Introduction
Attention deficit hyperactivity disorder (ADHD) is a pervasive neurodevelopmental disorder, which can manifest itself throughout human lifespan and is characterized by symptoms of inattention and hyperactivity/impulsivity. First-line treatment strategy for ADHD comprises psychopharmacological treatments, which can be further optimized with the use of psychosocial interventions. Most adults with ADHD suffer from at least one other psychiatric comorbidity with especially high prevalence of anxiety and mood disorders [Reference Kessler, Adler, Barkley, Biederman, Conners and Demler1]. In terms of somatic disease, sleep disorders are next to asthma and obesity, one of the best documented comorbidities in adults with ADHD [Reference Instanes, Klungsoyr, Halmoy, Fasmer and Haavik2].
A total of 50–70% of adults with ADHD experience sleep disorders [Reference Sobanski3,Reference Yoon, Jain and Shapiro4]. They also report higher daytime sleepiness [Reference Bjorvatn, Brevik, Lundervold, Halmoy, Posserud and Instanes5] and lower sleep quality [Reference Boonstra, Kooij, Oosterlaan, Sergeant, Buitelaar and Van Someren6–Reference Surman, Adamson, Petty, Biederman, Kenealy and Levine9] compared to neurotypical controls. These difficulties have a negative influence on quality of life [Reference Um, Hong and Jeong10] and make management of ADHD and diagnostic differentiation much more challenging, as they result in ADHD-like symptoms [Reference Bioulac, Micoulaud-Franchi and Philip11–Reference Poirier, Gendron, Vriend, Davidson and Corkum13]. However, although verbally reported sleep problems can be robustly linked to ADHD (with effect sizes ranging from medium to very large), there is only very limited evidence from objective sleep measures [Reference Diaz-Roman, Mitchell and Cortese14].
Electroencephalogram (EEG) slowing is a consequence of normal daytime sleepiness in healthy, neurotypical adults [Reference Santamaria and Chiappa15]. It can be used to detect the emergence of fatigue during tasks [Reference Jap, Lal, Fischer and Bekiaris16] and can be reversed by consumption of caffeine [Reference Patat, Rosenzweig, Enslen, Trocherie, Miget and Bozon17]. EEG slowing is a part of normal development, seen in children, adolescents, and young adults as well as part of normal aging, but can also be indicative of neurodegenerative and neurodevelopmental disorders [Reference Britton, Frey, Hopp, Korb, Koubeissi and Lievens18,Reference Petit, Gagnon, Fantini, Ferini-Strambi and Montplaisir19]. EEG slowing is also a sign of excessive daytime sleepiness due to hypoxia typical of sleep disorders such as obstructive sleep apnea (OSA) [Reference Morisson, Decary, Petit, Lavigne, Malo and Montplaisir20,Reference Morisson, Lavigne, Petit, Nielsen, Malo and Montplaisir21], where it has been linked with hypoxemic frontal lobe dysfunction and executive function deficits [Reference Bedard, Montplaisir, Richer, Rouleau and Malo22,Reference Loo, McGough, McCracken and Smalley23]. Measured as theta/beta ratio, EEG slowing is a prominent quantitative electroencephalographic marker in ADHD research [Reference Snyder and Hall24] and considered valuable in predicting response to stimulant medication [Reference Arns, Conners and Kraemer25], but not for diagnostic purposes [Reference Lenartowicz and Loo26]. Multiple studies reported EEG slowing in people with ADHD [Reference Snyder and Hall24,Reference Arns, Conners and Kraemer25,Reference Barry, Clarke and Johnstone27,Reference Michele, Prichep, John and Chabot28] and it has been used a target for neurofeedback treatments in ADHD [Reference Doehnert, Brandeis, Straub, Steinhausen and Drechsler29].
Here, we evaluate daytime sleepiness in adults with ADHD compared to neurotypical controls using observer-rated sleepiness [Reference Wiegand, McClafferty, McDonald and Hanowski30,Reference Wierwille and Ellsworth31] during the Sustained Attention Response Task (SART); and EEG slowing collected before the cognitive testing session during a short period of wakeful rest with eyes open [Reference Morisson, Decary, Petit, Lavigne, Malo and Montplaisir20,Reference Morisson, Lavigne, Petit, Nielsen, Malo and Montplaisir21,Reference Petit, Lorrain, Gauthier and Montplaisir32]. We aim to link sleepiness to ADHD-related cognitive performance deficits to determine the influence of excessive daytime sleepiness on cognitive processing and their relation to symptoms of ADHD. Daytime sleepiness and hypo-arousal are involved in cognitive physiopathology of ADHD [Reference Asherson, Buitelaar, Faraone and Rohde33,Reference Benikos and Johnstone34] and are considered to be some of the major research priorities in the field [Reference Owens, Gruber, Brown, Corkum, Cortese and O’Brien35].
