BACKGROUND
The latest World Health Organization (WHO) global estimates report 14·1 million new cancer cases to occur every year, 8·2 million cancer deaths and 32·6 million people living with cancer; these figures are projected to increase to 15·2 million new cases and 8·9 deaths by 2035 [1]. Cancers attributable to infections – which can be targeted by immunization-based primary prevention interventions – account for 16% of this burden [Reference de Martel2]. Cervical cancer is the fourth most common cancer in women, and the seventh overall, with an estimated 528 000 new cases and 266 000 deaths in 2012 [1]. Human papillomavirus (HPV) carcinogenicity has been solidly established for cervical cancer; HPV infection is responsible for virtually all cervical cancers and for a large number of other genitourinary cancers including vulvar, vaginal, penile and anal cancer, as well as for oropharyngeal cancers, resulting in HPV being responsible for a significant proportion of worldwide cancer burden [3–Reference Odone10]. There are more than 100 types of HPV, of which at least 12 have been identified as high-risk oncogenic types and are vaccine targets. HPV types 16 and 18 were classified as carcinogens by the International Agency for Research on Cancer (IARC) in 1995 [11] and are reported to account for approximately 70% of cervical cancers. HPV types 31, 33, 35, 39, 45, 51, 52, 56, 58, and 59 were included in the IARC carcinogens group in 2011 [12] and are reported to account for 30% of cervical cancers [Reference Wheeler13–Reference Castle and Maza17].
The quadrivalent (against HPV types 6, 11, 16, and 18, qHPV) and bivalent (against HPV types 16 and 18, bHPV) vaccines were licensed in the US in 2006 and 2009 and have since been widely introduced in immunization schedules at the global level. In Europe, qHPV is approved for use in males and females from the age of 9 years to protect against precancerous lesions in the cervix, vulva, vagina and anus, cervical and anal cancers and genital warts [18]; bHPV is approved for use in males and females from the age of 9 years to protect against cervix or anus cancers and against precancerous lesions in the genital area [19].
A second-generation HPV 9-valent vaccine (9vHPV) targeting five additional HPV types (against HPV types 6, 11, 16, 18, 31, 33, 45, 52, and 58) was approved in December 2014 by the US Food and Drug Administration (FDA) [20] and granted marketing authorization by the European Commission in June 2015 [21]. In Europe, 9vHPV is approved for use in males and females from the age of 9 years to protect against precancerous lesions and cancers of the cervix, vulva, or vagina and anus, and against genital warts [22].
Currently, 9vHPV is also licensed in Canada, Australia, Chile, and Hong-Kong and, more recently, Ecuador, South Korea, and New Zealand. The US Advisory Committee on Immunization Practices (ACIP) recommended 9vHPV as one of three HPV vaccines that can be used for routine vaccination in February 2015 [Reference Petrosky23] and, from May 2017, after all lots of qHPV have expired, will be the only HPV vaccine available in the USA [24].
The potential public health impact and cost-effectiveness of 9vHPV has been explored by mathematical models under different efficacy, cost, and vaccine coverage scenarios [Reference Boiron25–Reference Mennini30]. As clinical trial data is currently accumulating on 9vHPV efficacy, immunogenicity, and safety in different age groups and study populations, as well as on different dose regimens and co-administrations, no systematic assessment has been conducted so far to pool the available evidence on the topic. The aim of the current study is to systematically retrieve, qualitatively and quantitatively pool, and critically appraise all available evidence from randomized controlled trials (RCTs) on 9vHPV.
METHODS
We conducted a systematic review of the available published evidence on the efficacy, immunogenicity, and safety of 9vHPV, as well as a systematic search of the registered, completed, active, and/or ongoing clinical trials (RCTs) on 9vHPV. The review's methods were defined in advance following the PRISMA (Prepared Items for Systematic Reviews and Meta-Analysis) guidelines [Reference Moher31].
Search methods for studies’ identification
Published studies were identified by searching the electronic databases Medline, Embase and the Cochrane Library. The database search strategies were built around 9vHPV-related free-text key words. The search strategy was first developed for Medline and then adapted for use in Embase and the Cochrane Library. All three search strategies are available as online supplementary material (Table S1). In addition, further studies were retrieved from reference listing of relevant articles and consultation with experts in the field.
Registered clinical trials were identified searching the clinical trials’ registries and platforms: the WHO ICTRP (International Clinical Trials Registry Platform), the ClinicalTrials.gov registry, the Cochrane Central Register of Controlled Trials and the EU Clinical Trial Register (all registries’ search strategies are available as online supplementary material, Table S1).
