1. Introduction
The early Palaeozoic Caledonian mountain belt in Scotland (Fig. 1) resulted from the collision of three major plates, the Laurentian continent to the west, the Avalonian plate in the SE and Baltica in the NE (Dewey, Reference Dewey1969; Lambert & McKerrow, Reference Lambert and McKerrow1976; McKerrow et al. Reference McKerrow, Leggett and Eales1977, Reference McKerrow, MacNiocaill and Dewey2000; Dewey & Shackleton, Reference Dewey and Shackleton1984; Chew & Strachan, Reference Chew, Strachan, Corfu, Gasser and Chew2014; Dewey et al. Reference Dewey, Dalziel, Reavy and Strachan2015; Strachan et al. Reference Strachan, Alsop, Ramezani, Frazer, Burns and Holdsworth2020). The most widely accepted model involves the closure of the Iapetus ocean along a suture zone beneath the Southern Uplands, or buried beneath the Solway Firth, originally defined on faunal (mainly trilobites) differences. The precise location of the suture zone in Scotland is poorly known owing to the covering of later younger sedimentary rocks. The Southern Uplands contains fossils of the Pacific faunal province, whereas the Lake District and northern England contain fossils of the Atlantic faunal province (McKerrow & Cocks, Reference McKerrow and Cocks1976; Cocks & Fortey, Reference Cocks and Fortey1982). Deep seismic reflection profiling by the British Institutions Reflection Profiling Syndicate (BIRPS), attempting to locate the suture beneath the North Sea, revealed only a poorly defined NNW-dipping fabric in the upper 30 km of crust, but no indication of any continuity into the mantle, and little correlation between reflectivity and crustal age either side (Klemperer & Matthews, Reference Klemperer and Matthews1987). In contrast, receiver function analyses from East Greenland, the northern continuation of the Scottish Caledonides, show an E-dipping high-velocity slab, interpreted as a relict subduction zone (Schiffer et al. Reference Schiffer, Balling, Jacobsen, Stephenson and Nielsen2014).
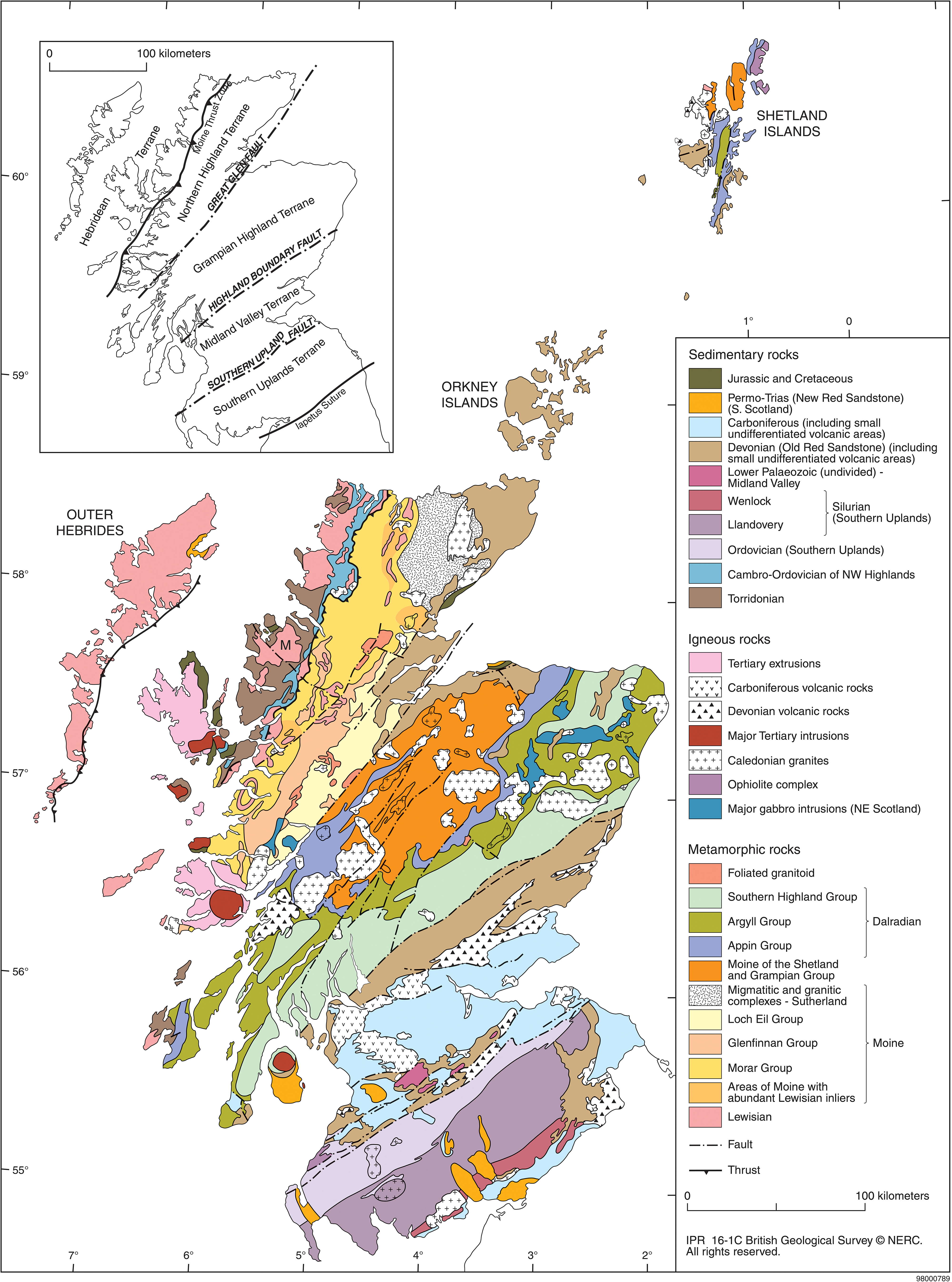
Fig. 1. Simplified geological map of Scotland showing major terranes and major structures, after the British Geological Survey, the Scottish Geology Trust, NERC and Leslie et al. (Reference Leslie, Smith, Soper, Higgins, Gilotti and Smith2008). Inset shows the major terranes of Scotland, after Strachan et al. (Reference Strachan, Harris, Fettes, Smith and Trewin2002). Reproduced with permission, Permit Number CP21/052: British Geological Survey © Material UKRI. All Rights Reserved, original source: Leslie et al. Reference Leslie, Smith, Soper, Higgins, Gilotti and Smith2008, p. 310.
