1. Introduction
The most distinctive and most commonly preserved soft-bodied Ediacaran taxa from the Flinders Ranges of South Australia are, in order of frequency, Aspidella Billings, Reference Billings1872, Dickinsonia Sprigg, Reference Sprigg1947, Funisia Droser & Gehling, Reference Droser and Gehling2008 and Phyllozoon Jenkins & Gehling, Reference Jenkins and Gehling1978 (Droser et al. Reference Droser, Gehling and Jensen2006; Evans et al. Reference Evans, Dzaugis, Droser and Gehling2016). These genera tend to be found in large numbers on particular horizons, often to the near exclusion of other forms. Here we describe a new occurrence of this type, an event bed from Bathtub Gorge, Heysen Range, central Finders Ranges (Figs 1–4), dominated by two distinctive taxa: Phyllozoon hanseni and a newly named tubular body fossil, Aulozoon soliorum gen. et sp. nov. We document this small window into the late Ediacaran seafloor, describe the morphology of the organisms, the taphonomy of the deposit and its stratigraphic context, and explore their roles in the microbial mat-dominated benthic community.
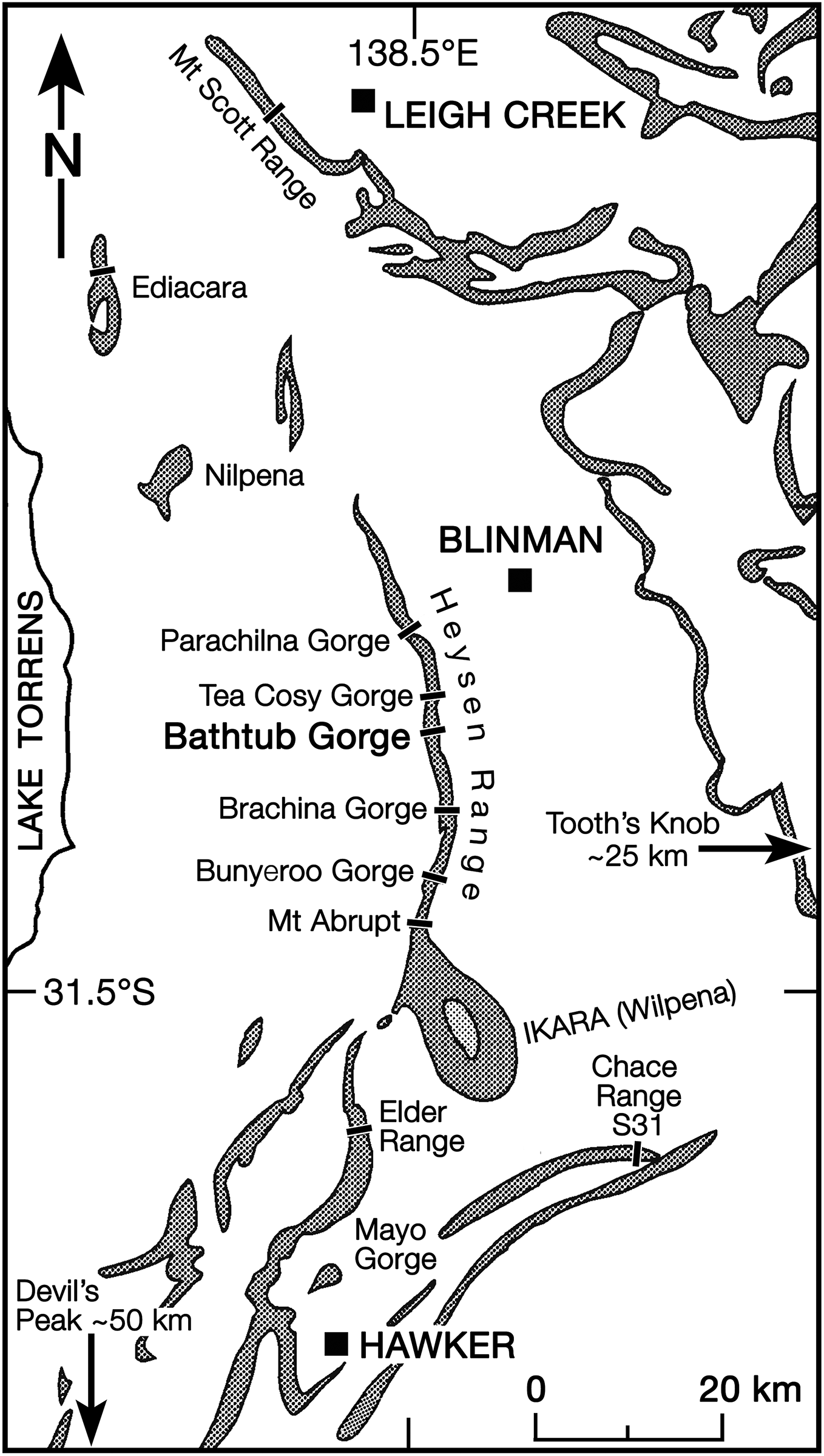
Fig. 1. Locality map for occurrences reported in the text. Grey shaded areas represent outcrops of the Pound Subgroup (Figs 2, 3). Tooth’s Knob is c. 25 km to the east of the right edge of the map, near Reaphook Hill; The Devil’s Peak, type locality for Phyllozoon hanseni, is c. 50 km south of the bottom edge of the map.

Fig. 2. Lithostratigraphy of the Ediacaran and lower Cambrian strata of the central Flinders Ranges, South Australia. The Ediacaran–Cambrian boundary is thought to coincide with the erosional surface at the base of the Uratanna Formation. The Uratanna Formation is missing from the section in Bathtub Gorge, Heysen Range (Fig. 3).
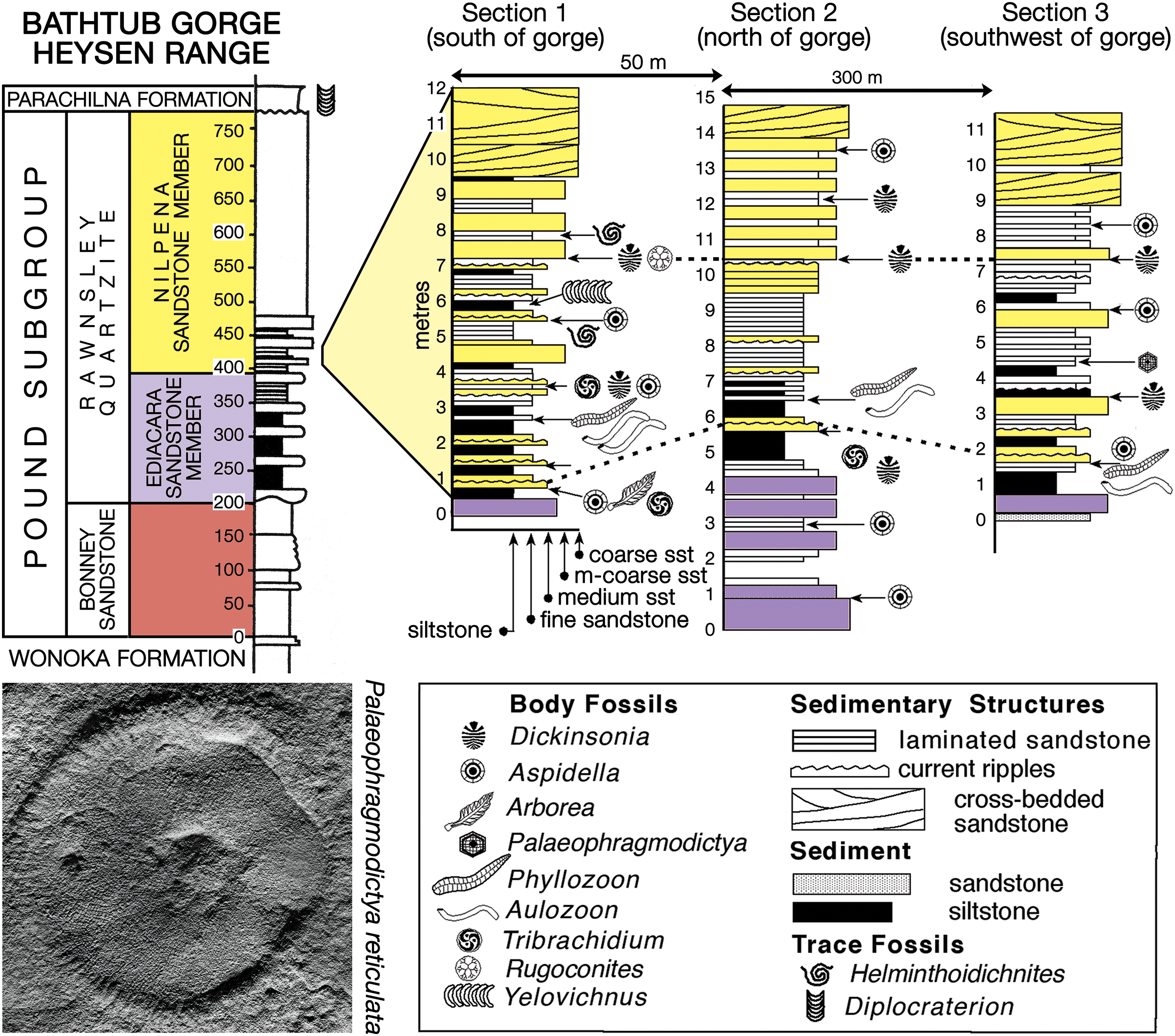
Fig. 3. Stratigraphy of the Pound Subgroup in Bathtub Gorge, Heysen Range, c. 30 km north of Ikara (Wilpena Pound), South Australia (Fig. 1); an outcrop view of section 1 is shown in Figure 4a. The assembled slab shown in Figure 5 is from the 6.7 m level of section 2 (Fig. 4b). The putative Ediacaran glass sponge Palaeophragmodictya reticulata (lower left, natural size) is found at the 4.5 m level in section 3 and Dickinsonia rex (online Supplementary Fig. S6b) at the 7.5 m level of section 1.

