1. Introduction
The Ediacaran Period (635–538.8 Ma) is the most recently defined and longest geological period (Knoll et al. Reference Knoll, Walter, Narbonne and Christie-Blick2006; Xiao et al. Reference Xiao, Narbonne, Zhou, Laflamme, Grazhdankin, Moczydłowska-Vidal and Cui2016), and it encompasses a time of significant environmental and biotic changes (Droser et al. Reference Droser, Tarhan and Gehling2017; Darroch et al. Reference Darroch, Smith, Laflamme and Erwin2018; Wood et al. Reference Wood, Liu, Bowyer, Wilby, Dunn, Kenchington, Cuthill, Mitchell and Penny2019). Efforts to subdivide and better constrain timing of these events include carbon isotope chemostratigraphy and biostratigraphy based on faunas of Ediacara-type macrofossils, microfossils (acanthomorphic acritarchs) and trace fossils (Grey, Reference Grey2005; Jensen et al. Reference Jensen, Droser, Gehling, Xiao and Kaufman2006; Willman & Moczydłowska, Reference Willman and Moczydłowska2011; Narbonne et al. Reference Narbonne, Xiao, Shields, Gehling, Gradstein, Ogg, Schmitz and Ogg2012; Macdonald et al. Reference Macdonald, Strauss, Sperling, Halverson, Narbonne, Johnston, Kunzmann, Schrag and Higgins2013; Xiao et al. Reference Xiao, Narbonne, Zhou, Laflamme, Grazhdankin, Moczydłowska-Vidal and Cui2016; Rooney et al. Reference Rooney, Cantine, Bergmann, Gómez-Pérez, Al Baloushi, Boag, Busch, Sperling and Strauss2020; Darroch et al. Reference Darroch, Cribb, Buatois, Germs, Kenchington, Smith, Mocke, O’Neil, Schiffbauer, Maloney and Racicot2021), although much work remains. These efforts face challenges compared with Palaeozoic or younger strata due to taphonomic bias, a relatively low diversity of fossil organisms and a high degree of endemism (cf. Droser et al. Reference Droser, Tarhan and Gehling2017; Muscente et al. Reference Muscente, Bykov, Boag, Buatois, Mángano, Eleish, Prabhu, Pan, Meyer, Schiffbauer, Fox, Hazen and Knoll2019). Use of trace fossils and biomineralizing taxa as biostratigraphic indicators is mostly applied to upper Ediacaran strata (e.g. Jensen et al. Reference Jensen, Droser, Gehling, Xiao and Kaufman2006; Tarhan et al. Reference Tarhan, Myrow, Smith, Nelson and Sadler2020; Darroch et al. Reference Darroch, Cribb, Buatois, Germs, Kenchington, Smith, Mocke, O’Neil, Schiffbauer, Maloney and Racicot2021; Chai et al. Reference Chai, Wu and Hua2021). However, through integration of diverse types of palaeontological and palaeoenvironmental records, a better understanding of the event timeline is emerging, and the community is moving towards a subdivision of the Ediacaran Period at stage level and a better understanding of the sequence of evolutionary events (Xiao et al. Reference Xiao, Narbonne, Zhou, Laflamme, Grazhdankin, Moczydłowska-Vidal and Cui2016; Shahkarami et al. Reference Shahkarami, Buatois, Mángano, Hagadorn and Almond2020).
Organic-walled microfossils (OWM; including acritarchs) are used as a proxy for diversity of eukaryotic microbiota and are also one of the biostratigraphic tools for constraining the age of Ediacaran successions (e.g. Grey, Reference Grey2005; Xiao et al. Reference Xiao, Narbonne, Zhou, Laflamme, Grazhdankin, Moczydłowska-Vidal and Cui2016). Acritarchs are a polyphyletic group of mostly single-celled organisms, likely representing a variety of microscopic eukaryotes. They are composed of acid-insoluble organic matter and commonly preserved as compressed carbonaceous vesicles in fine-grained siliciclastics, or by permineralization in cherts or phosphorites. Historically, three assemblages of OWM have been identified during the Ediacaran Period (Grey et al. Reference Grey, Walter and Calver2003; Grey, Reference Grey2005): Ediacaran leiosphere palynoflora (ELP); Ediacaran complex acanthomorph palynoflora (ECAP, also referred to as Doushantuo–Pertatataka acritarchs (DPA)) dominated by large process-bearing forms; and late Ediacaran leiosphere palynoflora (LELP), composed mainly of sphaeromorphic OWM. Eukaryotic microfossils were especially diverse during early Ediacaran time as exemplified by taxonomically rich assemblages from Australia (Zang & Walter, Reference Zang and Walter1992; Grey, Reference Grey2005; Willman & Moczydłowska, Reference Willman and Moczydłowska2011), China (Xiao et al. Reference Xiao, Zhou, Liu, Wang and Yuan2014; Liu & Moczydłowska, Reference Liu and Moczydłowska2019), the East European Platform (Vorob’eva et al. Reference Vorob’eva, Sergeev and Knoll2009), Siberia (Sergeev et al. Reference Sergeev, Knoll and Vorob’eva2011; Moczydłowska & Nagovitsin, Reference Moczydłowska and Nagovitsin2012), India (Prasad et al. Reference Prasad, Asher and Borgohai2010; Joshi & Tiwari, Reference Joshi and Tiwari2016) and Laurentia (Willman et al. Reference Willman, Peel, Ineson, Schovsbo, Rugen and Frei2020). They have been used in biostratigraphic endeavours to correlate Ediacaran strata (e.g. Grey, Reference Grey2005; Xiao et al. Reference Xiao, Narbonne, Zhou, Laflamme, Grazhdankin, Moczydłowska-Vidal and Cui2016), usually antedating the widespread occurrence of the macroscopic Ediacara-type biota (see Xiao et al. Reference Xiao, Narbonne, Zhou, Laflamme, Grazhdankin, Moczydłowska-Vidal and Cui2016). In contrast, the ELP and LELP assemblages are generally depauperate, mostly consisting of simple leiosphaerid acritarchs that possess few distinguishing characters, or other non-diagnostic taxa with very long stratigraphic ranges (e.g. Grey, Reference Grey2005; Chiglino et al. Reference Chiglino, Gaucher, Sial and Ferreira2015; Kolesnikov et al. Reference Kolesnikov, Marusin, Nagovitsin, Maslov and Grazhdankin2015; Lehn et al. Reference Lehn, Horodyski and Paim2019). Exceptions were discovered recently; some acanthomorphic (process-bearing) OWM persisted to the latest Ediacaran of Mongolia (Anderson et al. Reference Anderson, McMahon, Macdonald, Jones and Briggs2019) and Russia (Grazhdankin et al. Reference Grazhdankin, Nagovitsin, Golubkova, Karlova, Kochnev, Rogov and Marusin2020). Such assemblages remain rare towards the end of the Ediacaran Period and could have been restricted to specific environments where acanthomorphs thrived or were able to be preserved. More upper Ediacaran strata need to be examined for microfossils in detail, and their utility in a more refined stratigraphic subdivision of the later Ediacaran Period (the upper series) is in progress.
Only a handful of body-fossil taxa span the Ediacaran–Cambrian boundary (Slater et al. Reference Slater, Harvey, Bekker and Butterfield2020). Recent studies have shown that several fossil groups persisted through this interval, including skeletal metazoan taxa (cloudinids), small carbonaceous fossils (SCF) and organic problematica (Moczydłowska et al. Reference Moczydłowska, Budd and Agić2015; Yang et al. Reference Yang, Steiner, Zhu, Liu and Liu2016; Zhu et al. Reference Zhu, Zhuravlev, Wood, Zhao and Sukhov2017; Slater et al. Reference Slater, Harvey, Bekker and Butterfield2020). Microfossils in this study show a similar trend; we found the predominantly Cambrian taxon Granomarginata Naumova (Reference Naumova1960) deeper in time, within Ediacaran strata representing a shallow-water to distal shelf-marine environment in Arctic Norway, and nearshore shallow-water setting in Namibia and Newfoundland, Canada (Figs 1–4). In addition to Granomarginata previously reported from India (Kumar & Maithy, Reference Kumar and Maithy2008; Prasad et al. Reference Prasad, Asher and Borgohai2010) and Poland (Jachowicz-Zdanowska, Reference Jachowicz-Zdanowska2011), our new records suggest a geographically widespread distribution of Granomarginata by late Ediacaran time. We further report additional diagnostic OWM co-occurring with Granomarginata, including the new flask-shaped taxon Lagoenaforma collaris gen. et sp. nov.

Fig. 1. Localities of sample collections and outcrops with occurrences of Granomarginata and Lagoenaforma gen. nov. (a) Basal part of the Indreelva Member of the Stáhpogieddi Formation, Vestertana Group in coastal outcrops in northern portion of Árasulluokta Cove, southeastern shore of the Digermulen Peninsula in Norway. (b) Shales and siltstones of the Indreelva Member above the occurrence of macrofossil Palaeopascichnus. (c) Basal Nama Group strata on Farm Pockenbank in the Witputs sub-basin in Namibia. (d) Fine arenite of the Mara Member, Dabis Formation, Nama Group exposed on Farm Pockenbank. (e) Gibbett Hill Formation, exposed at the ‘Brasier Shale’ outcrop on the northern shore of Ferryland Head, eastern coast of the Avalon Peninsula, Newfoundland. This locality was named in honour of Professor Martin Brasier who spent many field excursions studying this outcrop. (f) Enhanced view of the Brasier Shale in the upper Gibbet Hill Formation.

Fig. 2. Simplified schematic regional stratigraphy of the units from which Granomarginata and Lagoenaforma microfossils were recovered, showing the occurrence of microfossils in relation to the Ediacara-type biota and other stratigraphic markers. Stratigraphic markers from each unit are included to inform about the broad age-rage of Granomarginata–Lagoenaforma. (a) Ediacaran part of the Vestertana Group exposed on the Digermulen Peninsula in Arctic Norway. The Ediacaran–Cambrian boundary is in the upper part of the Manndrapselva Member, indicated by the first occurrence (FO) of Treptichnus pedum. For the distribution of palaeopascichnids and trace fossils in the Vestertana Group see Jensen et al. (Reference Jensen, Högström, Høyberget, Meinhold, McIlroy, Ebbestad, Taylor, Agić and Palacios2018 b). (b) Stratigraphy of the Nama Group in the Witputs sub-basin, Namibia, modified from Hall et al. (Reference Hall, Kaufman, Vickers-Rich, Ivantsov, Trusler, Linnemann, Hofmann, Elliott, Cui, Fedonkin and Hoffmann2013). Previous record of depauperate microfossils from Germs et al. (Reference Germs, Knoll and Vidal1986). U–Pb zircon age constraints in the Spitzkop Member, Urusis Formation from Linnemann et al. (Reference Linnemann, Ovtcharova, Schaltegger, Gärtner, Hautmann, Geyer, Vickers-Rich, Rich, Plessen, Hofmann and Zieger2019). (c) Stratigraphy of the Conception, St John’s and Signal Hill Groups, based on King (Reference King1988) and Matthews et al. (Reference Matthews, Liu, Yang, McIlroy, Levell and Condon2021). The stratigraphic position of the ‘Brasier Shale’ outcrop at Ferryland Head is indicated.

Fig. 3. (a) Microfossil occurrence close to Palaeopascichnus and aspidellomorphs in the lower Indreelva Member, Digermulen Peninsula, Norway. (b) Microfossil occurrence in the Mara Member, Farm Pockenbank, Namibia.

Fig. 4. Transmitted light photomicrographs of the Ediacaran–Cambrian acritarch taxon Granomarginata from various Ediacaran units. (a–d) Granomarginata squamacea. (a) G. squamacea from the Mara Member, Dabis Formation, Nama Group on Farm Pockenbank, Namibia. N16-HA-P2 82×13. (b) N16-HA-P3 81×9, Mara Member. (c) TSGf18448, D18-HA-20 83×16, Indreelva Member, Stáhpogieddi Formation, Vestertana Group on Digermulen Peninsula, Arctic Norway. (d) TSGf18449a, D16-HA-80 87×19, Indreelva Member. (e, f) Granomarginata prima from the Indreelva Member in Norway. (e) TSGf18449b, D16-HA-80 84.5×18. (f) TSGf18450a, D16-HA-53 81×14.
2. Methods
Microfossils were isolated from the rock matrix utilizing a palynological acid extraction procedure (Grey, Reference Grey1999) that includes maceration in 40% hydrofluoric acid (HF) to dissolve silicates, and boiling of the residue in 30% hydrochloric acid (HCl) to remove fluorides. Calcareous fine sandstone samples were first macerated in HCl for > 24 hours to remove carbonates. Residue was filtered through 10 µm mesh and used to prepare strew mounts for a light microscopy overview. Microfossils were observed and imaged with a Zeiss Axioskop 40 transmitted light microscope with Q Imaging camera. All illustrated specimens from the Digermulen Peninsula will be deposited in the palaeontological collections (TSGf) of the Arctic University Museum of Norway, Tromsø, and specimens from Nambia at the Geological Survey of Namibia, Windhoek. To evaluate abundance and diversity, all microfossils were counted on one slide per sample.
