Introduction
Severe acute respiratory syndrome-coronavirus-2 (SARS-CoV-2) has infected many since the discovery of the virus in January 2020 [Reference Zhu1], and has led to significant morbidity and mortality [2]. An infection with SARS-CoV-2 leads to various clinical manifestations ranging from asymptomatic infections to severe coronavirus disease-2019 (COVID-19) [Reference Richardson3–Reference Streeck5]. The infection fatality ratio increases with age and underlying medical comorbidities [Reference Russell4, Reference Salje6].
An infection with SARS-CoV-2 is diagnosed through the detection of viral ribonucleic acid (RNA) using reverse transcriptase-polymerase chain reaction (RT-PCR) assays [Reference Zhu1, Reference Konrad7]. RT-PCR is measured in terms of cycle threshold (Ct) values, where lower Ct-values represent higher viral loads and residual nucleic acid [Reference Binnicker8]. Monitoring Ct-values supplemented by the date of symptom onset could provide a better picture on RT-PCR diagnostic sensitivity and shedding kinetics of viral RNA over time.
In Bavaria, Germany, the first cases of COVID-19 were observed in late January 2020 [Reference Böhmer9, Reference Böhm10]. Initial containment strategy consisted of testing contact persons regardless of symptoms of patients with a lab-confirmed infection of SARS-CoV-2 and returnees from risk areas with respiratory symptoms [11]. Contacts were tested for SARS-CoV-2 at the time of identification and 14 days after the last potential date of exposure. All positive patients were retested after a quarantine of 14 days. A positive test after 14 days extended the quarantine up to a negative RT-PCR. The repeated sampling of patients provided the opportunity to assess the diagnostic sensitivity of the RT-PCR relative to the symptom onset. This study aimed to quantify the sensitivity of RT-PCR and the presence of viral RNA through Ct-values relative to symptom onset.
Methods
Notification data
In Germany, COVID-19 is a mandatory notifiable disease. A case was defined as a person with SARS-CoV-2 infection confirmed by RT-PCR. Upon diagnosis, physicians are required to report a case to the local health authorities. For every reported case, local health authorities are requested to complete a questionnaire. This questionnaire covers, among others, demographic characteristics, date of disease onset, date of laboratory confirmation, hospitalisation status, probable place of infection and symptoms. Symptoms that were asked for included fever, coughing, running nose, headache, neck pain and pneumonia. Case information is subsequently shared with the Bavarian Health and Food Safety Authority (LGL) through an electronic notification system.
Specimen collection and laboratory confirmation
Initially, in Bavaria, most samples were tested at the Public Health Microbiology (PHM) laboratory of the LGL [Reference Konrad7]. As the epidemic advanced, additional private, hospital and university laboratories offered SARS-CoV-2 testing. All samples included in this study were tested at the PHM laboratory of the LGL. RT-PCR procedures at LGL were as follows: respiratory specimens (nasopharyngeal swabs, sputum or oral fluid) were obtained from patients and contact persons. Sputum specimens were diluted in 2 mL phosphate-buffered saline (PBS).
The following RNA extraction methods were used: QIAamp Bio Robot kit (QIAGEN) on a Hamilton Microlab Star (Hamilton, Bonaduz, Switzerland), RNAdvance Viral Kit on a Biomeki7 (Beckman Coulter, Indianapolis, USA), DNA Extraktion Kit on a m2000sp (Abbott, Chicago, USA), Maxwell 16 LEV Blood DNA Kit on Maxwell Instruments (Promega Fitchburg, USA) and BioSprint 96 One-For-All Vet Kit, IndiMag Pathogen Kit (INDICAL Biosciences, Germany), NucleoMag Vet Kit (Macherey-Nage, Germany) on KingFisher magnetic particle processors (ThermoFisherScientific, Waltham, USA). In the beginning in-house PCR detection for SARS-CoV-2-RNA was used according to references [Reference Konrad7, Reference Corman12], followed by the use of the RealStar SARS-CoV-2 RT-PCR Kit RUO (Altona Diagnostics) on the Bio-Rad CFX96 Touch Real-Time PCR Detection System (Bio-Rad, Feldkirchen, Germany), LightCycler 480 (Roche, Basel, Schweiz) or m2000rt System (Abbott, Chicago, USA). The assay detects E-Gene of B-lineage betacoronavirus in FAM and S-Gene specific for SARS-CoV-2 in Cy5. Threshold was manually set within the exponential phase of the detection curve. A specimen was considered positive when (i) both the E gene and the S gene, or (ii) when either the E gene (RealStar) tested positive or the S gene in the Realstar or RdRP with the Corman method tested positive [Reference Corman12]. Throughout the article, Ct-values concern Ct-values by using the E-gene target unless stated otherwise.
