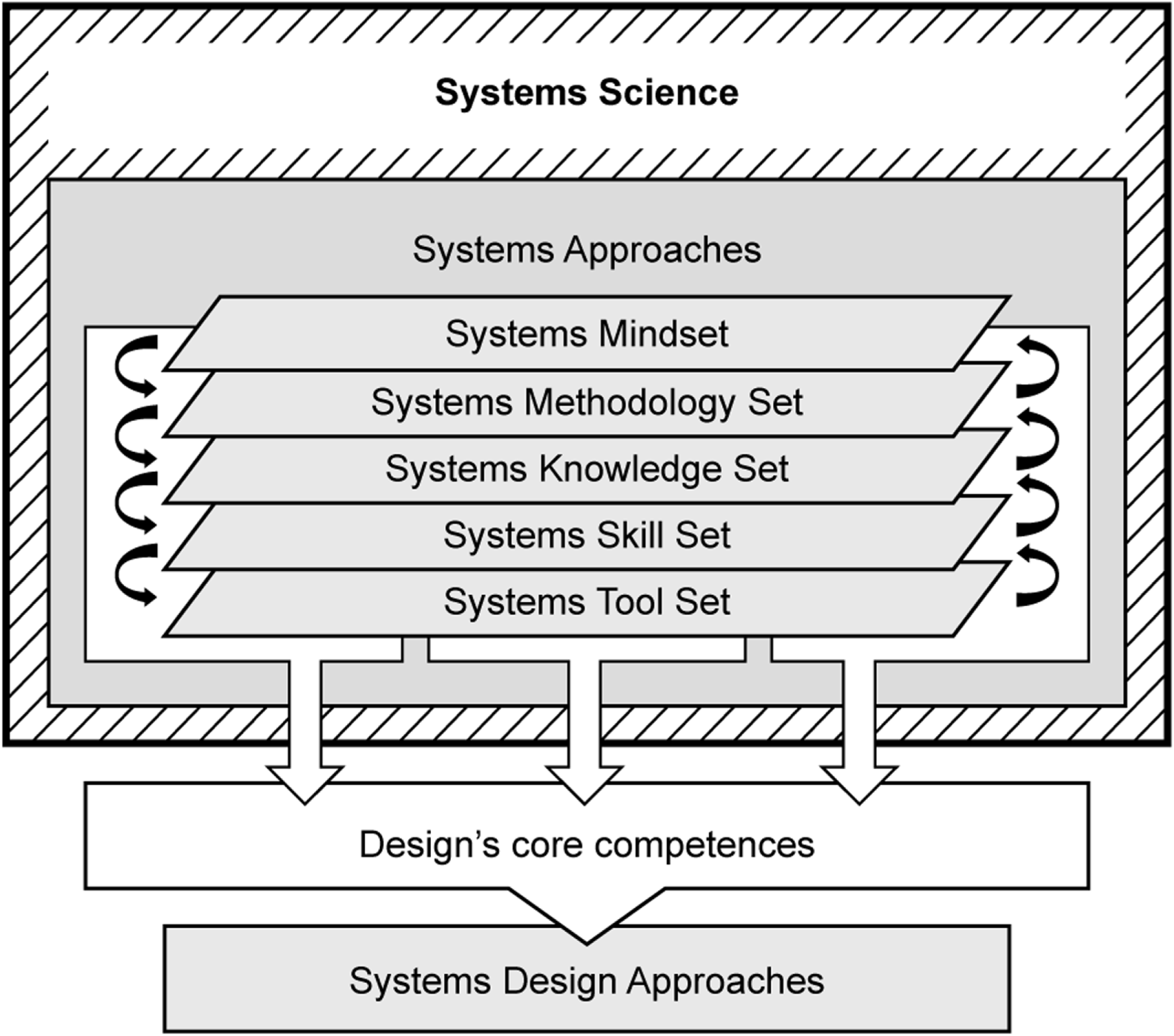
Figure 4. Conceptual framework for Systems Design Approaches.