We hypothesize that: (a) adults with ADHD will exhibit elevated levels of both on-task and at-rest sleepiness compared to neurotypical controls; (b) excessive on-task sleepiness will be linked to cognitive performance deficits; and (c) EEG slowing will differentiate adults with ADHD from neurotypical controls.
Methods
Sample
We used data from the OCEAN clinical trial (Oils and Cognitive Effects in Adult ADHD Neurodevelopment, ClinicalTrials.gov Identifier: NCT01750307). Research ethics approval was granted by the National Research Ethics Service Committee London (reference: 11/LO/1042). In total, 111 English-speaking adults volunteered to participate in the study (60 men, 51 women, mean age 32.4 years, SD 10 years, mean IQ = 110, SD = 13). Among them, 81 diagnosed with ADHD according to the DMS-5 criteria (73 of them with combined-type ADHD) [36] and recruited via South London and Maudsley Adult ADHD Outpatient Service, and 30 non-ADHD controls (see Table 1 for detailed characteristics). Participants were either on stable treatment with ADHD medication (stimulant or nonstimulant medication), or on no medication, and could also be taking a low dose of adjunctive medication for depression or anxiety. Participants had no mental or physical comorbidities; however, as a lifetime history of depression is common in adults with ADHD, only participants with recurrent depression or undergoing a depressive episode were excluded. This resulted in including 14 adults with ADHD additionally taking antidepressants.
Table 1. Background, clinical and cognitive variables of the study sample

Abbreviations: CPT-OX, Cued Continuous Performance Task with flankers; PSQI, Pittsburgh Sleep Quality Index; SART, Sustained Attention Response Task.
Clinical and cognitive measures
ADHD symptom severity was measured using the Conners’ Adult ADHD Rating Scales (CAARS) [Reference Conners, Erhardt and Sparrow37], a self-report 18-item scale assessing the level of inattention and hyperactivity/impulsivity consistent with the DSM-5 criteria for adult ADHD [36]. Two subtests (vocabulary and matrix reasoning) of The Wechsler Abbreviated Scale of Intelligence II (WASI-II) [Reference Wechsler38] were used to measure IQ. Participants with IQ below 80 were excluded from the study. Sleep quality was measured using the Pittsburgh Sleep Quality Index (PSQI) [Reference Buysse, Reynolds, Monk, Berman and Kupfer39], a 19-item questionnaire with high validity and reliability in retrospective self-assessment of disturbed sleep quality over the last month, including the ensuing daytime dysfunction [Reference Carpenter and Andrykowski40]. PSQI broadly assess both quantitative (sleep latency, number of awakenings) and qualitative (restlessness, functioning) aspects of sleep.
Cognitive function was measured using the SART and the Cued Continuous Performance Task with flankers (CPT-OX). See Supplementary Material for details of these experimental paradigms. ADHD participants did not take any stimulant medication for at least 48 h prior to cognitive performance test sessions. We recorded commission errors (failure to correctly withhold following a no-go target) and omission errors (OEs) (failure to correctly respond to a go target); as well reaction time variability calculated as the standard deviation (SD) of reaction times.
To evaluate on-task sleepiness during SART, we used video recordings from the cognitive testing sessions and an observer-rated sleepiness assessment protocol [Reference Wiegand, McClafferty, McDonald and Hanowski30,Reference Wierwille and Ellsworth31]. It is a well-established, low-cost, and reliable method of rating sleepiness and that has often been used in studies carried out while the participant is driving a car, which requires vigilance and sustained attention. Participants’ sleepiness was evaluated during the three blocks of the SART on a continuous scale from 0 to 100 and divided into six categories with detailed behavioral descriptions (ranging from “not drowsy” to “extremely drowsy”). A Behavior and Mannerism Checklist [Reference Wiegand, McClafferty, McDonald and Hanowski30] was additionally provided to support quantitative evaluation. Please refer to the Supplementary Material for a detailed description of the Observer-Rated Drowsiness Scale and the Behavior and Mannerism Checklist.