Statistical methods
To combine microbiological data of specimens tested at the LGL with notification data, we merged records based on the following variables: sex, month and year of birth, reporting municipality and date of laboratory confirmation. Records with identical variables were included for analyses on the condition that the date of laboratory confirmation was equal to or after date of sampling and date of reporting was equal to or after date of laboratory confirmation. We also excluded records of positive specimens taken five days before symptom onset, as 100% of specimens sampled before five days before symptom onset were measured negative [Reference Kucirka13].
To assess whether the positivity rate over time is different by age, sex or reported symptoms, Kaplan−Meier plots were used to visualise the empirical distribution of viral RNA detection. The estimated probability is a step function that changes at each event [Reference Clark14]. Probabilities were estimated for each additional day since symptom onset, and an event was the first upcoming negative result. Individuals who had no further tests after a positive result were right-censored. To consider that patients reached undetectable levels of viral RNA in between the last positive test and the first negative test, we left-censored the time to a negative test.
We used the locally weighted scatterplot smoothing (LOWESS) method to visualise the relationship between symptom onset and Ct-values. It is a non-parametric method where least-squares regression is performed in local subsets. We used R version 3.6.0 to conduct all analyses [Reference R Core Team15], and we used the R library survival [Reference Therneau and Lumley16] and survminer [Reference Kassambara17] to produce Kaplan−Meier plots and 95% confidence intervals (CI). Reported confidence intervals around the mean Ct-values in the text were estimated using bootstrapping (n = 1000) with R-library Boot. The lower and upper boundary of the confidence intervals were the 2.5th and 97.5 percentiles of the bootstrapped means.
Results
For 1306 COVID-19 cases, we identified corresponding records with both epidemiological and microbiological characteristics. Of these, 1300 (99.5%) COVID-19 cases complied with the condition that the date of sampling was before or equal to the date of laboratory confirmation and the date of notification took place on or before the date of laboratory confirmation. For 730 cases, the date of symptom onset was reported. Of these cases, 725 specimens were taken from 3 days before symptom onset until 48 days after symptom onset (Table 1, Fig. 1). Four of these cases tested negative for the E-gene but positive for the S-gene. Fifty-nine (8.1%) specimens were taken between three days to one day before symptom onset.

Fig. 1. Distribution of day of sampling relative to symptom onset for 725 COVID-19 cases in Bavaria, Germany.