Fossiliferous samples were collected from middle–upper Ediacaran strata in three distinct areas: Arctic Norway, Namibia and Newfoundland. Granomarginata was recovered from a new locality as well as in strata older than previously reported, which prompted a comparison of the OWM assemblages between these areas.
3. Geological setting
3.a. Arctic Norway
Samples from Arctic Norway were collected during the 2016 and 2018 field expeditions of the Digermulen Early Life Research Group (DELRG). The Digermulen Peninsula in the Finnmark region of mainland Arctic Norway hosts an approximately 3-km-thick succession of mainly siliciclastic sedimentary rocks of Cryogenian–Ordovician age (e.g. Högström et al. Reference Högström, Jensen, Palacios and Ebbestad2013; Figs 1a, b, 2a, 3a). The base of the Vestertana Group contains glaciogenic diamictites of the Smalfjorden and Mortensnes formations that have been linked to the Marinoan and Gaskiers glacial intervals, respectively (Halverson et al. Reference Halverson, Hoffman, Schrag, Maloof and Rice2005). These sequences bracket the siliciclastic shallow-marine to basinal, non-glacial Nyborg Formation. Organic-walled microfossil taxa characteristic of the early Ediacaran Period (ECAP/DPA), as well as organically preserved remnants of multicellular tissue, have been documented in the upper part of the Nyborg Formation (Agić et al. Reference Agić, Högström, Jensen, Ebbestad, Meinhold, Taylor, Palacios and Høyberget2018, Reference Agić, Högström, Moczydłowska, Jensen, Palacios, Meinhold, Ebbestad, Taylor and Høyberget2019). Overlying the Mortensnes diamictite is the Stáhpogieddi Formation, which starts with sandstones and shales of the Lillevannet Member, representing a transgressive interval (Banks et al. Reference Banks, Edwards, Geddes, Hobday and Reading1971; Jensen et al. Reference Jensen, Högström, Høyberget, Meinhold, McIlroy, Ebbestad, Taylor, Agić and Palacios2018 b). Above lies the Indreelva Member composed of mudstones, siltstones and sandstones, which hosts an assemblage of Ediacara-type macrofossils dominated by discoidal taxa (Farmer et al. Reference Farmer, Vidal, Moczydłowska, Strauss, Ahlberg and Siedlecka1992; Högström et al. Reference Högström, Jensen, Palacios and Ebbestad2013, Reference Högström, Jensen, Ebbestad, Taylor, Høyberget, Agić, Meinhold and Palacios2017; Jensen et al. Reference Jensen, Högström, Høyberget, Meinhold, McIlroy, Ebbestad, Taylor, Agić and Palacios2018 b). The overlying Manndrapselva Member consists of a basal sandstone unit, followed by two upwards-coarsening cycles of red and grey mudstone and sandstone alterations and, in the second cycle, carbonate concretions and calcareous siliciclastic beds (Meinhold et al. Reference Meinhold, Jensen, Høyberget, Arslan, Ebbestad, Högström, Palacios, Agić and Taylor2019 a). The stratigraphically highest occurrence of macrofossils Palaeopascichnus and Harlaniella is c. 15 m above the carbonates, and the Ediacaran–Cambrian boundary lies close to the base of the third cycle, indicated by the occurrence of the trace fossil Treptichnus pedum (Fig. 2a; McIlroy & Brasier, Reference McIlroy, Brasier, Brasier, McIlroy and McLoughlin2017; Jensen et al. Reference Jensen, Högström, Almond, Taylor, Meinhold, Høyberget, Ebbestad, Agić and Palacios2018 a, b).
Fossiliferous samples discussed here were collected from shales and siltstones in the basal part of Indreelva Member from Árasulluokta Cove (Fig. 1a, b). Samples D16-HA-80 (70° 34.165ʼ N, 28° 07.224ʼ E) and D18-HA-20 (70° 34.174ʼ N, 28° 07.204ʼ E, just above a bed with discoidal fossils), were collected 3 m and c. 10 m above the lowest occurrence of palaeopascichnids (cf. Jensen et al. Reference Jensen, Högström, Høyberget, Meinhold, McIlroy, Ebbestad, Taylor, Agić and Palacios2018 b). Sample D16-HA-53 was recovered 2 m below the earliest aspidellomorphs in the Stáhpogieddi section, 6 m above the Lillevannet–Indreelva transitional beds (70° 32.534ʼ N, 28° 00.929ʼ E). The sample from the Manndrapselva Member third cycle at the Manndrapselva River section (D16-HA-77, 70° 34.575ʼ N, 28° 06.847ʼ E) was collected immediately above the quartzite marking the top of the second cycle.
3.b. Namibia
Fossiliferous samples from Namibia were collected from the Dabis Formation during the field workshop on the Ediacaran Nama Group of southern Namibia, part of the IGCP 587 programme (Xiao et al. Reference Xiao, Vickers-Rich, Narbonne, Laflamme, Darroch, Kaufman and Kriesfeld2017) on Farm Pockenbank (Figs 1c, d, 2b, 3b). The Nama Group contains c. 3 km of fluvial to marine siliciclastics and carbonates, representing a tidal to below-wave-base environment of a foreland basin (Germs et al. Reference Germs, Knoll and Vidal1986; Germs & Gresse, Reference Germs and Gresse1991). It occurs in a northern Zaris sub-basin and a southern Witputz sub-basin, separated by the Osis Ridge (Germs, Reference Germs1983). The lower part of the Nama, the Kuibis Subgroup, consists of 200 m of mature siliciclastics and carbonates, and is subdivided into the Dabis and Zaris formations (Germs, Reference Germs1983; Saylor et al. Reference Saylor, Grotzinger and Germs1995). In the Pockenbank area (Witputz sub-basin), the lowermost Kanies Member of the Dabis Formation contains predominantly arkosic sandstones with ripples and desiccation cracks indicative of shallow, fluvial environments (Germs, Reference Germs1983; Saylor et al. Reference Saylor, Grotzinger and Germs1995). The Mara Member overlies the transgressively eroded top of the Kanies Member, and contains alternating fine-grained siliciclastics and limestones with microbialites and evaporites within a shallowing-upwards sequence (Germs, Reference Germs1972 b, Reference Germs1983; Saylor et al. Reference Saylor, Grotzinger and Germs1995). Above the Mara Member are the Kliphoek and Aar members that consist of cross-stratified coarse sandstones and carbonates grainstone (Germs, Reference Germs1983; Saylor et al. Reference Saylor, Grotzinger and Germs1995; Hall et al. Reference Hall, Kaufman, Vickers-Rich, Ivantsov, Trusler, Linnemann, Hofmann, Elliott, Cui, Fedonkin and Hoffmann2013). Saylor et al. (Reference Saylor, Grotzinger and Germs1995) interpreted the Dabis Formation strata as two transgressive sequences. Some of the oldest examples of the youngest Ediacaran evolutionary assemblage – the Nama assemblage – occur in the Kliphoek/Aar members (e.g. Germs, Reference Germs1983; Narbonne et al. Reference Narbonne, Saylor and Grotzinger1997; Vickers-Rich et al. Reference Vickers-Rich, Ivantsov, Trusler, Narbonne, Hall, Wilson, Greentree, Fedonkin, Elliott, Hoffmann and Schneider2013; Maloney et al. Reference Maloney, Boag, Facciol, Gibson, Cribb, Koester, Kenchington, Racicot, Darroch and Laflamme2020). Carbonates (micrite phases) in the Mara Member (Arasab section) preserve a negative δ13C excursion from −6.22‰ to −0.22‰, previously correlated with the recovery from the global Shuram–Wonoka anomaly (Kaufmann et al. Reference Kaufman, Hayes, Knoll and Germs1991; Saylor et al. Reference Saylor, Grotzinger and Germs1995, Reference Saylor, Kaufman, Grotzinger and Urban1998; Grotzinger et al. Reference Grotzinger, Bowring, Saylor and Kaufman1995; Hall et al. Reference Hall, Kaufman, Vickers-Rich, Ivantsov, Trusler, Linnemann, Hofmann, Elliott, Cui, Fedonkin and Hoffmann2013; Wood et al. Reference Wood, Poulton, Prave, Hoffmann, Clarkson, Guilbaud, Lyne, Tostevin, Bowyer, Penny and Curtis2015). The excursion is not fully manifested at the locality where the microfossils were collected (cf. Vickers-Rich et al. Reference Vickers-Rich, Narbonne, Laflamme, Darroch, Kaufman and Kriesfeld2016). Sandstones of the overlying Kliphoek and Aar members include fossils in offshore-shoreface settings, characteristic environments inhabited by the late Ediacaran Nama assemblage including such taxa as Ernietta, Pteridinium and Rangea, as well as macroscopic bacterial colonies Nemiana (Narbonne et al. Reference Narbonne, Saylor and Grotzinger1997, Reference Narbonne, Xiao, Shields, Gehling, Gradstein, Ogg, Schmitz and Ogg2012; Vickers-Rich et al. Reference Vickers-Rich, Ivantsov, Trusler, Narbonne, Hall, Wilson, Greentree, Fedonkin, Elliott, Hoffmann and Schneider2013). These macrofossiliferous strata span the late Ediacaran Period: an ash bed in the Kuibis Subgroup yielded a U–Pb age of 548.8 ± 1 Ma (Grotzinger et al. Reference Grotzinger, Bowring, Saylor and Kaufman1995), and ash beds from the Spitskop Member of the overlying Urusis Formation yielded ages of 538.99 ± 0.21 Ma in the most recent study using U–Pb chemical abrasion – isotope dilution – thermal ionization imaging spectrometry (CA-ID-TIMS) dating technique (Linnemann et al. Reference Linnemann, Ovtcharova, Schaltegger, Gärtner, Hautmann, Geyer, Vickers-Rich, Rich, Plessen, Hofmann and Zieger2019).
Collection for a pilot microfossil survey was carried out from the lower Mara Member, Nama Group on Farm Pockenbank, at the Quiver section (see Vickers-Rich et al. Reference Vickers-Rich, Narbonne, Laflamme, Darroch, Kaufman and Kriesfeld2016): N16-HA-P2 c. 9 m above the base of the Mara Member (27° 08.619ʼ S, 16° 26.803ʼ E), and N16-HA-P3 immediately above a grey limestone package. These strata are overlain by grey and pink dolomite, and dark limestone at the top.
3.c. Newfoundland, Canada
The microfossiliferous Gibbett Hill Formation of the Signal Hill Group is exposed on the eastern Avalon Peninsula, Newfoundland, Canada (Sala Toledo, Reference Sala Toledo2004; Hofmann et al. Reference Hofmann, O’Brien and King2008). The Signal Hill Group overlies the fossiliferous strata of the Conception and St John’s groups, which contain some of the oldest Ediacara-type macrofossils, that is, the Avalon assemblage (Fig. 2c; Misra, Reference Misra1969; Narbonne in Fedonkin et al. Reference Fedonkin, Mikhail, Gehling, Grey, Narbonne and Vickers-Rich2007; Liu et al. Reference Liu, Kenchington and Mitchell2015; Matthews et al. Reference Matthews, Liu, Yang, McIlroy, Levell and Condon2021). Temporal constraints in the upper part of this succession are generally scarce, but the Conception Group contains a glacial diamictite (Gaskiers Formation) dated between 580.90 ± 0.40 and 579.88 ± 0.44 Ma using CA-ID-TIMS U–Pb analyses on zircon grains (Pu et al. Reference Pu, Bowring, Ramezani, Myrow, Raub, Landing, Mills, Hodgin and Macdonald2016), and the rangeomorphs from the Fermeuse Formation in the upper St John’s Group, have a maximum age of 564.13 ± 0.65 Ma (Matthews et al. Reference Matthews, Liu, Yang, McIlroy, Levell and Condon2021). A tuffite sample from the lower Fermeuse Formation yielded zircon U–Pb dates from 563.67–569.01 Ma (Matthews et al. Reference Matthews, Liu, Yang, McIlroy, Levell and Condon2021).
The overlying Signal Hill Group contains c. 1500 m of mudstones, fine-grained sandstones and ash beds (Sala Toledo, Reference Sala Toledo2004). Its oldest unit is the Cappahayden Formation, containing laminated grey siltstones. It is overlain by the Gibbett Hill Formation consisting of 760 m of green-grey sandstone, mudstone and black shales (Sala Toledo, Reference Sala Toledo2004) and deposited in a shallow-marine environment.
Rare OWM dominated by sphaeromorphs were previously reported from siliciclastics of the Drook, Mall Bay, Fermeuse and Renews Head formations in the St John’s Group (Hofmann et al. Reference Hofmann, Hill and King1979; O’Brien & King, Reference O’Brien and King2004), and the Cappahayden Formation (underlying the Gibbett Hill Formation) in the Signal Hill Group (Hofmann et al. Reference Hofmann, Hill and King1979). The microfossils in this study derive from a single sample of the ‘Brasier Shale’ outcrop in the Gibbett Hill Formation, near Ferryland, Avalon Peninsula, Newfoundland (Fig. 1e–f).
4. Results
A flask-shaped OWM Lagoenaforma gen. nov. (Fig. 5) was found in several upper Ediacaran units. This taxon frequently occurs with rare Granomarginata squamacea and G. prima, which are typically early Cambrian taxa. This new material, along with previous reports (Fig. 4, Table 1), extend the distribution of Granomarginata back in time into the late Ediacaran Period. These OWM also co-occur with leiosphaerids and carbonaceous problematica (Fig. 6).