Inclusion criteria and outcomes
All published clinical trials’ on 9vHPV were included in the systematic review of the literature. All other study designs were considered not eligible for inclusion, neither were reviews or opinion papers. We considered the following primary outcomes: all measures of 9vHPV's clinical efficacy, immunogenicity, and safety in all possible age groups and study populations. Studies published in English through August 25, 2016 were included.
In our systematic search of the registered RCTs, we retrieved all RCT protocols through August 25, 2016 reporting 9vHPV to be administered in any of the RCTs’ arms. All age groups, study populations, comparisons, and dose regimens were included.
Collection and analysis
All identified studies were independently reviewed for eligibility by three authors (P.C., V.C., and A.O.) in a two-step-based process; a first screen was performed based on title and abstract while full texts were retrieved for the second screen. At both stages disagreements by reviewers were resolved by consensus. Data were extracted by two authors (P.C. and V.C.) supervised by a third author (A.O.), using a standardised data extraction spreadsheet. The data extraction spreadsheet was piloted on three randomly selected papers and modified accordingly. Published studies’ data extraction included authors’ name, year of publication, countries of study implementation, study setting, study period, study population, study design, intervention, control, and outcome measures. Data extraction from RCTs’ registered protocols included Trial's title, ClinicalTrials.gov identifier, EudraCT number, sponsor, sponsor protocol number, start date and current status. For each included registered RCT, we retrieved and reported on associated indexed published papers.
We performed a descriptive analysis to report the characteristics of included studies. A synthesis of the studies’ findings was carried out and results summary tables were produced on all considered outcomes. Depending on studies’ heterogeneity, we planned to perform meta-analysis on pre-specified outcomes, including efficacy, immunogenicity, and safety outcomes.
RESULTS
Characteristics of included studies
We identified 227 records by running the pre-defined search strategies on the three selected databases. After removing duplicates, 148 papers were assessed for eligibility by title and abstract. Papers were screened and selected as illustrated in Figure 1. Two papers were published on 9vHPV clinical trials’ protocols, describing the RCTs’ design and rationale but not reporting original data; they were excluded from the review [Reference Luxembourg32, Reference Chen, Gesser and Luxembourg33]. Ten papers met the inclusion criteria and were included in the review.

Fig. 1. PRISMA flowchart of included published papers.
Included papers’ characteristics are reported in Table 1.
Table 1. Characteristics of included published studies, reporting findings from randomized, controlled trial on 9vHPV
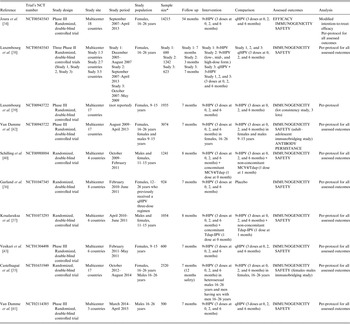
9vHPV, human papillomavirus 9-valent vaccine; 8vHPV, HPV 8-valent vaccine; 5vHPV, HPV 5-valent vaccine; qHPV, HPV quadrivalent vaccine; MCV4, meningococcal A/C/Y/W-135 vaccine; Tdap, tetanus, diphtheria, acellular pertussis vaccine; Tdap-IPV, diphtheria, tetanus, pertussis, and poliomyelitis vaccine; Pap test, Papanicolaou test; Yrs, years; Dose form., dose formulations.
* Subjects who underwent randomization.
Included papers reported findings from eight different clinical trials on 9vHPV's efficacy, immunogenicity, and/or safety. Of them, one paper reported data on 9vHPV clinical efficacy [Reference Joura34], 10 papers reported data on 9vHPV immunogenicity [Reference Joura34–Reference Vesikari43], and nine papers reported data on 9vHPV safety [Reference Joura34–Reference Luxembourg38, Reference Schilling40–Reference Vesikari43].
