1. Introduction and objective
During Late Cretaceous and Palaeogene times, the continental interior of Mexico was affected by the Mexican Orogeny (a term recently introduced by Fitz-Díaz et al. Reference Fitz-Díaz, Lawton, Juárez-Arriaga and Chávez-Cabello2018), an orogenic activity also known as the Laramide sensu lato (e.g. Garduño-Martínez et al. Reference Garduño-Martínez, Pi Puig, Solé, Martini and Alcalá-Martínez2015). The Mexican Orogen is a large tectonic province that has a spectacular topographic expression in the Sierra Madre Oriental and the Sierra Madre del Sur (Fig. 1). The shortening deformation encompassed the entire modern crustal domain lying between the Pacific Ocean and the Gulf of Mexico. This orogen has a length of more than 2000 km and is hundreds of kilometres wide along a territory that extends northward from the Tehuantepec Isthmus in Oaxaca to northeastern Sonora (Fig. 1). The geological structures in the foreland of the Mexican Orogen have a general NW–SE trend in central Mexico that changes to E–W in northeastern Mexico at the Monterrey salient and back again to NW–SE in northwestern Mexico at the Torreón re-entrant (Fig. 1). Several authors (e.g. Campa & Coney, Reference Campa and Coney1983; Nieto-Samaniego et al. Reference Nieto-Samaniego, Alaniz-Álvarez, Silva-Romo, Eguiza-Castro and Mendoza-Rosales2006; Cerca et al. Reference Cerca, Ferrari, López-Martínez, Martiny and Iriondo2007; Cuéllar-Cárdenas et al. Reference Cuéllar-Cárdenas, Nieto-Samaniego, Levresse, Alaniz-Álvarez, Solari, Ortega-Obregón and López-Martínez2012) suggested that these structures are associated with either Sevier or Laramide orogenic events that occurred in the United States. Following Fitz-Díaz et al. (Reference Fitz-Díaz, Lawton, Juárez-Arriaga and Chávez-Cabello2018), the kinematics, chronology and deformation style of thick-skinned structures of the Mexican Orogen may be correlated with those belonging to the Laramide structures of the United States. Most previous geochronological studies about the timing of the shortening across the Mexican Orogen were performed in the Sierra Madre Oriental physiographic province (Gray et al. Reference Gray, Pottorf, Yurewicz, Mahon, Pevear, Chuchla, Bartolini, Buffler and Cantú-Chapa2001; Fitz-Díaz et al. Reference Fitz-Díaz, Hudleston, Tolson and van der Pluijm2014; Garduño-Martínez et al. Reference Garduño-Martínez, Pi Puig, Solé, Martini and Alcalá-Martínez2015; Martini et al. Reference Martini, Solé, Garduño-Martínez, Puig and Omaña2016; Guerrero-Paz et al. Reference Guerrero-Paz, Abdullin, Ortega-Flores, Solari, Ortega-Obregón, Juárez-Arriaga, Martens and Molina Garza2020). The spatial and temporal distribution of foredeep deposits suggests that the shortening migrated from west to east in the Sierra Madre Oriental during Late Cretaceous to Eocene times (e.g. Martini et al. Reference Martini, Solé, Garduño-Martínez, Puig and Omaña2016; Guerrero-Paz et al. Reference Guerrero-Paz, Abdullin, Ortega-Flores, Solari, Ortega-Obregón, Juárez-Arriaga, Martens and Molina Garza2020). Furthermore, this progressive and episodic eastward migration of the deformation was also proposed by detailed structural analyses and geochronological studies carried out by Fitz-Díaz et al. (Reference Fitz-Díaz, Tolson, Hudleston, Bolaños-Rodríguez, Ortega-Flores and Serrano2012, Reference Fitz-Díaz, Hudleston, Tolson and van der Pluijm2014).
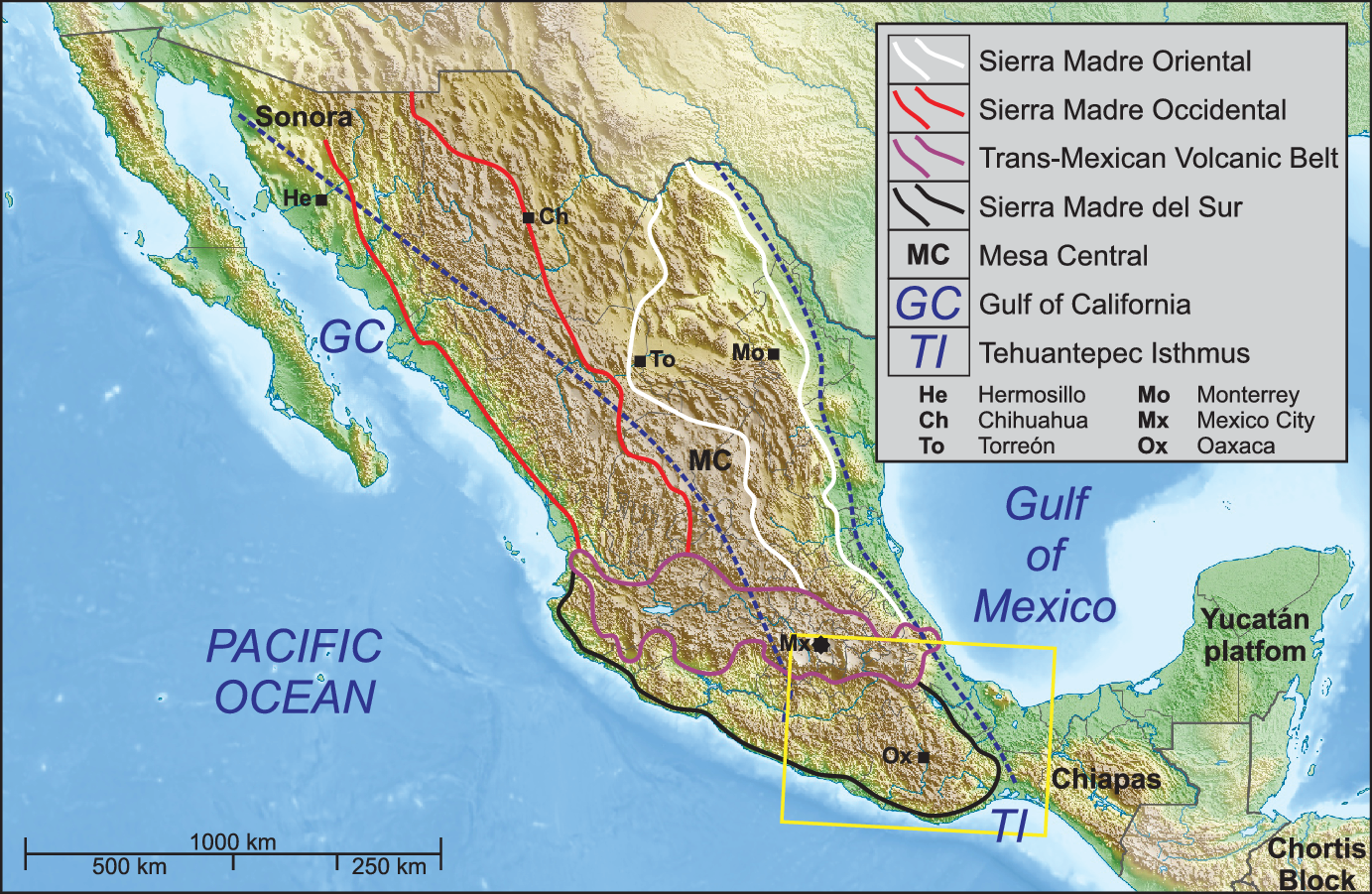
Fig. 1. Topographic map showing the main physiographic provinces of Mexico (modified after Fitz-Díaz et al. Reference Fitz-Díaz, Lawton, Juárez-Arriaga and Chávez-Cabello2018). Dashed lines display the approximate distribution of the Mexican Orogen structures. The yellow rectangle approximately corresponds to the geological map depicted in Figure 2.