Fig. 4. Field photographs of the fossiliferous intervals, Bathtub Gorge. (a) Outcrop view of the lower part of the Nilpena Sandstone Member, Rawnsley Quartzite, in section 1 of Figure 3. The palaeontologist is making a latex cast of the ‘beaver-tailed’ specimen of Dickinsonia rex figured by Jenkins (Reference Jenkins, Lipps and Signor1992, fig. 14; online Supplementary Fig. S6b), which is on the base of the bed above him (7.5 m level, section 1, Fig. 3). (b) Jim Gehling extracting the largest piece of the Bathtub slab in 1992. His right foot is on the thick bed just above 4 m in section 2, Figure 3; the arrow points to the bed at 6 m, and the Bathtub slab is in situ at 6.7 m.
Most Ediacaran fossils are preserved on bed soles as either convex or concave moulds, but not normally as both. For example, discoidal form taxa such as Aspidella and Cyclomedusa Sprigg, Reference Sprigg1949 are almost invariably preserved in convex hyporelief while more resilient, apparently tissue-grade forms such as Dickinsonia are preserved in shallow, concave hyporelief (Wade, Reference Wade1968; Gehling, Reference Gehling1999). One of the two taxa dealt with here, Phyllozoon hanseni, is always preserved in convex relief on bed bases, is nearly always found in side-by-side arrays with other members of its cohort (Figs 5–8; online Supplementary Fig. S4, available at http://journals.cambridge.org/geo), and frequently overlies or underlies other taxa. In contrast, Dickinsonia is invariably preserved in concave hyporelief and is almost always well separated from other members of its species or other soft-bodied taxa. The exception that proves the rule are the convex hyporelief ‘footprints’ of Dickinsonia that are commonly considered to be resting traces left by mobile animals (Gehling et al. Reference Gehling, Droser, Jensen, Runnegar and Briggs2005; Evans et al. Reference Evans, Gehling and Droser2019). These and related matters are the subject of this article.

Fig. 5. Map of the sole of the re-assembled slab, c. 1 m2 in size, extracted from the 6.7 m level of section 2 (Fig. 3) in Bathtub Gorge, Heysen Range. The surface is dominated by specimens of Phyllozoon hanseni and Aulozoon soliorum, but there are also four ‘footprints’ of Dickinsonia cf. costata (A–D). The rose diagram shows the orientations of specimens of Phyllozoon with respect to the azimuth of crescentic ripple marks on the upper surface of the bed. In this view, east is left because the slab is inverted. This slab is on permanent display in the Ediacaran Gallery of the South Australian Museum.

Fig. 6. Base of sizeable slab from the Nilpena Sandstone Member, Rawnsley Quartzite, at the Nilpena precinct of the Ediacara Conservation Reserve illustrating how specimens of Phyllozoon hanseni are frequently found together, ‘hugging’ each other and even themselves. There are also several raised circular structures, poorly defined ‘footprints’ of Dickinsonia costata (black arrows) and an incomplete body fossil of D. cotata preserved in concave hyporelief (outlined; white arrow) on the Phyllozoon in the upper left corner of the slab. Note how adjacent modules of the Phyllozoons are juxtaposed at the sharp corners of the insides of tight turns. Boxed area is enlarged in Figure 7d; the large U-shaped specimen of Phyllozoon is SAM P57687.

Fig. 7. Preservation and anatomy of Phyllozoon hanseni. (a) Image of the sole of the reassembled slab from Bathtub Gorge (Fig. 5), c. 1 m2 in size. (b, c) Field photographs of a loose slab that was probably derived from the excavated horizon (6.7 m level, section 2, Fig. 3); boxed area in (b) enlarged in (c) to illustrate the rounded terminations of individual modules (arrow) and telescoping of modules at a link in the margin (up arrow); the round object is probably a frond holdfast (Aspidella). (d) Enlargement of boxed area of Figure 6 to show details of the frond margins, contact zones, axial seam and telescoped inner margin of tight turn (arrow); image is 5 cm wide. (e) Sketches based on two specimens of P. hanseni on the Bathtub slab (Fig. 5, bottom centre); growth proceeded from the rounded proximal end to the distal tapered end and is indicated by the gradient in the upper sketch; arrow points to telescoped modules on inner side of bend.

Fig. 8. (a) Sketch and (b) image of the lower surface of a loose slab from Bathtub Gorge that is almost certainly from the same bed as the large slab in Fig. 5. Two of the three individuals of Phyllozoon hanseni on this slab (SAM P35687-9) are partially overlapped, but the overlapped edges were not preserved. Small size differences between the tubular modules of the two individuals resulted in the centres of some modules of the upper individual overlying the seam between two modules of the lower individual. The spacing of these particular relationships is due to the vernier effect, highlighted by red shading. Slab previously illustrated by Gehling (Reference Gehling1991, pl. 3, fig. 2) and Droser et al. (Reference Droser, Gehling, Jensen and Briggs2005, fig. 5); see also Figure 9.
2. Stratigraphy and methods
In the Heysen Range, the late Ediacaran Pound Subgroup (there, c. 770 m thick) is composed of the red Bonney Sandstone and overlying white Rawnsley Quartzite (Figs 1, 2). Ediacaran fossils first appear in the Ediacara Sandstone Member of the Rawnsley Quartzite, which cuts disconformably down through the underlying Chace Sandstone Member into the Bonney Sandstone. However, the recent discovery of an erosional sequence boundary within the Ediacara Member in the Nilpena precinct of the Ediacara Conservation Park (Coutts et al. Reference Coutts, Gehling and García-Bellido2016) has led to the separation of a new uppermost Nilpena Sandstone Member of the Rawnsley Quartzite (Figs 2, 3; Gehling et al. Reference Gehling, García-Bellido, Droser, Tarhan and Runnegar2019). The fossiliferous facies of the Nilpena Sandstone Member also form the uppermost sedimentary cycle of Rawnsley Bluff, the SE end of Ikara (Wilpena Pound), and in the contiguous Heysen Range they occur from c. 1 km south of Bunyeroo George northwards through Brachina, Bathtub and Tea Cosy gorges (Fig. 1).
