Introduction
Chemotherapy is a form of drug therapy meant to kill fast-growing tumour cells by powerful chemicals in the body. 5-Fluorouracil (5-FU) is one of the most commonly used chemotherapy drugs during clinical treatment of cancers in gastrointestinal tract, pancreas, ovary, oesophageal, colorectal and breast since 1957 (Refs Reference Ng1–Reference Vodenkova7). However, it has various side effects, such as long-term memory impairments, myelosuppression and cardiotoxicity (Refs Reference Muhammad8–Reference Ishibashi13). With the continuous development of modern oncology and pharmacology, a series of 5-FU derivatives and analogues, including 1-hexylcarbamoyl-5-fluorouracil, tegafur, uracil tegafur, capecitabine, TAS-102 (trifluridine-tipiracil) and S-1 (tegafur, gimeracil and oteracil potassium) have been developed, which could increase and maintain higher 5-FU concentration in the serum (Refs Reference Wang14–Reference Wormann18).
It is currently believed that 5-FU and its derivatives exert their anti-tumour effect mainly through inhibiting thymidylate synthase (TS) and incorporating its metabolites into DNA and RNA (Ref. Reference Baba19). After administration, most of the 5-FU is metabolised into inactive dihydrofluorouracil by the rate-limiting enzyme dihydropyrimidine dehydrogenase (DPD) in the liver (Ref. Reference Lee20). The rest of the administered 5-FU is transformed mainly into active metabolites of fluorodeoxyuridine monophosphate (FdUMP), fluorodeoxyuridine triphosphate (FdUTP) and fluorouridine triphosphate (FUTP). FdUMP binds with TS and the methyl donor, folate 5,10-methylenetetrahydrofolate to inhibit the normal function of TS. This ternary complex blocks deoxyuridine monophosphate binding to TS and inhibits synthesis of deoxythymidine monophosphate (dTMP). Since dTMP is a key substrate for the production of deoxythymidine triphosphate (dTTP), depletion of dTMP results in the subsequent depletion of dTTP, and induces perturbations in the levels of other deoxynucleotides (dATP, dGTP and dCTP), disrupting DNA synthesis and repair. Meanwhile, inhibition of TS also results in an increased level of dUTP, which along with the FdUTP can be misincorporated into DNA, leading to breaking of DNA strands (Ref. Reference Longley21). Additionally, the 5-FU metabolite FUTP is also incorporated extensively into RNA, disrupting normal RNA processing and function (Ref. Reference Machon22). Therefore, 5-FU severely disrupts synthesis, repair and function of nucleic acids through its active metabolites, inhibiting growth of cells and killing tumour cells ultimately. However, 5-FU also impairs cellular metabolism and viability in normal cells, which underlies the developmental and reproductive toxicities (Refs Reference Shuey23–Reference Kumar25).
To date, several attempts have been made to alleviate the reproductive side effects of 5-FU. Apart from the combined administration of 5-FU with other drugs such as triptorelin, 6-alkylguanine-DNA alkyltransferase (AGT) and iridoids-rich containing fraction of Pentas lanceolata leaves (IFPL) (Refs Reference Wang26–Reference Fahmy28), manipulation of gene expression including down-regulation of the uracil-DNA glycosylase (UNG) or overexpression of DPD and TS homologues also alleviates the reproductive toxicity (Refs Reference Kumar25, Reference Kim29). Moreover, targeted delivery and controlled release of 5-FU by biocompatible and biodegradable particles represents a promising direction to overcome the side effects of 5-FU (Ref. Reference Xu30). 5-FU has a narrow therapeutic window, and for this reason, selection of the appropriate dosage is crucial to reduce its side effects. In this context, the test of single-nucleotide polymorphisms (SNPs) shows great potential in optimising 5-FU dosage. In patients with DPYD variants (DPD coding genes) of c.190511G > A (rs3918290), c.1679T > G (rs55886062), c.2846A > T (rs67376798) and c.1129-5923C > G (rs75017182), lower dosage of 5-FU inhibits cancer cell growth and achieve equal anti-tumour efficacy (Ref. Reference Amstutz31). Similarly, SNPs in 5-FU metabolic genes such as CDA, CES2, TYMS (TS) and MTHFR can also be used to predict the efficacy and toxicity of capecitabine-based therapy (Ref. Reference Lam32). Therefore, genetic SNPs provide pharmacogenomics information for clinical application of 5-FU to adjust dosage, improve efficacy and reduce side effects, ultimately. However, it should be noted that though increasing efforts to alleviate reproductive toxicity of 5-FU, currently, there is no effective way to avoid the damage completely.