In particular:
-
• one paper reported the findings of the three Phase II RCTs that were conducted to select the best vaccine formulation to undergo Phase III evaluation [Reference Luxembourg38];
-
• one paper reported preliminary data on 9vHPV clinical efficacy in females aged 16–26 years, as well as on 9vHPV immunogenicity and safety in this population, compared with qHPV [Reference Joura34];
-
• one paper reported data on 9vHPV immunogenicity and safety in males aged 16–26 years, compared with qHPV [Reference Van Damme41];
-
• one paper reported data on immunogenicity and safety of 9vHPV in 9–15-year-old females, compared with qHPV [Reference Vesikari43];
-
• two papers reported on 9vHPV bridging efficacy to 9–15-year-old populations [Reference Van Damme42], 16–26-year-old heterosexual males and men having sex with men (MSM) [Reference Castellsagué35], respectively;
-
• one paper reported the findings of the Phase III trial conducted to evaluate vaccines’ lot consistency [Reference Luxembourg39];
-
• two papers reported data on concomitant administration of 9vHPV with other vaccines, compared with non-concomitant administration [Reference Kosalaraksa37, Reference Schilling40];
-
• one paper reported data on 9vHPV immunogenicity and safety in young females previously immunized with qHPV vaccine [Reference Garland36].
The sections below, together with Tables 2–4, report the synthesis of included RCTs’ findings, by outcome. Meta-analysis of RCTs’ estimates could not be performed due to studies’ heterogeneity in terms of: study design, tested interventions, and comparisons, as well as targeted study populations.
Table 2. HPV 9-valent vaccine clinical efficacy and bridging efficacy findings of included studies

MSM, men-having-sex with men; 9vHPV, human papillomavirus 9-valent vaccine; qHPV, HPV quadrivalent vaccine; GMT, geometric mean titer.
* Intention-to-treat populations (all other estimates are on per protocol populations).
Table 3. HPV 9-valent vaccine immunogenicity findings of included studies

qHPV, human papillomavirus quadrivalent vaccine; GMT, geometric mean titer.
* The P value for non-inferiority was <0·001 for all comparisons HPV 9-valent vaccine vs. qHPV vaccine.
Table 4. The 9vHPV safety findings of included studies; adverse events (AEs, %)

9vHPV, human papillomavirus 9-valent vaccine; qHPV, HPV quadrivalent vaccine.
* The difference in AE incidence between vaccines was statistically significant only for injection-site swelling (P = 0·003).
Dose formulation data
Data from the Phase II dose formulation study were published in June 2015 [Reference Luxembourg38]. The paper reports the findings of three RCTs conducted to compare immunogenicity and safety of seven vaccine candidates with licensed qHPV vaccine. The vaccine candidates were: three dose formulations (low-, mid-, and high-dose formulations) of an 8-valent HPV6/11/16/18/31/45/52/58 vaccine (Study 1), three dose formulations of a 9-valent HPV6/11/16/18/31/33/45/52/58 vaccine (Study 2), and qHPV vaccine concomitantly administered with a 5-valent HPV31/33/45/52/58 (Study 3). All vaccines were administered on a 3-dose schedule at months 0, 2, and 6. Six-hundreds and eighty, 1242 and 623 girls aged 16–26 years were, respectively, randomized in the three studies. Within each study, the primary immunogenicity objective was to demonstrate that month 7 anti four original vaccine HPV types (anti-HPV6/11/16/18) geometric mean titers (GMTs) were non-inferior in subjects who received experimental vaccines compared with subjects in the control group. Non-inferiority was defined as the lower bound of the two-sided 95% CI of the GMT ratio (experimental arm/control arm) being >0·5 for each of anti-HPV types 6/11/16/18. Among all vaccine candidates, the 9vHPV mid-dose formulation containing 30/40/60/40/20/20/20/20/20 mg of HPV6/11/16/18/31/33/45/52/58 virus such as particles, and 500 mg of amorphous aluminum hydroxyl-phosphate sulfate adjuvant was selected for all subsequent Phase III efficacy, immunogenicity, and safety evaluations.
Successful 9vHPV vaccine candidate was reported to: (i) provide non-inferior antibody responses compared with qHPV vaccine with respect to the four HPV types covered by both vaccines (the lower bound 95% CI GMT ratio exceeded 0·5 for all HPV types), (ii) be strongly immunogenic against five additional oncogenic HPV types (>95% subjects seroconverted at month 3), and (iii) be generally well tolerated. Injection-site adverse events (AEs) were slightly higher in the selected 9vHPV vaccine as compared with qHPV (92·4% vs. 90·3%).