It is not intended that the framework represents a tool for choice of methodology. Instead, it is meant to provide support for the exploration and interpretation of systems theory in the context of design. It predicates the appropriateness of different systems methodologies through reflection on the nature of the problem situation and the relationship between stakeholders. Answers to reflective questions assist in the systematic identification of relevant characteristics of the problem, system, and stakeholders, which are helpful in making decisions and selecting methodologies, tools, and techniques (see Table 1). Given these considerations, the proposed framework is an attempt to support designers to tailor a traditional design approach to a system-oriented approach by integrating system thinking into design through the consideration of five sets of elements described in Sections 7.1–7.5. Along with the explanation of each cluster, we provide a hypothetical example that illustrates the application of each element of the framework.
Table 1. Overview of the conceptual framework for systems design approaches

7.1 Systems mindset: establishing the need for systems thinking
This framework element is broadly applicable to complex societal problems. Fundamentally, systems mindset is proposed as a complement to, rather than a replacement for, traditional design approaches. Problem solvers should reflect on the main assumption underlying the current design approach to reveal its limitations. In a hypothetical energy solution for low-income households in rural areas, the design team may recognise that the current design approach lacks resources to examine the problem of energy access from multiple perspectives, to deal with the increasing complexity of low-income energy markets and to support a radical transition to sustainable energy systems. Once the limitations are outlined, system thinking tenets can be put in place to ensure that the desired outcome can be achieved. As proposed in the framework, the shift to a systems design approach concerns the adoption of three major systems thinking tenets: a holistic perspective; diversity of views; and complexity-handling capacity.
First, corroboration exists that a holistic perspective to the problem solution is paramount when designing solutions for complex societal problems such as those concerning sustainability issues like energy challenges in low-income markets (Clegg Reference Clegg2000; Cardenas et al. Reference Cardenas2010; Blizzard & Klotz Reference Blizzard and Klotz2012; Forlizzi Reference Forlizzi2012; Jones Reference Jones and Metcalf2014). A major challenge in complex societal systems is that system components in isolation do not achieve sustainability for the whole. In contrast, if the system of concern is sustainable, then the system components therein can be regarded as sustainable (Gaziulusoy Reference Gaziulusoy2015).
Second, many authors agree that systems thinking has the potential to hold differing world views (Jackson & Keys Reference Jackson and Keys1984; Daellenbach Reference Daellenbach2001; Jackson Reference Jackson2003) and promote a participatory design process that considers the interconnections between stakeholders and others components of the socio-technical system (Laszlo, Laszlo & Dunsky Reference Laszlo, Laszlo and Dunsky2010). This is particularly relevant in low-income energy markets because designing effective solutions involves multiple stakeholders including private companies, government, energy utilities, end-consumers, knowledge producers, community representatives and non-governmental organisations.
Finally, systems thinking is a problem-solving approach capable of handling high levels of problem complexity (Ackoff Reference Ackoff1974; Espinosa et al. Reference Espinosa, Harnden and Walker2008; DeTombe Reference DeTombe2015b ,Reference DeTombe a ), as well as multiple aggregation levels of a problem (Elzen, Geels & Green Reference Elzen, Geels and Green2004; Geels Reference Geels2005; Joore & Brezet Reference Joore and Brezet2015; DeTombe Reference DeTombe2015b ). Given the complexity of energy challenges in low-income markets, it is likely that improvements on a lower level (e.g., new products and technologies), although fundamental, are limited to create sustainable energy transitions. The transition to sustainable energy systems in low-income markets requires profound technological, institutional and sociocultural transformation, which requires the attention to multiple aggregation levels of the problem.
7.2 Systems methodology set: identifying a dominant way of thinking
Systems methodology set concerns the understanding of the underlying paradigms that guide the interpretation of systems approaches and the application of systems methodologies. Systems approaches are structured and dominant ways of thinking that follow different theoretical reasoning, namely functionalist, interpretative, and emancipatory (Daellenbach Reference Daellenbach2001; Jackson Reference Jackson2001). For this reason, it is important to take account of the dominant epistemological stance of a given system approach. The stance adopted can affect whether a piece of information generated or collected using a given systems methodology is accurate or not to develop a solution, given the characteristics of the problem and the relationship between stakeholders of the systems.