Two major orogenic events have been previously defined in the Scottish Caledonides, firstly the Grampian orogeny (c. 475–465 Ma), and secondly the Scandian orogeny (c. 437–415 Ma). The boundary between the Northern Highlands terrane and the Grampian terrane is the Great Glen strike-slip fault (GGF), which was initially studied in detail by Kennedy (Reference Kennedy1946) and later thought to be cumulatively responsible for 500–700 km of sinistral offset (Stewart et al. Reference Stewart, Strachan and Holdsworth1999; Dewey & Strachan, Reference Dewey and Strachan2003; Dewey et al. Reference Dewey, Dalziel, Reavy and Strachan2015). Dewey & Strachan (Reference Dewey and Strachan2003) proposed as much as 1200 km of cumulative displacement along the sinistral strike-slip faults, including the Southern Uplands, Highland Boundary and Great Glen faults, during Silurian–Devonian times. With this large amount of offset along the GGF, the Northern Highlands and Grampian terranes must presumably have been two different, widely separated terranes with disparate evolutionary histories, brought together by late Caledonian slip on the GGF. However, there is no direct evidence for these large offsets along the GGF, which does not connect north directly to the Walls Boundary fault (WBF) in the Shetland Islands and cannot be traced north of the Orkney Islands on seismic evidence (Chadwick & Evans, Reference Chadwick, Evans and Boldy1995). Rayleigh wave velocity maps across the British Isles using ambient noise tomography also show no differences across the GGF, but they do show a major difference across the Highland Boundary fault (HBF), defining the Midland Valley as a major terrane boundary (Nicolson et al. Reference Nicolson, Curtis and Baptie2014). (Note: A ‘terrane’ is defined as a geologically exotic, fault-bounded region with a distinctive stratigraphy, structure and geological history in comparison to adjacent regions. A ‘terrain’ is a geographically defined region, not disconnected from neighbouring regions, and therefore geologically part of the same ‘plate’ as neighbouring regions.)