Adverse effects of 5-FU on female reproduction
In Caenorhabditis elegans, 5-FU induces germ cell death and inhibits embryonic and larval development (Ref. Reference Kim and Shim33), which presumably because of cell-cycle arrest and apoptosis of germline cells (Ref. Reference Kumar25). Particularly, 5-FU down-regulates expression of several collagen genes, which are important players in extracellular matrix (ECM)–receptor interaction and focal adhesion. Therefore, impairment of the ECM–receptor interaction and focal adhesion during germ cell development may underlie the 5-FU-induced fertility decline in C. elegans (Ref. Reference Si Zhang34). 5-FU also down-regulates LIN-29, which is an important transcription factor that affects vulva development and egg laying system (Ref. Reference Kumar25). Interestingly, down-regulation of UNG could alleviate the 5-FU effects on embryo hatching (Ref. Reference Kumar25), suggesting that UNG-mediated removal of the misincorporated 5-FU is involved in this process. Indeed, it was proposed that UNG-1 excise uracil, but the subsequent repair synthesis results in uracil reincorporation, leading to futile cycling of the base excision repair pathway (Ref. Reference Seiple35). Overexpression of the homologues of DPD (DPYD-1) and TS (Y110A7A.4) in C. elegans prevented the death of germ cells following 5-FU exposure. In contrast, depletion of DPYD-1 increased sensitivity of 5-FU and depletion of the 110A7A.4 resulted in severe embryonic lethality (Ref. Reference Kim29) (Table 1). Therefore, down-regulation or overexpression of certain 5-FU metabolic enzymes could be an alternative way to reduce the reproductive toxicity.
Table 1. Adverse effects of 5-FU on female reproduction
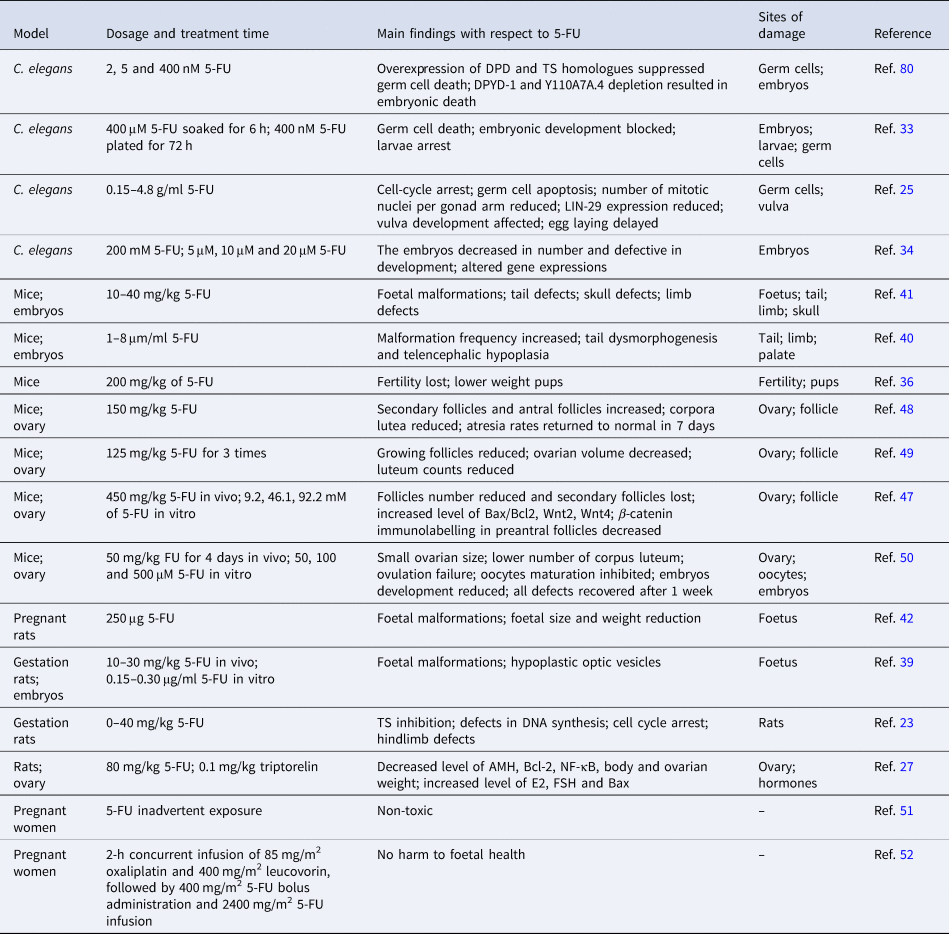
The mammalian fertility cycle is responsible for the coordination of various cellular events, including DNA synthesis in ovarian follicle cells, and of potential importance to the toxicity of 5-FU (Refs Reference Hrushesky36, Reference Meistrich37). Female mice received 5-FU during the oestrous phase were suffered from greater fertility loss compared with those exposed to 5-FU during the metestrus, diestrus and proestrus stages, probably because ovarian follicular DNA synthesis is most active within the oestrous phase and 5-FU leads to pronounced DNA damage accumulation (Refs Reference Hrushesky36, Reference Hirshfield38). Therefore, it is likely that choosing an appropriate oestrus cycle for 5-FU treatment is helpful to reduce reproductive damage.