Fig. 5. Transmitted light photomicrographs of the new flask-shaped organic-walled microfossils Lagoenaforma gen. nov. from the middle–upper Ediacaran strata. (a) Lagoenaforma collaris gen. et sp. nov. from the Indreelva Member, Stáhpogieddi Formation, Vestertana Group on Digermulen Peninsula, Norway. (a) Holotype, TSGf18449c, D16-HA-80 78×15. (b, c) L. collaris from the Mara Member, Dabis Formation, Nama Group on Farm Pockenbank in Namibia. (b) N16-HA-P2 76×7. (c) N16-HA-P2 77×5.5. (d) Unnamed Form A, microfossil with a neck-like protrusion from the Manndrapselva Member, Stáhpogieddi Formation, Vestertana Group. TSGf18451a, D16-HA-77 89×23. (e) Opaque neck-bearing microfossil Lagoenaforma sp. from the Gibbett Hill Formation, Signal Hill Group, Avalon Peninsula, Newfoundland. Brasier Shale A-1 87×11.
Table 1. A list of Ediacaran units containing the Granomarginata–Lagoenaforma association or either of the two taxa, and their approximate ages. Where this information is available, all organic-walled microfossils (OWM) are of low abundance, consistent with characterization of depauperate late Ediacaran leiosphere palynoflora (LELP) assemblage (cf. Volkova et al. Reference Volkova, Kirjanov, Piscun, Pashkyavichene, Jankauskas, Keller and Rozanov1979; Grey, Reference Grey2005).


Fig. 6. Other common organic-walled microfossils co-occurring with Granomarginata and Lagoenaforma gen. nov. in units of middle–late Ediacaran age, common components of the late Ediacaran leiosphaerid palynoflora (LELP, cf. Grey, Reference Grey2005) as well as older Precambrian assemblages, and small carbonaceous fossils. (a) Leiosphaeridia jacutica from the upper Mara Member, Dabis Formation, Nama Group on Farm Pockenbank, Namibia. N16-HA-P2 78×19. (b) Leiosphaeridia crassa from the Mara Member, N16-HA-P2 89×11. (c) Unnamed Form B, a small carbonaceous problematicum with lateral protrusions, from the Gibbett Hill Formation, Signal Hill Group, Avalon Peninsula, Newfoundland. Brasier Shale A-1 91×11. (d) L. crassa from the Indreelva Member, Stáhpogieddi Formation, Vestertana Group in Norway. TSGf18449d, D16-HA-80 85.5×9. (e) Unnamed Form C, tapering elongated and annulated microfossil from the Indreelva Member. TSGf18450b, D16-HA-53 85×9. (f) Simia annulare from the Mara Member, Dabis Formation, Nama Group in Namibia. N16-HA-P2 77×10. (g) SCF from the Manndrapselva Member, Stáhpogieddi Formation. TSGf18451b, D16-HA-77 88×14. (h) Bavlinella sp. from the Mara Member. N16-HA-P2 80×11. (i) Symplassosphaeridium sp. from the Indreelva Member. TSGf18449e, D16-HA-80 80×18. (j) SCF problematicum from the Mara Member. N16-HA-P2 77×16. (k) Lobate or dividing acritarch, from the Indreelva Member. TSGf18449f, D16-HA-80 81×7. (l) Fragmented microfossil with a spongy envelope, Granomarginata prima from the Gibbett Hill Formation, Newfoundland. Brasier Shale A-1 87×5. Scale bar is the same for all images: 25 µm. All images are transmitted light photomicrographs.
4.a. Ediacaran Indreelva Member, Stáhpogieddi Formation, Digermulen Peninsula, Norway
Out of 13 analysed samples from the Indreelva Member, 10 were barren or had very low organic content, and the rest yielded moderately well preserved OWM. These include: Granomarginata prima, G. squamacea, Leiosphaeridia crassa, L. jacutica, a tapering annulated microfossil, a lobate acritarch, fragmented SCF and aggregates of cells Symplassosphaeridium sp. A new type of flask-shaped OWM is described: Lagoenaforma collaris gen. et sp. nov. (Fig. 5a). Fragmented remains of filamentous prokaryotes and parts of rare larger SCF, as well as often torn membranous extensions of Granomarginata, are likely not a result of destructive processing because a low-manipulation acid maceration method was applied; this indicates either degradation within the sediment or transport. Leiosphaerids are the most common component of the Indreelva OWM assemblage (> 80%, Fig. 6a, b, d). There are few Granomarginata specimens; it is therefore rarer when compared with its abundance in the Cambrian strata (e.g. 3–10 counts, see Palacios et al. Reference Palacios, Högström, Ebbestad, Agić, Høyberget, Jensen, Meinhold and Talyor2020). OWM are generally scarce in the strata examined here.
The first record of G. squamacea and L. collaris in the Indreelva Member occurs in a laminated mudrock 3 m above the level hosting palaeopascichnids, and c. 10 m above the transitional beds of the Lillevannet Member (Figs 2a, 3a). Microfossils also occur c. 6 m below the first discoidal Ediacara-type macrofossils in the Indreelva Member in the Stáhpogieddi type section. Mudrock samples below the Palaeopascichnus horizon in the Árasulluokta Cove were devoid of microfossils apart from rare leiosphaerid fragments. This is likely not preservational bias because at least some OWM (leiosphaerids) are present. Granomarginata makes up 4% and L. collaris 7.5% of the Indreelva assemblage. Overall filaments and organic problematica represent nearly 10% of the assemblage. Samples from the upper part of the Indreelva Member did not yield any microfossils.
Distinct OWM are not commonly found very close to the beds containing Ediacara-type biota (Grey in Fedonkin et al. Reference Fedonkin, Mikhail, Gehling, Grey, Narbonne and Vickers-Rich2007). Although microfossils in the Indreelva Member are rare and occur sporadically, these results from the Digermulen Peninsula represent a rare distribution of acritarchs and Ediacaran macrofossils within a few metres of each other.
4.b. Ediacaran–Cambrian Manndrapselva Member, Stáhpogieddi Formation, Digermulen Peninsula, Norway
Granomarginata was previously documented higher up in the stratigraphy on the Digermulen Peninsula, in association with leiosphaerids in the third cycle of the Manndrapselva Member of the Stáhpogieddi Formation, correlated with the basal Terreneuvian (Högström et al. Reference Högström, Jensen, Palacios and Ebbestad2013), as well as in the Cambrian Series 2 to Miaolingian Duolbagáisá Formation further up in the stratigraphy (Palacios et al. Reference Palacios, Högström, Ebbestad, Agić, Høyberget, Jensen, Meinhold and Talyor2020). Only two samples (one fossiliferous) from the Manndrapselva Member below the Ediacaran–Cambrian boundary were analysed here, but no Granomarginata was recovered. Instead, these strata contain organic problematica such as a neck-bearing microfossil (Fig. 5d), as well as fragments of leiosphaerids, bacterial filaments and SCF. Palacios et al. (Reference Palacios, Ou, Högström, Jensen, Høyberget, Meinhold, Taylor, Ebbestad and Agić2017) observed lobate SCF problematica upsection in the third cycle similar to microfossils from the Gibbett Hill Formation on Newfoundland (Fig. 6c).
4.c. Mara Member assemblage, Dabis Formation, Nama Group, Farm Pockenbank, Namibia
Organic-walled microfossils recovered from the Mara Member of the Nama Group on Farm Pockenbank occur in fine sandstones/silts interbedded with limestones, deposited in a shallow-marine environment. Taxa include: Granomarginata squamacea, Lagoenaforma collaris gen. et sp. nov., Leiosphaeridia crassa, Simia annulare (Fig. 6f), Bavlinella sp. (Fig. 6h), Symplassosphaeridium sp. and vesicle fragments of smooth-walled microfossils or possible SCF (Fig. 6j). Fragments of broad filaments are rare, but similar to material identified as Vendotaenia sp. from the Kuibis Subgroup by Germs et al. (Reference Germs, Knoll and Vidal1986). The newly reported microfossils here occur below strata containing possible first occurrence of cloudinids (following an unillustrated report of Cloudina by Germs, Reference Germs1972 a; cf. Wood et al. Reference Wood, Poulton, Prave, Hoffmann, Clarkson, Guilbaud, Lyne, Tostevin, Bowyer, Penny and Curtis2015), and they are uncomformably overlain by the Kliphoek and Aar members, which contain the Ediacara-type macrofossils Ernietta (Pflug, Reference Pflug1966; Elliot et al. Reference Elliott, Trusler, Narbonne, Vickers-Rich, Morton, Hall and Schneider2016), Pteridinium (Gürich, Reference Gürich1930) and Rangea (Narbonne et al. Reference Narbonne, Saylor and Grotzinger1997; Vickers-Rich et al. Reference Vickers-Rich, Ivantsov, Trusler, Narbonne, Hall, Wilson, Greentree, Fedonkin, Elliott, Hoffmann and Schneider2013) characteristic of the late Ediacaran Nama assemblage (Narbonne et al. Reference Narbonne, Saylor and Grotzinger1997).
OWM from the weathered sediments of the Mara Member are slightly lighter in colour than the specimens from Newfoundland and Arctic Norway (thermal alteration index (TAI) = 3–4 sensu Hayes et al. Reference Hayes, Kaplan, Wedeking and Schopf1983), which indicates a lower degree of thermal alteration. Leiosphaerids make up the bulk of the OWM assemblage in the Mara Member (c. 80 % of the overall microfossil abundance). Cell aggregates Symplassosphaeridium are the next most common component. Granomarginata represents 3.5% and L. collaris 6% of the assemblage. This preliminary record of Ediacaran OWM from Namibia, despite being of low diversity, is encouraging future explorations of the late Ediacaran microfossil record hosted in the Nama Group.
4.d. Gibbett Hill Formation microfossils, Signal Hill Group, Avalon Peninsula, Newfoundland, Canada
The Gibbett Hill Formation contains rare and poorly to moderately preserved OWM. The most common component are fragments of prokaryotic filaments and leiosphaerids. As a low-manipulation acid maceration method was used, the fragmentation is likely not a result of palynological processing and could instead indicate transport. Additional microfossils include prokaryotic clusters of cells Symplassosphaeridium sp., a small carbonaceous problematicum with lateral protrusions (Unnamed Form B, Fig. 6c), a fragment of a single-celled microfossil with a spongy envelope – likely Granomarginata prima (Fig. 6l) – and a dark flask-like microfossil with an elongate neck-like structure Lagoenaforma sp. (Fig. 5e). The dark colour of the Gibbett Hill OWM indicates high thermal alteration of the organic matter. Only a single specimen of a poorly preserved Granomarginata has been recovered. However, due to the presence of the late Ediacaran organic problematica (Fig. 6c) and L. collaris, which co-occur with Granomarginata in the Ediacaran strata of Norway and Namibia, the identification of envelope-bearing microfossil from Gibbett Hill as Granomarginata is plausible. These microfossils occur hundreds of metres above the fossiliferous successions hosting some of the oldest assemblage of Ediacaran macrofossils (cf. Hofmann et al. Reference Hofmann, O’Brien and King2008; Liu et al. Reference Liu, Kenchington and Mitchell2015; Matthews et al. Reference Matthews, Liu, Yang, McIlroy, Levell and Condon2021), consistent with a broadly late Ediacaran age of the Gibbett Hill Formation.
5. Discussion
5.a. Ediacaran Granomarginata
The lower Ediacaran strata accommodate a rich and diverse record of organically preserved microfossils of biostratigraphic importance (e.g. Zang, Reference Zang1988; Grey, Reference Grey2005; Vorob’eva et al. Reference Vorob’eva, Sergeev and Knoll2009; Sergeev et al. Reference Sergeev, Knoll and Vorob’eva2011; Willman & Moczydłowska, Reference Willman and Moczydłowska2011). On the contrary, however, the strata postdating localized short-lived glaciations, the Shuram negative carbon isotope excursion (CIE) and the first appearance of the macroscopic Ediacara-type biota are generally depauperate of microfossils, with little distinctive morphologies. The strata bearing Ediacaran macrofossils rarely contain acritarchs, mainly leiosphaerids and prokaryotes (cf. Hofmann et al. Reference Hofmann, Hill and King1979; Grey, Reference Grey2005; Leonov & Ragozina, Reference Leonov, Ragozina, Vickers-Rich and Komarower2007) in lower abundance than in older, pre-Gaskiers or pre-Shuram strata. This pattern is observed on the Digermulen Peninsula where the lower Ediacaran Nyborg Formation contains acanthomorphs (Agić et al. Reference Agić, Högström, Jensen, Ebbestad, Meinhold, Taylor, Palacios and Høyberget2018), whereas the strata above in the Stáhpogieddi Formation are mostly barren and relatively depauperate until Cambrian time. OWM assemblages of low diversity, with few eukaryotic forms, have also been reported from Argentina, Australia, Brazil, East European Platform, Namibia and Siberia (Germs et al. Reference Germs, Knoll and Vidal1986; Gaucher et al. Reference Gaucher, Boggiani, Sprechmann, Sial and Fairchild2003; Grey, Reference Grey2005; Leonov & Ragozina, Reference Leonov, Ragozina, Vickers-Rich and Komarower2007; Chiglino et al. Reference Chiglino, Gaucher, Sial and Ferreira2015; Kolesnikov et al. Reference Kolesnikov, Marusin, Nagovitsin, Maslov and Grazhdankin2015; Ragozina et al. Reference Ragozina, Dorjnamjaa, Serezhnikova, Zaitseva and Enkhbaatar2016; Arrouy et al. Reference Arrouy, Gaucher, Poiré, Xiao, Peral, Warren, Bykova and Quaglio2019; Arvestål & Willman, Reference Arvestål and Willman2020). Late Ediacaran acanthomorphic acritarchs were found in Mongolia (Anderson et al. Reference Anderson, McMahon, Macdonald, Jones and Briggs2019) and in a drillcore from Siberia (Grazhdankin et al. Reference Grazhdankin, Nagovitsin, Golubkova, Karlova, Kochnev, Rogov and Marusin2020), but these occurrences are exceptions among the generally low-diversity late Ediacaran OWM assemblages.