In southern Mexico, the Cretaceous–Cenozoic major lithological units and structures of the Sierra Madre del Sur are well known (e.g. Nieto-Samaniego et al. Reference Nieto-Samaniego, Alaniz-Álvarez, Silva-Romo, Eguiza-Castro and Mendoza-Rosales2006). The Laramide sensu lato is considered the main cause of the contractile structures within the Sierra Madre del Sur; however, details about the migration, kinematics and intensity of deformation are poorly understood. Nieto-Samaniego et al. (Reference Nieto-Samaniego, Alaniz-Álvarez, Silva-Romo, Eguiza-Castro and Mendoza-Rosales2006) compiled published structural data and performed a detailed field-based study, analysing the geometry, kinematics and chronology of the main structures of southern Mexico. These authors proposed that the deformation migrated in the Sierra Madre del Sur from west to east between Maastrichtian and middle Eocene times and also recommended that more geochronological (thermochronological) data would be needed to establish the migration paths. Low-temperature thermochronometric techniques (i.e. He dating and fission-track analysis) have been applied sparsely to elucidating the timing of exhumation periods in the Sierra Madre del Sur (Ducea et al. Reference Ducea, Valencia, Shoemaker, Reiners, DeCelles, Campa, Morán-Zenteno and Ruiz2004; Abdullin et al. Reference Abdullin, Solari, Solé and Ortega-Obregón2021; Ramírez-Calderón et al. Reference Ramírez-Calderón, Bedoya, Abdullin, Martini, Solari and Ortega-Obregón2021). For example, Abdullin et al. (Reference Abdullin, Solari, Solé and Ortega-Obregón2021) reported apatite fission-track (AFT) data from the northern–central Grenvillian Oaxacan Complex and identified two Mesozoic cooling periods that were interpreted as resulting from exhumation: a Middle Triassic to Middle Jurassic cooling event that is probably linked to the break-up of Pangaea, and a younger one related to an exhumation episode during Early Cretaceous time that is coeval with the final stages of rifting of the Gulf of Mexico. Ramírez-Calderón et al. (Reference Ramírez-Calderón, Bedoya, Abdullin, Martini, Solari and Ortega-Obregón2021) also detected Triassic-aged cooling signals from the Pennsylvanian–Cisuralian Totoltepec pluton, northeastern Acatlán Complex. However, there have been no detailed thermochronological studies performed to reconstruct the exhumation histories for the Late Cretaceous and Palaeogene period. In this work, we report the AFT results obtained from three different sectors of the Sierra Madre del Sur, which were obtained by laser ablation inductively coupled plasma mass spectrometry (LA-ICP-MS) for in situ quantification of 238U (Hasebe et al. Reference Hasebe, Barbarand, Jarvis, Carter and Hurford2004; Donelick et al. Reference Donelick, O’Sullivan and Ketcham2005; Vermeesch, Reference Vermeesch2017). The main objective of our study is the timing of the exhumation that took place in the Sierra Madre del Sur physiographic province during the Mexican Orogeny (or Laramide sensu lato). The results obtained during this work from three distinct study areas provide new insights into the deformation and exhumation history of the Sierra Madre del Sur during approximately Campanian–middle Eocene time.
2. Geological overview
The geological history of Mexico during the Mesozoic–Cenozoic period preserves a polyphase geodynamic evolution, and some significant tectonic events that occurred during those times may be described briefly as follows. During the Mesozoic period, the continental extensional tectonics was controlled by two important geodynamic processes: the subduction of the Farallon Plate beneath the western margin of North America and the rupture of Pangaea during Late Triassic–Middle Jurassic time (e.g. Martini & Ortega-Gutiérrez, Reference Martini and Ortega-Gutiérrez2018; Parolari et al. Reference Parolari, Martini, Gómez-Tuena, Ortega-Gutiérrez, Errázuriz-Henao and Cavazos-Tovar2022). Martini et al. (Reference Martini, Solari and López-Martínez2014) and Palacios-García & Martini (Reference Palacios-García and Martini2014) suggested that the Late Jurassic to Early Cretaceous back-arc spreading of the Arperos Basin separated the Guerrero terrane from the Mexican mainland. After the accretion of the Guerrero composite terrane with the continental interior of Mexico, the Cretaceous sedimentation was dominantly calcareous (Fitz-Díaz et al. Reference Fitz-Díaz, Tolson, Camprubí, Rubio-Ramos and Prol-Ledesma2008). The Laramide sensu lato deformation event occurred after the accretion of the Guerrero terrane, and the shortening was active between Late Cretaceous and Eocene times (Nieto-Samaniego et al. Reference Nieto-Samaniego, Alaniz-Álvarez, Silva-Romo, Eguiza-Castro and Mendoza-Rosales2006). It has been documented that the beginning and ending of the shortening deformation were diachronic (e.g. Cuéllar-Cárdenas et al. Reference Cuéllar-Cárdenas, Nieto-Samaniego, Levresse, Alaniz-Álvarez, Solari, Ortega-Obregón and López-Martínez2012; Martini et al. Reference Martini, Solé, Garduño-Martínez, Puig and Omaña2016; Fitz-Díaz et al. Reference Fitz-Díaz, Lawton, Juárez-Arriaga and Chávez-Cabello2018).