Teratogenesis is another severe reproductive abnormality induced by 5-FU. The number of externally malformed foetuses increases in a dose-dependent fashion after single administration of 10–30 mg/kg 5-FU during pregnancy in rats (Ref. Reference Kuwagata39). Studies using in vitro whole embryo culture systems also demonstrated that 5-FU dose-dependently induces tail and hindlimb bud defects, and leads to hypoplastic optic vesicles in rat embryos (Refs Reference Kuwagata39, Reference Grafton40). Similarly, 5-FU exposure reduces embryo implantation rate and increases embryo deformity and mortality in a dose- and time-dependent manner in mice (Ref. Reference Dagg41). Of note, when injected into pregnant mice, 5-FU is incorporated into the embryos and accumulates mostly in RNAs. Although varied among different strains, the incorporation amount of 5-FU is positively correlated with the weight of the embryos (Ref. Reference Dagg CP and Offutt42). These findings collectively indicates that negative effects of 5-FU on embryonic development is closely related to its dosage, which in turn suggests that it is crucial to apply an optimal dosage that has expected anticancer activity but does not induce significant developmental defects (Refs Reference Arshad43, Reference Beumer44). In this context, the mouse embryonic stem cell test seemed extremely useful to assess the developmental toxicities and determine the optimal dosage of 5-FU (Ref. Reference van Oostrom45).
Cytotoxicity of 5-FU potentially contributes to the ovarian dysfunction and puts the patients at risk of menopause-related complications and infertility (Ref. Reference Marhhom and Cohen46). When young C57BL/6J female mice were injected with 5-FU, secondary follicles were lost totally (Ref. Reference Almeida47). Furthermore, genes involved in apoptosis and Wnt signalling pathways were significantly up-regulated when ovaries from young mice were cultured in vitro with 5-FU (Ref. Reference Almeida47). In adult mice, administration of 5-FU-induced atresia of secondary and antral follicles, and profoundly reduced corpus luteum counts, leading to a decreased ovarian volume (Refs Reference Lambouras48, Reference Stringer49). However, primordial or primary follicles were not affected by the 5-FU treatment (Refs Reference Lambouras48, Reference Stringer49), suggesting that the reproductive toxicity of 5-FU could be recovered with continuous growth of the follicles. In support of this view, we recently reported that multiple intraperitoneal administration of 5-FU in adult female mice resulted in small ovarian size and reduced number of corpus luteum, and led to ovulation failure. However, these defects could be recovered and no obvious abnormality was observed in their offspring, suggesting that the adverse effects could be reversed following withdrawal of 5-FU administration (Ref. Reference Naren50). In addition to self-recovery, combined administration of triptorelin, a GnRH agonist often used as a hormone responsive anti-cancer drug, alleviates 5-FU-induced follicle number reduction, probably via decreasing the levels of E2, follicle-stimulating hormone (FSH), Bax and nuclear factor (NF)-κB, and increasing the levels of anti-Müllerian hormone (AMH) and Bcl-2 in the serum (Ref. Reference Wang26). Additionally, when 5-FU was loaded in poly-glucono-δ-lactone particle and delivered precisely to the target sites and released in a controlled manner, the toxic effect on non-cancer cells was effectively avoided (Ref. Reference Xu30).