Table 1. Characteristics of 725 individuals with a SARS-CoV-2 infection reported between 20 January and 19 May 2020 in Bavaria, Germany
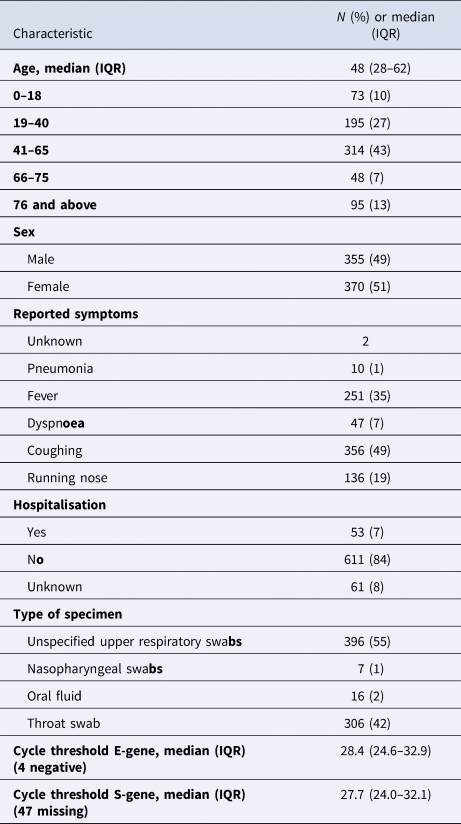
The mean Ct-value among 721 patients was 28.6 (95% CI 28.2–29.0). The mean Ct-value of specimens taken three days before symptom onset was 28.6 (95% CI 26.1–30.7), of specimens taken two days before symptom onset was 26.7 (95% CI 24.5–29.0) and of specimens sampled one day before symptom onset was 26.6 (95% CI 24.7–28.7). Ct-values depicted by the LOWESS curve were lowest among specimens taken close to symptom onset (Fig. 2), ranging from −3 to 3 days post symptom onset. From four days after symptom onset and onwards, the LOWESS curve showed an increase in Ct-values (Fig. 2).

Fig. 2. Ct values (to the E-gene target) of positive specimens shown by days since illness onset (n = 711). The smoothed line was fitted using the LOWESS method. Grey area is the 95% confidence interval.
Repeated sampling of COVID-19 patients
We identified 389 individuals who were tested twice including at least one positive specimen. None of these specimens had either a date of sampling after date of laboratory confirmation or a date of laboratory confirmation after date of notification. For 208 patients, date of symptom onset was available (Table 2). Thirty individuals had exclusively positive specimens, 139 started with positive specimens followed by negative specimens, 23 started with negative test result(s) followed by positive specimens only and 16 individuals had a mixed pattern of positive and negative test results (supplement, Fig. 1).
Table 2. Characteristics of 208 individuals with a SARS-CoV-2 infection who were sampled at least twice

Patients were reported between 20 January and 19 May 2020 in Bavaria, Germany.
The median days post symptom onset to a negative RT-PCR was 12.5 (95% CI 10.5–21.5, Fig. 3). In all, 10% tested negative after 7.5 days (95% CI 0.5–12.5), 25% after 10.5 days (95% CI 7.5–17), 75% after 21.5 days (95% CI 12.5–28) and 95% after 39 days (95% CI 30.5–43.5). The median time to a negative RT-PCR test was comparable between males (12.5, 95% CI 9.5–28.5) and females (12.5, 95% CI 10.5–21.5) and patients with (13.5, 95% CI 9.5–30.5) or without fever (12.5, 95% CI 10.5–21.5, Fig. 4). The median time to a negative test of patients older than 65 was longer (17.0, 95% CI 13.5–33.5) than patients below 19 (10.5, 95% CI 10.5–43.5+) and between 19 and 65 (12.5, 95% CI 10.5–21.5), but these estimates should be carefully interpreted given the wide confidence intervals. A Kaplan−Meier plot with the number of patients reported in the estimation of the probability of testing positive is provided as supplementary material (Figure S2).

Fig. 3. Time until the loss of SARS-CoV-2 RNA detection after symptom onset among 208 patients tested at least twice in Bavaria, Germany. The red line and red-shaded area depict the empirical distribution of persistence of positive tests and 95% confidence interval, respectively.

Fig. 4. Time until the loss of SARS-CoV-2 RNA detection after symptom onset among 208 patients tested at least twice in Bavaria, Germany stratified by (a) sex, (b) age and (c) reported fever.
Discussion
In our study, we aimed to describe the diagnostic sensitivity of RT-PCR and Ct-values of SARS-CoV-2 relative to the symptom onset in symptomatic COVID-19 cases reported in Bavaria, Germany. As all contact persons of patients with COVID-19 were tested regardless of the presence of symptoms, many infections were detected prior to symptom onset (8%) and most COVID-19 cases concerned non-hospitalised cases (84%). Ct-values were lowest, hence highest in viral load, close to symptom onset, with higher Ct-values from four days post symptom onset and onwards. This underlines the possibility of transmission and infection occurring prior to symptom onset as described elsewhere [Reference Böhmer9, Reference Arons18].