The Sierra Madre del Sur, the principal object of this study, is located in southern Mexico and represents a large physiographic province composed of distinct crustal blocks with different lithologies bounded by major structures (Ortega-Gutiérrez et al. Reference Ortega-Gutiérrez, Elías-Herrera, Morán-Zenteno, Solari, Weber and Luna-González2018). The crystalline basements of these blocks constitute outcrops of the lower and middle crust. According to the terrane division model of Sedlock et al. (Reference Sedlock, Ortega-Gutiérrez and Speed1993), this physiographic province encompasses the Nahuatl, Mixteco, Zapoteco, Cuicateco, Chatino and Maya tectonostratigraphic terranes (Fig. 2). It is notable that most of the geological structures and the styles of deformation seem unrelated to terrane divisions (e.g. Nieto-Samaniego et al. Reference Nieto-Samaniego, Alaniz-Álvarez, Silva-Romo, Eguiza-Castro and Mendoza-Rosales2006). In this study, we report the AFT results obtained from three study areas, presented here as three different sectors (Fig. 3). The geological settings for the studied sectors are described below.
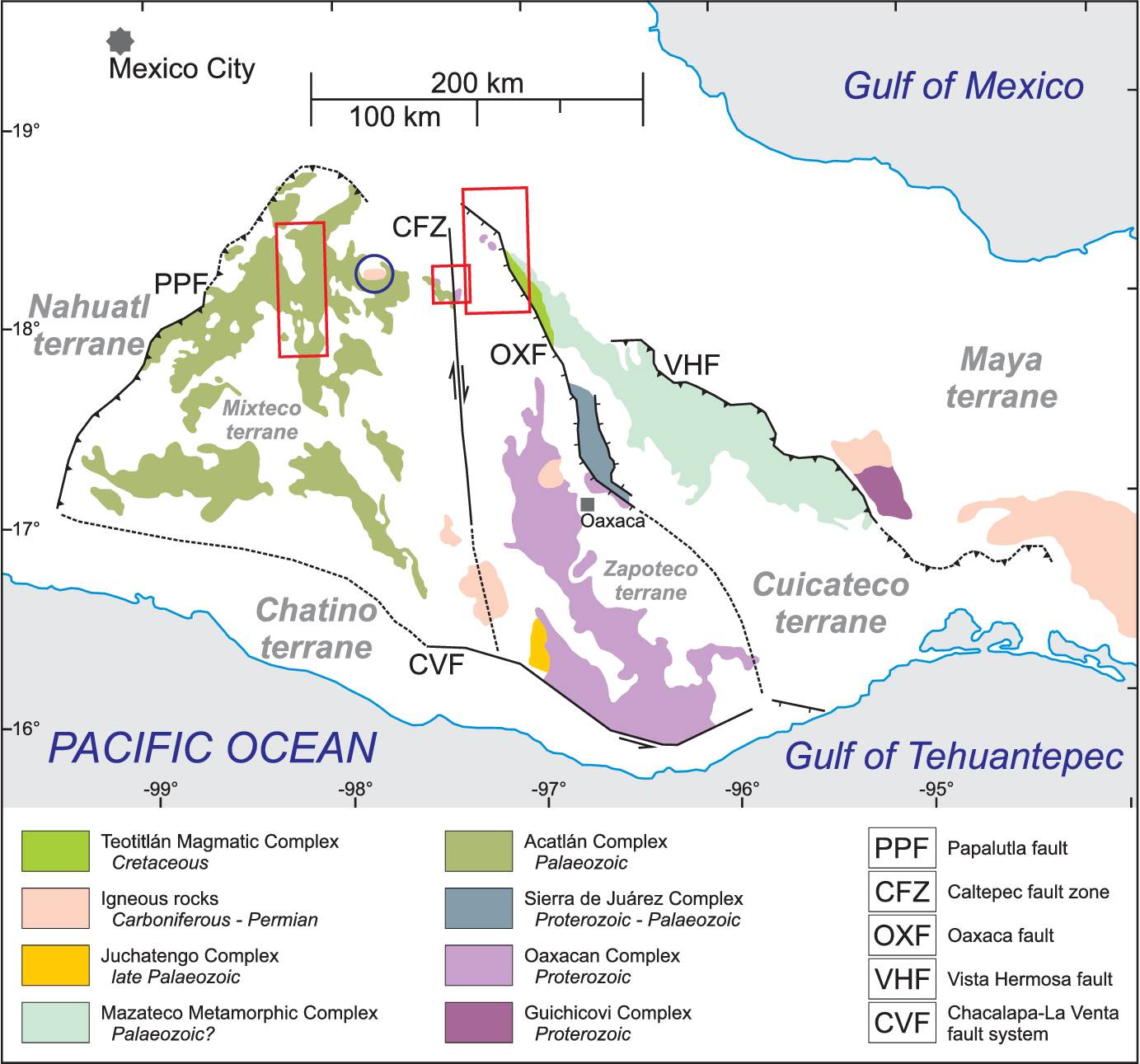
Fig. 2. Geological map showing basement lithologies and tectonostratigraphic terranes of southern Mexico (according to Ortega-Gutiérrez et al. Reference Ortega-Gutiérrez, Elías-Herrera, Morán-Zenteno, Solari, Weber and Luna-González2018; Espejo-Bautista et al. Reference Espejo-Bautista, Ortega-Gutiérrez, Solari, Maldonado and Valencia-Morales2022). Red rectangular regions represent the studied sectors where apatite fission-track results were obtained during this study (see also Fig. 3 for details). Blue circle is the area studied by M. G. Ramírez-Calderón (unpub. M.Sc. thesis, Univ. Nacional Autónoma de México, 2018).