Mesozoic–Cenozoic rifting of the North Sea has separated the Caledonian geology of Scandinavia from the UK, and Atlantic rifting has separated Caledonian geology of the North American Appalachians and Greenland from Norway, Great Britain and Ireland. A poorly known buried suture along the Tornquist line separates the Baltic shield area in Scandinavia from the UK and central-southern Europe (Torsvik & Rehnström, Reference Torsvik and Rehnström2003). Detailed geological mapping over the last ∼120 years in the Scottish and Irish Caledonides has delineated the major terranes, stratigraphy and structure (Fig. 1), but debate continues concerning key tectonic elements and events. In northern Scotland, two major metamorphic terranes are the Moine Supergroup in the Northern Highlands terrane, bounded by the Moine thrust zone in the WNW and the GGF in the SE, and the Dalradian Supergroup in the Grampian terrane, bounded by the GGF to the NW and the HBF in the south (Fig. 1). The Midland Valley and Southern Uplands terranes are thought to be the location of the Iapetus suture zone, showing highly disrupted ophiolites (Highland Border ophiolites), a ?Cambrian–Ordovician island arc (Midland Valley arc), deep-sea sediments that were deposited in the intervening Iapetus ocean, as well as post-orogenic Devonian–Carboniferous continental molasse-type sedimentary and volcanic rocks (Stone, Reference Stone2014). The Midland Valley terrane, SE of the HBF, is dominantly composed of post-Caledonian Devonian–Carboniferous sandstones, volcanic and continental sedimentary rocks that could be interpreted as having formed in a molasse basin (Cameron & Stephenson, Reference Cameron and Stephenson1985). The basement of the Midland Valley terrane is not known, but U–Pb dating of felsic xenoliths have recently allowed interpretation of it being part of an Ordovician felsic arc terrane (Badenszki et al. Reference Badenszki, Daly, Whitehouse, Kronz, Upton and Horstwood2019). Dewey (Reference Dewey1971), McKerrow et al. (Reference McKerrow, Leggett and Eales1977, Reference McKerrow, MacNiocaill and Dewey2000) and Leggett et al. (Reference Leggett, McKerrow and Eales1979, Reference Leggett, McKerrow and Soper1983) interpreted the imbricated sedimentary rocks in the Southern Uplands terrane as a Middle Ordovician to a late Silurian accretionary prism with SE-vergent thrust faults related to NW-directed subduction. Sedimentary structures show a derivation from the Laurentian continent to the NW (Waldron et al. Reference Waldron, Floyd, Simonetti and Hearman2008). Younging direction is consistently to the north, but the thrust packages of turbidites become younger towards the south (Leggett et al. Reference Leggett, McKerrow and Eales1979, Reference Leggett, McKerrow and Soper1983; Dewey et al. Reference Dewey, Dalziel, Reavy and Strachan2015).
Metamorphism in the Scottish Highlands has been traditionally classified into (a) the regional medium-pressure–high-temperature (MP–HT) Barrovian facies (garnet–staurolite–kyanite–sillimanite) series, present across the Northern Highlands and Grampian terranes, and (b) the localized low-pressure–high-temperature (LP–HT) Buchan (andalusite–cordierite–sillimanite) series, spatially restricted to the NE Grampian region, and spatially coincident with a series of gabbroic intrusions (Barrow, Reference Barrow1893, Reference Barrow1912; Read, Reference Read1955; Fettes et al. Reference Fettes, Graham, Sassi and Scolari1976; Fettes, Reference Fettes, Harris, Holland and Leake1979; Johnson et al. Reference Johnson, Kirkland, Reddy, Evans and McDonald2016). Metamorphism results from the increase of temperature and pressure due to crustal thickening, with some heat generated from igneous intrusions or from frictional heating along faults. Heat sources for regional Barrovian-type metamorphism in mountain belts have generally been thought to have been a result of internal radiogenic heating and thermal relaxation following crustal thickening (e.g. England & Thompson, Reference England and Thompson1984). However, some authors believe that the Grampian metamorphism was only of short duration, related to either ophiolite obduction (<12 m.y.; Dewey, Reference Dewey2005) or to lithospheric extension (Viete et al. Reference Viete, Richards, Lister, Oliver, Banks, Law, Butler, Holdsworth, Krabbendam and Strachan2010, Reference Viete, Oliver, Fraser, Forster