The organic-walled microfossil genus Granomarginata is one of the more distinguishable OWM taxa of the Terreneuvian epoch, known from units in Canada, China, the East European Platform, Finland, India, Norway, Siberia, and Spain (Moczydłowska, Reference Moczydłowska1991, Reference Moczydłowska2011; Palacios & Moczydłowska, Reference Palacios and Moczydłowska1998; Kumar & Maithy, Reference Kumar and Maithy2008; Yin et al. Reference Yin, Yang, Peng and Kong2009; Palacios et al. Reference Palacios, Jensen, Barr, White and Myrow2018, Reference Palacios, Högström, Ebbestad, Agić, Høyberget, Jensen, Meinhold and Talyor2020; Slater & Willman, Reference Slater and Willman2019), and was also reported from the uppermost Ediacaran strata of India (Prasad et al. Reference Prasad, Asher and Borgohai2010) and Poland (Gunia, Reference Gunia1990; Jachowicz-Zdanowska, Reference Jachowicz-Zdanowska2011). It is a common component of the Granomarginata prima Zone of the East European Platform (EEP) (Jankauskas & Lendzion, Reference Jankauskas and Lendzion1992), the Granomarginata Zone in Newfoundland (Palacios et al. Reference Palacios, Jensen, Barr, White and Myrow2018), and rare to common in abundance in Skiagia-bearing zones (e.g. Palacios et al. Reference Palacios, Jensen, Barr, White and Myrow2018, Reference Palacios, Högström, Ebbestad, Agić, Høyberget, Jensen, Meinhold and Talyor2020) that characterize the latest Terreneuvian and Cambrian Series 2. The new record documented here extends its distribution deeper in time as well as to a new region: Namibia.
Granomarginata is a single-celled eukaryote consisting of a central body and an uneven equatorial extension with granular surface. Its morphology resembles a phycoma of prasinophyte algae (Moczydłowska, Reference Moczydłowska2011). Because of its widespread palaeogeographic distribution and occurrence in sediments deposited in both shallow and deep waters, it is assumed to have been a cyst of a planktonic organism. Microfossils with distinct morphological elements (e.g. processes and envelopes) provide a useful biostratigraphic tool, and the envelope-bearing Granomarginata is one of the few non-leiosphaerid acritarchs present in low-diversity microfossil assemblages of upper Ediacaran strata, in addition to organically preserved problematica (cf. Golubkova & Raevskaya, Reference Golubkova, Raevskaya, Steemans and Javaux2005; Leonov & Ragozina, Reference Leonov, Ragozina, Vickers-Rich and Komarower2007; Moczydłowska et al. Reference Moczydłowska, Budd and Agić2015; Slater et al. Reference Slater, Harvey, Bekker and Butterfield2020). Microfossils of this material differ from more ubiquitous Proterozoic envelope-bearing taxa such as Simia (also present in the Nama Group) in their fluffy, granular envelope with an uneven outline, which is likely a result of its less resistant nature compared with the central body (Naumova, Reference Naumova1960; Moczydłowska, Reference Moczydłowska1991). Although the material from Norway and Newfoundland is relatively poorly preserved, it possesses sufficient diagnostic features that fall into the preservational range of Granomarginata reported in younger, thermally altered sedimentary successions (e.g. Moczydłowska, Reference Moczydłowska2002).
Until now, Granomarginata was considered characteristic of the lower Cambrian Lontovan regional stage of the EEP (Volkova et al. Reference Volkova, Kirjanov, Piskun, Paskeviciene, Jankauskas, Urbanek and Rozanov1983; Jankauskas & Lendzion, Reference Jankauskas and Lendzion1992). Yet similarly to recent observations that the fossil record of some traditionally Cambrian groups such as SCF extends back into the Ediacaran (Slater et al. Reference Slater, Harvey, Bekker and Butterfield2020, see also Chiglino et al. Reference Chiglino, Gaucher, Sial and Ferreira2015), Granomarginata first occurs in older strata globally, albeit in lower abundance. In the lower Cambrian Chapel Island Formation on Newfoundland, Granomarginata precedes the first appearance of small process-bearing acritarchs characteristic of the early Cambrian Period, which was the rationale used for the establishment of the Granomarginata Zone (Palacios et al. Reference Palacios, Jensen, Barr, White and Myrow2018). Considering our findings and previously published data on the Ediacaran Granomarginata (Gunia, Reference Gunia1990; Prasad et al. Reference Prasad, Asher and Borgohai2010; Jachowicz-Zdanowska, Reference Jachowicz-Zdanowska2011), the Granomarginata Zone could represent an extension of late Ediacaran OWM assemblages into the Cambrian. Granomarginata’s appearance on the Digermulen Peninsula is just above the beds containing the macroscopic fossil Palaeopascichnus attributed to the middle–upper Ediacaran (Fig. 2a; Jensen et al. Reference Jensen, Högström, Høyberget, Meinhold, McIlroy, Ebbestad, Taylor, Agić and Palacios2018 b) and below and through the horizons bearing discoidal and dickinsoniamorph Ediacara-type macrofossils in Norway (cf. Högström et al. Reference Högström, Jensen, Palacios and Ebbestad2013, Reference Högström, Jensen, Ebbestad, Taylor, Høyberget, Agić, Meinhold and Palacios2017). On Newfoundland, Granomarginata occurs in upper Ediacaran strata (Fig. 2c), in units well above formations bearing Avalon assemblage macrofossils (cf. O’Brien & King, Reference O’Brien and King2004; Matthews et al. Reference Matthews, Liu, Yang, McIlroy, Levell and Condon2021). In Namibia, Granomarginata occurs in the unit below strata bearing Cloudina (cf. Germs, Reference Germs1972a, Reference Germs1983), and in strata overlain by a member containing the late Ediacaran Nama assemblage (Fig. 2; cf. Narbonne et al. Reference Narbonne, Saylor and Grotzinger1997; Vickers-Rich et al. Reference Vickers-Rich, Ivantsov, Trusler, Narbonne, Hall, Wilson, Greentree, Fedonkin, Elliott, Hoffmann and Schneider2013; Elliot et al. Reference Elliott, Trusler, Narbonne, Vickers-Rich, Morton, Hall and Schneider2016; Maloney et al. Reference Maloney, Boag, Facciol, Gibson, Cribb, Koester, Kenchington, Racicot, Darroch and Laflamme2020). Rocks in these localities are not coeval, and we do not correlate them, but all three sequences represent the post-Shuram late Ediacaran time interval (cf. Xiao et al. Reference Xiao, Narbonne, Zhou, Laflamme, Grazhdankin, Moczydłowska-Vidal and Cui2016) and offer insights about the age range of flask-shaped microfossils and Granomarginata.
Two Granomarginata morphotypes are recognized in the present material. The type with a wider equatorial extension is consistent with the diagnosis of G. squamacea (Fig. 4a–d). The morphotype with a narrower extension (Fig. 4e, f) is consistent with G. prima. This taxon is also known from the upper Ediacaran strata of Poland (Jachowicz-Zdanowska, Reference Jachowicz-Zdanowska2011), but it is the more pervasive morphotype in younger strata, known from the Terreneuvian deposits of the Digermulen Peninsula (Högström et al. Reference Högström, Jensen, Palacios and Ebbestad2013; Palacios et al. Reference Palacios, Högström, Ebbestad, Agić, Høyberget, Jensen, Meinhold and Talyor2020), New Brunswick (Palacios et al. Reference Palacios, Jensen, Barr, White and Miller2011) and Newfoundland (Palacios et al. Reference Palacios, Jensen, Barr, White and Myrow2018) in Canada, from the lower–middle Cambrian of China (Yin et al. Reference Yin, Yang, Peng and Kong2009), as well as from elsewhere on Baltica (Volkova et al. Reference Volkova, Kirjanov, Piskun, Paskeviciene, Jankauskas, Urbanek and Rozanov1983; Jankauskas & Lendzion, Reference Jankauskas and Lendzion1992), the EEP (Jachowicz-Zdanowska, Reference Jachowicz-Zdanowska2013; Szczepanik & Żylińska, Reference Szczepanik and Żylińska2016) and the eastern Cordillera (Rubinstein et al. Reference Rubinstein, Mángano and Buatois2003). In the stratigraphic correlation chart presented by Kumar & Maithy (Reference Kumar and Maithy2008), the Stáhpogieddi Formation in Norway was aligned with the Ediacaran to lowermost Cambrian Kauriyala Formation of the Lesser Himalayas in India, which also contains G. prima. It was potentially reported from the Kuibis Subgroup in Namibia by Germs et al. (Reference Germs, Knoll and Vidal1986, fig. 6i) as a ‘Comasphaeridium-like fossil’.
Granomarginata is a rare component (c. ≤ 5 specimens per slide) of the leiosphaerid-dominant palynomorph assemblage (c. 50–70 leiosphaerids per sample) in the upper Ediacaran successions studied here, where it co-occurs with rare small carbonaceous problematica. Both Granomarginata morphotypes are more common and abundant in the Fortunian Stage, and define the Granomarginata Zone. Their higher abundance (> 10 specimens per slide), along with Cambrian-characteristic taxa, can be viewed as more indicative of lower Cambrian strata (e.g. Palacios et al. Reference Palacios, Jensen, Barr, White and Myrow2018). On the other hand, a low abundance of Granomarginata within a leiosphaerid-dominant assemblage, in association with flask-shaped L. collaris, is so far found in upper Ediacaran or transitional strata.
5.b. The life and times of the Granomarginata–Lagoenaforma association
The new flask-shaped microfossil Lagoenaforma (Fig. 5) was observed in the horizons containing Ediacaran Granomarginata. Flask-shaped microfossils resembling chitinozoans are common in Ordovician–Devonian strata and some non-chitinozoan flask-shaped problematica are present in Ordovician strata (e.g. Loeblich & McAdam, Reference Loeblich and MacAdam1971), but have not been previously described from the Ediacaran. L. collaris is a minor component of leiosphaerid-dominated assemblages, but in addition to Granomarginata it is one of the few OWM taxa outside of dominant simple sphaeromorphs in the upper Ediacaran (LELP assemblage). As L. collaris is currently unknown from Cambrian units, it serves as a better representative of the late Ediacaran microbiota of the two. A low-diversity OWM assemblage with G. prima occurs along with ‘flask-shaped bodies’ in the lower Cambrian Withycombe Formation, UK (Rushton & Molyneux, Reference Rushton and Molyneux1990). No microfossils were illustrated in that publication, so affinities with Lagoenaforma could not be determined. However, the presence of other soft-bodied flask-shaped microfossils higher up in the stratigraphy on the Digermulen Peninsula (A. Högström, pers. obs.) and in other upper Ediacaran – Cambrian units worldwide (Table 1; cf. Sabouri et al. Reference Sabouri, Frahani and Narimani2003), as well as agglutinated forms (Gaucher & Sprechmann, Reference Gaucher and Sprechmann1999), suggests that flask-shaped OWM may be a common component of the microbiota during this transitional interval.