Fig. 3. Simplified geological maps of the western, central and eastern sectors displaying the sampled points (western sector – data according to Ortega-Gutiérrez et al. Reference Ortega-Gutiérrez, Elías-Herrera, Morán-Zenteno, Solari, Weber and Luna-González2018; central sector – data according to Bedoya et al. Reference Bedoya, Anaya-Guarneros, Abdullin, Martini and Solari2021; Martini et al. Reference Martini, Anaya Guarneros, Solar, Bedoya, Zepeda-Martínez and Villanueva-Amadoz2022; eastern sector – data from R. E. Milián de la Cruz, unpub. M.Sc. thesis, Univ. Nacional Autónoma de México, 2019).
2.a. Geology of the western sector
The western sector forms part of the Mixteco terrane (Figs 2, 3). This terrane is bounded by the Papalutla fault that separates the Mixteco terrane from the Nahuatl terrane, by the dextral-transpressional Caltepec fault zone to the east, which separates the Acatlán Complex from the Oaxacan Complex, as well as by the Chacalapa–La Venta fault system on the southern side (Fig. 2). The basement of the Mixteco terrane is represented by polymetamorphic rocks of the Palaeozoic Acatlán Complex. This metamorphic complex was originally divided into two tectonic assemblages: the structurally lower Petlalcingo subgroup and the upper Acateco subgroup (Ortega-Gutiérrez, Reference Ortega-Gutiérrez1978), both of which are components of a deformed thrust nappe overlain by upper Palaeozoic, low-grade metamorphic rocks. The geological record of this basement has undergone considerable revision in recent years owing to the improvement and enrichment of the geochronological, isotopic and palaeontological database (Keppie et al. Reference Keppie, Dostal, Murphy and Nance2008, Reference Keppie, Dostal and Li2018 and references therein). The Acateco subgroup includes the Piaxtla Suite and the Esperanza Granitoids, which underwent high-pressure metamorphism (e.g. Vega-Granillo et al. Reference Vega-Granillo, Talavera-Mendoza, Meza-Figueroa, Ruiz, Gehrels, López-Martínez and de la Cruz-Vargas2007). For the AFT analysis, we focused on the Esperanza Granitoids, which have been described as a sequence of metamorphosed and strongly deformed intrusive bodies such as granite and pegmatite (Ortega-Gutiérrez, Reference Ortega-Gutiérrez1978; Ortega-Gutiérrez et al. Reference Ortega-Gutiérrez, Elías-Herrera, Reyes-Salas, Macías-Romo and López1999; S. L. Florez-Amaya, unpub. M.Sc. thesis, Univ. Nacional Autónoma de México, 2021). According to J. Ramírez-Espinosa (unpub. Ph.D. thesis, Univ. Arizona, 2001) and M. Reyes-Salas (unpub. Ph. D. thesis, Univ. Autónoma del Estado de Morelos, 2003), the protolith for this unit is a peraluminous S-type granitic rock. The emplacement ages of the Esperanza Granitoids vary from ∼485 Ma to ∼440 Ma (Ortega-Gutiérrez et al. Reference Ortega-Gutiérrez, Elías-Herrera, Reyes-Salas, Macías-Romo and López1999; Talavera-Mendoza et al. Reference Talavera-Mendoza, Ruiz, Gehrels, Meza-Figueroa, Vega-Granillo and Campa-Uranga2005; Vega-Granillo et al. Reference Vega-Granillo, Talavera-Mendoza, Meza-Figueroa, Ruiz, Gehrels, López-Martínez and de la Cruz-Vargas2007; S. L. Florez-Amaya, unpub. M.Sc. thesis, Univ. Nacional Autónoma de México, 2021). Although some authors, for example, Vega-Granillo et al. (Reference Vega-Granillo, Talavera-Mendoza, Meza-Figueroa, Ruiz, Gehrels, López-Martínez and de la Cruz-Vargas2007), have argued for a Silurian high-pressure metamorphism event recorded by mafic intrusions associated with the Esperanza Granitoids unit, presently available direct dating of the eclogite- and blueschist-facies metamorphism has yielded Mississippian dates, more precisely, at 353 ± 2 Ma (Estrada-Carmona et al. Reference Estrada-Carmona, Weber, Scherer, Martens and Elías-Herrera2016; S. L. Florez-Amaya, unpub. M.Sc. thesis, Univ. Nacional Autónoma de México, 2021).
2.b. Geology of the central sector
The central sector is located within an area between the Mixteco and Zapoteco tectonostratigraphic terranes (Figs 2, 3). For the AFT analysis in this sector, most samples were collected from the Matzitzi Formation. This unit unconformably overlies Palaeozoic and Proterozoic rocks belonging to the Acatlán and Oaxacan complexes (e.g. Centeno-García et al. Reference Centeno-García, Mendoza-Rosales and Silva-Romo2009; Bedoya et al. Reference Bedoya, Anaya-Guarneros, Abdullin, Martini and Solari2021). The Oaxacan Complex is mainly composed of Proterozoic, granulite-facies, mafic to felsic gneisses as well as numerous pegmatitic intrusions (Shchepetilnikova et al. Reference Shchepetilnikova, Solé, Solari and Abdullin2015). The boundary between the Oaxacan and the Acatlán complexes is a NNE-trending dextral transpressive shear zone, i.e. the Caltepec fault zone (Elías-Herrera et al. Reference Elías-Herrera, Ortega-Gutiérrez, Sánchez-Zavala, Macías-Romo, Ortega-Rivera and Iriondo2005). The ∼270 Ma age Cozahuico pluton (Fig. 3) is exposed along the Caltepec fault and is interpreted as a syntectonic intrusion dating the collision between the Oaxacan and Acatlán complexes (Elías-Herrera et al. Reference Elías-Herrera, Ortega-Gutiérrez, Sánchez-Zavala, Macías-Romo, Ortega-Rivera and Iriondo2005). The Matzitzi Formation was first described by Aguilera (Reference Aguilera1896), and then defined by Calderón-García (Reference Calderón-García1956) as a succession composed of sandstone, dark shale, conglomerate and coal containing a diverse fossil flora. Centeno-García et al. (Reference Centeno-García, Mendoza-Rosales and Silva-Romo2009) recognized in the Matzitzi Formation the typical facies associations that characterize modern anastomosing fluvial systems. The age of the Matzitzi Formation has been an object of debate for decades (for example, see details in Bedoya et al. Reference Bedoya, Anaya-Guarneros, Abdullin, Martini and Solari2021; Martini et al. Reference Martini, Anaya Guarneros, Solar, Bedoya, Zepeda-Martínez and Villanueva-Amadoz2022). Based on stratigraphic correlations, Aguilera (Reference Aguilera1896) and Calderón-García (Reference Calderón-García1956) first proposed a Triassic and Jurassic age, respectively. Later, Silva (Reference Silva1970) reported the occurrence of Pennsylvanian fossil plants in the Matzitzi Formation. After a careful re-evaluation of the flora association studied by Weber (Reference Weber1997), Flores-Barragán et al. (Reference Flores-Barragán, Velasco-de León, Lozano-Carmona and Ortega-Chavez2019) assigned a late Permian age to the Matzitzi Formation. This depositional age was confirmed by recent geochronological studies performed by Martini et al. (Reference Martini, Anaya Guarneros, Solar, Bedoya, Zepeda-Martínez and Villanueva-Amadoz2022).