Efficacy
The 9vHPV efficacy findings of included studies are summarized in Table 2. One paper has been published on 9vHPV clinical efficacy so far [Reference Joura34]. It reports preliminary data of a Phase III multinational multicenter efficacy study conducted on 14 215 women aged 16–26 years comparing 9vHPV to qHPV. The primary efficacy outcome was the combined incidence of several conditions related to 9vHPV additional HPV types (HPV-31, 33, 45, 52, 58) – with follow-up over a 54-month period. Analysis in the modified intention-to-treat population (which included subjects both not HPV-infected and HPV-infected at the time of vaccination, who received at least one dose of vaccine and for whom there was at least one measurement of efficacy) showed high-grade cervical, vulva, and vaginal disease incidence to be the same in the 9vHPV and qHPV arms, irrespective of HPV testing results (14·0 per 1000 persons-years, risk reduction = 0·7, 95% CI −15·7 to 14·8), while when restricting the analysis to not HPV-infected participants at the time of vaccination, 9vHPV risk reduction was 42·5%; (95% CI 7·9–65·9), this reaching 100% (95% CI 70·4–100) when only considering disease related to 9vHPV HPV types. Analysis conducted on the per-protocol population (subjects who received all three doses of vaccine within 1 year, who were seronegative on day 1 and PCR-negative from day 1 through month 7 and had no protocol violations) reported 9vHPv vaccine efficacy to be 96% or above for all considered clinical outcomes related to HPV types 31, 33, 45, 52, or 58 as well as persistent infection (⩾6 months’ duration, Table 2) [Reference Joura34].
Two published immunobridging efficacy studies inferred 9vHPV efficacy in males and females aged 9–15 years [Reference Van Damme42], in heterosexual males and in MSM aged 16–26 years [Reference Castellsagué35], comparing immunogenicity data between individuals in the intervention arms and 16–26-year-old female controls. By definition, bridging studies generate immunogenicity data to support the extrapolation of data on efficacy or safety obtained under specific circumstances of use (in our case 9vHPV efficacy in 16–26-year-old females) to other situations (e.g. different age groups or different populations) [44]. Both studies reported month 7 GMTs in the intervention arms to be non-inferior (lower bound of the two-sided 95% CI of the GMT ratio >0·67) to the control arm for all 9vHPV vaccine types, as well as seroconversion non-inferiority in more than 99% of study participants (defined as the lower bound of the two-sided 95% CI for the differences in seroconversion rates being >−5 percentage points for each 9vHPV HPV type) (Table 2). Data on 9vHPV immunogenicity MSM aged 16–26 years showed lower antibody response compared with heterosexual males for all tested HPV types (GMT ratios MSM/women for all 9vHPV vaccine types are reported in Table 2) [Reference Castellsagué35].
Immunogenicity
All included articles assessed 9vHPV immunogenicity. The majority of studies adopted a non-inferiority design. Immunogenicity outcomes included intervention–control comparisons of month 7 GMTs for anti-HPV types and proportion of studies’ participants with seroconversion to the 9vHPV vaccine types at 4 weeks after the administration of the third vaccine dose (month 7).
The immunogenicity analyses findings of the dose-formulation Phase II study has been described in the previous section [Reference Luxembourg38] and reference was previously made to the published lot-consistency study – conducted to meet regulatory requirements – that reported three different lots of 9vHPV to elicit equivalent antibody response and seroconversion rates [Reference Luxembourg39]. In addition, data are available on: (i) 9vHPV vs. qHPV immunogenicity [Reference Joura34, Reference Van Damme41, Reference Vesikari43]; (ii) 9vHPV immunogenicity in females aged 16–26-years old vs. other age and gender populations to infer bridging efficacy [Reference Castellsagué35, Reference Van Damme42]; (iii) 9vHPV immunogenicity when co-administrated with other vaccines (including meningococcal, tetanus, diphtheria, pertussis, polio vaccines) [Reference Kosalaraksa37, Reference Schilling40]; and (iv) 9vHPV immunogenicity in subjects previously vaccinated with qHPV [Reference Garland36].
Three studies reported immunogenicity data comparing 9vHPV to qHPV in females aged 16–26 years [Reference Joura34], in females aged 9–15 years [Reference Vesikari43] and in males aged 16–26 years [Reference Van Damme41]. These are summarized in Table 3. They showed: (i) 9vHPV non-inferiority as compared with qHPV with regard to HPV types 6, 11, 16, and 18 in females and males aged 16–26 years (GMT ratios ranging from 0·80 and 1·19 and from 0·89 and 1·23, respectively, Table 3) [Reference Joura34, Reference Van Damme41]; (ii) 9vHPV non-inferiority as compared with qHPV with regard to HPV types 16 and 18 in females aged 9–15 years [Reference Vesikari43]; and (iii) virtually all studies’ participants seroconversion for HPV 31/33/45/52/58 at month 7 (in females aged 9–15 years and 16–26 years and in males aged 16–26 years [Reference Joura34, Reference Van Damme41, Reference Vesikari43]; Table 3).