and Lister2013; Viete & Lister, Reference Viete and Lister2016). With more geochronology, especially U–Pb monazite and Sm–Nd and Lu–Hf garnet ages that can be linked to mineral growth at thermal peak, and therefore dating the timing of peak metamorphism, the previously interpreted narrow time span of metamorphism is increasing (e.g. Kinny et al. Reference Kinny, Friend, Strachan, Watt and Burns1999; Baxter et al. Reference Baxter, Ague and DePaolo2002; Bird et al. Reference Bird, Thirlwall, Strachan and Manning2013; Mako et al. Reference Mako, Law, Caddick, Thigpen, Ashley, Cottle and Kylander-Clark2019, Reference Mako, Law, Caddick, Kylander-Clark, Thigpen, Ashley, Mazza and Cottle2021). Although metamorphic isograds generally show an increase of pressure–temperature (P–T) conditions with structural depth, inverted metamorphism can be attained either through underthrusting of cold rocks below hot rocks, as in ophiolite metamorphic soles, or through post-metamorphic folding of locked-in isograds, as mapped along the Greater Himalaya (e.g. Searle & Rex, Reference Searle and Rex1989; Searle, Reference Searle and Watts2015). Intrusion of syn-metamorphic plutonic rocks, either as intrusive gabbros, as in the Buchan metamorphism of Aberdeenshire (Read, Reference Read1923, Reference Read1955; Tanner, Reference Tanner, Corfu, Gasser and Chew2014), or as sillimanite-grade migmatites and leucogranites, as in the Himalaya (Searle et al. Reference Searle, Yeh, Lin and Chung2010 b; Searle, Reference Searle and Watts2015), can bring hot, lower crustal rocks towards the surface through a combination of compressional shearing beneath a low-angle normal fault, and erosion. Metamorphism in the Dalradian appears to be generally right ‘way-up’ with higher temperature sillimanite-grade assemblages at deeper structural levels. Stephenson et al. (Reference Stephenson, Mendum, Fettes and Leslie2013a,b) showed that the major fold nappes were post-peak metamorphism. The metamorphic sequence in the Northern Highlands Moine sequence is structurally inverted with higher grade sillimanite-bearing migmatites of the W-vergent Naver and Sgurr Beag thrust sheets emplaced over lower grade lithologies (Ashley et al. Reference Ashley, Thigpen and Law2015; Mako et al. Reference Mako, Law, Caddick, Thigpen, Ashley, Cottle and Kylander-Clark2019).
Although there are undoubtedly many major structural, metamorphic and magmatic events related to earlier Precambrian events (e.g. Vance et al. Reference Vance, Strachan and Jones1998; Cawood et al. Reference Cawood, Nemchin, Strachan, Kinny and Loewy2004, Reference Cawood, Strachan, Cutts, Kinny, Hand and Pisarevsky2010, Reference Cawood, Strachan, Merle, Millar, Loewy, Dalziel, Kinny, Jourdan, Nemchin and Connelly2015; Mako et al. Reference Mako, Law, Caddick, Kylander-Clark, Thigpen, Ashley, Mazza and Cottle2021), this paper is concerned only with the early Palaeozoic Caledonian orogeny in Scotland. It does not include reviews or analyses of the geology of Ireland or Greenland, or the evolution of the Norway Caledonides, which, although very important, lie outside the scope of this paper. Major questions concerning the precise tectonic evolution of the Scottish Caledonides remain. These include the following:
-
(1) Were the Moine and Dalradian supergroups deposited on the same continental plate, or are they two different terranes, brought together by subsequent strike-slip faulting?
-
(2) What is the nature of the Iapetus suture zone, and where is it?
-
(3) Was the GGF a major terrane boundary, with >500–700 km sinistral offset, active during the latter part of the Caledonian orogeny?
-
(4) Were the Grampian and Scandian metamorphic events two separate, short-lived, orogenic events, or part of one long-lived orogeny?
-
(5) Was LP–HT Buchan and MP–HT Barrovian metamorphism in the Dalradian related to crustal thickening or to lithospheric-scale crustal extension and crust and mantle thinning?
-
(6) What is the tectonic origin and significance of the Silurian–Devonian (c. 435–405 Ma) ‘Newer Granites’ across Scotland?
-
(7) Was there a flip in subduction direction from SE-dipping in Ordovician time to NW-dipping in Silurian time?
-
(8) Was the Scandian event in Scotland related to collision of Laurentia with Baltica, or was it part of the greater Laurentia – Midland Valley arc/microcontinent – Avalonia continental collision?