Richly fossiliferous horizons in the basal part of the Nilpena Sandstone Member are well exposed in Bathtub Gorge, which is a deep incision through the Heysen Range north of Ikara (Fig. 1). There, individual fossil horizons were traced over a strike distance of c. 300 m and logged into sections measured through a shallowing-upwards cycle, which begins near the top of the Ediacara Sandstone Member and ends in the lower part of the Nilpena Sandstone Member (Fig. 3). These three local stratigraphic sections, measured to decimetric accuracy, were keyed into a more general section through the Pound Subgroup in Bathtub Gorge (left column of Fig. 3). Phyllozoon hanseni and Dickinsonia rex Jenkins, Reference Jenkins, Lipps and Signor1992 are exclusive to the Nilpena Sandstone Member in both Bathtub Gorge and at Nilpena (Gehling et al. Reference Gehling, García-Bellido, Droser, Tarhan and Runnegar2019). The holotype of D. rex is from approximately the same level in Brachina Gorge (Wade, Reference Wade1972; Jenkins, Reference Jenkins, Lipps and Signor1992) and another specimen of D. rex from this interval, originally c. 1 m in length (Runnegar & Fedonkin, Reference Fedonkin, Lipps and Signor1992, fig. 7.5.7D), was partially extracted, cast and replaced in Brachina Gorge (online Supplementary Fig. S5, available at http://journals.cambridge.org/geo). The only other taxon from Bathtub Gorge that appears to be restricted to the Nilpena Sandstone Member is Palaeophragmodictya reticulata Gehling & Rigby (Reference Gehling and Rigby1996), a putative glass sponge that occurs at the 4.5 m level in section 3 of Fig. 3. Elsewhere (Nilpena, Chace Range, The Devil’s Peak; Fig. 1), Eoandromeda octobrachiata Feng et al. Reference Feng, Chongyu, Bengtson, Pengju, Ziqiang and Linzhi2008, Arkarua adami Gehling, Reference Gehling1987, Inaria karli Gehling, Reference Gehling1988 and an undescribed new species of Tribrachidium Glaessner in Glaessner & Daily, Reference Glaessner and Daily1959 are also confined to the Nilpena Member (Gehling et al. Reference Gehling, García-Bellido, Droser, Tarhan and Runnegar2019).
In Bathtub Gorge, as at Ediacara and Nilpena, the Nilpena Sandstone Member is disconformably overlain by the Diplocraterion-rich Parachilna Formation (Fig. 3; Jago et al. Reference Jago, Gehling, Betts, Brock, Dalgarno, García-Bellido, Haslett, Jacquet, Kruse, Langsford, Mount and Paterson2020), but in sections further north near Leigh Creek, the Uratanna Formation lies disconformably on the Pound Subgroup and is, in turn, conformably overlain by the Parachilna Formation (Fig. 2; Daily, Reference Daily1973). Trace fossils and acritarchs suggest an early Cambrian (Terreneuvian) age for the Uratanna Formation (Daily, Reference Daily1973; Jago et al. Reference Jago, Sun and Zang2002; Betts et al. Reference Betts, Paterson, Jacquet, Andrew, Hall, Jago, Jagodzinski, Preiss, Crowley, Brougham, Mathewson, García-Bellido, Topper, Skovsted and Brock2018).
Several fossiliferous horizons in Bathtub Gorge were traced out to link the three local measured sections; links were checked using bedding characteristics, and the consistency of preservation and composition of the fossils on each bed surface (Fig. 3). An oriented 1 m2 sample was extracted and transported as three separate pieces from the 6.7 m horizon in section 2 (Figs 3, 4b; 31.245011° S, 138.538350° E). Many specimens of two common species – Phyllozoon hanseni and Aulozoon soliorum – are preserved as a complex tangle on this reconstructed slab, parts or all of which have been illustrated previously by Runnegar (Reference Runnegar and Bengtson1994), Seilacher et al. (Reference Seilacher, Grazhdankin and Legouta2003, Reference Seilacher, Buatois and Mángano2005), Gehling et al. (Reference Gehling, Droser, Jensen, Runnegar and Briggs2005), Retallack (Reference Retallack2007), Seilacher (Reference Seilacher2007) and Seilacher & Gishlick (Reference Seilacher and Gishlick2015). Prior to reassembly (Fig. 5, online Supplementary Fig. S7, available at http://journals.cambridge.org/geo), the three separate pieces, including the edges, were copied using water-soluble latex plus medicinal bandages as a strengthening material. The resulting latex moulds were cast using uncoloured dental plaster. The edges and internal subdivision of fossils on the surfaces of these plaster casts were then traced with graphitic pencils, which provided sufficient contrast with the plaster to permit direct photocopying at half the original size. The reduced-scale photocopies were assembled into a mosaic, which was scanned electronically and used as the basis for the line drawing shown in Figure 5. The original photocopied mosaic has been rescanned for this work (online Supplementary Fig. S7); it represents raw data based directly on faithful copies of the fossils themselves. In contrast, the line drawing (Fig. 5) incorporates some degree of interpretation, albeit slight.
The azimuths of 22 specimens of the sword-fern-shaped frond of Phyllozoon hanseni on this slab were measured in 5 cm axial increments with respect to the average unidirectional transport direction indicated by asymmetric ripples on the upper surface of the slab and then averaged for each individual frond (Fig. 5). The cumulative widths of lateral modular elements were measured parallel to the axis on both the left and right sides of selected specimens and plotted to show the rate of growth (online Supplementary Fig. S1, available at http://journals.cambridge.org/geo). All other specimens used in this analysis were collected from horizons that were traced to the three measured sections. Sawn and smoothed sections were cut of the Phyllozoon bed in order to study the texture of the sole surface and the sand fill of tubular fossils. Catalogued figured material is included in the palaeontological collection of the South Australian Museum with numbers prefixed SAM P; other illustrated material remains in the field.
3. Taphonomy of the Phyllozoon bed in Bathtub Gorge
3.a. Introduction
A small window to the sea floor is provided by the undersurface of the reassembled c. 1 m2 slab (Figs 5, 7a; online Supplementary Figs S2, S7, available at http://journals.cambridge.org/geo), which was extracted from the north wall of Bathtub Gorge (6.7 m level of section 2; Figs 3, 4b) and is now part of the permanent display of Ediacaran fossils at the South Australian Museum. We use that surface and other pieces of the same bed found in place or as loose blocks in Bathtub Gorge to reconstruct the death assemblage captured by the deposition of this of 3–4-cm-thick event horizon. In addition to Phyllozoon hanseni and Aulozoon soliorum, which dominate the surface (Fig. 5), there are four indistinct impressions of sizeable individuals of Dickinsonia cf. costata, also preserved in convex hyporelief (Fig. 5, A–D). These are regarded as resting traces of the kind that elsewhere are commonly associated with overlapping ‘footprints’ made by individuals of Yorgia waggoneri and Dickinsonia costata (Ivantsov & Malakhovskaya, Reference Ivantsov and Malakhovskava2002; Gehling et al. Reference Gehling, Droser, Jensen, Runnegar and Briggs2005; Ivantsov, Reference Ivantsov2011; Evans et al. Reference Evans, Gehling and Droser2019). One sizeable piece of sandstone, which was not in situ but is probably from the slab level, has crisp, fan-shaped arrays of paired scratch marks – Kimberichnus teruzzii Ivantsov, Reference Ivantsov2013 – partly overlapping fronds of Phyllozoon hanseni (online Supplementary Fig. S3a, available at http://journals.cambridge.org/geo).
In which order did these four unrelated species end up on this deeply subtidal matground? In order to answer this question it is necessary to derive the superpositional order from overlapping relationships as seen on the bed base (Figs 5, 8–10; online Supplementary Figs S2, S3), which is equivalent to looking upwards into the water column from beneath the sediment-water interface. In this view, structures in the foreground were there first but were cast by sand falling from the background. However, two or more taxa may have been superimposed and then amalgamated by compaction into a composite cast of the underlying bed top following the removal of all organic matter (Fig. 9; online Supplementary Fig. S3b–d). It may therefore be difficult to be certain of the original order of deposition. An additional complicating factor is that understanding of the biology and preservation of the organisms has also evolved over several decades. In order to summarize these disparate biological and taphonomic alternatives, Table 1 gives a history of the various interpretations of the Bathtub slab surface and the organisms that are found on it; Figure 11 summarizes the three main taphonomic hypotheses: (a) ex situ death assemblage (Gehling et al. Reference Gehling, Droser, Jensen, Runnegar and Briggs2005); (b) in situ life assemblage (Seilacher et al. Reference Seilacher, Grazhdankin and Legouta2003, Reference Seilacher, Buatois and Mángano2005; Retallack, Reference Retallack2007, Reference Retallack2016); or (c) in situ Phyllozoon, ex situ Aulozoon (this study).