For example, for the case of an energy solution for low-income households in rural areas, the design team would likely adopt a participatory approach in which all parties need to be willing to share information to achieve shared understanding, giving the users the role of co-creators. This process plays an important role in the outcomes of the system, in particular if the low-income population have limited access to information and education and do not actively participate in the definition of policies and solutions for the problems of the communities in which they live. Energy-related issues are often hard to tackle due to limited information about specific problems that impact the everyday life of the population and the societal complexity within the network of stakeholder involved. In this context, the design team would greatly benefit from the application of resources from interpretative and emancipatory systems methodologies.
There are two major aspects involving stakeholders’ relationships in societal complexity. The first is the impact of individual and group relations in social processes, while the second, and perhaps more crucial, is the impact of human relations and social processes on society as a whole. In this context, individuals and groups within a system may exhibit (dis)similar values, beliefs, and interests, and exert (un)equal influence in decision-making processes. Based on these characteristics, the relationship of those concerned with the problem situation (stakeholders) can be classified as unitary, pluralist, or coercive (Jackson & Keys Reference Jackson and Keys1984). Stakeholders in a unitary relationship have similar values, beliefs, and interests; they share common objectives and are all involved in the decision-making process to some extent. Stakeholders in a pluralistic relationship may not share the same values and beliefs. However, through debate, disagreement, or even conflict, compromises are made, and shared understanding can be reached in a participatory decision-making process. In a coercive relationship, stakeholders usually share little interest and experience conflicting values and beliefs. Furthermore, the decision maker can enforce the implementation of a solution and compromises are hardly possible (Daellenbach Reference Daellenbach2001).
Table 2 provides an overview of the general characteristics of systems methodologies based on their fundamental systems approach and can be used as a starting point to gain a better understanding of systems practice. Hard systems thinking is a functionalist approach, which is most effective when the desired end state of the system is known and the problem addressed is large of technical complexity. Conversely, this approach has limitations in handling problem situations with significant societal complexity (Daellenbach Reference Daellenbach2001) because it assumes that stakeholders’ values and beliefs are simple enough to be modelled or simulated (Jackson Reference Jackson2003).
Soft system thinking is an interpretative approach which assumes that social processes depend on the world view of their interpreters (Bausch Reference Bausch2014). Soft systems thinking can cope with a fair degree of societal complexity (Daellenbach Reference Daellenbach2001). On the other hand, this approach cannot be applied if the conditions for open debate and shared understanding are absent (Jackson Reference Jackson1985; Oliga Reference Oliga1988).
Critical systems thinking is an emancipatory approach that takes into account that stakeholders are often unequal regarding power over the problem situation, and therefore, certain stakeholders’ views may be privileged over others (Phelan Reference Phelan1999). It contends that functionalist and interpretive systems approaches neglect the existing structures of inequality of power, wealth, status, and authority which may be operative without the stakeholders concerned being aware of them (Oliga Reference Oliga1988). Therefore, critical systems thinking aims to expose such inequalities so that radical change to emancipate those who are often most affected by the system outputs take priority over the interests of the decision maker.