We found these OWM in upper Ediacaran units with a depauperate palynomorph assemblage, but their full stratigraphic range still needs to be determined. We used information on the age of the rocks hosting Granomarginata–Lagoenaforma to assess their age range. Granomarginata and Lagoenaforma do not co-occur with the classic acanthomorphic acritarch assemblage (ECAP/DPA) in the underlying lower Ediacaran strata in Norway (H. Agić, pers. obs.), nor have they been recognized so far in the older deposits on Newfoundland (see Hofmann et al. Reference Hofmann, Hill and King1979) or elsewhere (e.g. Grey, Reference Grey2005), so we view them as constituents of the late Ediacaran OWM assemblage (LELP sensu Grey, Reference Grey2005). On Newfoundland, a flask-shaped microfossil was found in an assemblage hundreds of metres above the youngest occurrence of rangeomorphs in the St John’s Group that have a maximum age of 564.13 ± 0.65 Ma (U–Pb zircon data; Matthews et al. Reference Matthews, Liu, Yang, McIlroy, Levell and Condon2021). In Namibia Granomarginata and Lagoenaforma are found in the Mara Member, below all local occurrences of Ediacaran body fossils, and which records a negative δ13C excursion that has been interpreted as correlative with the Shuram–Wonoka CIE (cf. Grotzinger et al. Reference Grotzinger, Bowring, Saylor and Kaufman1995; Saylor et al. Reference Saylor, Grotzinger and Germs1995; Narbonne et al. Reference Narbonne, Saylor and Grotzinger1997). In Arctic Norway, these fossils appear immediately above the first occurrence of palaeopascichnids and below the first Ediacara-type fossils including discs and dickinsoniamorphs (Högström et al. Reference Högström, Jensen, Palacios and Ebbestad2013, Reference Högström, Jensen, Ebbestad, Taylor, Høyberget, Agić, Meinhold and Palacios2017; Jensen et al. Reference Jensen, Högström, Høyberget, Meinhold, McIlroy, Ebbestad, Taylor, Agić and Palacios2018 b), suggesting an age of 565–550 Ma based on radioisotopic ages that constrain similar fossils assemblages (cf. Jensen et al. Reference Jensen, Högström, Høyberget, Meinhold, McIlroy, Ebbestad, Taylor, Agić and Palacios2018 b; Kolesnikov et al. Reference Kolesnikov, Liu, Danelian and Grazhdankin2018; Soldatenko et al. Reference Soldatenko, El Albani, Ruzina, Fontaine, Nesterovsky, Paquette, Meunier and Ovtcharova2019). These examined units are not coeval, but their ages are mostly younger than the Shuram anomaly, the most negative carbon isotope excursion in Earth’s history and a global, synchronous event with bounding Re–Os ages of 574.0 ± 4.7 to 567.3 ± 3.0 Ma (Rooney et al. Reference Rooney, Cantine, Bergmann, Gómez-Pérez, Al Baloushi, Boag, Busch, Sperling and Strauss2020). Based on these occurrences, and depending on the age of palaeopascichnids in the Indreelva Member (see Jensen et al. Reference Jensen, Högström, Høyberget, Meinhold, McIlroy, Ebbestad, Taylor, Agić and Palacios2018 b), the Granomarginata–Lagoenaforma association first appeared after, or coincides with, the latest part of the Shuram excursion. It has a relatively long range to the uppermost Ediacaran, and Granomarginata becomes more common in the Cambrian strata.
The age uncertainty of the Norwegian strata presents two possibilities for the overall age range of the Granomarginata–Lagoenaforma association (Fig. 7b). The Mortensnes Formation diamictite (separated from the Indreelva Member by the Lillevannet Member, Fig. 2a) is thought to be of Ediacaran age (Halverson et al. Reference Halverson, Hoffman, Schrag, Maloof and Rice2005). A dolomite bed 20 m below the Mortensnes diamictite on the neighbouring Varanger Peninsula contains depleted δ13C values (< −8‰) considered ‘a likely correlative of the Shuram–Wonoka anomaly’ (Rice et al. Reference Rice, Edwards, Hansen, Arnaud and Halverson2011, p. 598), and it is thought to correlate with the Shuram CIE in the upper Johnnie Formation in Death Valley, USA (Halverson et al. Reference Halverson, Hoffman, Schrag, Maloof and Rice2005). This suggests a younger age of the Mortensnes diamictite than the Marinoan glaciation and it was correlated with the short-lived Ediacaran Gaskiers glaciation expressed in diamictites on Newfoundland. At the time that model was proposed, the Gaskiers was assumed to be near in age to the Wonoka (=Shuram) anomaly (cf. Halverson et al. Reference Halverson, Hoffman, Schrag, Maloof and Rice2005). Subsequently, the age of the Gaskiers glaciation was constrained to c. 579 Ma (Pu et al. Reference Pu, Bowring, Ramezani, Myrow, Raub, Landing, Mills, Hodgin and Macdonald2016), which is older than the recent age constraints on the Shuram–Wonoka excursion based on Re–Os geochronology of strata in Oman and NW Canada (c. 574–567 Ma, Rooney et al. Reference Rooney, Cantine, Bergmann, Gómez-Pérez, Al Baloushi, Boag, Busch, Sperling and Strauss2020) and estimates from astrochronology (c. 570–562 Ma, Gong & Li, Reference Gong and Li2020). This places the Shuram–Wonoka excursion after the Gaskiers glaciation. Accepting the interpretation that the negative δ13C values in the Nyborg Formation carbonates are representative of the Shuram–Wonoka anomaly, the Mortensnes diamictite could be younger than the Gaskiers and Trinity diamictites on Newfoundland, which suggests an even younger age for the base of the overlying Indreelva Member. This places it closer in age to the studied strata in Newfoundland and Namibia, and implies a narrower stratigraphic range of OWM at the end of the Ediacaran Period.
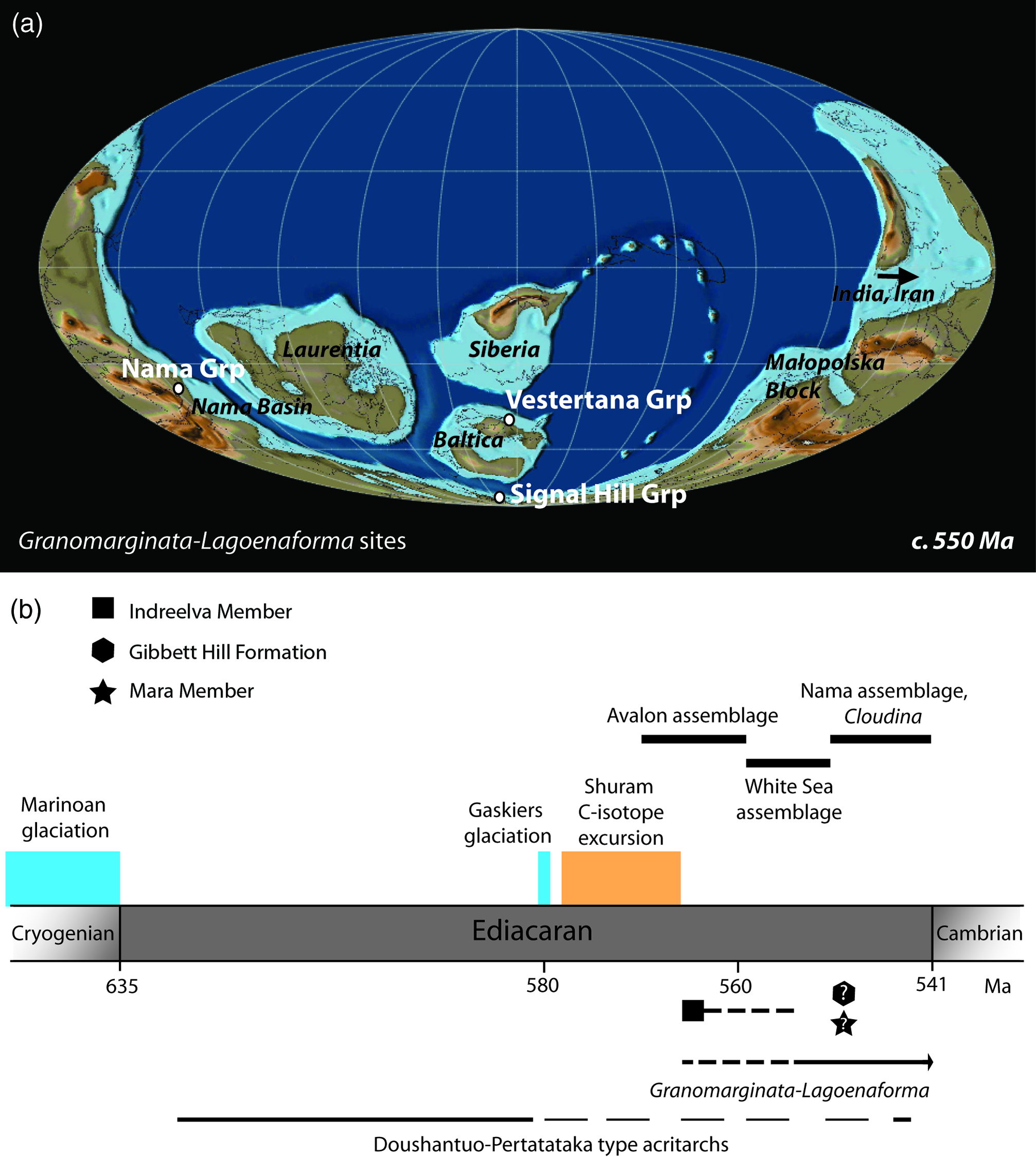
Fig. 7. Distribution and occurrences of documented Granomarginata–Lagoenaforma in the Ediacaran Period. (a) Microfossils from the studied units occur in three distinct areas: Baltica (Indreelva Member, Vestertana Group), Avalonia (Gibbett Hill Formation, Signal Hill Group) and Nama Basin (Mara Member, Nama Group), in white. Localities with additional occurrences of Ediacaran Granomarginata or flask-shaped organic-walled microfossils are marked in black. Continental reconstruction around 550 Ma was produced using PaleoAtlas for GPlates program (Scotese, Reference Scotese2016). (b) Schematic timeline of the appearance and the stratigraphic range of Granomarginata–Lagoenaforma relative to macrofossil assemblages and major environmental events in the Ediacaran Period. The orange box represents the permissible time of the duration of the Shuram negative carbon isotope excursion, following Rooney et al. (Reference Rooney, Cantine, Bergmann, Gómez-Pérez, Al Baloushi, Boag, Busch, Sperling and Strauss2020). Fossiliferous localities are marked by black symbols. A dashed line represents the interval of age-uncertainty for each locality (see Discussion for details).
Alternatively, if the Mortensnes Formation is of Gaskiers age (Halverson et al. Reference Halverson, Hoffman, Schrag, Maloof and Rice2005) and therefore older than the Shuram excursion, the Granomarginata–Lagoenaforma association has approximately the same age constraint as Palaeopascichnus (Fig. 7b). This is supported by the presence of palaeopascichnids of a type around 565 Ma or younger in age in the Indreelva Member (Jensen et al. Reference Jensen, Högström, Høyberget, Meinhold, McIlroy, Ebbestad, Taylor, Agić and Palacios2018 b), just below the occurrence of OWM, which coincides with the later part of the permissible duration of the Shuram excursion. Moreover, the OWM and the palaeopascichnids occur prior to the appearance of dickinsoniamorphs (Högström et al. Reference Högström, Jensen, Ebbestad, Taylor, Høyberget, Agić, Meinhold and Palacios2017), whose stratigraphic range is around 558–550 Ma (Narbonne et al. Reference Narbonne, Xiao, Shields, Gehling, Gradstein, Ogg, Schmitz and Ogg2012). The older age of the Mortensnes diamictite, probably coeval with the Gaskiers and Trinity diamictites, is therefore more likely, and indicates a longer age range of Granomarginata–Lagoenaforma through late Ediacaran time.
A negative carbon isotope excursion in the Nama Group was correlated to excursions recorded in the upper Nafun (c. 550 Ma) and lower Ara groups in Oman, above the Shuram Formation (Grotzinger et al. Reference Grotzinger, Bowring, Saylor and Kaufman1995; Halverson et al. Reference Halverson, Hoffman, Schrag, Maloof and Rice2005). However, these depleted δ13C values in the carbonates of the Dabis Formation, including the Mara Member, have also been interpreted as heralding the end of the Shuram excursion (Hall et al. Reference Hall, Kaufman, Vickers-Rich, Ivantsov, Trusler, Linnemann, Hofmann, Elliott, Cui, Fedonkin and Hoffmann2013; Wood et al. Reference Wood, Poulton, Prave, Hoffmann, Clarkson, Guilbaud, Lyne, Tostevin, Bowyer, Penny and Curtis2015). The excursion recorded in the Mara Member elsewhere (Kaufman et al. Reference Kaufman, Hayes, Knoll and Germs1991; Wood et al. Reference Wood, Poulton, Prave, Hoffmann, Clarkson, Guilbaud, Lyne, Tostevin, Bowyer, Penny and Curtis2015) is not fully expressed in the sampled section, possibly because the Pockenbank area was on a palaeo-high compared with that of other localities that record the Shuram anomaly in full (see Vickers-Rich et al. Reference Vickers-Rich, Narbonne, Laflamme, Darroch, Kaufman and Kriesfeld2016). Additional micropalaeontological investigation is needed to evaluate the extent of the association’s range through the lower Nama Group and in relation to its chemostratigraphy.
Throughout the late Ediacaran interval, both Granomarginata and Lagoenaforma are rare in comparison to leiosphaerid and filamentous microfossils, but still more morphologically distinct than most other OWM in the upper Ediacaran strata. The association also co-occurs with the coccoid aggregate structure Bavlinella sp. (Fig. 6h), which is relatively common in Neoproterozoic strata (cf. Vidal, Reference Vidal1976) and was previously recovered from the Ediacaran strata of Namibia by Germs et al. (Reference Germs, Knoll and Vidal1986). Smooth-walled OWM (leiosphaerids; Fig. 6a, b, d) are the most abundant component of the examined assemblages, consistent with the idea that the late Ediacaran microbiota was generally of low diversity and dominated by simple leiosphaerids (Moczydłowska, Reference Moczydłowska1991; Grey, Reference Grey2005). Lophosphaeridium is another OWM best known from Cambrian strata that was also reported from the upper Ediacaran Frecheirinha Formation (Chiglino et al. Reference Chiglino, Gaucher, Sial and Ferreira2015) and Maricá and Bom Jardin groups in Brazil (Lehn et al. Reference Lehn, Horodyski and Paim2019). This taxon was not found in the present material, so it may not have been distributed globally or it may have been restricted to a specific environment, and more work is needed to assess its distribution at this time. Regardless, both Lophosphaeridium and Granomarginata first appeared during the rise of macroscopic Ediacara-type biota, and have subsequently diversified and became more prominent components of OWM assemblages in the Cambrian strata (e.g. Moczydłowska, Reference Moczydłowska1991; Palacios et al. Reference Palacios, Jensen, Barr, White and Myrow2018).