Fig. 9. Holotype of Aulozoon soliorum, SAM P35690 (black arrows) and another partly superimposed unregistered specimen of A. soliorum, preserved in convex hyporelief, that ends in a rounded termination (white arrows); lower Nilpena Sandstone Member, Bathtub Gorge; see also Figure 8. Both ends of the unregistered Aulozoon are overprinted by Phyllozoon, whereas the holotype interrupts the two Phylozoon fronds where it crosses them. This is the kind of evidence that supports the ‘winnowed and transported’ hypothesis (Fig. 11a).
Table 1. History of interpretations of the biology and taphonomy of the Bathtub Gorge slab surface and the organisms that inhabited it. Az – Aulozoon; Pz – Phyllozoon; Dick – Dickinsonia; mat – microbial mat; – symbolizes adjacency and / represents an interface between overlying and underlying organisms

3.b. Previous interpretations of the biostratinomy and taphonomy
Adolf Seilacher spent some time studying and sketching the first piece of the Bathtub slab, which was collected in 1991 (Runnegar, Reference Runnegar and Bengtson1994; Seilacher et al. Reference Seilacher, Grazhdankin and Legouta2003, Reference Seilacher, Buatois and Mángano2005; Seilacher, Reference Seilacher2007; Seilacher & Gishlick, Reference Seilacher and Gishlick2015). He interpreted the palaeoecology of the site in the following way: ‘Uniformly sized vendobionts (Phyllozoon) were living below the mat and are therefore perfectly preserved in their original “hugging” positions. Dickinsonia, in contrast, lived solitarily on top, where it could use its limited mobility … to digest new areas of the living mat. Accordingly, Dickinsonia specimens are preserved only as vague phantoms pressed through the mat by compaction. In the trace fossil interpretation, while bulldozing along the base of the biomat, the Aulozoon producer reacts upon collision with an undermat Phyllozoon in specific ways. If approaching it at a low angle, the animal contours the vendobiont and turns away, while collisions at larger angles are avoided by passing either above or below the Phyllozoon. In contrast, Aulozoon does not react to the Dickinsonia phantoms. All this speaks for a smothered matground, in which Phyllozoon grew below the biomat and Dickinsonia lived on top.’ (Table 1; Fig. 11b; Seilacher et al. Reference Seilacher, Buatois and Mángano2005, p. 329; Seilacher, Reference Seilacher2007, p. 178). This view was reinforced prior to his death in 2014: ‘Another member of this community, “Aulozoon” (invalid name) is here interpreted as the backstuffed burrow of a flatworm. As it moved under the mat for getting food and oxygen, it avoided the vendobionts by either turning away or passing underneath them.’ (Seilacher & Gishlick, Reference Seilacher and Gishlick2015, p. 142).
Greg Retallack (Reference Retallack2007, Reference Retallack2016) came to a radically different interpretation. He agreed with Gehling et al. (Reference Gehling, Droser, Jensen, Runnegar and Briggs2005) that Aulozoon was a body fossil rather than a trace fossil, but speculated that, because of an association of the ends of Aulozoon tubes with all four specimens of Dickinsonia of the Bathtub slab (Fig. 5, A–D), Aulozoon must be the vegetative mycelial rhizomorph of the thallus (mushroom), Dickinsonia. This was another in a long list of criteria for considering the classical Ediacaran assemblages to be terrestrial (e.g. Retallack, Reference Retallack2013).
Unsurprisingly, we reject Retallack’s scenario for reasons discussed elsewhere (Xiao et al. Reference Xiao, Droser, Gehling, Hughes, Wan, Chen and Yuan2013; Runnegar, Reference Runnegar2021). Seilacher’s idea that Phyllozoon was a benthic organism that lived below the mat is similar to our proposal that Phyllozoon was a prostrate inhabitant of the matground but, by analogy with other erniettomorphs that seem to have lived in the water column (Runnegar, Reference Runnegar2021), we prefer the scenario of a mat top lifestyle (Table 1; Fig. 11c). Seilacher’s undermat miner interpretation of Aulozoon is falsified by the geometry of the tubes, their lenticular cross-sections (Fig. 10) and the fact that they are in the event bed, not beneath the mat. The possibility suggested by Ivantsov (Reference Ivantsov2011) that Phyllozoon is the resting trace of a proarticulate animal similar to Dickinsonia was based mainly on a reinterpretation of the holotype, which is not well preserved. Better material found subsequently (Fig. 6) makes this suggestion implausible.

Fig. 10. Sketches (a, c) and images (b, d) of a paratype of Aulozoon soliorum (SAM P58399A-D), Ediacara Sandstone Member, Rawnsley Quartzite, Mount Scott Range, South Australia. Specimen (b), which was not in situ, has been sawn into four pieces to reveal cross-sections of the tube, A and D in (d), as shown diagrammatically in (a) and (c). Note ripple crests on top and base of bed in (b) and (d); black arrowed line indicates trend of ripple crest on bed sole.

Fig. 11. Three hypotheses for the taphonomy of the assemblage on the Bathtub slab surface: (a) ‘winnowed and transported assemblage’ Gehling et al. (Reference Gehling, Droser, Jensen, Runnegar and Briggs2005); (b) benthic community of mat-hugging photosynthetic vendobionts (Phyllozoon) and undermat flatworm bulldozers (Aulozoon), after Seilacher et al. (Reference Seilacher, Grazhdankin and Legouta2003, Reference Seilacher, Buatois and Mángano2005); and (c) our current hypothesis of in situ matground fronds (Phyllozoon) and sand-filled Aulozoon body fossil tubes, which were carried in by the storm surge that deposited the 3–4-cm-thick event bed.
3.c. Preservation of the fossils and biostratinomy
The two common body fossils – Phyllozoon and Aulozoon – seem to be tangled together as if transported and deposited by the storm surge itself (Gehling et al. Reference Gehling, Droser, Jensen, Runnegar and Briggs2005). However, this begs the question as to how incompletely sand-filled tubes (Aulozoon) and fluid- or tissue-filled fronds with a specific gravity of c. 1 (Phyllozoon) could be laid down together prior to burial by denser quartz sand. In addition, there is a notable paucity of overlap between adjacent individuals of Phyllozoon, and even Phyllozoon by Aulozoon, many of which ‘hug’ the outlines of the fronds (Figs 5, 6, 8; online Supplementary Fig. S4). We shall return to these matters, but first look at the preservation of this bed in more detail.
The smoothness of the bed sole, and its cementation with quartz and iron oxides, contrasts with the sugary, iron-oxide-poor surface of the underlying bed top. The parting medium was likely to have been the microbial mat that sealed the underlying sediment surface like cling film and allowed sand grains to settle and pack into the most compact arrangement possible. In contrast, the underlying bed top was formed during the waning current of a previous storm surge, so the sand grains remained loosely stacked. All specimens of Phyllozoon are preserved in convex hyporelief, presumably cast from impressions of the frond in the mat substrate prior to collapse of the modules and the onset of decay. Numerous examples of convex parts and concave counterparts, without an intervening clay or silt lamina, have been found. Phyllozoon may either have been pressed into the mat and the underlying unconsolidated bed top by the weight of the 3–4 cm thick storm surge sand that buried it, or have grown in place and moulded the mat accordingly. Deflation of the tubular modules must have preceded the cementation of the sole of the overlying sand, which cast the whole collapsed organism and the surrounding mat interface. Specimens of Phyllozoon that cross one another are preserved as composite moulds displaying the intersecting rib patterns of each individual (Figs 5, 6). There is no evidence that any specimens of Phyllozoon were filled with sand prior to burial or that the tubular modules collapsed unevenly. Relatively few specimens are visible in their entirety because the tapering distal ends are made from modules having progressively smaller width and relief, which are therefore less amenable to preservation. Most fronds are either straight or, more often, gently curved on the bed surface (Figs 5, 8; online Supplementary Fig. S4), but tight curvature also occurs (Fig. 6). On the inside edges of curved specimens, modules concertina rather than cross (Figs 6, 7b–d), which shows that they were joined to one another and not pinnate like sword fern fronds. The quilted arrangement of the modules and their resultant hydrostatic properties seem to have given the organism a considerable degree of planar rigidity, as indicated by the lack of folded or torn specimens.