This paper discusses these major tectonic questions after first briefly outlining the geology of each terrane. Extensive use has been made of numerous excellent summaries, reviews and books, most notable those of Peach & Horne (Reference Peach and Horne1884), Peach et al. (Reference Peach, Horne, Gunn, Clough, Hinxman and Cadell1888, Reference Peach, Horne, Gunn, Clough and Hinxman1907), Harris et al. (Reference Harris, Bradbury and McGonigal1976), Stephenson et al. (Reference Stephenson, Bevins, Millward, Highton, Parsons, Stone and Wadworth1999, Reference Stephenson, Mendum, Fettes and Leslie2013 a), Trewin (Reference Trewin2002), Leslie et al. (Reference Leslie, Smith, Soper, Higgins, Gilotti and Smith2008), Mendum et al. (Reference Mendum, Barber, Butler, Flinn, Goodenough, Krabbendam, Park and Stewart2009), Law et al. (Reference Law, Johnson, Law, Butler, Holdsworth, Krabbendam and Strachan2010), Strachan et al. (Reference Strachan, Holdsworth, Krabbendam, Alsop, Law, Butler, Holdsworth, Krabbendam and Strachan2010), Law & Johnson (Reference Law, Johnson, Law, Butler, Holdsworth, Krabbendam and Strachan2010), Goodenough et al. (Reference Goodenough, Millar, Strachan, Krabbendam and Evans2011), Woodcock & Strachan (Reference Woodcock and Strachan2012), Chew & Strachan (Reference Chew, Strachan, Corfu, Gasser and Chew2014), Tanner (Reference Tanner, Corfu, Gasser and Chew2014), Stone (Reference Stone2014) and Dewey et al. (Reference Dewey, Dalziel, Reavy and Strachan2015). For detailed geological reports of key areas the reader is referred to these publications. Figure 2 shows the widely accepted two-stage orogenic model involving Neoproterozoic to Early Ordovician SE-dipping subduction followed by a flip to NE-dipping subduction in Late Ordovician – Silurian times, after Leslie et al. (Reference Leslie, Smith, Soper, Higgins, Gilotti and Smith2008). This paper proposes an alternative, simpler tectonic solution to explain the Caledonian orogeny in Scotland based on a review of regional mapping, stratigraphic, structural, metamorphic, magmatic and geochronological data across northern Scotland. This model involves continuous SE-directed subduction, Cambrian NW-directed ophiolite obduction and collision of the Laurentian margin with the Midland Valley arc–microcontinent, without involvement of Baltica. Figure 3 is a pre-collision reconstruction (to ∼470 Ma) of the Laurentian plate foreland across the Northern Highlands (Moine Supergroup) and Grampian Highlands (Dalradian Supergroup) terranes to the Midland Valley and Southern Uplands suture zone, showing the age ranges of protolith rocks in each terrane. The gaps are locations of the major late or post-orogenic, mainly strike-slip, faults that bound the metamorphic terranes, notably the GGF and the HBF. Figure 4 shows a lower Palaeozoic time chart showing age ranges of lithostratigraphic units and radiometric dates of metamorphic and magmatic rocks across each terrane. The chronology of events shown on Figure 4 points to a simpler orogenic cycle where the compressional, ocean closure stage begins with ophiolite obduction (∼499–475 Ma) onto a previous passive continental margin, and accretion of the Midland Valley arc–microcontinent, crustal shortening, thickening and regional metamorphism (∼470–420 Ma) related to terrane accretion and continental collision to the end of the Caledonian orogeny with regional deposition of post-collision molasse deposits (Old Red Sandstone), from ∼405 to 400 Ma.