EEG data from the resting-state eyes-open baseline condition was used for this analysis. EEG slowing was calculated as a ratio of the slow frequency bands (delta + theta) to the fast frequency bands (alpha + beta) for each scalp region as well as across regions. This spectral calculation of EEG slowing ensures that the ratio stays sensitive to both simultaneous changes across bands as well as in any individual frequency [Reference Petit, Lorrain, Gauthier and Montplaisir32]. See Supplementary Material for details of EEG recording, pre-processing, and statistical analysis.
Analysis and Results
Observer-rated sleepiness
A main effect of time was found and showed a statistically significant difference in mean on-task sleepiness at the three different time points, F(1.65, 155.50) = 15.018, p < 0.001, partial η 2 = 0.138. Bonferroni-corrected pairwise comparisons between the time points revealed that participants became gradually sleepier as showed by statistically significant t2 – t1 difference (0.119; 95% confidence intervals [CI] 0.050–0.188, p < 0.001) and t3 – t1 difference (0.148; 95% CI 0.064–0.232, p < 0.001).
A main effect of group was also identified and showed a statistically significant difference in mean sleepiness scores between adult ADHD and the control group, F(1, 94) = 12.274, p = 0.001, partial η 2 = 0.115. Bonferroni-corrected pairwise comparisons between groups revealed that participants with ADHD were on average much sleepier than neurotypical participants (mean difference = 0.40; 95% CI 0.173–0.627, p = 0.001). There was no statistically significant interaction between group and sleepiness, F(2, 188) = 1.643, p = 0.19, partial η 2 = 0.017. See Figure 1 for a graphical representation of these results.
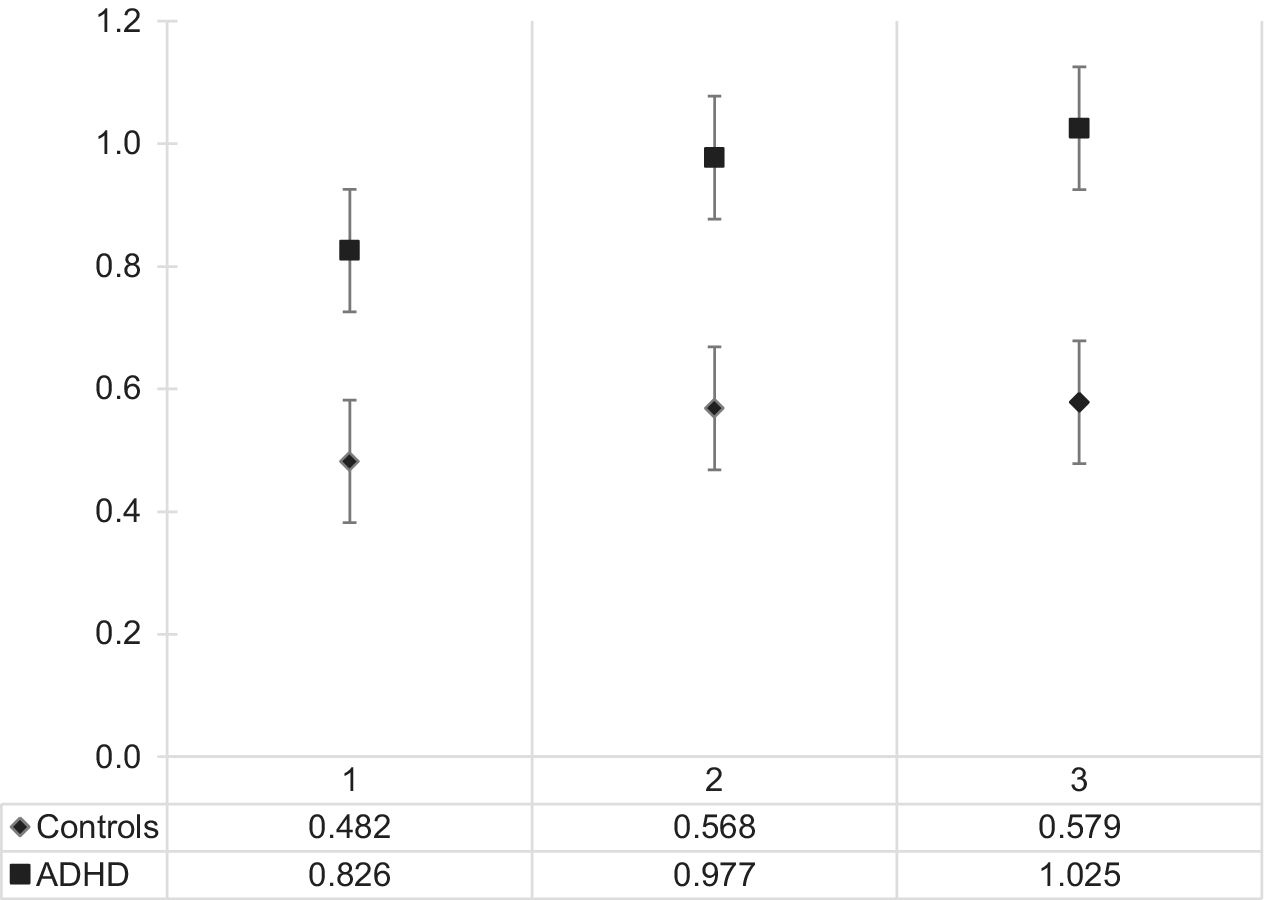
Figure 1. On-task sleepiness (log-transformed) in the adult ADHD and neurotypical group across the three time points. t1 is the 5th minute of the Sustained Attention Response Task, t2 the 10th, and t3 the 15th minute. Abbreviation: ADHD, attention deficit hyperactivity disorder.