Two studies have been published on concomitant administration of 9vHPV with other vaccines, such as meningococcal (MCV4), tetanus, diphtheria, pertussis (Tdap) and polio vaccines, reporting non-inferior immune response in subjects receiving 9vHPV concomitantly with other vaccines as compared with non-concomitant administration [Reference Kosalaraksa37, Reference Schilling40]. In particular, authors reported concomitant administration of 9vHPV together with MCV4 and Tdap to elicit non-inferior GMTs and seroconversion rates for all 9vHPV antigens as well as non-inferior immune response and seroconversion to MCV4 and Tdap vaccines’ components as compared with non-concomitant vaccines’ administration in subjects aged 11–15-years old [Reference Schilling40]. Similarly, findings from another RCT showed all 9vHPV HPV types 7 month GMTs and seroconversion non-inferiority in the concomitant group compared with the non-concomitant group. Non-inferiority of immune response was established for diphtheria, tetanus, all pertussis, and polio antigens for both groups [Reference Kosalaraksa37].
One study has been published reporting findings from an RCT that assessed 9vHPV immunogenicity vs. placebo in females aged 12–26, previously vaccinated with qHPV [Reference Garland36]. Seroconversion at month 7 was reported to be >98% for all 9vHPV HPV types, with marked elevations in GMTs. Data from cross-study analysis showed anti-HPV 31/33/45/52/58 GMTs to be lower than in study subjects administered 9vHPV vaccine who had not previously received qHPV vaccine [Reference Garland36].
Safety
Nine papers reported data on 9vHPV vaccine safety; of them, one is the Phase II RCTs conducted to select the right 9vHPV formulation [Reference Luxembourg38], three made comparisons between 9vHPV and qHPV vaccine [Reference Joura34, Reference Van Damme41, Reference Vesikari43], two made comparisons between 9vHPV administered in different age and gender populations (immunobridging studies) [Reference Castellsagué35, Reference Van Damme42], two assessed 9vHPV safety comparing concomitant and non-concomitant administration with other vaccines [Reference Kosalaraksa37, Reference Schilling40] and one assessed 9vHPV safety against placebo in girls previously vaccinated with qHPV vaccine [Reference Garland36]. In five studies, participants recorded injection-site events (AE, within 5 days after vaccination) and systemic events (within 15 days after vaccination) on VRCs (vaccination report cards) [Reference Joura34, Reference Castellsagué35, Reference Luxembourg38, Reference Van Damme41, Reference Van Damme42]. Injection-site AEs were rated by study subjects to be mild/moderate/severe. AEs causality was assessed by investigators and classified as possibly, probably, or definitely vaccine related. Serious AEs were pre-defined as any AE resulting in deaths or in which discontinuation due to AEs was reported. AEs were summarized as frequencies and percentages by study arm and AE type. The 9vHPV safety findings are reported in Table 4. Overall, 9vHPV vaccine recipients were more likely than qHPV vaccine recipients to have AEs related to the injection site (90·7% vs. 84·9% in females aged 16–26) [Reference Joura34], while systemic AEs distribution was similar between intervention and control groups (55·8% vs. 54·9% in female aged 16–26) [Reference Joura34]. Discontinuation rates because of vaccine-related AE were rare and all but one study [Reference Joura34] reported no vaccine-related serious AEs [Reference Joura34, Reference Luxembourg38, Reference Van Damme41, Reference Vesikari43]. In immunobridging studies injection-site or systemic AEs were lower in heterosexual men and MSM aged 16–26 and in males and females aged 9–15 years as compared with controls (females aged 16–26 years) [Reference Castellsagué35, Reference Van Damme42]. In studies assessing concomitant vaccines administration, injection-site AEs of swelling after 9vHPV and Tdap-IPV were more frequent in concomitant administration arms as compared with non-concomitant ones (after 9vHPV: 14·4% vs. 9·4% [Reference Schilling40], after Tdap-IPV 21·7% vs. 31·3% [Reference Kosalaraksa37]); the risk difference between the groups being statistically significant. No other statistically significant differences were reported in terms of AEs after all vaccines’ administration between study arms [Reference Kosalaraksa37, Reference Schilling40]. In both studies, few subjects discontinued because of an AE and no deaths were reported [Reference Kosalaraksa37, Reference Schilling40]. In the placebo-controlled trial conducted in females who previously received qHPV, injection-site AEs were more frequent in the intervention arm (91·1% vs. 43·9) and increased with subsequent 9vHPV vaccine doses, the incidence of serious vaccine-related AEs and discontinuation was reported to be low and no subjects died during the study [Reference Garland36].