2.c. Geology of the eastern sector
The eastern sector is located within the Cuicateco terrane (Fig. 2). In this study area, we sampled the Chivillas Formation for analysis by the AFT method. The age of the crystalline basement upon which the Chivillas Formation was deposited is still unconstrained. This formation is in fault contact with the Oaxacan Complex (Fig. 3). This contact had multiple reactivations, the youngest as a Cenozoic normal fault (i.e. the Oaxaca fault; Fig. 2). The basin-fill covers the contact between the Cuicateco terrane and the Oaxacan Complex. Most rocks from the Cuicateco terrane belong to the Sierra de Juárez mylonitic belt, composed of mylonitized gneisses and volcano-sedimentary rocks as well as serpentinites (Delgado-Argote, Reference Delgado-Argote1988). The Sierra de Juárez mylonitic belt was formed in a dextral strike-slip fault (Alaniz-Álvarez et al. Reference Alaniz-Álvarez, Nieto-Samaniego and Ortega-Gutiérrez1994). The radiometric ages from this belt range between 192 ± 1 Ma (i.e. the protolith age of a syntectonic metagranite; Espejo-Bautista et al. Reference Espejo-Bautista, Ortega-Gutiérrez, Solari, Maldonado and Valencia-Morales2022) and 169 ± 2 Ma (muscovite 40Ar–39Ar age; Alaniz-Álvarez et al. Reference Alaniz-Álvarez, van der Heyden, Nieto Samaniego and Ortega-Gutiérrez1996). The serpentinitic bodies yielded hornblende 40Ar–39Ar cooling ages of 138 ± 8, 132 ± 4 and 123 ± 7 Ma (Delgado-Argote et al. Reference Delgado-Argote, López-Martínez, York and Hall1992). The Sierra de Juárez mylonitic belt is thrust over the oldest rocks of the Cuicateco terrane (i.e. the Mazateco Complex), made up of schist and amphibolite of probably Palaeozoic age (Mendoza-Rosales et al. Reference Mendoza-Rosales, Centeno-García, Silva-Romo, Campos-Madrigal and Bernal2010). The Cuicateco terrane also contains thick limestone units. The mylonitic rocks are thrust over the limestone units. To the south, the Mazateco Complex is thrust over Upper Triassic(?) to Jurassic (e.g. Pérez-Gutiérrez et al. Reference Pérez-Gutiérrez, Solari, Gómez-Tuena and Valencia2009) red beds from the Todos Santos Formation. The Chivillas Formation consists of a quite thick succession of pillow lavas and basaltic lava flows, interbedded with turbidites and is overlain depositionally by limestone. Mendoza-Rosales et al. (Reference Mendoza-Rosales, Centeno-García, Silva-Romo, Campos-Madrigal and Bernal2010) interpreted the upper contact of the Chivillas Formation as transitional, based on the gradational change from siliciclastic turbidites to calcareous turbidites belonging to the Miahuatepec Formation. Based on detrital zircon U–Pb dating, a Barremian stratigraphic age was preliminarily proposed for the Chivillas Formation (Mendoza-Rosales et al. Reference Mendoza-Rosales, Centeno-García, Silva-Romo, Campos-Madrigal and Bernal2010). The deformation history of the Cuicateco terrane is very complex, and some authors have detected three to five different deformation episodes (e.g. Alaniz-Álvarez et al. Reference Alaniz-Álvarez, van der Heyden, Nieto Samaniego and Ortega-Gutiérrez1996; E. Ángeles-Moreno, unpub. M.Sc. thesis, Univ. Nacional Autónoma de México, 2006; Mendoza-Rosales et al. Reference Mendoza-Rosales, Centeno-García, Silva-Romo, Campos-Madrigal and Bernal2010).
3. Samples and methods
To detect Late Cretaceous and Cenozoic cooling ages, we sampled those areas that are close to key regional structures (Figs 2, 3), as was recommended by Abdullin et al. (Reference Abdullin, Solari, Solé and Ortega-Obregón2021). A total of four augen gneiss samples were collected from the Esperanza Granitoids in the western sector (EG-1, EG-2, EG-3 and EG-4; Fig. 3). In the central study area, we primarily focused on the late Permian Matzitzi Formation (sandstone samples MF-1 and MF-2; Fig. 3). In this area, additionally, one sample (AM-X; sandstone) from the Agua de Mezquite formation (a Middle Jurassic? clastic unit; Bedoya et al. Reference Bedoya, Anaya-Guarneros, Abdullin, Martini and Solari2021) and another one from the Grenvillian Oaxacan Complex (OC-X; granulite gneiss) were also obtained for the AFT thermochronology. Two sandstone samples, CF-1 and CF-2, were collected from the Barremian(?) Chivillas Formation in the easternmost sector (Fig. 3).