In a subset of repeatedly sampled patients, we found that specimens taken up to 7 days post symptom onset had a 90% chance to test positive, this remained 75% up to 10.5 days and reduced to 50% with 12.5 days. The mean median duration of viral RNA shedding was found to be 17 days in upper respiratory specimens from 43 studies [Reference Cevik19]. The majority of these studies were testing hospitalised patients, which could explain the longer shedding time compared with our estimate as only 16% of notified COVID-19 cases in our sample had been hospitalised. Another explanation could be due to the use of different methods either incorporating or not incorporating the time between the last positive and first negative test. As the time between sampling was considerable (Figure S1) due to a testing policy which consisted of testing contact persons upon identification and after 14 days, we took into account the time between the last positive test and the first negative test. Without this correction (i.e. left-censoring), the median duration until a negative test was 22 days (95% CI 21–23). This estimate resembles three other estimates ranging from 19 to 23 days derived using the Kaplan−Meier method [Reference Wang20–Reference Yan22]. With a sharp decrease from around 7–10 days post symptom onset, one could consider complementing diagnosis by using serology to detect a (past) infection, as the sensitivity of detecting antibodies (IgM and IgG) increases [Reference Long23]. Eleven days post symptom onset, the sensitivity of antibody testing was estimated to be more than 80% (IgG) [Reference Long23]. In another study, serology, measuring total antibody levels outperformed RT-PCR after seven days [Reference Lou24], and in another study measuring IgM even after 5.5 days [Reference Guo25].
Positive specimens were found up to 3 days before symptom onset. A limitation of our study is that we cannot assess the denominator, i.e. the total number of infected people who were tested three days before symptom onset. Kucirka et al. have estimated that 68% of specimens taken one day before symptom onset were falsely negative [Reference Kucirka13]. Given that the incubation period is estimated to be around 4–6 days [Reference Böhm10, Reference Linton26], it should be considered to not sample a contact person 1–2 days after exposure to avoid false-negative test results.
Compared with other studies assessing sensitivity and Ct-values [Reference Cevik19], the majority of our cases were in non-hospitalised patients. As the majority of COVID-19 cases are mild cases who do not require hospitalisation [Reference Streeck5, Reference Mizumoto27], our study provides a necessary insight into the sensitivity and viral RNA shedding of milder COVID-19 cases. We obtained these estimates by combining laboratory data on the date of sampling and Ct-values, with notification data on symptoms and symptom onset. In theory, it could be that specimens were matched to the wrong records in the notification data. However, we combined records based on identical values for four variables. Subsequently, only a few records were excluded based on contradictory timing of date of laboratory confirmation and date of notification. Therefore, we believe that the number of specimens that were matched to a different patient remained very low and can be considered negligible on our estimates.
Our data concern Ct-values, and we did not examine the relationship with propagating virus from samples and their Ct-values. An assessment of the infectiousness of these samples was therefore not possible. However, we expect that lower Ct-values are associated with a lower probability of isolating virus in culture [Reference Bullard28–Reference Van Kampen32]. Finally, we assessed the Ct-values using several RNA extraction methods, different PCR platforms and PCR detection systems. Furthermore, specimens have been sampled in different ways.
In conclusion, the probability of detecting viral RNA in respiratory samples in symptomatic COVID-19 patients is highest close to symptom onset and reduced considerably 10 days after symptom onset. The lowest Ct-values of SARS-CoV-2 were found around symptom onset up to three days past symptom onset as depicted by the LOWESS curve. Similar Ct values were found 1–3 days before symptom onset and suggest the potential of pre-symptomatic transmission. Our findings underline the importance of early identification of contact persons to implement timely quarantine, and careful timing of sampling to prevent false-negatives test results.
Supplementary material
The supplementary material for this article can be found at https://doi.org/10.1017/S0950268821001345.
Data availability statement
The data underlying this article will be shared on reasonable request to the corresponding author.