Ongoing clinical studies
One hundred and forty records were initially retrieved searching the selected clinical trials’ registries and platforms (Fig. 2). After removing duplicates, 87 RCTs were excluded as they did not include 9vHPV administration in the intervention or control study arms. Twenty-four relevant clinical trial protocols met the inclusion criteria, referring to 16 clinical trials conducted on the 9vHPV vaccine (some RCTs were registered more than once in different RCT registries; Table 5).

Fig. 2. PRISMA flowchart of included registered trials (update August 25, 2016).
Table 5. Existing randomized controlled trials on human papillomavirus 9-valent vaccine

* As reported by ClinicalTrials.gov.
† As reported by European Union Clinical Trials Register.
As of August 25, 2016, 16 Phase II or Phase III multicenter multinational RCTs have been conducted on 9vHPV, the first of which was started in February 2007 [45] while the last was started in August 2016 [46]. Thirteen (81·2%) RCTs are directly sponsored by pharmaceutical companies, two are sponsored by universities [46, 47], one by the US National Cancer Institute [48] and one by the Danish Department of Infectious Diseases [49].
Of the 16 identified RCTs, eight (50%) have been completed, three (18·8%) are active but not recruiting [50–52], four (25%) are ongoing or recruiting [47–49, 53], and one (6·3%) is not recruiting participants yet [46].
For eight RCTs (50%) results or preliminary results have been published in scientific peer-reviewed journals (Tables 1–4, results described in previous sections); one completed RCTs does not have published studies [54].
Of the active RCTs, three are assessing immunogenicity and tolerability of less than three-dose 9vHPV vaccine schedules [46, 47, 51]. The first one – started in December 2013 and no longer anymore – is a 37-month safety and immunogenicity study conducted with the aim of assessing whether investigational two-dose regimens (0, 6 months and 0, 12 months) administered in males and females aged 9–14 years elicit non-inferior immunogenicity and safety profiles as compared with three-dose regimens administered to females aged 16–26 [51]. The second one – started in September 2015, and still recruiting – aims to assess immunogenicity of 9vHPV and bHPV vaccines administered to males and females aged 9–10 years, according to a 0–6 month schedule to infer possible interchangeable use of the two vaccines [47]. The third one – about to start recruiting females aged 9–14 years in Tanzania – will compare immunogenicity between one-dose and two-dose 9vHPV and bHPV vaccines and three-dose regimens of both vaccines [46].
One active RCT is a long-term follow-up study of the first 9vHPV vaccine RCT (NCT00543543), currently being conducted in countries with centralized cervical cancer screening infrastructures (Denmark, Norway, and Sweden) that aims to evaluate the long-term effectiveness, immunogenicity, and safety of 9vHPV vaccine in females aged 16–26 years [52].
Within ongoing European RCTs, one efficacy immunobridging study started in July 2016 and currently recruiting subjects in four European countries aims at assessing immunogenicity and tolerability of the 9vHPV vaccine in females aged 27–45 years compared with females aged 16–26 years [53]. Another RCT – currently recruiting subjects in Denmark – aims at inferring 9vHPV vaccine efficacy in HIV-infected sexually active MSM, measuring changes in the prevalence of HPV types in either anus, oral cavity, or penis as well as well as antibody response [49].
One Phase IIA RCT started in March 2016 aims at assessing immunogenicity of a prime and deferred-booster dosing 9vHPV vaccine schedule among females aged 9–11 years [48]. Primary and secondary objectives are to determine persistence and stability of the immune response to HPV types 16/18 and HPV types 6/11/31/33/45/52/58, respectively, between 6, 12, 18, and 24 months after the prime dose and prior to the administration of the second dose [48].
DISCUSSION
We systematically retrieved and comprehensively summarized all the available published evidence on 9vHPV efficacy, immunogenicity, and safety derived from RCTs, as well as systematically presented an update of the ongoing research on the topic.
To date, 10 papers have been published reporting results or preliminary results from RCTs on 9vHPV, of which nine papers reported results from clinical studies included in 9vHPV clinical development program. Overall, there are 16 registered RCTs on 9vHPV, of which eight are currently active or ongoing.