Because of the presence of taxa and fossil groups that ‘cross’ the Ediacaran–Cambrian boundary, the composition of whole assemblages may also be relevant for the OWM biostratigraphy of the Ediacaran–Cambrian transition. A leiosphaerid-dominant assemblage with Lagoenaforma in association with Granomarginata is present in multiple palaeogeographic areas and, pending further studies, has potential to be broadly used as one of the indicators of the upper series Ediacaran. However, this association may also reflect a specific environmental control.
5.c. Palaeoenvironmental implications
Our new data show that Granomarginata and Lagoenaforma are relatively rare components of the late Ediacaran microbiota, but were present on several palaeocontinents (Fig. 7a; Table 1). The studied strata in Norway, Newfoundland and Namibia are not coeval, but can be viewed as part of the upper series Ediacaran (cf. Xiao et al. Reference Xiao, Narbonne, Zhou, Laflamme, Grazhdankin, Moczydłowska-Vidal and Cui2016). Considering the palaeogeographic reconstruction for this time, the Granomarginata–Lagoenaforma association was widely dispersed (Fig. 7a), and possibly an assemblage of cosmopolitan taxa. The association could be representative of taxa occupying a specific type of environment. All strata in which this assemblage occurs (including the upper Ediacaran rocks bearing Granomarginata in India, Poland and Baltica; Prasad et al. Reference Prasad, Asher and Borgohai2010; Jachowicz-Zdanowska, Reference Jachowicz-Zdanowska2011; Arvestål & Willman, Reference Arvestål and Willman2020), represent a marine shallow-water to marginal shelf environment. Leiosphaerids are usually highly abundant in nearshore, shallow-water environments (e.g. Li et al. Reference Li, Servais, Yan and Zhu2004). They comprise most of the OWM assemblage containing Granomarginata and Lagoenaforma, so this is further indication of a shallow-water setting.
Acanthomorphic OWM characteristic of ECAP/DPA do not occur in the studied strata, although rare cases of late Ediacaran acanthomorphs are documented in Mongolia (Anderson et al. Reference Anderson, Macdonald, Jones, McMahon and Briggs2017, Reference Anderson, McMahon, Macdonald, Jones and Briggs2019) and Siberia (Grazhdankin et al. Reference Grazhdankin, Nagovitsin, Golubkova, Karlova, Kochnev, Rogov and Marusin2020). These assemblages are an exception among the depauperate OWM assemblages prevalent at this time (Grey, Reference Grey2005; Leonov & Ragozina, Reference Leonov, Ragozina, Vickers-Rich and Komarower2007). While the pre-Shuram ECAP assemblages are generally diverse (e.g. 54 taxa in the Doushantuo Formation; Xiao et al. Reference Xiao, Zhou, Liu, Wang and Yuan2014), the LELP assemblages tend to contain fewer taxa (e.g. 16 in the Lyamtsa Formation, White Sea region; Leonov & Ragozina, Reference Leonov, Ragozina, Vickers-Rich and Komarower2007), many of which are bacterial. The exceptionally preserved microfossils from the Khesen Formation in Mongolia exhibit Doushantuo-style preservation (Anderson et al. Reference Anderson, Macdonald, Jones, McMahon and Briggs2017), similar to ECAP microbiota from phosphorites of the lower Ediacaran Doushantuo Formation in China (e.g. Xiao et al. Reference Xiao, Zhou, Liu, Wang and Yuan2014). The Khesen assemblage is preserved in phosphatic grainstones, within a sediment-starved, condensed carbonate succession. The acid-extracted acanthomorphs from the Oppokun Formation in Siberia occur in a thick succession of mudstones (Grazhdankin et al. Reference Grazhdankin, Nagovitsin, Golubkova, Karlova, Kochnev, Rogov and Marusin2020), probably deposited in a low-energy environment. These occurrences in different depositional environments may result in different assemblages: nearshore shallow marine in the case of the Granomarginata–Lagoenaforma association and depauperate leiosphaerid assemblage (LELP) recognized by Grey (Reference Grey2005), or low-energy environments in the case of ECAP. The overall dearth of microfossils across the Ediacaran–Cambrian transition could also be a result of the lack of sampling for palynological analyses, or a depositional hiatus. Many relevant successions consist of mainly unsuitable lithologies for OWM preservation (especially in Australia; Grey, Reference Grey2005), although this is not the case on EEP and Baltica. The dearth of OWM could also relate to the Kotlinian Crisis: a drop in diversity of soft-bodied taxa near the increase of bioturbation and expansions of reef-builders (Kolesnikov et al. Reference Kolesnikov, Marusin, Nagovitsin, Maslov and Grazhdankin2015).
Some prasinophyte-like OWM (in addition to Granomarginata, e.g. Tasmanites and Pterosperma-like morphotypes) persisted through the Ediacaran–Cambrian transition, and it was suggested that perhaps these organisms played a role as primary producers in sustaining the metazoan ecosystem during this transitional interval (Moczydłowska, Reference Moczydłowska2011). This is difficult to reconcile with biomarker evidence for bacterially dominated primary production on Baltica and the EEP in the upper Ediacaran strata (Pehr et al. Reference Pehr, Love, Kuznetsov, Podkovyrov, Junium, Shumlyanskyy, Sokur and Bekker2018). Prasinophytes are generally successful in low diversities of other phytoplankton taxa and in an aftermath of crises, which labels them disaster-taxa (e.g. van de Schootbrugge et al. Reference van de Schootbrugge, Tremolada, Rosenthal, Bailey, Feist-Burkhardt, Brinkhuis, Pross, Kent and Falkowski2007). A community of such disaster-taxa and potential mixotrophs (flask-shaped microfossils) is therefore a likely response of eukaryotic microbiota to ecosystem perturbations (cf. Kolesnikov et al. Reference Kolesnikov, Marusin, Nagovitsin, Maslov and Grazhdankin2015; Wood et al. Reference Wood, Liu, Bowyer, Wilby, Dunn, Kenchington, Cuthill, Mitchell and Penny2019) through the late Ediacaran Period.
6. Conclusions
The organic-walled microfossil Granomarginata, otherwise a constituent of lower Cambrian acritarch assemblages, was recovered from middle–upper Ediacaran strata, in addition to a new taxon Lagoenaforma collaris gen. et sp. nov. New locations include three palaeocontinents: Avalonia (Newfoundland), Baltica (Norway) and adjacent to the Kalahari Craton (Namibia). While Granomarginata survived into and became more prominent in the Cambrian, Lagoenaforma is so far known only from the Ediacaran, although other neck-bearing forms occur in the lowermost Cambrian strata in Norway. Problematic microfossils in other Ediacaran units resembling Granomarginata are reviewed in this study; the taxon’s occurrence was additionally documented elsewhere on Baltica, the Indian palaeocontinent and the Małopolska Block of Poland (Table 1). The stratigraphic range of Granomarginata is therefore extended further back in time, which places it into the so-called late Ediacaran leiosphaerid palynoflora (LELP; sensu Grey, Reference Grey2005), a depauperate assemblage of predominantly sphaeromorph and envelope-bearing organic-walled microfossils. Our records from three palaeocontinents and previous reports show that both taxa were geographically widespread by late Ediacaran time. Their occurrence deeper in the stratigraphy suggests a protracted transition into Cambrian-like assemblages.
Co-occurring taxa include prokaryotic OWM and organic problematica (Table 1). Granomarginata and flask-shaped microfossils occur in strata that mostly postdate the Shuram–Wonoka excursion and so far have not been recovered from lower Ediacaran assemblages (cf. Hofmann et al. Reference Hofmann, Hill and King1979; Grey, Reference Grey2005; Agić et al. Reference Agić, Högström, Moczydłowska, Jensen, Palacios, Meinhold, Ebbestad, Taylor and Høyberget2019). Based on these occurrences in post-Gaskiers and post-Shuram rocks, the age range of the Granomarginata–Lagoenaforma association is broadly late Ediacaran.
Together, the record presented here along with other reports on late Ediacaran microfossils support the conclusion that some OWM and SCF survived into the Phanerozoic Eon with their abundance increasing during the Cambrian Period. The LELP assemblage is indeed dominated by leiosphaerids, but contains more taxa than previously recorded, even outside the few Doushantuo–Pertatataka acritarch assemblages that persisted into the upper Ediacaran. Although this record is rather limited, it calls for a more thorough palynological investigation of middle–upper Ediacaran strata. Globally, few units have been examined in detail (with the exception of Russia and Ukraine), so perhaps the diversity of late Ediacaran protistan microfossils is underestimated. Additional OWM records in such little-studied deposits could not only aid in biostratigraphic endeavours, but also help constrain the timing of the turnover of late Neoproterozoic microscopic eukaryotes, and the onset of the microbiota characteristic of the early Phanerozoic Eon.
7. Systematic palaeontology
Genus Granomarginata Naumova (Reference Naumova1960)
Type species
Granomarginata prima Naumova (Reference Naumova1960, p. 114) described from the lower Cambrian ‘Blue Clay’ (=Lontova Formation) of Estonia.
Remarks
Specimens of Granomarginata in the present material resemble Ostiumsphaeridium complitum (Vorob’eva et al. Reference Vorob’eva, Sergeev and Knoll2009, figs. 14.1, 14.2) from the upper Neoproterozoic strata on the East European Platform, found in association with large spinose OWM (DPA/ECAP). Both taxa have a darker central body with a fluffy extension, yet the vesicle of Ostiumsphaeridium additionally possesses a large, oval slit-like excystment opening. No openings are observed in present or previously described Granomarginata.
Granomarginata can be distinguished from more common and long-ranging Proterozoic envelope-bearing OWM such as Simia annulare Timofeev (Reference Timofeev1969) emend. Mikhailova in Jankauskas et al. (Reference Jankauskas, Mikhailova and Hermann1989), in its texture and shape of its outer envelope. Both taxa have a central body surrounded by an extension in the equatorial plane that forms a rim of varying width between species. Whereas the extension in Simia is sharply outlined and crisp, with occasional concentric folding caused by compression (e.g. Vorob’eva et al. Reference Vorob’eva, Sergeev and Knoll2009, fig. 14.7; Agić et al. Reference Agić, Moczydłowska and Yin2017, fig. 14h–i), the extension of G. prima is fluffy and filmy (e.g. Moczydłowska, Reference Moczydłowska1991). Jankauskas et al. (Reference Jankauskas, Mikhailova and Hermann1989, p. 66) noted that Simia has a ‘less-rough surface’. Simia co-occurring in the same sample as Granomarginata in the Nama Group (Fig. 6f) has a well outlined and thick-walled central body as characteristic for the taxon, and its extension shows different properties: faint concentric folding from compression that is not observed in Granomarginata. Furthermore, the outline between the central body and the extension in Simia is sharp and well-defined (Jankauskas et al. Reference Jankauskas, Mikhailova and Hermann1989), whereas Moczydłowska (Reference Moczydłowska1991, p. 57) observed that the spongy nature of the Granomarginata wall may ‘appear as irregular filaments on the outline of the equatorial zone’. The extension in Granomarginata often appears fragmented or uneven (e.g. Volkova, Reference Volkova1968), and is likely less resistant than the central body (Moczydłowska, Reference Moczydłowska2011). Material presented here supports this. Many documented Simia specimens also have a fragmentary extension (e.g. Agić et al. Reference Agić, Moczydłowska and Yin2017, fig. 10g, h), but the breakage there is sharper than seen in Granomarginata, indicating a more brittle nature of the envelope in Simia. Granomarginata also differs from envelope-bearing Pterospermopsimorpha. A disphaeromorph, Pterospermopsimorpha is a sphere-within-sphere with a well outlined inner vesicle and a robust outer envelope (e.g. Jankauskas et al. Reference Jankauskas, Mikhailova and Hermann1989, pl. 3, figs 3–8; Agić et al. Reference Agić, Moczydłowska and Yin2017, fig. 10a–c). The inner vesicle is not always centrally located within the envelope, as is the case in Simia or Granomarginata. Pterospermopsimorpha is quite rare in Ediacaran strata relative to other OWM taxa (Grey, Reference Grey2005), as well as compared with its abundance in pre-Cryogenian fossiliferous units (H. Agić, pers. obs.).
Granomarginata prima Naumova (Reference Naumova1960)
Figure 4e, f
Synonymy
cf. 1986 ‘Comasphaeridium-like fossil’; Germs et al., p. 56, fig. 5i.
1990 Granomarginata sp.; Gunia, p. 109, pl. 1, figs 10, 11.
2010 Granomarginata prima; Prasad et al., p. 420, pl. 1, fig. 6.
2011 Granomarginata prima; Jachowicz-Zdanowska, p. 91, fig. 4I.
See additional synonymy of Cambrian specimens by Palacios et al. (Reference Palacios, Jensen, Barr, White and Myrow2018).