Some Phyllozoon fronds are found lying in apposition, such that the module boundaries appear to line up between adjacent individuals and may even seem to continue from one individual to another (Figs 6, 8). This apparent biological continuity is explained by partial overlap followed by amalgamation during compaction; the Y-shaped connections are explained by a vernier effect, whereby two abutted sets of parallel lines with different spacing, such as the groves between modules of differently sized individuals, correspond only at certain positions. With some amount of overlap between subparallel individuals at the time of burial, younger modules of smaller size in one individual may split to accommodate the less numerous modules of an older portion of another individual. Parting at the seams between modules demonstrates that modules were stronger than the joins between them (Fig. 8). That this close proximity frequently occurs nearer the proximal ends of individuals is noteworthy. For a total of 62 specimens on 12 slabs, 49 are nearly parallel for most of their length, 13 of these pairs are touching at their margins and another 12 pairs display a significant amount of oblique overlap. This preferred alignment presents a striking contrast with other Ediacaran taxa that are routinely preserved as external moulds in negative hyporelief. For example, more than 160 well separated specimens of Dickinsonia costata occur on a 6 m2 jig-sawed bed extracted from nearby Crisp Gorge (Reid et al. Reference Reid, García-Bellido, Payne, Runnegar and Gehling2017). This contrast between Dickinsonia and Phyllozoon implies important differences in both biology and taphonomy between the two taxa. We suggest that Phyllozoon was a prostrate mat dweller that grew gregariously in such a way that individuals only slightly overlapped each other. Dickinsonia, on the other hand, was mobile enough to avoid the close proximity or even overlap that would inevitably result from the growth of closely spaced immobile juveniles (Reid et al. Reference Reid, García-Bellido, Payne, Runnegar and Gehling2017).
The lens-shaped sand-filled tubes of Aulozoon (Figs 5, 8–10; online Supplementary Fig. S2) may occasionally be separated from bed bases, revealing wrinkle marks on both sides of the extracted sausage-shaped tubes. Sawn sections show that the sand within the tubes is identical to that of the bed that buried them (Fig. 10), suggesting that the sand entered the tubes during the process of burial rather than by active incorporation. Some of the individuals on the studied slab are the largest known, reaching at least 1 m in observed length. The complex manner in which individuals of Aulozoon are interwoven shows that Aulozoon was a coherent, flexible tubular body fossil, not the mucus-lined gallery of a burrow like the early Cambrian trace fossil Plagiogmus Roedel, Reference Roedel1929 (McIlroy & Heys, Reference McIlroy and Heys2008). Haematitic silt, which separates the sand-filled tubes from the bed soles, may have been derived from an original pyritic film that seems to have stiffened many Ediacaran organic materials during decay (Gehling, Reference Gehling1999). The physical integrity of the Aulozoon tubes and their pattern of deformation imply a secreted tissue rather than an agglutinated grade of construction.
The putative traces, Dickinsonia ‘footprints’ and Kimberichnus scratch marks must be both benthic and formed in situ if they are really trace fossils (Gehling et al. Reference Gehling, Runnegar and Droser2014; Evans et al. Reference Evans, Gehling and Droser2019). The fact that Kimberichnus is superimposed on Phyllozoon (online Supplementary Fig. S3a) could be regarded as evidence that the Phyllozoon fronds were not prostrate lichens inhabiting the matground (Retallack, Reference Retallack2007), but instead were transported prior to burial by the overlying bed. However, it is also possible the scratches were cut through the Phyllozoon frond. The situation with the Dickinsonia ‘footprints’ is equally puzzling, because in all four cases the ‘footprints’ are interrupted by Aulozoon tubes (Fig. 5, A–D). At first, it is difficult to imagine that the sandy tubes could have eliminated any trace of the pre-existing concave footprints during burial, but there seem to be only five possibilities: (1) the footprints pre-date the sandy tubes and were partly obliterated by them; (2) the footprints post-date the deposition of the sandy tubes; (3) the footprints are transported body fossils, not trace fossils, and they overlie the tubes; (4) the footprints are the bounce marks of bodies carried by currents and then moved on again; or (5) the sandy tubes are compacted horizontal burrows within the event bed, and not body fossils. The last alternative is discussed above and rejected.
The preservation of the footprints as effaced, convex hyporelief structures is similar to that seen at other sites, most notably those on the IT-NA surface of the Nilpena precinct, where a long series of overlapping footprints is strong evidence for the trace fossil interpretation (Evans et al. Reference Evans, Gehling and Droser2019). Perhaps the most likely scenario for the Bathtub ‘footprints’ is that they were already on the matground and were overwritten by the sand-filled tubes, which settled quickly like gravel clasts prior to any sand grains during the storm surge event. As a result, there was no sediment between tube and the footprint to record the presence of the former beneath the latter. Although only some Aulozoon tubes are clearly underlain by Phyllozoon fronds (Fig. 10; online Supplementary Fig. S3d), those Phyllozoon that are interrupted by Aulozoon (Figs 5, 8, 10; online Supplementary Fig. S3b, c) may therefore also have been underneath the tubes. The difference in preservation may simply be a result of the amount of surge sand that ended up between the frond and the tube. This is well shown by the two highlighted Aulozoon tubes in Figure 10; the holotype (black arrows) interrupts the Phyllozoon fronds because it was laid down directly on them, and the other Aulozoon (white arrows) is a sand-filled sausage within the event bed, meaning that the sediment underneath it could record the passage of the fronds where they crossed the tube. In this and other cases, sediment within the tubes was able to cast underlying specimens of Phyllozoon leaving a composite impression of both taxa (online Supplementary Fig. S3d). Where specimens of Phyllozoon are interrupted by Aulozoon tubes (Figs 5, 8; online Supplementary Figs S2, S3b,c), it has been assumed that the former overlies the latter (Gehling et al. Reference Gehling, Droser, Jensen, Runnegar and Briggs2005). This is also suggested in some cases by the way the bed base curves inwards (upwards) as the Phyllozoon approaches and leaves the Aulozoon tube. A reviewer suggested testing this hypothesis by removing a section of a sandy Aulozoon tube to expose part of an overlying Phyllozoon. This was performed (online Supplementary Fig. S3b, c) and the hypothesis was falsified; there is no trace of Phyllozoon above the sand-filled tube (online Supplementary Fig. S3c). We therefore conclude that, of the five alternatives, option (1) is correct; the sandy tubes of Aulozoon obscure underlying body and trace fossils because they were deposited on them without any intervening sediment. A benthic community represented by Phyllozoon fronds and Dickinsonia ‘footprints’ was therefore overprinted with a death assemblage of Aulozoon sandy tubes carried in by the storm surge that deposited the Bathtub slab event bed.
3.d. Discussion and taphonomic conclusions
In summary, this snapshot of the sea floor was captured by a thin sandy event bed deposited by the waning phase of a storm surge in a deep subtidal environment. The surface on which the event bed was deposited was a well developed matground inhabited by numerous prostrate Phyllozoon fronds, scattered individuals of Dickinsonia costata, the producer of the Kimberichnus scratch marks and rare examples of a few other common Ediacaran taxa. During the surge, evacuated Aulozoon tubes were washed in and those that had been infiltrated with sand sank to the bottom first at the speed of gravel clasts. Following deposition of the sandy component of the storm surge, the hydrostatic skeletons of the fluid- or tissue-filled Phyllozoon fronds collapsed so that the bodies are preserved as convex hyporelief casts on the fine sandstone bed base. This then is a snapshot of a death assemblage of in situ and ex situ organisms that may all have had a patchy distribution on this subtidal sea floor.
4. Systematic palaeontology
Orphan plesion Erniettomorpha Pflug, Reference Pflug1972
Discussion. The higher taxonomy of Ediacaran soft-bodied organisms is in a state of flux but there is widespread agreement that the Namibian genera Pteridinium Gürich, Reference Gürich1933 and Ernietta Pflug, Reference Pflug1966 are sufficiently closely related to be included in the same extinct clade, the Erniettomorpha Pflug, Reference Pflug1972. This ‘orphan plesion’ may also include Phyllozoon, in the sense that Phyllozoon is something like a two-vaned Pteridinium (Jenkins & Gehling, Reference Jenkins and Gehling1978).