Heavy minerals from the collected samples were concentrated using conventional mineral separation techniques such as crushing, sieving, Wilfley table, Frantz separator, and heavy liquids (we used bromoform and sodium polytungstate). Approximately 500 apatite grains, extracted from each concentrate sample under a dissecting microscope, were mounted with EpoFix (Struers) in a 2.5 cm diameter plastic ring (i.e. most crystals were mounted with their surfaces parallel to the c-axis). Mounted crystals were polished to expose their internal surfaces (i.e. for 4π geometry), and then were etched in 5.5M HNO3 at 21 °C for 20 s to reveal spontaneous fission tracks (e.g. see Donelick et al. Reference Donelick, O’Sullivan and Ketcham2005). Fission-track counting and track length measurements were performed using a Zeiss AxioScope.A1 microscope upgraded with a digital camera, image processing software and dry objectives. LA-ICP-MS-based single-spot analyses, using a laser beam spot diameter of ∼60 µm, were performed exactly within the same counting areas observed previously to obtain the spontaneous track densities (Abdullin et al. Reference Abdullin, Solé, Meneses-Rocha, Solari, Shchepetilnikova and Ortega-Obregón2016; Vermeesch, Reference Vermeesch2017). Polished and etched sections of Durango F-apatite parallel to the crystallographic c-axis were also analysed during the same sessions of track counting and LA-ICP-MS analysis. This fluorapatite, with a standard age of 31.4 ± 0.5 Ma (e.g. Solé & Pi, Reference Solé and Pi2005; Abdullin et al. Reference Abdullin, Solé and Solari2014), was used for ζ-equivalent calibration (Hasebe et al. Reference Hasebe, Barbarand, Jarvis, Carter and Hurford2004; Donelick et al. Reference Donelick, O’Sullivan and Ketcham2005; Vermeesch, Reference Vermeesch2017, Reference Vermeesch2018) as well as to determine chlorine levels in unknown apatite grains (i.e. Durango, with an average Cl of 0.43 ± 0.04 wt %, was used as a primary standard for Cl measurements; Chew et al. Reference Chew, Donelick, Donelick, Kamber and Stock2014). Raw data were reduced using Iolite 3.4 (Paton et al. Reference Paton, Hellstrom, Paul, Woodhead and Hergt2011). The results for measured isotopes using NIST612 (Pearce et al. Reference Pearce, Perkins, Westgate, Gorton, Jackson, Neal and Chenery1997) were normalized using 43Ca as an internal standard and taking an average CaO of 55 % for all apatite grains analysed. Single-grain AFT ages and 1σ-errors were calculated with IsoplotR (Vermeesch, Reference Vermeesch2018). The central (i.e. weighted mean) ages were obtained with RadialPlotter (Vermeesch, Reference Vermeesch2009). LA-ICP-MS AFT analysis was carried out at Laboratorio de Estudios Isotópicos (LEI), Centro de Geociencias, Campus Juriquilla, Universidad Nacional Autónoma de México. The LA-ICP-MS protocol (including laser ablation and ICP-MS operating conditions, data acquisition parameters, scheme of microsampling, measured isotopes, etc.) used routinely at LEI for apatite is described in detail by Ortega-Obregón et al. (Reference Ortega-Obregón, Abdullin, Solari, Schaaf and Solís-Pichardo2019) and Ramírez-Calderón et al. (Reference Ramírez-Calderón, Bedoya, Abdullin, Martini, Solari and Ortega-Obregón2021).
4. Apatite fission-track results
The AFT results obtained from all the analysed rock samples are summarized in Table 1 and Figure 4, while detailed information on our fission-track and LA-ICP-MS experiments (number of tracks counted, confined track length measurements, 238U and Cl concentrations, single-grain ages with 1σ-errors, analytical uncertainties, etc.) are given in the online Supplementary Material. The AFT-based time–temperature (t–T) histories were reconstructed for six samples (EG-2, EG-4, AM-X, MF-2, CF-1 and CF-2) using HeFTy 1.9.3 (Ketcham, Reference Ketcham2005) with inverse Monte Carlo modelling and based on the multicomponent annealing model of Ketcham et al. (Reference Ketcham, Carter, Donelick, Barbarand and Hurford2007). The ‘best-fit’ cooling curves were obtained testing 200 ‘good’ thermal history scenarios. Most apatite grains analysed during this study are F-apatite, with average Cl contents of less than 0.9 wt % (Table 1). The closure temperature for the AFT system in most common apatite specimens, i.e. in F-apatite, generally varies between ∼120 and 90 °C depending on the cooling rates (Donelick et al. Reference Donelick, O’Sullivan and Ketcham2005). For fission tracks in F-apatite, the temperature span of ∼60–110 °C is referred to as the partial annealing zone (Gleadow et al. Reference Gleadow, Duddy, Green and Lovering1986). Boxes limiting possible solutions to the t–T models (Fig. 5) were added based on known and reasonable geological constraints (Abdullin et al. Reference Abdullin, Solé, Meneses-Rocha, Solari, Shchepetilnikova and Ortega-Obregón2016, Reference Abdullin, Solari, Ortega-Obregón and Solé2018, Reference Abdullin, Solari, Solé and Ortega-Obregón2021; A. M. Bedoya-Mejía, unpub. M.Sc. thesis, Univ. Nacional Autónoma de México, 2018; M. G. Ramírez-Calderón, unpub. M.Sc. thesis, Univ. Nacional Autónoma de México, 2018; R. E. Milián de la Cruz, unpub. M.Sc. thesis, Univ. Nacional Autónoma de México, 2019; S. L. Florez-Amaya, unpub. M.Sc. thesis, Univ. Nacional Autónoma de México, 2021).
Table 1. LA-ICP-MS-based apatite fission-track results obtained from three different study areas across the Sierra Madre del Sur, southern Mexico

Note: Ngr is the number of grains dated. The central ages (i.e. weighted mean ages) were obtained using RadialPlotter (Vermeesch, Reference Vermeesch2009). D and P(χ2) are the dispersion of ages and the chi-squared probability test, respectively. MTL represents the mean track length. Ntr is the number of confined track lengths tested (only track-in-track-type horizontally confined tracks were measured; e.g. please see details in Donelick et al. Reference Donelick, O’Sullivan and Ketcham2005). Cl is the chlorine content determined with LA-ICP-MS. The types of samples (i.e. lithologies and ages) can be consulted in the main text.

Fig. 4. Apatite fission-track (AFT) results obtained from the three studied sectors.

Fig. 5. Apatite fission-track (AFT)-based time–temperature modelling. PAZ – partial annealing zone for the AFT chronometry. Purple areas in the models represent ‘good’ thermal history scenarios, while green areas represent ‘acceptable’ paths. Solid lines represent the ‘best-fit’ cooling histories. GOF – goodness-of-fit between the measured and the model ages. GOF (K-S) – goodness-of-fit between the measured and the model fission-track lengths. K-S – Kolmogorov–Smirnov test.