Material
Two specimens (D16-HA-80 84.5×18 and D16-HA-53 81×14) from the Indreelva Member, Stáhpogieddi Formation, Vestertana Group in Norway. One complete specimen and one hemisphere fragment (N16-HA-P3 79×16) from the Mara Member, Dabis Formation, Nama Group, Pockenbank in Namibia. A fragment of a vesicle with a spongy envelope from the Gibbett Hill Formation, Signal Hill Group on Avalon Peninsula in Newfoundland (Brasier Shale A-1 87×5).
Description
Round to oval, discoid vesicles with central body that is surrounded by a narrow, membrane-like, spongy extension. The extension rim on our specimens is uniform in width, but it seems less resistant than the central body, so it is occasionally fragmentary or appears etched and uneven.
Dimensions
Vesicle diameter range observed in G. prima is 24–40 µm (cf. Moczydłowska, Reference Moczydłowska1991). In the present material, the overall diameter of the microfossil ranges over 31–39 µm, n = 4, mean (x̅) = 35.5 µm, standard deviation (σ) = 4.8 µm.
Remarks
G. prima differs from G. squamacea in a narrower rim. There is no prior known occurrence of Granomarginata from Namibia. However, Germs et al. (Reference Germs, Knoll and Vidal1986) have documented an acritarch specimen strongly resembling G. prima, identified as ‘Comasphaerdium-like fossil’ (Germs et al. Reference Germs, Knoll and Vidal1986, fig. 6i) from the upper Kuibis subgroup. Although the specimen is poorly preserved, and its features are difficult to discern in the black-and-white photomicrograph, it appears to bear a narrow spongy rim characteristic of G. prima. Poorly preserved specimens of Granomarginata may superficially resemble acanthomorphic acritarchs with thin processes (cf. Spina et al. Reference Spina, Cirilli, Ghorban, Rettori, Sorci and Servais2020, pl. 1 figs 6–8). Specimens of G. prima from upper Ediacaran strata represent this species’ oldest known occurrence to date, but they are very rare in Ediacaran units, in comparison to the taxon’s ubiquity in the Cambrian strata. It is a more common component of the Granomarginata Zone and Asteridium tornatum–Comasphaeridium velvetum Zone in the Terreneuvian Series (Fortunian) of lower Cambrian strata, as well as in the Volkovia dentifera–Liepaina plana and Skiagia ornata–Fimbriaglomerella membranacea zones in Cambrian Series 2 (Palacios et al. Reference Palacios, Jensen, Barr, White and Myrow2018, Reference Palacios, Högström, Ebbestad, Agić, Høyberget, Jensen, Meinhold and Talyor2020). Examples of Granomarginata with a darker central body are common in the thermally altered rocks, for example in the Såvvovare/Grammajukku formations in Sweden (Moczydłowska, Reference Moczydłowska2002) and higher up in the stratigraphy on the Digermulen Peninsula (Manndrapselva Member; Högström et al. Reference Högström, Jensen, Palacios and Ebbestad2013, fig. 6C), as well as in some strata with lower thermal alteration such as the File Haidar Formation in Sweden (Eklund, Reference Eklund1990).
Occurrence
In Ediacaran-age deposits, Granomarginata prima occurs in the following units: Indreelva Member, Stáhpogieddi Formation, Vestertana Group, Norway; Dabis Formation, Nama Group, Namibia; Jodhpur Formation, Marwar Supergroup, India (Prasad et al. Reference Prasad, Asher and Borgohai2010); boreholes from the Małopolska block adjacent to East European Platform, Poland (Jachowicz-Zdanowska, Reference Jachowicz-Zdanowska2011); and potentially Gibbett Hill Formation, Signal Hill Group, Newfoundland. For a list of Cambrian occurrences of G. prima, see work by Moczydłowska (Reference Moczydłowska1991, Reference Moczydłowska2011) and Palacios et al. (Reference Palacios, Jensen, Barr, White and Myrow2018). G. prima and organic problematica are found also in the Cambrian strata on the Digermulen Peninsula, in the third cycle of the Manndrapselva Member, Stáhpogieddi Formation (Fig. 2a; Högström et al. Reference Högström, Jensen, Palacios and Ebbestad2013), and in the Duolbagáisá Formation, Digermulen Group correlated to Cambrian Stage 3–4 (Palacios et al. Reference Palacios, Högström, Ebbestad, Agić, Høyberget, Jensen, Meinhold and Talyor2020), which overlies the Vestertana Group.
Stratigraphic range
The first appearance of G. prima is in the upper Ediacaran strata above the first appearance of Palaeopascichnus and discoidal macrofossils in Norway, and below the strata containing upper Ediacaran macrofossils in Namibia. The taxon ranges to the Miaolingian Series (Moczydłowska, Reference Moczydłowska1991).
Granomarginata squamacea Volkova (Reference Volkova1968)
Figure 4a–d
Synonymy
cf. 2020 ‘unknown c’; Arvestål & Willman, fig. 12d.
See additional synonymy of Cambrian occurrences by Moczydłowska (Reference Moczydłowska2011).
Material
Three specimens and three fragments (D18-HA-20 81×6, D16-HA-80 87×19, D16-HA-80 85×21) from near the base of the Indreelva Member, Stáhpogieddi Formation (Vestertana Group), exposed on the Digermulen Peninsula, Norway. Two specimens and three fragments (N16-HA-P2 82×13, N16-HA-P3 81×9) from the Mara Member, Dabis Formation, Nama Group, from Farm Pockenbank, southern Namibia.
Description
Circular to oval vesicles consisting of a central body surrounded by a wide, spongy, membranous extension in the equatorial part. The extension is thin and of varying breadth; it often appears uneven in outline or etched.
Dimensions
Vesicle diameter of G. squamacea in the material reported here ranges over 32–58 µm, n = 9, x̅ = 45.7 µm, σ = 8.2 µm, marginally larger than the reported size range for this taxon of 20–55 µm by Moczydłowska (Reference Moczydłowska2011). Central body diameter is 25–32 µm.
Remarks
Unlike G. prima, the extension in G. squamacea is often creased. Moczydłowska (Reference Moczydłowska1991, p. 57) noted that the spongy part of G. squamacea “appears as irregular ‘filaments’ on the outline of the equatorial zone”. In a few poorly preserved specimens this may lead to their erroneous identification as an acanthomorph taxon with dense thin processes, for example, Comasphaeridium. This filamentous appearance of the extension was probably obtained as the vesicle underwent post-depositional compression. Specimens of G. squamacea in this material differ from other Proterozoic envelope-bearing taxa such as Simia and Pterospermopsimorpha in a membranous, spongy extension and fluffy wall (Moczydłowska, Reference Moczydłowska1991), as mentioned above. The distinction between the two taxa was also illustrated by Moczydłowska (Reference Moczydłowska2002, fig. 9.3, 9.4). G. squamacea with an opaque central body is also known from the thermally altered Cambrian strata of the Swedish Caledonides (Moczydłowska, Reference Moczydłowska2002, fig. 9.4). Such an appearance is then not unexpected within the Indreelva Member, where the sediments have experienced tectono-metamorphic deformation related to the late Scandian Orogeny, and have a postmature overprint of 200–250 °C (Meinhold et al. Reference Meinhold, Wemmer, Högström, Ebbestad, Jensen, Palacios, Høyberget, Agić and Taylor2019 b). Some Neoproterozoic specimens of G. squamacea (Pyatiletov, Reference Pyatiletov, Khomentovsky and Schenfil1988) were subsequently dismissed as globular kerogen particles (Vidal et al. Reference Vidal, Moczydłowska and Rudavskaya1995). G. squamacea is rare compared with leiosphaerids or filamentous organic-walled microfossils from the same samples.
Occurrence
The first appearance of Granomarginata squamacea to date is in the Ediacaran Stáhpogieddi Formation, Vestertana Group (Arctic Norway), above the first occurrence of Palaeopascichnus delicatus estimated at < 565 Ma (cf. Jensen et al. Reference Jensen, Högström, Høyberget, Meinhold, McIlroy, Ebbestad, Taylor, Agić and Palacios2018 b). Other Ediacaran occurrences include an additional record from Baltica – Granomarginata-like acritarch from the Kotlin Formation in Estonia (Arvestål & Willman, Reference Arvestål and Willman2020) – as well as the material documented here from the Dabis Formation, Nama Group in Namibia. For a compilation of global Cambrian occurrences, we refer to Moczydłowska (Reference Moczydłowska1991, Reference Moczydłowska2011).
Stratigraphic range
The range of G. squamacea is hereby extended lower in the stratigraphy, from above the deposits of the Ediacaran glaciation and the first appearance of palaeopascichnids in Norway, and below the unit containing macrofossils of the Nama assemblage in Namibia.
Genus Lagoenaforma gen. nov.
Type species
Lagoenaforma collaris gen. et sp. nov.
Etymology
From the Latin lagoena, - ae, f. (loaned from the Greek λάγηνoς) meaning pitcher or flask, and forma, - ae, f., meaning shape, describing the microfossil’s resemblance to a wine pitcher. Pliny the Younger (Mynors, Reference Mynors1963) refers to his hunting flask as lagena/laguncula.
Synonymy
cf. 1988 ‘chitinozoan-like microfossil, Form II’; Zang, pl. 16, figs A, B.
1989 Germinosphaera guttaformis; Jankauskas et al., pl. 47, fig. 7.
2003 ‘Chitinozoa-like microfossils’; Sabouri et al., pl. 3, fig. 10.
Etymology
From Latin collum, -i, m. meaning neck, in reference to the neck-like protrusion from the elongate vesicle.
Holotype
TSGf18449c 78×15, sample D16-HA-80 (Fig. 5a) from the Ediacaran Indreelva Member, Stáhpogieddi Formation, Vestertana Group in Norway.
Material
Ten specimens in total. Four specimens (D16-HA-80 78×15, D16-HA-80 83×4, D16-HA-53 85×9, D16-HA-53 79×18) well to moderately well preserved from the Indreelva Member, Stáhpogieddi Formation (Vestertana Group) on Digermulen Peninsula, Arctic Norway. Three specimens (N16-HA-P2 76×7, N16-HA-P2 77×5.5, N16-HA-P2 79×11) from the Mara Member, Dabis Formation, Nama Group, on Farm Pockenbank, southern Namibia.
Diagnosis
Oval to elongate vesicle with a neck-like protrusion. The neck is open distally and terminates by widening outwards; it never tapers. The neck is up to one third of the overall vesicle length. Wall texture smooth to chagrinate.
Dimensions
The length of the vesicle including the ‘neck’ is 67–94 μm (x̅ = 78 μm, σ = 10.7 μm, n = 9). The opening of the ‘neck’ ranges over 15–32 μm. The neck width is 12–30 μm, except the outlier specimen from the Gibbett Hill Formation which possesses a 4-μm-wide ‘neck’.
Remarks
L. collaris exhibits no ornamentation or sculpture, but its wall texture can be smooth (Fig. 5a) or ‘fluffy’ (Fig. 5b, c), which is likely a preservational feature. The Newfoundland specimen is opaque akin to other microfossils from the Gibbett Hill Formation (e.g. Fig. 6c). This is common in OWM from units that have undergone a higher degree of thermal alteration (Spina et al. Reference Spina, Vecoli, Riboulleau, Clayton, Cirilli, Di Michele, Marcogiuseppe, Rettori, Sassi, Servais and Riquier2018), but it can also result from accelerated degradation in oxygenated environments (Schiffbauer et al. Reference Schiffbauer, Wallace, Hunter, Kowalewski, Bodnar and Xiao2012). The Newfoundland specimen has a narrower ‘neck’ compared with other specimens of L. collaris (4 μm in width), but the widening-outwards at the tip indicates that the ‘neck’ structure is not a process, but likely an open-ended protrusion.
Jankauskas et al. (Reference Jankauskas, Mikhailova and Hermann1989) erected a new species of a long-ranging Precambrian taxon Germinosphaera that includes one flask-shaped morphotype: G. guttaformis from the upper Riphean (=Tonian–Cryogenian) of Siberia. Only one specimen in their material has an elongate, flask-shaped main vesicle and a thick single process, truncated at the tip, whereas the rest possess a thick, circular vesicle 60–80 µm in diameter (Jankauskas et al. pl. 47, figs 7, 8). G. guttaformis specimen on plate 47, figure 8 has a narrow process, more similar to the narrow ‘neck’ in the Gibbett Hill specimen described here, but its vesicle is still bigger and more rounded than that of L. collaris. Although the rest of G. guttaformis material described by Jankauskas et al. (Reference Jankauskas, Mikhailova and Hermann1989) is relatively consistent, a single elongate specimen (pl. 47, fig. 7) is unlike other members of this genus; both the original and emended diagnoses for Germinosphaera imply a circular vesicle (Mikhailova, Reference Mikhailova and Sokolov1986; Butterfield et al. Reference Butterfield, Knoll and Swett1994). That morphotype therefore does not belong to Germinosphaera, and we do not include our similar specimens into this genus. Willman & Moczydłowska (Reference Willman and Moczydłowska2011, pl. 7, fig. 7) illustrated a flask-like microfossil interpreted as a fragmented cyanobacterial sheet from the upper Dey Dey Mudstone in the Officer Basin, Australia, Tanarium-bearing beds. That morphotype differs from L. collaris in its longer and straighter ‘neck’.