In human clinical cases, inadvertent exposure to 5-FU (Ref. Reference Kopelman and Miyazawa51) or treatment with FOLFOX, a mixture of 5-FU, leucovorin and oxaliplatin, during the second and third trimesters of pregnancy had no harm to foetal health (Ref. Reference Jeppesen and Osterlind52). However, the developmental and reproductive toxicities of 5-FU cannot be evaluated comprehensively in humans and are assessed instead in human-induced pluripotent stem cells (hiPSCs), which has been suggested to achieve similar findings (Ref. Reference Aikawa53). In hiPSCs, 5-FU inhibited neural differentiation via down-regulating expression of the mitochondrial fusion proteins Mfn1/2 and decreasing intracellular ATP levels (Ref. Reference Yamada54), suggesting that 5-FU-induced mitochondrial dysfunction may underlie the developmental and reproductive toxicities.
Adverse effects of 5-FU on the reproduction and development were also reported in amphibian, arthropods and aquatic species. Exposure to 5-FU at environmentally relevant concentrations during the early developmental stage did not adversely affect the survival or behaviour in larval zebra fish, but larvae growth represented by body length was significantly increased when exposed to higher concentration of 5-FU (Ref. Reference Ng55). In Xenopus laevis embryos, malformations in abdominal oedema, axial flexure, head, eyes, gut and heart were observed after 5-FU treatment (Ref. Reference Anderson56). Moreover, 5-FU treatment resulted in reduction in the number of offspring and DNA damage in Ceriodaphnia dubia (Ref. Reference Russo57).
In general, 5-FU inhibits embryonic or larval development in C. elegans, arthropods, amphibian, mouse and rat, and dose-dependently induces embryonic malformation in mice and rat. Of note, 5-FU impairs ovarian function and leads to ovulation failure, but the negative effects could be reduced by combinatorial drug administration or eliminated naturally after 5-FU withdrawal in mice. However, the underlying mechanisms remain largely unknown and the potential long-term effect could not be ruled out.
Side effects of 5-FU on male reproduction
Usually, chemotherapy is toxic for testicular tissue and increases the risk of infertility in males (Ref. Reference Delessard58). Studies in mice demonstrated that 5-FU induces morphological changes of Sertoli cells and reduces weights of reproductive organs including seminal vesicle and prostate. This effect is probably mediated by hormonal imbalance in the serum, whereby the levels of GnRH and pro-alpha C were remarkably increased, whereas the levels of testosterone, activin A, prolactin and inhibin B were significantly decreased (Table 2) (Ref. Reference Horii59). 6-Mercaptopurine (6-MP) is an antimetabolite drug as the 5-FU, which induces ROS, activates caspase 3 and promotes apoptosome generation, ultimately leading to the loss of Leydig cells in mice (Ref. Reference Lynch60). Therefore, it is tempting to speculate that whether 5-FU also impairs the Leydig cells that produce testosterone through similar mechanism.
Table 2. Adverse effects of 5-FU on male reproduction
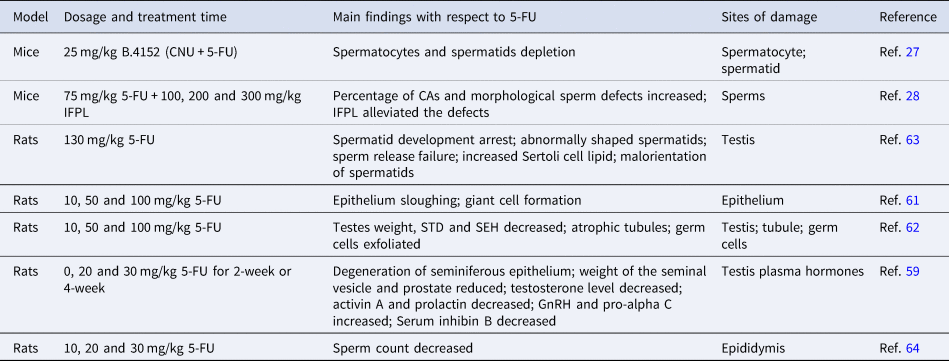
In male rats, 5-FU induces sloughing of epithelium and promotes giant cell formation (Ref. Reference Narayana61), accompanied by a significant decrease of the testis weights (Ref. Reference D'Souza and Narayana62). Moreover, tubular shrinkage, atrophy and abnormal sperm cells were also observed following 5-FU administration (Refs Reference D'Souza and Narayana62, Reference Russell and Russell63), eventually leading to a significant reduction of spermatocytes/spermatids cell count in a dose- and time-dependent manner (Ref. Reference D'Souza64). Although direct mechanistic evidence is missing for 5-FU-induced reproductive toxicity in males, 6-MP has been reported to induce DNA damage in rat spermatocytes (Ref. Reference Habas65), suggesting that 5-FU may potentially induce DNA damage in spermatocytes. Moreover, 5-FU-induced swelling and crazing of tubules (Ref. Reference Ghafouri-Fard66) is reminiscent of the alkylating agent cisplatin-induced degenerative changes in seminiferous tubules and germ cell depletion, mediated by aggravated oxidative damage (Refs Reference Ghafouri-Fard66, Reference Yadav67). Therefore, it will be interesting to test whether 5-FU-induced abnormal seminiferous tubules is also because of the free radical-associated oxidative stress.