Phyllozoon Jenkins & Gehling, Reference Jenkins and Gehling1978
Type species. Phyllozoon hanseni Jenkins & Gehling, Reference Jenkins and Gehling1978, by original designation and monotypy.
Phyllozoon hanseni Jenkins & Gehling, Reference Jenkins and Gehling1978
Figs 5–8; online Supplementary Figs S1, S3, S4.
1978 Phyllozoon hanseni Jenkins & Gehling, pp. 357–58, fig. 7.
1991 Phyllozoon hanseni Jenkins and Gehling; Gehling, pl. 3, fig. 2.
1992 Phyllozoon Jenkins and Gehling; Runnegar, fig. 3.10.
1994 Phyllozoon hanseni Jenkins and Gehling; Runnegar, fig. 3.
2003 Phyllozoon hanseni Jenkins and Gehling; Seilacher, Grazhdankin & Legouta, pp. 45–46, Fig. 5.
2005 Phyllozoon Jenkins and Gehling; Gehling, Droser, Jensen & Runnegar, p. 51, Fig. 5.
2005 Phyllozoon Jenkins and Gehling; Seilacher, Buatois & Mángano, pp. 328–29, Fig. 4.
2007 Phyllozoon Jenkins and Gehling; Seilacher, p. 178, pl. 62.
2007 Phyllozoon hanseni Jenkins and Gehling; Jenkins & Nedin, p. 209, Fig. 8c.
2007 Phyllozoon hanseni Jenkins and Gehling; Retallack, p. 17, Fig. 7.
Type material. SAM P19508A (holotype) and P19508B-C (paratypes), all on same slab, Nilpena Sandstone Member, Rawnsley Quartzite, The Devil’s Peak, south of Quorn, southern Flinders Ranges; 32.414995° S, 137.991176° E (Jenkins & Gehling, Reference Jenkins and Gehling1978, Fig. 7).
Revised diagnosis. Frond elongate and biserial, rounded at the presumed proximal end, parallel sided for some distance then tapering to a narrow distal growing tip formed of progressively narrower and shorter modules. Frond constructed entirely from straight to slightly curved units (collapsed tubular modules), gently convex in profile on bed bases, alternating in a zig-zag fashion across the midline, and extending at 70–90° to it; adjacent ribs are separated by well defined narrow grooves; marginal terminations of modules rounded, often indistinct; first three to five modules of proximal region of frond short and wide; others decrease in size gradually away from the proximal end of frond but maintain approximately equal length perpendicular to axis until frond begins to taper distally.
Distribution. The type locality, The Devil’s Peak, is c. 140 km SSW of Bathtub Gorge (Fig. 1). Apart from these two sites, Phyllozoon has been recorded from 30 localities throughout the central Flinders Ranges, including several with measured stratigraphic sections. These include the Nilpena Sandstone Member (Mayo Gorge, Chace Range, Tooth’s Nob, Nilpena precinct of the Ediacara Conservation Park); specimens have also been photographed from stratigraphic levels well above the richly fossiliferous Ediacara Sandstone Member beneath Mount Abrupt and within Tea Cosy Gorge, north of Bathtub Gorge (Fig. 1). Notably, Phyllozoon has not been recovered from the Ediacara Hills sector of the Conservation Park, c. 20 km north of Nilpena (Fig. 1).
Description. Phyllozoon is an elongate strap-shaped frondose organism with the presumed proximal end being more rounded and wider than the tapering distal end, where the addition of new tubular modules probably occurred. Both the midline and boundaries between collapsed modules form sharp grooves, suggesting quilting. The peripheral ends of the modules are generally imprecisely defined. The boundaries of the modules are slightly curved in the plane of the bed, and weakly concave toward the narrower, apical end of the frond. With the exception of near the proximal end, the modules are relatively constant in length (axis to margin) for much of the frond but decrease in length for the distal third. The incomplete holotype is 18 cm long and 5.5 cm at the widest point. Six nearly complete specimens from Bathtub Gorge vary in length from 21 to 26 cm (average 23.5 cm) and from 3.8 to 4.2 cm (average 4 cm) in maximum width. The average length and width of complete specimens from all localities is 24.1 cm and 3.9 cm, respectively. Complete specimens have 80–100 modules on each side (online Supplementary Fig. S1), varying in width from 1 to 5 mm (average 2.1 mm).
Discussion. Phyllozoon does not seem to be an upright frondose or petaloid form, as previously inferred by Jenkins & Gehling (Reference Jenkins and Gehling1978). Over-printing by examples of well-known Ediacaran taxa (e.g. Fig. 6) implies that Phyllozoon was arrayed as recumbent gregarious sets of prostrate fronds at the time of burial. There is no evidence of a point of attachment, rachis or stolon. Modules were probably simple tubes lacking partitions, and joined laterally and at the zig-zag axis. Cumulative widths of modules plotted against number of modules gives a growth curve that is slightly negatively allometric (online Supplementary Fig. S1). The only suggestion that the modules were not closed distally is the often poor preservation of their peripheral ends. However, evidence for air-mattress-like hydrostatic strength (Fig. 8) – the ‘quilted pneu’ of Seilacher (Reference Seilacher1992) – makes that improbable.
Phyllozoon is also found at other places in the Flinders Ranges (Fig. 6; online Supplementary Fig. S4) but at almost all other sites is not associated with Aulozoon. On the other hand, Aulozoon occurs at Ediacara in the Mount Scott Range (Fig. 10) and in a number of other areas without Phyllozoon. These separations negate the idea that Phyllozoon might somehow be part of the inhabitant of Aulozoon tubes. However, several other sites do show the ‘hugging’ that seems characteristic of Phyllozoon and also an association with Dickinsonia footprints and body fossils (Fig. 6). This seems to be a characteristic feature of its gregarious habit.
Unlike Pteridinium from Namibia (Pflug, Reference Pflug1970), Russia (Fedonkin, Reference Fedonkin, Lipps and Signor1992), North Carolina (Gibson et al. Reference Gibson, Teeter and Fedonkin1984), Ediacara (Glaessner & Wade, Reference Glaessner and Wade1966) and Nilpena (Gehling & Droser, Reference Gehling and Droser2013), a third ribbed vane is not present in Phyllozoon (Jenkins & Gehling, Reference Jenkins and Gehling1978). Like other erniettomorphs, Phyllozoon lacks evidence for discrete structures smaller than the modules. Resolution of its affinities will require a fuller understanding of the whole clade.
Orphan plesion Dickinsoniomorpha Erwin et al. Reference Erwin, Laflamme, Tweedt, Sperling, Pisani and Peterson2011
Genus Dickinsonia Sprigg, Reference Sprigg1947
Type species. Dickinsonia costata Sprigg, Reference Sprigg1947, by monotypy.
Dickinsonia rex Jenkins, Reference Jenkins, Lipps and Signor1992
Online Supplementary Figs S5, S6.
1972 Dickinsonia elongata Glaessner and Wade, Reference Glaessner and Wade1966; Wade, p. 178, pl. 7, Fig. 2.
1992 Dickinsonia elongata Glaessner and Wade, Reference Glaessner and Wade1966; Runnegar & Fedonkin, p. 383, Fig. 7.5.7D.
2005 Dickinsonia rex Jenkins, Reference Jenkins, Lipps and Signor1992; Gehling, Droser, Jensen & Runnegar, p. 51, Fig. 4.
Type material. Holotype, SAM P18086 (Wade, Reference Wade1972, pl. 2, Fig. 2) probably from the base of the Nilpena Sandstone Member, Rawnsley Quartzite, Brachina Gorge, Heysen Range; 31.342481° S, 138.569593° E; specimen collected by RJF Jenkins prior to 1972. De facto paratype (Fig. 6b), specimen illustrated by Jenkins (Reference Jenkins, Lipps and Signor1992, fig. 14) from the base of thick sandstone bed at the 7.5 m level (section 1, Figs 3, 4a), Nilpena Sandstone Member, Rawnsley Quartzite, Bathtub Gorge; 31.245011° S, 138.538350° E. This significant figured specimen remains in the field (last seen 24 May 2005); two unregistered plaster casts of it are currently held in the palaeontological collection of the South Australian Museum.