As most study participants were not visibly sleepy during the cognitive testing procedure and because the reliability of the observer-rated sleepiness tool is higher at the higher levels of sleepiness, we created an additional group for participants falling into the “at least slightly sleepy” category. See Supplementary Material for details, including analysis of cognitive variables controlled for IQ.
To investigate whether the sleepy group and the ADHD group differ on relevant clinical scales, we compared mean ADHD symptom severity and sleep quality (as measured by PSQI) in the two groups. The analysis showed that sleep quality is slightly lower in the sleepy group (14.03 ± 8.52) compared to the ADHD group (12.88 ± 5.82) and that the ADHD group has a slightly higher ADHD symptom severity (63.64 ± 15.79) than the sleepy group (60.64 ± 24.91), but none of these results were statistically significant.
To further verify whether sleepiness has a detrimental effect on cognitive performance over and beyond ADHD symptoms, we re-analyzed the rate of OEs in the SART task using ADHD symptom severity as a covariate in the model. The result remained statistically significant F(2, 95) = 7.817, p = 0.001, partial η 2 = 0.141; although the adjusted values have changed with the highest error rate still in the sleepy group (OE = 4.88; standard error [SE] = 0.67; 95% CI 3.55–6.22), followed by the neurotypical group (OE = 3.28; SE = 1.04; 95% CI 1.22–5.34), followed by the ADHD group (OE = 1.61; SE = 0.59; 95% CI 0.45–2.78).
Additionally, we run correlations within the ADHD group alone to explore the relationship between observer-rated sleepiness as a continuous variable and the cognitive measures. We did this with and without covarying ADHD symptom severity and IQ. OEs in the SART were strongly correlated with the sleepiness level, r(66) = 0.49, p < 0.001, and the strength of this correlation was independent of ADHD symptom severity, r(66) = 0.45, p < 0.001, or IQ r(66) = 0.44, p < 0.001. Table 2 shows the details on the other cognitive measures.
Table 2. Correlation coefficients between observer-rated sleepiness and cognitive measures

All correlations reported as coefficient (p-value).
Abbreviations: CPT-OX, Cued Continuous Performance Task with flankers; SART, Sustained Attention Response Task.
* p < 0.05.
The multiple regression model statistically significantly predicted OEs in the SART, F(3, 64) = 4.201, p = 0.009. R 2 for the overall model was 16.5% with an adjusted R 2 of 12.5%, a small effect size. Only observer-rated sleepiness added statistically significantly to the prediction, p = 0.004. Regression coefficients and SEs are presented in Table 3.
Table 3. Summary of multiple regression analysis for omission errors in the SART task

Abbreviations: B, unstandardized regression coefficient; SART, Sustained Attention Response Task; SEB, standard error of the coefficient; β, standardized coefficient.
EEG slowing
The main effect of group, F(1,100) = 9.016, p = 0.003, η 2 = 0.083, as well as location, F(2,200) = 3.184, p < 0.001, η 2 = 0.097, and no significant interaction, F(2,200) = 2.610, p = 0.076, η 2 = 0.25, were identified. EEG slowing in all regions was higher in the ADHD versus neurotypical group and was highest in the frontal region in the ADHD group (3.00 ± 1.64), see Figure 2 for details. Prominently, frontal delta was almost twice as high in the ADHD group (1.04 ± 0.43) than in the neurotypical group (0.53 ± 0.62) and statistically significant at Bonferroni-corrected α = 0.004, t(103) = 4.597, p = 0.000012 (see Figure 2 for details).