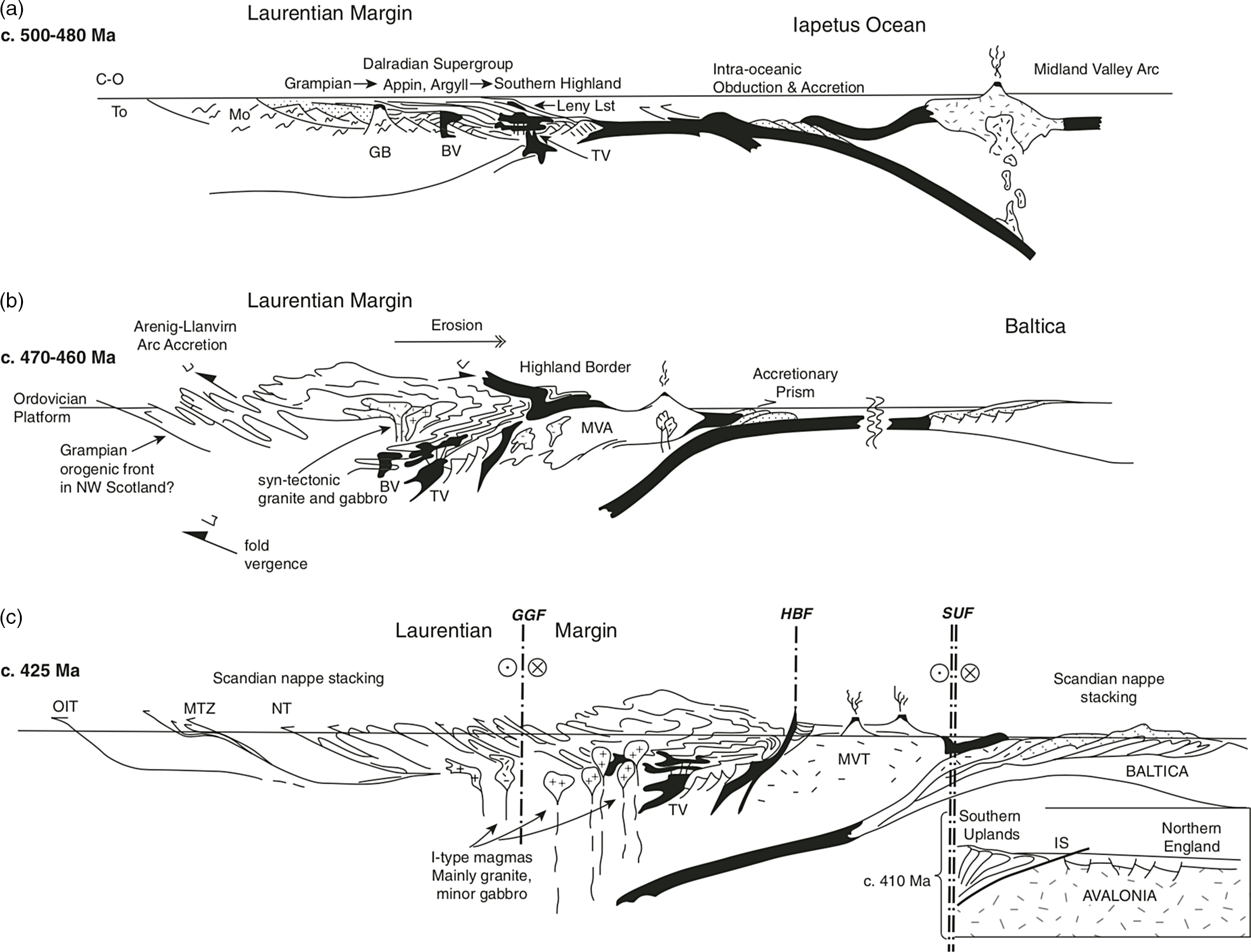
Fig. 2. Generally accepted model for the Neoproterozoic to Silurian evolution of the Scottish Caledonides, from Strachan et al. (Reference Strachan, Harris, Fettes, Smith and Trewin2002), Leslie et al. (Reference Leslie, Smith, Soper, Higgins, Gilotti and Smith2008) and Dunk et al. (Reference Dunk, Strachan, Cutts, Lasalle, Storey, Burns, Whitehouse, Fowler, Moreira, Dunlop and Pereira2019), based on a flip of subduction polarity from Ordovician SE-dipping to Silurian NW-dipping; after Dewey (Reference Dewey1969), Dewey & Shackleton (Reference Dewey and Shackleton1984), Stephenson et al. (Reference Stephenson, Mendum, Fettes, Smith, Gould, Tanner and Smith2013b) and several others. (a) Cambrian restoration showing Dalradian sedimentary rocks on the Laurentian passive margin, NW-vergent oceanic thrust sheets associated with ophiolite obduction and SE-dipping subduction beneath the Midland Valley arc (MVA). (b) Ordovician ‘Grampian phase’ involving a flip in subduction direction to NW-dipping beneath the Laurentian margin. Ordovician crustal thickening, folding and thrusting of the Laurentian margin simultaneously with oceanic subduction and accretionary prism formation in the Southern Uplands. BV – Ben Vuirich granite; TV – Tayvallich volcanics. (c) Silurian ‘Scandian phase’ involving NW subduction of Baltica beneath the Midland Valley terrane (MVT) and the Laurentian plate with NW-vergent nappe stacking across the Northern Highlands and Grampian Highlands terranes. Positions of the late strike-slip faults are shown, the Great Glen fault (GGF), Highland Boundary fault (HBF) and the Southern Uplands Fault (SUF). OIT – Outer Isles thrust

Fig. 3. Model showing the restoration of the Laurentian plate passive continental margin to c. 475 Ma, showing the range and ages of rock types in the stable foreland, Moine thrust zone, Northern Highlands terrane (Moine Supergroup), Grampian Highlands terrane (Dalradian Supergroup) and the allochthonous Highland Border – Midland Valley arc terranes. Timing of igneous rocks are in red, metamorphic rocks in purple. See text for sources of data.

Fig. 4. Lower Palaeozoic time chart for the Scottish Caledonides showing age ranges of marine sediments (blue), ophiolites (green), peak or near-peak metamorphism (mauve), alkaline syenite intrusive rocks (orange) and granites (red). Crosses are I-type granites; plain red crosses are S-type granites. Post-Caledonian continental sedimentary rocks (Old Red Sandstone) are yellow. See text for sources of data. Ages are based on the International Chronostratigraphic Chart 2016/04.