Importantly, combinatory use of certain agents also relieves the side effects of 5-FU on male reproduction. N-2-Chloroethyl-N-nitrosourea (CNU) is an alkylating agent often used in combination with 5-FU against a range of cancers (Ref. Reference Mitchell and Schein68). The B.4152, composed of 5-FU and CNU, only induces minor damage in spermatogenic tissue in mice when administrated with AGT. AGT is a DNA repair protein that repairs mutagenic lesions in DNA and protects testis from alkylating agent-induced damage (Ref. Reference Thompson27). Another agent IFPL, which is the iridoids-rich fraction of P. lanceolata leaves, also plays protective role during 5-FU-induced sperm defects (Ref. Reference Fahmy28). Furthermore, several extracts from medicinal plants and herbs possess antioxidant, anti-inflammatory or anti-oedematous activities, and potentially protect sperm from inflammation and oxidative stress induced by chemicals such as 5-FU, and reduce the adverse effects (Refs Reference Tahvilzadeh69, Reference Diab70).
The report regarding the effects of 5-FU on human male reproduction is extremely limited, but some other chemotherapy agents have been reported to exert non-negligible adverse effects. Cisplatin treatment resulted in a remarkable reduction of the number of germ cells both in human foetal and prepubertal testis, which involves an initial loss of gonocytes followed by a significant reduction in spermatogonia (Ref. Reference Tharmalingam71). Paclitaxel, a taxane-based chemotherapy drug, reduced serum inhibin B and testicular volume, while elevated serum FSH level in male patients (Ref. Reference Chatzidarellis72). Therefore, it is reasonable to speculate that 5-FU, as a chemotherapeutic agent, also negatively affects male reproduction. Given the current status that lacking mechanistic studies of the 5-FU-induced reproductive toxicities in males, the integrated multi-organoid body-on-a-chip system containing male reproductive organoid would be a desirable model to systematically investigate the potential mechanisms of 5-FU toxicity on male reproduction (Refs Reference Skardal73, Reference Rajan74).
Conclusion and perspectives
Collectively, 5-FU causes reproductive and developmental toxicities mainly via disrupting cellular functions and inducing hormonal imbalance. The adverse effects could be alleviated by combinatory administration of certain agents or eliminated naturally following 5-FU withdrawal, probably because 5-FU has minor gonadal toxicity compared with other chemotherapy agents and induces a lower degree of gonadal damage (Ref. Reference Oktem75). However, long-term reproductive effect of 5-FU treatment is still under debates and further in-depth evaluation including systematic analysis of health condition and life span of the descendants should be performed. Given the superior advantages of nanocarriers in drug delivery and release, it will be crucial to develop novel, efficient nanocarriers to reduce the reproductive and developmental toxicities of 5-FU (Refs Reference Paroha76–Reference Patra79). Moreover, metabolic and multiomics analysis combined with organoid studies will be a promising strategy to elucidate mechanistic details of the 5-FU-induced reproductive toxicities.
Acknowledgements
We are grateful to all members of Buhe Nashun lab for stimulating discussions. Apologise to all colleagues whose work could not be cited because of the space constraints. This study is funded by the National Natural Science Foundation of China (31970759), the National Natural Science Foundation of China (32160145), the National Natural Science Foundation of China (31760335), the Fund for Excellent Young Scholars of Inner Mongolia (2021JQ04) and the Science and Technology Major Project of Inner Mongolia Autonomous Region of China to the State Key Laboratory of Reproductive Regulation and Breeding of Grassland Livestock (2019ZD031).
Conflict of interest
The authors declare that they have no conflict of interest.