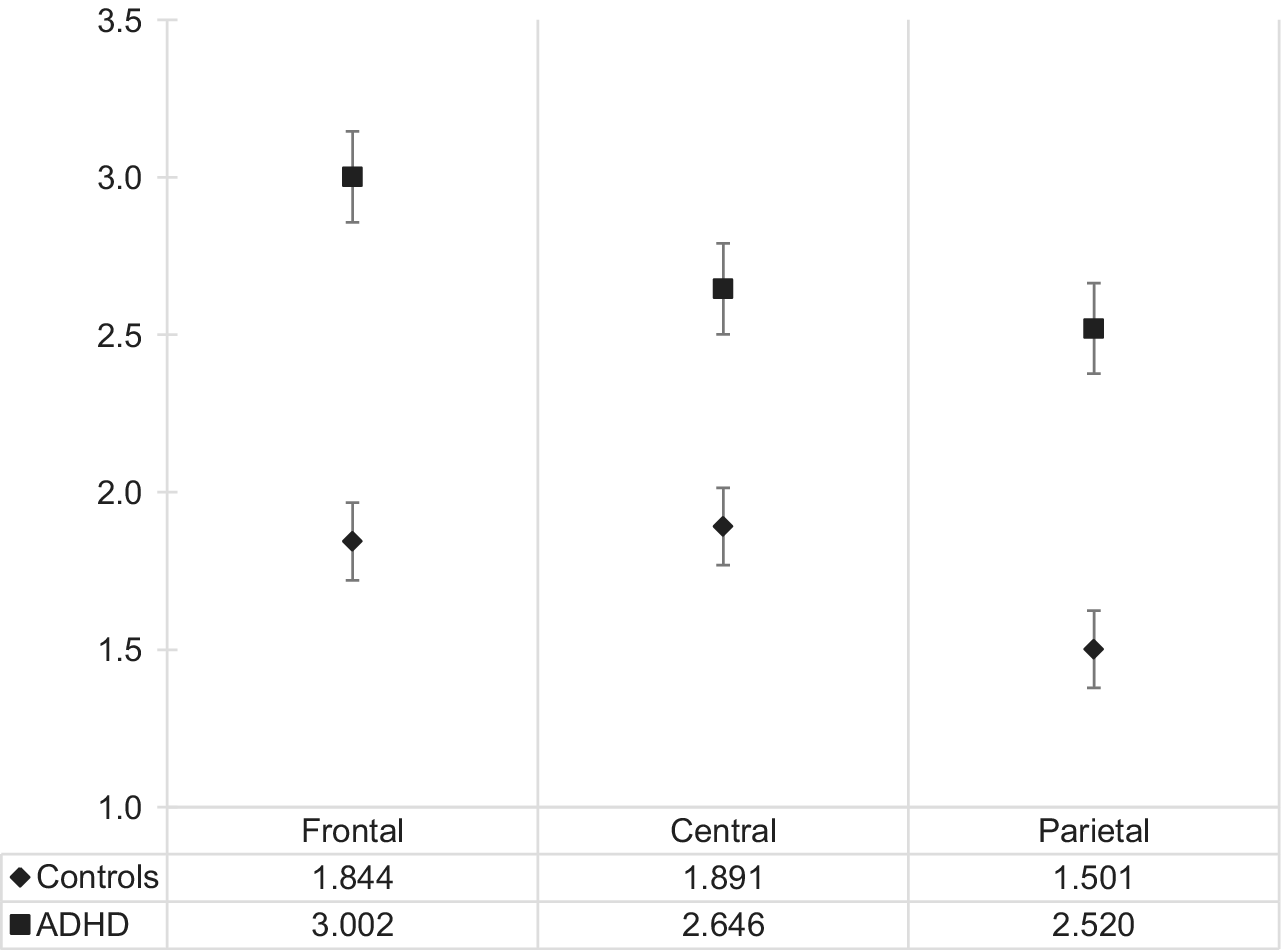
Figure 2. Electroencephalogram slowing in the adult ADHD and neurotypical group across three scalp locations. Abbreviation: ADHD, attention deficit hyperactivity disorder.