2. Laurentian foreland sequence
The Hebridean terrane west of the Moine thrust zone comprises the stable foreland of the Laurentian plate and is continuous across to Greenland and North America. The foreland in NW Scotland comprises Archaean (Scourian complex) and Palaeoproterozoic (Laxfordian complex) basement gneisses of the Lewisian gneiss complex, separated in space and time by a major swarm of intrusive basic dykes, the Scourie dykes (Sutton & Watson, Reference Sutton and Watson1951; Park & Tarney, Reference Park, Tarney, Park and Tarney1987; Park et al. Reference Park, Stewart, Wright and Trewin2002; Wheeler et al. Reference Wheeler, Park, Rollinson, Beach, Law, Butler, Holdsworth, Krabbendam and Strachan2010). Grey tonalitic gneisses, with trondhjemites and granodiorites (tonalite–trondhjemite–granodiorite suite), mafic gneisses and ultramafic enclaves show a dominant granulite-facies metamorphism during Scourian metamorphism, exposed in the central part of the Lewisian of NW Scotland. P–T conditions range from 8 to 12 kbar and 800 to 1000 °C, and timing constraints are ∼3.0–2.7 Ga (Cartwright & Barnicoat, Reference Cartwright, Barnicoat, Park and Tarney1987; Whitehouse, Reference Whitehouse1988, Reference Whitehouse1989; Corfu et al. Reference Corfu, Heaman and Rogers1994). A major phase of basic dyke intrusion (Scourie dykes) with compositions ranging from orthopyroxene-bearing picrite, norite and olivine gabbro to quartz dolerite, dated at 2.4–2.0 Ga, generally striking WNW–ESE, intrudes the Archaean granulites. To the north and south of the Scourian terrane, the Archaean gneisses and the Scourie dykes have been overprinted by a Palaeoproterozoic amphibolite-facies metamorphism during the Laxfordian event at 1.8–1.75 Ga (Kinny & Friend, Reference Kinny and Friend1997; Corfu et al. Reference Corfu, Crane, Moder and Rogers1998). Characteristic K-feldspar-rich granite–pegmatite sheets dated at 1.85–1.7 Ga cut the Laxfordian gneisses (Friend & Kinny, Reference Friend and Kinny2001). Major sub-vertical ductile shear zones bound the central Scourian granulite terrane to the north (Laxford shear zone) and south (Canisp shear zone). These shear zones are not terrane boundaries or suture zones separating different plates, but instead represent either metamorphic/fluid infiltration fronts or different crustal levels (lower crust Scourian granulites; middle crust Laxfordian amphibolites) during progressive collision-related Palaeoproterozoic Laxfordian orogenesis.
The Lewisian basement is overlain unconformably by a thick sequence of fluvial, lacustrine and red-bed continental conglomerates, sandstones and siltstones, mainly derived from erosion of high mountain topography dominantly in the west. These include the Stoer and Sleat Groups, which fill in a highly irregular Lewisian topography. Both are unconformably overlain by the Torridon Group, which is up to 6 km thick (Stewart, Reference Stewart2002) and is well exposed on many of the high peaks of NW Scotland, including Suilven, Canisp and Quinag. A series of Precambrian E-dipping normal faults bound several half-grabens. The Torridonian sandstones were either deposited in these half-grabens, or more likely, the faulting occurred after Torridonian sedimentation and prior to the overlying Cambrian (Bonsor et al. Reference Bonsor, Strachan, Prave and Krabbendam2012). These Precambrian normal faults have up to 5 km of throw, with the unconformably overlying Cambrian basal quartzites deposited above thick Torridonian in the half-grabens, and in places deposited directly on Lewisian basement gneisses (Searle et al. Reference Searle, Cornish, Heard, Charles and Branch2019).
A regional unconformity marks the base of the Cambrian quartzites (Eriboll Formation sandstones and quartzites), which are interpreted as marking shallow marine deposition on the stable passive continental margin of Laurentia during the spreading of the Iapetus ocean (Woodcock & Strachan, Reference Woodcock and Strachan2012). The 150–200 m thick quartzites show extensive cross-bedding indicating easterly flow directions, and contain characteristic trace fossils, Skolithos ‘pipes’ or worm burrows. The quartzites are overlain by thin pale brown siltstones (Fucoid beds) and sandstones (Salterella grits), both showing abundant trace fossils. A major regional unconformity above the Cambrian strata marks the base of the Early Ordovician Durness Group limestone–dolomite marine transgression. The ending of shallow marine sedimentation in the Durness Group is coincident with the beginning of ophiolite obduction and accretion of the Midland Valley arc terrane, and subsequent collision-related uplift, crustal thickening and metamorphism in the Moine and Dalradian hinterland (Fig. 4).