Some chitinozoan-like microfossils from several sections of the upper Ediacaran Kahar Formation in northern Iran (Sabouri et al. Reference Sabouri, Frahani and Narimani2003, pl. 3, fig. 10) are comparable to L. collaris. Similar ‘chitinozoan-like microfossils’ were also reported from the Liulaobei Formation, Huainan Group in China (Zang, Reference Zang1988), now understood to be Tonian in age (see Tang et al. Reference Tang, Pang, Xiao, Yuan, Ou and Wan2013). An outlier among those microfossils, called ‘Form II’ (pl. 16, figs a, b), resembles L. collaris in shape and size. Zang (Reference Zang1988) noted that distribution of ‘chitinozoan-like’ microfossils declined from deep to shallow water, which is in contrast to present material where L. collaris occurs in shallow-water environments. The relationship of L. collaris to these Tonian OWM is unclear. Additional soft-bodied forms similar to L. collaris from the Proterozoic Vindhyan Supergroup of India (Maithy & Babu, Reference Maithy and Babu1989, pl. 1) were attributed to chitinozoans, including Melanocyrillium. However, those microfossils lack diagnostic characteristics of Melanocyrillium like a well-defined aperture and a curved neck (Bloeser, Reference Bloeser1985). Superficially similar morphology to L. collaris is seen in mineralized vase-shaped microfossils (VSM) with long necks from the Neoproterozoic Urucum Formation in Brazil (Morais et al. Reference Morais, Fairchild, Lahr, Rudnitzki, Schopf, Garcia, Kudryavtsev and Romero2017, figs 6.7–6.9), interpreted as testate amoebae. VSMs with a mineralized or organic wall coated by minerals are mostly found in upper Tonian organic-rich shales (Porter et al. Reference Porter, Meisterfeld and Knoll2003). Chai et al. (Reference Chai, Hua, Ren, Dai and Cui2020) reported an uncharacteristically young occurrence of VSM from the late Ediacaran Dengying Formation in China. In contrast to Lagoenaforma, those are permineralized in three dimensions, and differ in that they have a shorter and narrower ‘neck’.
The flask-shaped morphology mostly resembles chitinozoans, organic-walled marine microfossils common in Ordovician–Devonian strata. Few flask-shaped, neck-bearing, organic taxa are known from the Proterozoic and Cambrian strata (e.g. Yin, Reference Yin1980), and there is a rare Cambrian Stage 5 occurrence of chitinozoans (Shen et al. Reference Shen, Aldridge, Williams, Vandenbroucke and Zhang2013); however, due to the simple morphology of L. collaris, further comparisons are impractical. Lagoenaforma is also similar to Ordovician flask-like OWM Aremoricanium (Deunff, Reference Deunff1955). They differ in size and shape of the neck structure, which is wider and shorter in L. collaris, and the texture of its organic wall, which is thinner and smoother among Aremoricanium species. The difference in the vesicle wall could potentially be attributed to preservation as the Ediacaran fossils studied here are not as exquisitely preserved as the Ordovician material. Nevertheless, Aremoricanium has a well defined stratigraphic range (Loeblich & MacAdam, Reference Loeblich and MacAdam1971) and lacks a Cambrian record, so such a significant time gap makes it less likely that these fossils represent the same or a related organism. L. collaris also differs from the Cambrian acanthomorphic acritarch Volkovia dentifera (Downie, Reference Downie1982) by its overall larger size, and a shorter and wider protrusion instead of the narrow, single process of Volkovia. The distal tip of the process in Volkovia is pointed and closed (cf. Moczydłowska, Reference Moczydłowska1991), whereas the neck in L. collaris is open-ended and slightly widens outwards. The possibility that the protrusion in L. collaris is truncated and could therefore represent a Volkovia with a broken-off process (or another single-processed OWM such as Aliumella baltica Umnova & Vanderflit, Reference Umnova and Vanderflit1971) is dismissed because the L. collaris ‘neck’ terminates with a widening end (Fig. 5).
A single microfossil from the Manndrapselva Member (Fig. 5d) possesses a neck-like structure akin to L. collaris, but its main vesicle is rounded and its ‘neck’ is shorter, which is more similar to Sinianella uniplicata (Yin, Reference Yin1980). The Manndrapselva Member includes additional neck-bearing OWM but with a more rounded vesicle and much longer neck structures (Palacios et al. Reference Palacios, Ou, Högström, Jensen, Høyberget, Meinhold, Taylor, Ebbestad and Agić2017; A. Högström, pers. obs.).
L. collaris shares the morphology of a flask-shaped body plan with a variety of living non-testate protists. A neck-like opening is commonly an indicator of a mixotrophic lifestyle. Some prostomateid ciliates possess a variety of oral bulges used in feeding (e.g. Enchelyodon longikineta, Şenler & Yįldįz, Reference Şenler and Yįldįz2003, fig. 2), similar to the ‘neck’ of L. collaris. Vasicola ciliata (Taylor & Sanders, Reference Taylor, Sanders and Thorp2010; fig. 3.22.O) has a soft-bodied, chitinous lorica (protective outer covering) in the shape of a pitcher, and the chitinous composition would increase its preservation potential. However, apart from these morphological similarities, the affinity of L. collaris is unclear.
Occurrence
The taxon appears in the Ediacaran shales and siltstones of the Indreelva Member, Stáhpogieddi Formation, Vestertana Group in Norway; Mara Member, Dabis Formation, Nama Group in Namibia; and the Gibbett Hill Formation, Signal Hill Group in Newfoundland.
Material
One specimen and one fragment (‘Brasier Shale A’-1 89×7, 91×11,) from the Gibbett Hill Formation, Signal Hill Group on Avalon Peninsula, Newfoundland.
Description
Oval to elongate vesicles with a neck-like protrusion. The base of the neck is slightly wider. The necks are open distally and terminate by widening outwards; they never taper. The necks are up to one third of the overall vesicle length. Wall texture smooth to chagrinate.
Dimensions
The length of the vesicle including the ‘neck’ is 87 μm. The ‘neck’ is 5 μm wide and 7 µm wide at the tip.
Remarks
This flask-shaped OWM differs from Lagoenaforma collaris in a longer and narrower ‘neck’ structure. It is similar to, but half the size of, some rare, enigmatic, flask-shape structures from the Wallara 1 drill hole, lower Ediacaran Pertatataka Formation in Australia (Grey, Reference Grey2005, fig. 274c).
Unnamed Form A
Figure 5d
Material
One specimen (TSGf18451a 89×23, sample D16-HA-77) from the Manndrapselva Member, Stáhpogieddi Formation, Vestertana Group on the Digermulen peninsula, Arctic Norway.
Description
Rounded vesicle with short and wide neck-like protrusions, flaring outwards. The vesicle wall has bulbous sculpture.
Remarks
This specimen resembles Sinianella uniplicata (Yin, Reference Yin1980), but it differs from it in having a bulbous wall sculpture. It is unclear from observing a single specimen if the body is composed of smaller cells or if those are a part of the wall sculpture. A similar microfossil with an opaque wall and a wide-based, neck-like protrusion from the middle–upper Santa Bárbara Group, Camaquã Basin in Brazil was assigned to Germinosphaera sp. (Lehn et al. Reference Lehn, Horodyski and Paim2019, fig. 3j). The Brazilian specimen bears a strong similarity to a truncated Germinosphaera, but also possesses a wider process base compared with members of this genus (cf. Butterfield et al. Reference Butterfield, Knoll and Swett1994), and appears as a ‘neck’ structure terminating in an opening. Unnamed Form A differs only slightly from the Camaquã Basin specimen in a widening-outwards ‘neck’. Further micropalaeontological examination of middle–upper Ediacaran strata worldwide will show the frequency of this morphotype. OWM with short, neck-like protrusions are not uncommon in the Precambrian though; such morphologies were also reported from the Mesoproterozoic strata of northern China (Agić et al. Reference Agić, Moczydłowska and Yin2017, fig. 13d, e), and the genus Sinianella from Tonian–lower Cambrian strata (Zang, Reference Zang1988).
Similar OWM occur in the Ediacaran Kahar Formation in Iran as ‘Melanocyrillum’ (=Melanocyrillium), alongside late Ediacaran SCF Cochleatina (Sabouri et al. Reference Sabouri, Frahani and Narimani2003, pl. 3, figs 7–9). However, Melanocyrillium is a Tonian VSM taxon that differs from these chitinozoan-like microfossils with a curved neck and well defined aperture, as well as in the mode of preservation (cf. Bloeser, Reference Bloeser1985).
Unnamed Form B
Figure 6c
Material
Two specimens (‘Brasier Shale A’-1 91×11, ‘Brasier Shale A’-1 90×13) in the Gibbett Hill Formation, Signal Hill Group, Ferryland on the Avalon Peninsula, Newfoundland.
Description
Elongate and straight, organically preserved microfossil with short lateral protrusions. Both specimens are truncated, up to 160 µm in length and 32 µm in width. Individual protrusions are broken distally, and up to 7 µm wide.
Remarks
Problematic SCF are common in uppermost Ediacaran and lower Cambrian units worldwide (Moczydłowska et al. Reference Moczydłowska, Budd and Agić2015; Slater et al. Reference Slater, Harvey, Bekker and Butterfield2020). Previously, the youngest occurrence of SCF in Newfoundland was documented from the Random Formation (Cambrian Stage 2) on Burin Peninsula (Palacios et al. Reference Palacios, Jensen, Barr, White and Myrow2018).
This form co-occurs with leiosphaerids and fragments of Granomarginata prima in the Gibbett Hill Formation. Similar forms have been observed in the Ediacaran–Cambrian transition strata on the Digermulen Peninsula in Norway (A. Högström, pers. obs.). Arvestål & Willman (Reference Arvestål and Willman2020, fig. 12K, p. 26) documented a hooked microfossil with lateral serrations from the Ediacaran of Estonia, ‘possibly related to Ceratophyton’. In contrast, the Gibbett Hill microfossils are straight and have more defined and spread out lateral protrusions.
A high degree of coalification is evident in the dark colour of Gibbett Hill Formation microfossils (including leiosphaerids), corresponding to TAI = 4.
Unnamed Form C
Figure 6e
Material
One specimen (TSGf18450b 85×9, D16-HA-53) in the Indreelva Member, Stáhpogieddi Formation, Digermulen Peninsula, Norway.
Description
Elongate and conical vesicle. One end is tapering outwards, closed and blunt. The other end is nearly twice as wide and terminates in a wide opening. The vesicle appears creased, almost annulated.
Remarks
This microfossil shares an ovoidal shape and a similar polar opening to the Ediacaran–Cambrian taxon Teophipolia lacerata Kirjanov in Volkova et al. Reference Volkova, Kirjanov, Piscun, Pashkyavichene, Jankauskas, Keller and Rozanov1979 (e.g. Jankauskas et al. Reference Jankauskas, Mikhailova and Hermann1989, pl. 54, fig. 5); however, Unnamed Form C is smaller and narrower, with a faintly annulated vesicle. Similar microfossils also occur in the Cambrian Breidvika Formation, upper Vestertana Group on the Digermulen Peninsula (A. Högström, pers. obs.). This form is also strongly similar to Navifusa crassa (Sin & Liu, 1978), a rod-like vesicle with rounded ends and occasionally a distal opening on one end (cf. Zang, Reference Zang1988, pl. 40, fig. H). However, even though specimens of N. crassa with a crumpled or faintly annulated wall have been documented (Zang, Reference Zang1988), the original description of this taxon implies a smooth surface texture and a spongy wall. This contrasts with the delicate annulation in Unnamed Form C, so we consider it a separate entity.
Acknowledgements
This research was supported by the Swedish Research Council (Vetenskapsrådet) international postdoctoral grant (VR2016-06810) to H. Agić. Fieldwork in Arctic Norway was part of an expedition by the Digermulen Early Life Research Group (DELRG) funded by the Norwegian Research Council (grant no. 231103) to A. Högström. S. Jensen acknowledges funding from Ministerio de Economía, Industria y Competitividad (grant no. CGL 2017-87631-P). Material from Namibia was collected during the 35th IGC pre-conference field workshop to the Nama Group of southern Namibia, sponsored by IGCP 587 project ‘Identity, Facies and Time: The Ediacaran (Vendian) Puzzle’ and the ICS Subcommission on Ediacaran Stratigraphy, organized by P. Vickers-Rich, G. Narbonne, M. Laflamme, S. Darroch, A.J. Kaufman and L. Kriesfeld. In addition to workshop attendees, they are thanked for a delightful journey and engaging discussions in the field. Thanks also to G. Schneider, then Director, and staff at the Namibian Geological Survey for facilitating the IGCP 587 field conference, and to the owners of Farm Aar and Farm Pockenbank, B. Boehm-Erni and L. Gessart, for allowing access to their lands. We are also grateful to T. Palacios (University of Extremadura) and K. Grey (Geological Survey of Western Australia) who provided valuable feedback on an earlier draft, and thank three anonymous reviewers for their constructive comments.