EEG slowing was 0.95 (95% CI 0.51–1.39) higher in the ADHD group (2.67 ± 1.56) than in the neurotypical group (1.72 ± .64). This difference was statistically significant, t(92.314) = 4.343, p < 0.001.
Additionally, a statistically significant difference in global EEG slowing between groups was identified when the sleepy group was again separated and included as a third group (see details above), F(2, 58.648) = 9.226, p < 0.001. Importantly, highest EEG slowing was in the ADHD group (2.81 ± 1.76; 95% CI 2.27–3.35), followed by the sleepy group (2.39 ± 1.29; 95% CI 1.91–2.87), followed by the neurotypical group (1.64 ± 0.62; 95% CI 1.35–1.93). The Games–Howell post-hoc tests revealed a statistically significant difference between sleepy and neurotypical groups (0.75; 95% CI 0.09–1.41, p = 0.023) as well as between ADHD and neurotypical group (1.17; 95% CI 0.44–1.90, p = 0.001), but not between ADHD and sleepy group (0.42; 95% CI −0.44 to 1.28, p = 0.473). Although too small of a group for statistical testing, the four neurotypical controls from the sleepy group for whom EEG slowing data was available showed elevated global slowing (2.11 ± 0.66) compared to others from the neurotypical group (1.64 ± 0.62).
There was a small positive correlation between EEG slowing and ADHD symptom severity, r(100) = 0.196, p = 0.049, as well as a large positive correlation between EEG slowing and ADHD diagnostic status rs(109) = 0.762, p < 0.001. The logistic regression model was statistically significant, χ 2(4) = 4.112, p = 0.042. EEG slowing was a statistically significant predictor of ADHD, p = 0.047, as well as ADHD symptom severity, F(1, 100) = 3.978, p = 0.049.
Discussion
Adults with ADHD were much sleepier during the attention task compared to neurotypical controls and they were becoming sleepier as the task progressed. However, they did not get sleepier at a faster rate than neurotypical controls as the task progressed. To investigate whether sleepiness or ADHD plays a larger role in cognitive performance, we created a third group consisting of the sleepiest subjects. This group had the same ADHD symptom severity as the ADHD group. We found that participants in the sleepy group made more OEs in both cognitive tasks (SART and CPT-OX) and had the highest reaction time variability in the SART compared to the non-sleepy ADHD and neurotypical groups even when we controlled for ADHD symptom severity in the analysis.
These findings indicate that the cognitive performance deficits routinely attributed to ADHD and often conceptualized as cognitive endophenotypes of ADHD, are largely due to on-task sleepiness and not exclusively due to ADHD symptom severity. Among the most established of these cognitive performance deficits associated with ADHD are increased OEs and reaction time variability thought to reflect preparation-vigilance deficits [Reference Cheung, Rijsdijk, McLoughlin, Brandeis, Banaschewski and Asherson41]. Here, we show that apart from ADHD symptom severity, these measures are to a significant extent negatively affected by on-task sleepiness. This fits well with some previous findings, where objectively measured sleepiness correlated with OEs but not commission errors in a group of people with narcolepsy [Reference Van Schie, Thijs, Fronczek, Middelkoop, Lammers and Van Dijk42] or where sleep deprivation in adolescents with ADHD resulted in more OEs [Reference Velez-Galarraga, Guillen-Grima, Crespo-Eguilaz and Sanchez-Carpintero43]. Sleepiness is associated with suboptimal arousal [Reference Jackson, Van Dongen, Thorpy and Billiard44] which leads to impairments in cognitive performance [Reference Yerkes and Dodson45]. The cognitive-energetic model of ADHD stressed the role of arousal in neurodevelopmental cognitive performance deficits, suggesting that they emerge from reduced energetic states [Reference Sergeant46]. According to this model the optimization of under-arousal (low energetic state) in ADHD results in reduction of attentional lapses and faster, less variable, and more accurate responses.
Although ADHD participants went through a wash-out phase to ensure elimination of any short-term medication effect, more long-term influence of ADHD medication cannot be excluded. ADHD medication is known to normalize performance measures in people with ADHD [Reference Skirrow, McLoughlin, Banaschewski, Brandeis, Kuntsi and Asherson47]; however, data on long-term effects of stimulant medication are limited. Additionally, the only way to ensure that participants were medication free during the testing session would be to assess the blood concentration of ADHD medication, which we considered too burdensome for our study volunteers.