The 9vHPV clinical efficacy has been directly assessed on females aged 16–26 years in one study that reported 96·7% 9vHPV vaccine efficacy against composite high-grade cervical, vulvar, or vaginal disease related to HPV types 31, 33, 45, 52, and 58 [Reference Joura34]. Efficacy against HPV types 6, 11, 16, and 18 was inferred through a non-inferiority approach that demonstrated 9vHPV non-inferior immunogenicity compared with qHPV. Non-inferiority study design represents the primary tool to demonstrate that a new or reformulated vaccine, or a new regimen of already licensed products, is equivalent to the existing vaccine or current vaccine schedule [Reference Plikaytis and Carlone55, Reference Wang56].
Although it is well known that HPV vaccine efficacy is highest in HPV infection naïve populations and that young age groups are therefore the target group for prophylactic HPV vaccination, 9vHPV clinical efficacy is not directly tested on these populations due to low exposure to HPV but, instead, inferred extending older subjects efficacy data to younger populations (immunobridging efficacy). In fact, adult-to-adolescent, as well as female-to-male published immunobridging studies have allowed inference of 9vHPV efficacy in males and females aged 9–15 years and in males aged 16–26 years. Overall, 9vHPV has been proven to be safe and well tolerated in both females and males in different age groups, with AE profiles similar to that of the qHPV vaccine.
Other RCTs, including currently ongoing ones, address other aspects relevant to the 9vHPV vaccine, including: vaccine co-administration with other vaccines; administration in subjects that previously received qHPV; and 9vHPV efficacy in less than three-dose regimens.
Evidence from completed RCTs has been used to support marketing authorization applications and to inform National Immunization Technical Advisory Groups (NITAGs). After the FDA licensed 9vHPV for use in females aged 9–26 years and in males aged 9–15 years in 2014, it extended its indication to include use in males aged 16–26 years in December 2015. In February 2015, ACIP recommended 9vHPV as one of three HPV vaccines to be used for routine vaccination at age 11 or 12 years as well as for females aged 13–26 and males aged 13–21 years not previously vaccinated. Vaccination is also recommended through age 26 years for MSM and for immunocompromised subjects [Reference Petrosky23]. Medical and scientific associations are progressively including the 9vHPV immunization in their recommendations [Reference Kimberlin, Brady, Jackson and Long57–Reference Crosignani60].
As scientific evidence is accumulating on the 9vHPV vaccine from clinical trials and from other study designs, and as immunization recommendations are built and constantly updated on the basis of their findings, several crucial aspects are to be taken into consideration when trying to forecast the public health impact of universal 9vHPV immunization – to begin with, the broadened protection associated with the five additional HPV types contained in the 9vHPV vaccine. Although some evidence is available on bHPV and qHPV vaccine cross-protection [Reference Herrero61], there is no doubt that extending direct protection to five additional HPV types offers great potential. In fact, in the USA, 10% of invasive HPV-associated cancers (14% for females; 4% for males), 15% of cervical cancers, and 25% of ⩾CIN2 are attributable to the five additional types contained in the 9vHPV [Reference Saraiya62–Reference Hariri64]. Although accurate global estimates are missing, a large amount of data is available on HPV genotype-specific prevalence of cervical cancers and other HPV-related cancers in different settings and study populations [Reference de Sanjose16, Reference Joura65–Reference Serrano70]. This has allowed estimation that 9vHPV vaccine use might expand overall protection against cervical cancer to over 90% [Reference Serrano70, Reference Serrano71], and – in general – significantly reduce the burden of HPV-related disease [Reference Pillsbury29, Reference Largeron72–Reference Serrano75].
For broader roll-out of the 9vHPV vaccine a number of other clinical and organizational aspects are to be considered and are currently being assessed by ongoing research. For instance, long-term follow-up data on 9vHPV efficacy are not yet available. The longest follow-up trial data for bHPV and qHPV vaccines showed them to protect against infection for at least 5 [Reference Naud76] and 9·4 [Reference Villa77] years, respectively. Currently, the longest available follow-up data on 9vHPV reports >90% of vaccinated males and females aged 9–15 to remain seropositive through 2·5 years after third vaccination [Reference Van Damme42]. In the future, an extension of the first 9vHPV efficacy trial, currently ongoing in three Scandinavian countries with an efficient centralized cancer screening program with the aim of monitoring long-term safety, effectiveness, and immunogenicity of 9vHPV, will allow inference of information on duration of effect as well as to track viral-type replacement [52]. Furthermore, the National Cancer Institute is currently running an RCT which will provide evidence on immunogenicity of a prime and deferred-booster dosing schedule among young girls [48].