4.a. AFT results from the western sector
The central AFT ages, obtained for four gneiss samples from the Esperanza Granitoids (Acatlán Complex), range from 69 ± 3 (1σ) Ma (sample EG-3) to 57 ± 3 (1σ) Ma (for EG-1) (Table 1; Fig. 4). All the dated samples passed the chi-squared probability test with P(χ2) higher than 0.05 (Fig. 4), indicating that these apatite groups represent a single cooling event. Track length measurements were performed for two samples, EG-2 and EG-4, which have mean track length (MTL) values of 12.81 ± 1.48 (SD) µm and 13.07 ± 1.35 (SD) µm, respectively (Table 1). The results of the t–T modelling performed for samples EG-2 and EG-4 are given in Figure 5.
4.b. AFT results from the central sector
As shown in Figure 4, all the four samples yielded similar central AFT ages (i.e. ∼60 Ma; see also Table 1) of 60 ± 2 (1σ) Ma for sample AM-X, 59 ± 1 (1σ) Ma for MF-1, 61 ± 2 (1σ) Ma in MF-2 and 61 ± 3 (1σ) Ma for the Grenvillian sample OC-X. These cooling ages are significantly younger than the stratigraphic ages of the Matzitzi Formation (late Permian; Martini et al. Reference Martini, Anaya Guarneros, Solar, Bedoya, Zepeda-Martínez and Villanueva-Amadoz2022) and the Agua de Mezquite unit (Middle Jurassic?; Bedoya et al. Reference Bedoya, Anaya-Guarneros, Abdullin, Martini and Solari2021). This implies that detrital apatite grains from the studied sandstone samples AM-X, MF-1 and MF-2 were reset totally for the AFT system after their deposition, certainly due to burial-related heating of these clastic units during diagenesis. All these samples passed the chi-squared probability test with P(χ2) values of 0.08 for sample AM-X, 0.51 for MF-1, 0.13 for MF-2 and 1.00 for sample OC-X (Fig. 4). Track length measurements were performed for two samples, AM-X and MF-2, which yielded MTLs of 13.15 ± 1.38 (SD) and 13.16 ± 1.10 (SD) µm, respectively (Table 1). This implies that these detrital apatite populations belong to a single monotonic cooling event. The cooling histories obtained from samples AM-X and MF-2 are displayed in Figure 5.
4.c. AFT results from the eastern sector
Two clastic samples from the Barremian Chivillas Formation (Mendoza-Rosales et al. Reference Mendoza-Rosales, Centeno-García, Silva-Romo, Campos-Madrigal and Bernal2010), CF-1 and CF-2, were analysed using the AFT technique. These samples yielded identical central ages of 40 ± 1 (1σ) Ma (sample CF-1) and 41 ± 1 (1σ) Ma (CF-2). Both samples passed the chi-squared probability test with P(χ2) higher than 0.05 (Table 1; Fig. 4). These sandstone samples display AFT ages that are younger than the depositional age of the Chivillas Formation. CF-1 and CF-2 have MTL values of 13.3 ± 1.35 (SD) µm and 13.08 ± 1.27 (SD) µm, respectively (Table 1). The AFT results obtained from the Chivillas Formation indicate that both samples cooled rapidly through the partial annealing zone of ∼60–110/120 °C. HeFTy-derived cooling paths constructed for the Chivillas Formation are presented in Figure 5.
5. Discussion and concluding remarks
The development of extensive Cretaceous platforms with deposition of thick carbonate successions along the rim of the Gulf of Mexico (Wilson & Ward, Reference Wilson, Ward, Simo, Scott and Masse1993; Padilla y Sánchez, Reference Padilla y Sánchez2007) was enough to reset apatite grains for the fission-track chronometry in the rocks, particularly in clastic ones (i.e. in samples of the Agua de Mezquite, Matzitzi and Chivillas formations), owing to burial-related heating during diagenesis. In these areas, the thermochronometric results should represent the cooling histories controlled by relatively young exhumation processes (i.e. post-platform ages). The AFT ages decrease from west to east (i.e. from the Mixteco terrane through Zapoteco to the Cuicateco terrane; Fig. 2): from ∼70 to 60 Ma in the western part through ∼60 Ma in the central part to ∼40 Ma in the eastern sector (Fig. 4), a time span that is interpreted as a period of cooling of the Sierra Madre del Sur due to the exhumation and erosion between Late Cretaceous and middle Eocene times. This exhumation period was also observed in Chiapas, southeastern Mexico (Fig. 1), which could be interpreted as the southernmost continuation of the Late Cretaceous–Eocene orogenic system in Mexico (Meneses-Rocha, Reference Meneses-Rocha, Bartolini, Buffler and Cantú-Chapa2001; Padilla y Sánchez, Reference Padilla y Sánchez2007; Abdullin et al. Reference Abdullin, Solé, Meneses-Rocha, Solari, Shchepetilnikova and Ortega-Obregón2016, Reference Abdullin, Solari, Ortega-Obregón and Solé2018). According to Martini et al. (Reference Martini, Solé, Garduño-Martínez, Puig and Omaña2016), the shortening of the westernmost part (i.e. the Sierra de los Cuarzos area) of the Sierra Madre Oriental started in Campanian time. Radiometric data compiled in Guerrero-Paz et al. (Reference Guerrero-Paz, Abdullin, Ortega-Flores, Solari, Ortega-Obregón, Juárez-Arriaga, Martens and Molina Garza2020) for the Sierra Madre Oriental as well as our AFT data obtained from the Sierra Madre del Sur support that the deformation initiated in Campanian time, because K–Ar and 40Ar–39Ar dates of authigenic illite determined in shear zones and folds from the Sierra Madre Oriental (Fitz-Díaz et al. Reference Fitz-Díaz, Hudleston, Tolson and van der Pluijm2014; Garduño-Martínez et al. Reference Garduño-Martínez, Pi Puig, Solé, Martini and Alcalá-Martínez2015; Martini et al. Reference Martini, Solé, Garduño-Martínez, Puig and Omaña2016), along with the AFT ages obtained in the Sierra Madre del Sur (this study), are younger than 85 Ma. Further, M. G. Ramírez-Calderón (unpub. M.Sc. thesis, Univ. Nacional Autónoma de México, 2018) reported young AFT peaks at 88 ± 8, 78 ± 5 and 64 ± 6 Ma from the Totoltepec pluton, Acatlán Complex (circle-shaped study area in Fig. 2), cooling ages which lie roughly in the Santonian–Maastrichtian period. These AFT ages were tentatively interpreted by M. G. Ramírez-Calderón (unpub. M.Sc. thesis, Univ. Nacional Autónoma de México, 2018) as belonging to the Mexican Orogen.