This is the first paper using video recordings to evaluate on-task sleepiness in ADHD. We have adopted a well-established sleepiness assessment protocol, specifically developed for observer evaluation of naturalistic videos of participant’s head and torso during a cognitive task [Reference Wiegand, McClafferty, McDonald and Hanowski30]. This systematic protocol adopts a highly reliable scale of sleepiness [Reference Wierwille and Ellsworth31], which is in widespread research use outside psychiatry and resulted in strong inter-rater reliability. We recommend our adopted protocol as a low-cost, reliable tool for assessing on-task sleepiness in psychiatric research.
We also found that global quantitative EEG slowing, as well as EEG slowing in all analyzed scalp regions was higher in adults with ADHD versus neurotypical controls and was highest in the frontal region in the ADHD group. This can be indicative of frontal slowing in ADHD. In terms of individual frequency bands, elevated frontal delta was very prominent in the ADHD group. Contrary to our hypothesis, when the sleepy group was added to the analysis, we found that EEG slowing is greater in the non-sleepy ADHD group than in the sleepy group or in the non-sleepy neurotypical group. Importantly, EEG slowing significantly predicted both ADHD diagnostic status, as well as ADHD symptom severity. Overall, this suggests that EEG slowing is more strongly related to ADHD psychopathology than to normal sleepiness in this group (although data from four neurotypical sleepy subjects showed elevated global slowing).
In a recent latent class analysis study with a large (N = 620) sample of children with ADHD, it was found that a sub-group of children with increased delta had a significantly worse cognitive performance relative to all other groups, as measured by OEs in a go/no-go task and reaction-time variability in a spatial working memory task [Reference Loo, McGough, McCracken and Smalley23]. Elevated delta has also been linked to reduced resting state connectivity in the default-mode network of the brain [Reference Luchinger, Michels, Martin and Brandeis48], which is a prominent feature of ADHD [Reference Posner, Park and Wang49].
According to the Developmental Origins of Health and Disease Hypothesis (DOHaD) [Reference Smith, Schmidt-Kastner, McGeary, Kaczorowski and Knopik50,Reference Wadhwa, Buss, Entringer and Swanson51], our results could be interpreted as a residue of developmentally early ischemic/hypoxic events. According to DOHaD, deficient prenatal blood and oxygen supply results in lowered weight at birth, leading to an enhanced risk of developing ADHD [Reference Smith, Schmidt-Kastner, McGeary, Kaczorowski and Knopik50,Reference Wadhwa, Buss, Entringer and Swanson51]. EEG slowing and the elevated frontal delta could perhaps serve as indicators of these altered neurodevelopmental pathways in adults with ADHD.
EEG slowing with elevated frontal delta is also characteristic of people with OSA and can be attributed to the hypoxia apneic patients experience during sleep [Reference Morisson, Decary, Petit, Lavigne, Malo and Montplaisir20]. A similar delta increase has been found in healthy awake subjects with hypoxia experimentally induced by gas inhalation [Reference Saletu, Grunberger, Anderer, Linzmayer and Konig52]. Children suffering from OSA-hypopnea syndrome (OSAHS) with comorbid ADHD have a significantly higher hypoxia than children with OSAHS alone [Reference Wu, Gu, Chen, Chen, Ni and Xu53]. Crucially, converging evidence now exist suggesting that pre- and perinatal hypoxia is a major environmental pathogen for ADHD with the common risk factors associated with ADHD, such as low birth weight, prematurity, obstetric complications, and maternal smoking, all strongly linked to hypoxia-ischemia [Reference Smith, Schmidt-Kastner, McGeary, Kaczorowski and Knopik50,Reference Zhu, Gan, Huang, Li, Qu and Mu54]. Animal models suggest that pre-, peri-, or neonatal ischemia-hypoxia results in neurocognitive and behavioral deficits lasting into adulthood [Reference Miguel, Schuch, Rojas, Carletti, Deckmann and Martinato55,Reference Rehn, Van Den Buuse, Copolo, Briscoe, Lambert and Rees56]. Hypoxia results in neuroinflammation which has been linked to ADHD diagnosis and neurocognitive deficits related for ADHD [Reference Oades57,Reference Oades, Myint, Dauvermann, Schimmelmann and Schwarz58]. Taken together, this body of evidence gives room for speculation that EEG slowing and the elevated frontal delta in adults with ADHD might be a residue of either developmentally early or sleep-related hypoxic events.