Description. Large, stadium-shaped species of Dickinsonia with numerous closely spaced modules. The holotype has the tail end folded over but Wade (Reference Wade1972) estimated its length as 38–39 cm and width c. 14 cm, a L/W ratio of c. 1.5 compared with a L/W of c. 1 for D. costata (Evans et al. Reference Evans, Droser and Gehling2017). Capable of growth to extraordinary sizes (c. 750 mm; online Supplementary Fig. S5) and, in larger specimens, having the outer edges of the modules expanded and imbricated. This feature is strikingly obvious in SAM P40168 (Gehling et al. Reference Gehling, Droser, Jensen, Runnegar and Briggs2005, fig. 4), a huge intact individual c. 840 mm in length that is part of the permanent display at the South Australian Museum. In this specimen the modules are expressed as a closely spaced curved or even sinuous ridges and the module boundaries as deep grooves. The midline is crisp but exceedingly narrow for the size of the specimen, and there is no topographic evidence for a coincident internal organ. Where the distal edges of the modules flare and imbricate, there are ridges on the module walls approximately midway between the original dorsal and ventral surfaces. The edges of the specimen are expanded or withdrawn at a number of places on the periphery, presumably from perimortem processes. The number of modules is c. 400.
Distribution. As for Phyllozoon hanseni, D. rex may be confined to the Nilpena Sandstone Member of the Rawnsley Quartzite (Gehling et al. Reference Gehling, García-Bellido, Droser, Tarhan and Runnegar2019). The holotype came from ‘high in the cliff’ in Brachina Gorge (Wade, Reference Wade1972, p. 178), and the in-place topotype shown in online Supplementary Figure S5 is from the base of a 25 cm thick sandstone at the approximate position of the lowest occurrence of facies 7 in the Brachina Gorge section of McMahon et al. (Reference McMahon, Liu, Tindal and Kleinhans2021, fig. 3). Both occurrences are low in the Nilpena Sandstone Member. Elsewhere, D. rex occurs within the Nilpena Sandstone Member at Nilpena (Gehling et al. Reference Gehling, García-Bellido, Droser, Tarhan and Runnegar2019), Bathtub Gorge (online Supplementary Fig. S6a, available at http://journals.cambridge.org/geo) and in the Chace Range (online Supplementary Fig. S6b), where it occurs with Arkarua adami Gehling, Reference Gehling1987 and Inaria karli Gehling, Reference Gehling1988 in measured section S31, east end of the range (Fig. 1; Gehling, Reference Gehling1987, Reference Gehling2000).
Discussion. Glaessner & Wade (Reference Glaessner and Wade1966) proposed Dickinsonia elongata for elongate species of Dickinsonia that taper slightly posteriorly. They illustrated only the holotype (SAM P13767) that, although 16 cm long, they regarded as juvenile in comparison with incomplete specimens that were 30–40 cm in length. However, Jenkins (Reference Jenkins, Lipps and Signor1992) considered the holotype to be a poorly preserved specimen of D. costata (we concur), so elongata became a subjective junior synonym of costata and the name elongata should be restricted to its holotype. For the larger elongate examples of Dickinsonia, Jenkins (Reference Jenkins, Lipps and Signor1992) introduced the specific epithet rex that he described as a new name rather than a new species. Perhaps for this reason, the taxonomic status of D. rex has seemed unclear. This brief re-description is aimed at eliminating any uncertainty about the validity of the species.
When Jenkins (Reference Jenkins, Lipps and Signor1992) proposed D. rex, he did not provide a systematic description of the new species because elongata had previously been well described by Glaessner & Wade (Reference Glaessner and Wade1966) and Wade (Reference Wade1972). At the time, there was a community understanding of the species elongata, based on specimens from Ediacara and Brachina Gorge. However, the unfortunate choice of an unrepresentative holotype because it was ‘a juvenile specimen with both anterior and posterior ends’ (Glaessner & Wade, Reference Glaessner and Wade1966, p. 621) required suppression of the name. Equally unfortunately, only the holotype of elongata was figured in 1966 and the paratypes were not listed by SAM P number. A similar situation pertained in 1972, when Wade mentioned two almost-complete adult specimens from Brachina Gorge but figured only one, the holotype of D. rex (Wade, Reference Wade1972, pl. 7 fig. 2). When Jenkins (Reference Jenkins, Lipps and Signor1992) proposed D. rex as a replacement name for D. elongata, only two specimens of the then well understood species had therefore been illustrated. For this reason, the specimen figured by Jenkins (Reference Jenkins, Lipps and Signor1992, fig. 14) from Bathtub Gorge (Fig. 6b) serves as a de facto paratype of D. rex.
Some individuals of Dickinsonia costata grew to a substantial size (Runnegar, Reference Runnegar2021, fig. 4b) but nowhere near the size of the largest individuals of D. rex (online Supplementary Fig. S6; Gehling et al. Reference Gehling, Droser, Jensen, Runnegar and Briggs2005, fig. 4). Furthermore, D. costata maintains an almost 1:1 L/W ratio throughout growth in contrast to D. rex (Runnegar, Reference Runnegar1982; Evans et al. Reference Evans, Droser and Gehling2017). Dickinsonia tenuis Glaessner & Wade (Reference Glaessner and Wade1966) is another large species of Dickinsonia, but it is more equidimensional (L/W c. 1.3) and had approximately three times as many modules per unit length than rex. Small specimens of D. tenuis and D. rex may be difficult to tell apart; however, in large specimens the differences are obvious because of the difference in proportions and the spacing of the modules. Dickinsonia lissa Wade, Reference Wade1972 is even more elongate (L/W c. 3) and has a very large number of narrow modules and a prominent axial ridge, features that are also obvious in Russian members of this species (Ivantsov, Reference Ivantsov2007). The great differences in proportions and module size readily distinguish lissa from rex.
Phylum Annelida Lamarck, Reference Lamarck1809?
Genus Aulozoon gen. nov.
Type species. Aulozoon soliorum sp. nov.
Derivation of name. From aulos, Greek, flute or pipe and zoön, Greek, animal.
Diagnosis. Large, cylindrical, unmineralized, flexible tube, one to several centimetres in diameter and up to at least 1 m in length, often with at least one rounded end, typically preserved in slightly to significantly convex hyporelief as empty or partly sand-filled structures; straight, looped or coiled, lenticular or D-shaped in cross-section, and separated from surrounding matrix by ferruginous quartz silt.
1969 Form D Glaessner, pp. 381–2, Fig. 5E.
1995 Palaeophycus tubularis Hall, Reference Hall1847; Jenkins, pp. 56–8, pl. 1F.
2003 Aulozoon (nomen nudum); Seilacher, Grazhdankin & Legouta, pp. 45–50, Fig. 5.
2005 Aulozoon (nomen nudum); Seilacher, Buatois & Mángano, pp. 328–29, Fig. 4.
2005 Form D Glaessner; Droser, Gehling & Jensen, pp. 134–5, Fig. 5.
2007 Aulozoon (nomen nudum); Seilacher, p. 178, pl. 62.
2007 Aulozoon (nomen nudum); Retallack, p. 17, Fig. 7.
2015 Aulozoon (nomen nudum); Seilacher & Gishlick, p. 142.
Aulozoon soliorum sp. nov.
Figs 5, 8–10; online Supplementary Figs S2, S3b–d.
Derivation of name. From solium, Latin, bathtub; genitive plural because Bathtub Gorge has many bathtubs (waterholes).
Discussion. This new genus and species was described as Aulozoon arteria Gehling (Gehling, Reference Gehling1996). The generic name was adopted informally by Seilacher et al. (Reference Seilacher, Grazhdankin and Legouta2003, Reference Seilacher, Buatois and Mángano2005) and thus became by definition a nomen nudum, lacking a designated type specimen and an adequate description and definition. Here, we rectify that situation.