Thermal history models indicate that the exhumation migrated across the Sierra Madre del Sur from west to east between Late Cretaceous and middle Eocene times (Fig. 5), a tectonic activity that is coeval with the Laramide sensu lato (Mexican Orogen) as was previously suggested by Nieto-Samaniego et al. (Reference Nieto-Samaniego, Alaniz-Álvarez, Silva-Romo, Eguiza-Castro and Mendoza-Rosales2006). Samples EG-2 and EG-4, obtained from the western area, detected an exhumation period for a time interval of ∼80–60 Ma (Campanian to Paleocene) with a low cooling rate of ∼4 °C Ma−1. In contrast, in the central and eastern sectors, the analysed samples yielded younger cooling episodes of ∼70–60 Ma (Maastrichtian to Paleocene) and ∼45–35 Ma (i.e. middle Eocene) with moderate and elevated cooling rates of ∼12 °C Ma−1 and ∼17 °C Ma−1, respectively (Fig. 5). The AFT cooling ages become younger from west to east across the Sierra Madre del Sur, whereas the cooling rates become higher in the same direction. Our AFT results (in particular, the cooling rates; Fig. 5), thus, confirm that the deformation and associated exhumation migrated from west to east during Campanian–middle Eocene time. The AFT ages obtained during this study were mainly interpreted as belonging to the Laramide sensu lato shortening event. However, ∼45–35 Ma cooling ages with high cooling rates (Fig. 5) in the easternmost sector (Cuicateco terrane) are most likely related to an early extensional phase that initiated approximately during middle Eocene time (e.g. Dávalos-Álvarez et al. Reference Dávalos-Álvarez, Nieto-Samaniego, Alaniz-Álvarez, Martínez-Hernández and Ramírez-Arriaga2007), shortly after the end of the Laramide sensu lato. This extensional event reactivated the Oaxaca fault in Eocene time as a normal fault producing the Tehuacán Valley with deposition of the Tilapa red beds (Fig. 3) and uplift and erosion of the Mazateca range (Nieto-Samaniego et al. Reference Nieto-Samaniego, Alaniz-Álvarez, Silva-Romo, Eguiza-Castro and Mendoza-Rosales2006; Dávalos-Álvarez et al. Reference Dávalos-Álvarez, Nieto-Samaniego, Alaniz-Álvarez, Martínez-Hernández and Ramírez-Arriaga2007).
According to some authors, for example, Fitz-Díaz et al. (Reference Fitz-Díaz, Lawton, Juárez-Arriaga and Chávez-Cabello2018), active magmatism during the entire evolution of the Mexican Orogen implicates the subducted Farallon slab as a principal driver of crustal thickening. Besides, deformation–magmatic cycles coincide well with periods of westward acceleration of the North America Plate and further corroborate subduction as the main geodynamic driver for the Mexican orogenesis during Late Cretaceous–Eocene time (van der Meer et al. Reference van der Meer, Spakman, Van Hinsbergen, Amaru and Torsvik2010; Fitz-Díaz et al. Reference Fitz-Díaz, Lawton, Juárez-Arriaga and Chávez-Cabello2018). In southern Mexico, the convergence between circum-Pacific terranes (e.g. the Guerrero terrane and the Chortis Block) and mainland southern Mexico (Mixteco and Zapoteco) may have triggered thickening and orogenic metamorphism of the Mesozoic crust of the Xolapa Complex (i.e. part of the Chatino terrane; Fig. 2) (Maldonado et al. Reference Maldonado, Corona-Chávez, Solari, Ortega-Obregón and Poli2020). Therefore, the possible influence of movement of the Chortis Block on the development of the Sierra Madre del Sur cannot be ruled out (Nieto-Samaniego et al. Reference Nieto-Samaniego, Alaniz-Álvarez, Silva-Romo, Eguiza-Castro and Mendoza-Rosales2006; Maldonado et al. Reference Maldonado, Corona-Chávez, Solari, Ortega-Obregón and Poli2020) (see simplified reconstruction in Fig. 6). Based on the available chronological dataset, it cannot be proposed that the Sierra Madre del Sur was formed by a single orogenic event or by multiple pulses during Late Cretaceous to Eocene times. To resolve this doubt, additional structural and detailed thermochronological data are required from further studies of distinct areas along the whole Mexican Orogen. Nevertheless, and this is the finding of our study, the cooling rates increased systematically in the Sierra Madre del Sur from west to east approximately between Campanian and middle Eocene times. Abdullin et al. (Reference Abdullin, Solari, Solé and Ortega-Obregón2021), based on AFT results obtained from the Oaxacan Complex, suggested that the major fault systems of the Sierra Madre del Sur including the Caltepec and the Oaxaca faults (Fig. 2) have remained episodically active since, at least, Middle Triassic time. In our view, the activity of these large fault systems played a key role in the formation of geological structures as well as in the exhumation of the Sierra Madre del Sur during the Campanian–middle Eocene Laramide sensu lato.

Fig. 6. Simplified reconstruction of the tectonic evolution of southern Mexico during Campanian–Eocene time (modified from Nieto-Samaniego et al. Reference Nieto-Samaniego, Alaniz-Álvarez, Silva-Romo, Eguiza-Castro and Mendoza-Rosales2006).
Supplementary material
For supplementary material accompanying this paper visit https://doi.org/10.1017/S0016756822000929
Acknowledgements
The authors are very grateful to Juan Tomás Vazquez Ramírez and Ofelia Pérez Arvizu (both from Centro de Geociencias, UNAM) for their help with sample preparation for AFT analysis. Sandra Lorena Florez-Amaya, Alejandra Bedoya-Mejía and Ricardo Enrique Milián de la Cruz thank CONACyT for scholarships. Roberto Maldonado acknowledges DGAPA UNAM for a postdoctoral fellowship. This research was supported by the PAPIIT DGAPA UNAM project IN101520, granted to Luigi Solari. The second author also thanks Mónica Ramírez-Calderón and Sandra Guerrero Moreno (both fodongas from PCT, UNAM) for early comments that helped to improve this work. Gilby Jepson, one anonymous reviewer, and the associate editors (Professor Olivier Lacombe and Professor Guido Meinhold) are acknowledged for their constructive suggestions that improved our manuscript significantly.
Conflict of interest
None.