The number of doses contained in a 9vHPV regimen is another relevant aspect on which research is currently ongoing and whose findings are likely to impact immunization effectiveness. In fact, there is a general interest in simplified HPV vaccine schedules, which reduce required resources, facilitate immunization programs’ implementation and might increase vaccine acceptability and uptake. In Europe, as well as in other countries, two-dose bHPV and qHPV vaccine schedules have been approved for subjects aged 9–14 and 9–13 years, respectively, based on non-inferiority immunogenicity data. Since 2014, WHO has recommended two-dose regimens for subjects younger than 15. With regards to 9vHPV, research on less than three-dose regimens’ efficacy is ongoing and promising preliminary results from one active RCT were presented to ACIP in February 2016 [51, 78]. The study's findings report two-dose regimens in males and females aged 9–14 to elicit no inferior immunogenicity and a safety profile comparable with the standard three-dose regimen in older females [51]. Other dose reduction immunobridging and safety studies will evaluate reduced-dose regimes in low-income countries where the benefits of lower priced and more accessible immunization programmes would be highly beneficial in reducing the burden of HPV-related disease [46].
Another issue to consider is how to manage the transition from qHPV or bHPV vaccines to 9vHPV. The Centers for Disease Control and Prevention has issued a guidance document on the topic [79]. ACIP stated that the 9vHPV vaccine may be used to continue or complete a series started with a different HPV vaccine product, but no formal ACIP recommendations were released for subjects previously fully vaccinated with qHPV or bHPV vaccines who are willing to receive 9vHPV in order to benefit from broader protection. On the contrary, the European summary of product characteristics of the three HPV vaccines states that individuals who received a first dose with a given HPV vaccine should complete the vaccination course with that same vaccine [Reference Van Damme80, 81]. As we have described, one RCT has assessed 9vHPV vaccine for safety and immunogenicity in prior qHPV vaccine recipients and showed it to be well tolerated but to elicit an immune response against HPV types 31/33/45/52/58 lower than in girls who have never been exposed to HPV [Reference Garland36]. Experts recently combined available data with their opinion and judgment and concluded that – considering age at the start of vaccination, the number of doses already received and time interval between doses – 9vHPV might be used to complete an incomplete immunization regimen as well as added to a previous completed schedule to extend protection [Reference Van Damme80].
Last but not the least economic considerations should be mentioned. Several cost-effectiveness exercises projected potential health service savings derived by introduction of universal 9vHPV immunization programmes in different epidemiological settings and under different assumptions of infection transmission, vaccine efficacy, cross-protection, vaccine coverage, and costs. As emerges from different scenarios’ analysis, 9vHPV cost-effectiveness as compared with qHPV will depend on broader protection against HPV types but also on different duration of protection and cost per dose [Reference Drolet28, Reference Kiatpongsan and Kim82–Reference Chesson84].
Our study has both strengths and limitations. With regard to the latter, we could not carry out a quantitative pooling of retrieved findings (i.e. a meta-analysis) due to the heterogeneity of included studies. In the long run, when other studies – including of observational study design – become available, it will be useful to update the current study, possibly including implementation and effectiveness data. Since 9vHPV was licensed, a few narrative reviews and experts opinions have reported and commented on available efficacy, immunogenicity, and safety data, as well as on potential 9vHPV public health impact [Reference Yang and Bracken85–Reference Mariani87]. However, to the best of our knowledge, this has never been carried out in a systematic way. In this systematic review, we provide a comprehensive and critical update on the published available evidence as well as on the present status of ongoing research on relevant clinical and public health aspects of 9vHPV.
CONCLUSION
The new 9vHPV vaccine appears to be non-inferior to other existing HPV vaccines in terms of safety and short-term immunogenicity and efficacy against common HPV types. The inclusion of additional HPV types in the vaccine offers great potential to expand protection against HPV infection and associated disease burden. However, 9vHPV impact in reducing the global burden of HPV-related cancer will greatly depend on vaccine uptake and coverage, availability, and – last but not least – affordability. For this to happen, international and national health authorities should engage in planning, implementing and evaluating effective immunization programmes, as well as invest in increasing the knowledge and awareness of HPV prevention among providers, parents, and people receiving the vaccine.
DECLARATION OF INTEREST
The authors declare no conflicts of interest.
SUPPLEMENTARY MATERIAL
The supplementary material for this article can be found at https://doi.org/10.1017/S0950268817000747
ACKNOWLEDGEMENTS
The authors would like to thank Richard Tran for scientific English editing.