Table 2. Characteristics of systems methodologies
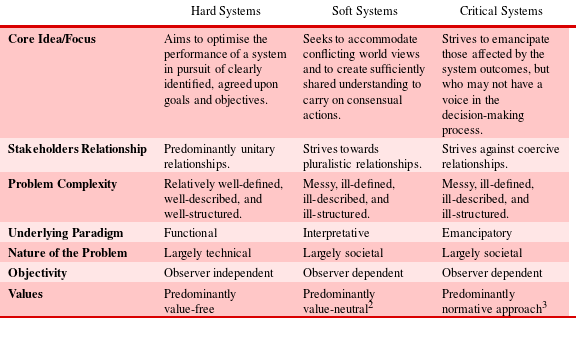
7.3 Systems knowledge set: determining the knowledge creation process
Knowledge emerges from data and information. It is best defined as the theoretical and practical understanding of a subject. By using different systems approaches and methodologies, data can be collected in quantitative and qualitative form for the purpose of explaining, interpreting, and reflecting on the various aspects of a system. The creation of knowledge is influenced by a variety of human interests (Habermas Reference Habermas1972): a technical interest in the prediction and control of natural and social systems (causal explanation); a practical interest in communication and creation of shared understanding among all stakeholders in social systems (practical understanding); and an emancipatory interest in humans to protect them from constraints imposed by power structures (reflection). According to Jackson (Reference Jackson1991), Habermas’ Theory of Knowledge and Human Interests helps to adequately assess the theoretical and methodological legitimacies and limitations of different pieces of knowledge. Such an assessment provides criteria for careful consideration of the relationships of those concerned with the problem situation and the knowledge creation process.
In the case of an energy solution for low-income households in rural areas, relying largely on data from national household consumption surveys conducted by official national statistic offices would likely restrict the design process creating an inability to understand the unique characteristics of the local context. Instead, the design team should produce context-specific knowledge because user behaviour and habits towards energy consumption in low-income communities tend to be profoundly influenced by a number of local norms, beliefs and circumstances that are not contemplated in large-scale national surveys. Moreover, to realise a transition to a sustainable energy-efficient rural community the design team should generate different types of knowledge such as knowledge about the system components relevance to the transition, knowledge on how to realise the transition from ‘current’ to ‘future’ state (scenarios), and knowledge of the desired state (vision) (see Gaziulusoy Reference Gaziulusoy2010).
Table 3. Knowledge creation in systems approaches

Table 3 provides an overview of the characteristics of knowledge creation in different systems approaches, and it is helpful in defining the appropriate knowledge that needs to be acquired during design inquiry and applied in design action. Design action needs to take into account both the human interest underlying knowledge generation and the embodied understanding of designers, referred to by Nelson (Reference Nelson2005) as design knowing. Design knowing includes: knowing based on reason (conscious knowing); intuition (unconscious knowing); imagination (subconscious knowing); and conscious not knowing (Nelson Reference Nelson2005, p. 4).
7.4 Systems skill set: identifying the need for complementary skills
Systems thinking skills provide a new foundation for design’s core competencies and skilful performance when tackling complex problems situations. Conley (Reference Conley2004) proposes seven core design competencies: understand the context or circumstances and frame the problem; define the appropriate situations level of abstraction; model and visualise solutions, even with ill-defined information; simultaneously create and evaluate multiple alternatives to the problem; add and maintain value as the process of problem solving unfolds; establish purposeful relationships among elements of a solution and between the solution and its context; and finally, use form to embody ideas and to communicate their values. When addressing complex societal problems, problems solvers realise that the skills they acquired during traditional design training do not align with the nature of the challenges that they are expected to tackle, and therefore, new skills are required. Systems skill set comprises a set of novel abilities that complement design competencies by providing a new foundation for dealing with complexity. Based on Costa Junior et al. (Reference Costa Junior, Diehl and Secomandi2018), the authors suggest five key systems-oriented skills necessary for skilful performance when tackling complex societal systems or problems (see Table 4).
Table 4. Key systems skills

For instance, in the case of an energy solution for low-income households in rural areas, a design team with a multilevel perspective would strive for an energy solution with the ability to create impact at multiple aggregation levels: at micro-level by improving existing or developing new energy-efficient products and promoting more sustainable consumption patterns; at meso-level by creating new product–service combinations and facilitating the collaboration between energy utilities, non-governmental organisations and community facilitators; and at the macro-level by striving towards the adoption of policy choices that stimulate the creation of economic and regulatory instruments favourable to the implementation of systemic solutions. Because the competences required to achieve such solutions are interdisciplinary and diverse, the design team is likely to build a multidisciplinary project team. Moreover, adopting an open-framingFootnote 4 approach to the problem allows the team to cope with the uncertainty and unpredictability involving low-income energy markets. Finally, the team should acknowledge that each stakeholder may exhibit a different perception of the functionality and adequacy of the energy solution and a particular motivation to engage in the development of the system.