Based on the results presented here and some recently published findings [Reference Bijlenga, Vollebregt, Kooij and Arns59], we would like to propose a simple working hypothesis that daytime sleepiness plays a major role in cognitive functioning of adults with ADHD. This will come to no surprise to clinicians, who consistently appreciate sleepiness as an integral part of the disorder [Reference Bioulac and Philip60]. However, the experimental neuro-cognitive literature on adult ADHD leaves an impression that sleepiness is a rather peripheral phenomenon and that the cognitive deficits related to ADHD should be attributed to state-independent neurocognitive deficits.
Sleep plays a key role in restoring brain function responsible for higher-order cognition [Reference Nofzinger61]. Functional neuroimaging studies showed that sleep deprivation reduces global cerebral metabolism, especially in fronto-parietal cortex and thalamus [Reference Thomas, Sing, Belenky, Holcomb, Mayberg and Dannals62,Reference Thomas, Sing, Belenky, Holcomb, Mayberg and Dannals63], as well as reduces the hemodynamic response in the dorsolateral prefrontal cortex and bilateral posterior parietal cortices [Reference Mu, Nahas, Johnson, Yamanaka, Mishory and Koola64]—regions significantly overlapping with the attentional networks of the brain. Moreover, the extent of such reductions is larger in people with higher susceptibility to sleep deprivation [Reference Mu, Mishory, Johnson, Nahas, Kozel and Yamanaka65]. Therefore, a simple brain mechanism underlying sleep deprivation and the resulting daytime sleepiness in ADHD emerges. As adults with ADHD are more severely sleep deprived compared to neurotypical control subjects [Reference Bjorvatn, Brevik, Lundervold, Halmoy, Posserud and Instanes5] and are more vulnerable to sleep deprivation [Reference Bioulac, Micoulaud-Franchi and Philip11], in various neurocognitive tasks, they should manifest larger sleepiness-related reductions in cognitive performance. Many of the cognitive performance deficits might be linearly related to the level of daytime sleepiness.
This circadian-dependent pattern of arousal in ADHD [Reference Bijlenga, Vollebregt, Kooij and Arns59] could exacerbate many of the impairments linked with ADHD. For example, sleep deprivation could be exacerbated by educational demand of early morning wakefulness [Reference Roberts, Roberts and Duong66], which over time can lead to increased mental health problems [Reference Roberts, Roberts and Duong66,Reference Altevogt and Colten67]. Additionally, evening hyperarousal interferes with falling asleep despite high sleep drive [Reference Cluydts, De Valck, Verstraeten and Theys68], leading to a vicious circle of sleep deprivation in ADHD [Reference Brown and McMullen69]. This might also explain why morning bright light therapy seems to be promising in ADHD resulting in phase advance in circadian preference and reduction of symptoms [Reference Rybak, McNeely, Mackenzie, Jain and Levitan70].
One clear testable prediction of the working hypothesis would be that carefully controlling for sleepiness, time of day and/or individual circadian rhythms, would result in substantial reduction in the neurocognitive deficits in replications of classic ADHD studies. This might possibly lead to identification of specific areas of cognition that are influenced by excessive daytime sleepiness to a different extent, which would enable a more precise specification of the state- and context-independent nature of cognition in adults with ADHD.
Acknowledgments.
The authors thank the National Adult ADHD Clinic at the South London and Maudsley Hospital (SLaM) and all study participants.
Financial Support.
The OCEAN study was funded by Vifor Pharma (PADWUDB), awarded to P.A. with King’s College London as sponsor. B.H. was supported by the European Union’s Horizon 2020 research and innovation program under the Marie Sklodowska-Curie grant agreement no. 643051. P.A. was supported by NIHR Biomedical Research Centre for Mental Health, NIHR/MRC (14/23/17), Action Medical Research (GN 2315), and European Union (643051, 602805, and 667303). This study reflects the authors’ views and none of the funders holds any responsibility for the information provided.
Conflict of Interest.
P.A. has received funds for consultancy on behalf of KCL to Shire, Eli-Lilly, and Novartis, regarding the diagnosis and treatment of ADHD; educational/research awards from Shire, Eli-Lilly, Novartis, Vifor Pharma, GW Pharma, and QbTech; speaker at sponsored events for Shire, Eli-Lilly, and Novartis. All funds are used for studies of ADHD. The other authors report no conflicts of interest.
Data Availability Statement.
The datasets generated during and analyzed during the current study are available from the corresponding author on reasonable request.
Supplementary Materials.
To view supplementary material for this article, please visit http://dx.doi.org/10.1192/j.eurpsy.2020.28.
Comments
No Comments have been published for this article.