Type material. Holotype, SAM P35690A, part (Figs 8, 9) and SAM P35690B, counterpart; paratype, SAM P58400 (online Supplementary Fig. S2), all from the same surface (Fig. 5), Nilpena Sandstone Member, Bathtub Gorge; 31.245011° S, 138.538350° E; paratype, SAM P58399A–D (Fig. 10), Rawnsley Quartzite, Mount Scott Range, via Leigh Creek, South Australia; 30.621092° S, 138.356203° E (Fig. 1).
Description. Aulozoon is a tubular body fossil, probably originally circular in cross-section, that varies in diameter from c. 1–3 cm and is normally preserved in convex hyporelief on bed bases. The full length is unknown, but some individuals exceed c. 1 m. Individuals on the same surface frequently overlap and cross each other, but do not merge at intersections. Unfilled tubes are flat or slightly convex in hyporelief and have sharply incised margins. Tubes are generally sinuous, or make broad loops with a radius of turn roughly proportional to the tube width (Figs 4–6). Angular bends in the loops involve marked kinking on the inside of the turns (Figs 6, 7). The margins are smooth in straight or sinuous specimens, but more irregular or slightly scalloped on some loops and near poorly preserved terminations. The surface of specimens varies from smooth and featureless to transversely or obliquely wrinkled with sharp grooves that are most prominent on the inside of the bends. Wrinkle marks are more common in sand-filled specimens close to the basal rounded termination, when preserved, than on the hyporelief casts of unfilled tubes. A deep, discontinuous, median longitudinal groove occurs on parts of some specimens without transverse wrinkles. Apparent terminations are simple and rounded, but may only represent the point at which the tube curved upwards into the bed that buried it or where the sediment fill ended.
Distribution. Aulozoon soliorum is a common fossil at Ediacara and is widespread in the Flinders Ranges. We illustrate specimens from Bathtub Gorge and the Mount Scott Range (Fig. 1), but it occurs at many other sites in both the Ediacara and Nilpena members of the Rawnsley Quartzite.
Discussion. In a review of the then known Ediacaran and early Cambrian trace fossils, Glaessner (Reference Glaessner1969) identified Aulozoon soliorum as vermiform burrow, which he called ‘Form D’. Runnegar & Fedonkin (Reference Fedonkin, Lipps and Signor1992) and Fedonkin & Runnegar (Reference Runnegar and Schopf1992) recognized the three-dimensional nature of these fossils, and suggested that they may have been mucilaginous tubes made by worm-like metazoans. A sediment-filled specimen was illustrated by Jenkins (Reference Jenkins1995, pl. 1F) as the trace fossil Palaeophycus tubularis Hall, Reference Hall1847, but there is really no close similarity; Aulozoon differs from Palaeophycus in its lack of burrow junctions, more uniform cylindrical shape and the fact that it never branches. Other previous references to Aulozoon soliorum as the nomen nudum ‘Aulozoon’ are discussed in Section 3.
Several of Glaessner’s (Reference Glaessner1969) trace fossils have turned out to be tubular body fossils (Droser et al. Reference Droser, Gehling, Jensen and Briggs2005; Sappenfield et al. Reference Sappenfield, Droser and Gehling2011). One of these, Somatohelix sinuosis, overlaps the size range of Aulozoon soliorum but Somatohelix – as the name suggests – was helically coiled, albeit at a long wavelength. No evidence of systematic curving or coiling is present in the tubes of Aulozoon. The only other form that is comparable to Aulozoon soliorum seems to be Vittatusivermis annularius from the earliest Cambrian Period of China (Zhang et al. Reference Zhang, Liu, Isozaki and Sato2017). Although clearly a body fossil, the tube of Vittatusivermis is finely annulated and, in one case, twisted through 180°. Neither of these features is seen in Aulozoon.
Runnegar (Reference Runnegar and Bengtson1994) discussed the resemblance of Aulozoon to the tubes of the living vestimentiferan annelid, Riftia pachyptila. Tubes of Riftia, described by Jones (Reference Jones1980) from the Galápagos Rift on the East Pacific Rise, share the same dimensional proportions, basal blind ending, transverse wrinkles and manner of kinking as Aulozoon but, in the absence of any evidence of the nature of the inhabitant of the Aulozoon tubes, the significance of the similarities is difficult to assess. Riftia tubes are cylindrical, fast growing from both ends (tens of cm–1), constructed of chitin (c. 33%) and protein, but also have complications such as basal septa and forking not seen in Aulozoon (Gaill & Hunt, Reference Gaill and Hunt1986; Gaill et al. Reference Gaill, Shillito, Ménard, Goffinet and Childress1997). In the related vestimentiferan Teunia jerichonana, the tube is formed from criss-crossed ribbons of fibrous chitin arranged in a ‘nematic’ fashion (Gaill et al. Reference Gaill, Persson, Sugiyama, Vuong and Chanzy1992). This arrangement is similar to that seen in the Ediacaran fossil Sabellidites cambriensis, which Moczydłowska et al. (Reference Moczydłowska, Westall and Foucher2014) interpreted to be the tube of a siboglinid, and perhaps a vestimentiferan annelid worm. Comparable worm tubes attributed to annelids have a substantial Phanerozoic record (Georgieva et al. Reference Georgieva, Little Crispin, Watson, Sephton, Ball and Glover2019), and most of the Ediacaran–Terreneuvian cylindrical body fossils of Budd’s ‘tube world’ (Budd & Jackson, Reference Budd and Jackson2016), whether mineralized or not, are now thought to be stem or crown annelids (Yang et al. Reference Yang, Steiner, Schiffbauer, Selly, Wu, Zhang and Liu2020). It is therefore plausible to regard an annelid affinity for Aulozoon as the null hypothesis. Recent discoveries of widespread methane leaks from oceanic seafloor sediments (Goffredi et al. Reference Goffredi, Tilic, Mullin, Dawson, Keller, Lee, Wu, Levin, Rouse, Cordes and Orphan2020) makes a chemosymbiotic lifestyle for the inhabitant of Aulozoon tubes a possibility.
5. Conclusions
The event bed with Phyllozoon and Aulozoon in Bathtub Gorge is an example of an assemblage that is younger and different from the classical fossiliferous horizons of the older Ediacara Sandstone Member. Because the site is in a deep gorge that cuts the Heysen Range, only one small c. 1 m2 window was amenable for study. Despite this limitation, it is clear that this event bed preserves a time-lapse video of the depositional environment and its ecology, rather than a single snapshot. The deeply subtidal matground that developed during quiet stable conditions was inhabited by patchy gregarious aggregations of prostrate Phyllozoon fronds and some dispersed members of other clades, including the animals that made the Dickinsonia cf. costata ‘footprints’ and the Kimberichnus teruzzi scratch traces. During an energetic storm surge, vacated sand-filled tubes of Aulozoon soliorum were carried in and deposited swiftly, falling like pebbles rather than sand grains. As a result, their lower surfaces did not replicate the parts of the Phyllozoon fronds that lay beneath them, giving the impression when seen from the bed sole side that the fronds were above the tubes. In fact, all Aulozoon tubes probably overlie all Phyllozoon fronds, meaning that this is an example of an in situ life assemblage overprinted by an ex situ death assemblage. Exactly how Phyllozoon and Aulozoon lived and operated remains unclear, and their biological affinities are still obscure. However, Phyllozoon is almost certainly a member of the orphan plesion Erniettomorpha – which includes Pteridinium and Ernietta – and Aulozoon is not.
Supplementary material
To view supplementary material for this article, please visit https://doi.org/10.1017/S0016756821000509
Author contributions and acknowledgements
JGG and BR discovered and collected the material from Bathtub Gorge in 1991–1992 with the assistance of Stefan Bengtson, Lars Holmer, Simon Conway Morris, Christine Runnegar and Matt Saltzman. We are grateful to Keith Wiseman for access via Commodore station and to Bill McIntosh for access via Gum Creek. JGG and BR wrote the paper, prepared the illustrations and contributed equally to this work; authorship is in alphabetical order. Diego García-Bellido generously allowed us to illustrate a specimen from Nilpena (Fig. 6). We thank Sören Jensen and the IMECT 2019 organizing committee for the invitation to participate and some financial support to do so. JGG was funded by the Australian Research Council; BR by the US National Science Foundation and UCLA. Thoughtful criticism and suggestions from Marc Laflamme and an anonymous reviewer helped to improve the published manuscript.