7.5 Systems tool set: define appropriate tools and techniques
Tools provide means to explore competencies and capabilities accurately. They mediate tasks to facilitate collaborative inquiry, reasoning, visualisation, modelling, simulation, and creation. Systems methodologies provide tools that can support designers to see (e.g., causal loop diagrams, behaviour over time graphs, stock/flow diagrams and systems maps), understand (e.g., mental models, generative and participatory tools) and act (e.g., formal models) in problem situations (Skyttner Reference Skyttner2006). Systems tool sets draw on tools and techniques developed according to the strengths of a particular systems methodology.
Taking our example, to gain a better understanding of energy demand and consumption patterns in low-income contexts, a design team tackling the lack of energy access for low-income households in rural areas would benefit from soft systems thinking tools such as rich picture, root definition and conceptual model (see Checkland Reference Checkland1981). For instance, visualisation tools such as rich picture can be used to synthesise and interrelate knowledge, communicate complexity, and to facilitate a shared understanding of the system among stakeholders (see Figure 5).

Figure 5. Rich picture of the problem of energy access in low-income rural areas.
For Jackson (Reference Jackson2001), the application of systems tools and techniques should consider the following guidelines. Tools and techniques, as applied in hard systems methodologies, aim to gain knowledge of the real world and capture the logic of the problem situation. The outcomes of these tools are mainly connected with the purpose of the design and provide input to improve the problem situation. Soft systems methodologies employ tools to deepen understanding of the real world and promote debate about feasible and desirable actions for change. In critical systems methodologies, tools and techniques focus on creating awareness among marginalised groups about their situation and suggesting improvements in their problem situation.
8 Conclusion
In this paper, an attempt has been made to explore the integration of a systems thinking foundation for design theory and practice and some of its applications. It covers a portion of the vast field of systems thinking research and focuses on its contributions to design. Based on the selected theoretical foundations of systems thinking, the paper elaborates on three systems approaches unfolding in their capacity to handle complex problems and to conduct a social inquiry. The different systems approaches can be appropriate to address different types of (complex) problem situations in which designers are required to intervene. We demonstrate that each systems approach is useful in terms of managing a particular combination of complexity and stakeholder relationships, and should be used in such appropriate/specific circumstances. To support this process, we provide a conceptual framework for systems design approach applied to complex societal problems.
Although the framework focuses on the broader context of complex societal problems, it was developed within the more narrow context of energy solutions for low-income markets which may contribute to bias due to their particular focus on energy PSSs. For that reason, further case studies are needed to assess the use of the framework in conjunction with other traditional product–service approaches in multiple industry and service sector such as healthcare. Moreover, although the framework helps to identify the resources (e.g., systems skills) required to the application of systems thinking into design, those resources still need to be developed by designers or outsources in order to be applied in design practice. Similarly, identifying the strengths of different systems approaches and the appropriateness of different systems methodologies does not create the perfect fit to handle all real-world problem situations. Future studies on the main topic described in this paper are therefore required to test and validate the framework as well as research on the process of capacity building for a systems design approach.
Despite the limitations of the contributions of this paper, we contend that uncovering the process of integrating system thinking into design can advance towards a better understanding of systems design approaches and complex societal problems. In contrast, the eventual lack of thoughtful exploration hinders problem solvers from making a more significant contribution to this front. The framework offers designers insights to promote informed decision-making and a complementary application of systems thinking to increase the competency and effectiveness of their approach to complex societal problems. In practice, there are many challenges in the implementation of systems design approaches. At the same time, this conceptual framework can assist in addressing some of these challenges and support emerging research on the integration of system thinking into design.