3. Moine thrust zone
The Moine thrust zone in the Assynt region comprises at least four major W-vergent thrust sheets, which, from hinterland to foreland include the earliest and deepest Moine thrust, further down-section to the Ben More and Glencoul thrusts, and finally to the Sole thrust (Elliott & Johnson, Reference Elliott and Johnson1980; Butler, Reference Butler, Law, Butler, Holdsworth, Krabbendam and Strachan2010; Searle et al. Reference Searle, Law, Dewey, Streule, Law, Butler, Holdsworth, Krabbendam and Strachan2010 a). All rock units of the foreland occur within the Moine thrust zone including, in the Assynt area, a suite of highly alkaline igneous intrusions ranging from alkaline ultramafic rocks through pseudoleucite (nepheline + muscovite + K-feldspar) + melanite garnet borralanite, to nordmarkite and quartz syenite (Parsons, Reference Parsons1965, Reference Parsons1972; Goodenough et al. Reference Goodenough, Young and Parsons2004, Reference Goodenough, Evans and Krabbendam2006; Searle et al. Reference Searle, Law, Dewey, Streule, Law, Butler, Holdsworth, Krabbendam and Strachan2010 a). The Loch Borralan and Loch Ailsh syenite intrusions have ages between ∼431 and 429 Ma (Goodenough et al. Reference Goodenough, Millar, Strachan, Krabbendam and Evans2011), and are cut by thrusts above and below, providing a maximum age of initiation of the Moine thrust (Searle et al. Reference Searle, Law, Dewey, Streule, Law, Butler, Holdsworth, Krabbendam and Strachan2010 a; Fox & Searle, Reference Fox and Searle2021). One nordmarkite dyke cuts across mylonitic fabrics along the Moine thrust (Law et al. Reference Law, Casey and Knipe1986; Law, Reference Law1987, Reference Law, Snoke, Tullis and Todd1998, Reference Law2014; Thigpen et al. Reference Thigpen, Law, Lloyd and Brown2010 a, Reference Thigpen, Law, Loehn, Strachan, Tracy, Lloyd, Roth and Brown2013), but none appear to intrude the un-mylonitized Moine schists above the Moine thrust zone. Two large composite alkaline syenites do intrude the Moine Supergroup structurally above the Moine thrust. The Loch Loyal syenite in the far north, east of Loch Eriboll, has a poorly constrained single grain 206Pb–238U zircon age of c. 425 Ma, and the Glenn Dessary syenite in the southern part of the Northern Highlands terrane, east of Knoydart, has a mean 206Pb–238U age of 445.7 ± 8 Ma and a concordia age of 445.3 ± 1.9 Ma (Goodenough et al. Reference Goodenough, Millar, Strachan, Krabbendam and Evans2011).
The Glencoul and Ben More thrusts, structurally beneath the Moine thrust, bring up slices of Lewisian basement as well as imbricated Cambrian–Ordovician sedimentary strata. The structural geometry suggests a foreland-propagating thrust system from the Moine thrust down-structural section to the Arnaboll thrust (Loch Eriboll), and Ben More, Glencoul, Borralan and Sole thrusts (Assynt) with time (Elliott & Johnson, Reference Elliott and Johnson1980; Coward, Reference Coward1983, Reference Coward1984; Butler, Reference Butler, Law, Butler, Holdsworth, Krabbendam and Strachan2010; Searle et al. (Reference Searle, Law, Dewey, Streule, Law, Butler, Holdsworth, Krabbendam and Strachan2010 a, Reference Searle, Cornish, Heard, Charles and Branch2019). The Moine thrust wraps around the Assynt culmination preserving deeper structural units in the culmination, which enables construction of balanced cross-sections across the entire Moine thrust zone (Elliott & Johnson, Reference Elliott and Johnson1980; Searle et al. Reference Searle, Law, Dewey, Streule, Law, Butler, Holdsworth, Krabbendam and Strachan2010 a; Fox & Searle, Reference Fox and Searle2021). The Moine thrust truncates underlying imbricate structures along the southern part of the Assynt Window (Peach et al. Reference Peach, Horne, Gunn, Clough, Hinxman and Cadell1888; Coward, Reference Coward1983) and rests almost directly on the undisturbed foreland in the south suggesting some late-stage out-of-sequence displacement. The Assynt culmination has been bulged up by deep thrusting exhuming several thrust sheets with Lewisian basement (e.g. Ben More, Glencoul thrusts). Total amounts of shortening across the Moine thrust zone are at least 70–80 km (Elliott & Johnson, Reference Elliott and Johnson1980; Coward, Reference Coward1984, Reference Coward1985; Searle et al. Reference Searle, Law, Dewey, Streule, Law, Butler, Holdsworth, Krabbendam and Strachan2010 a). A maximum age of shearing is given by the 431–429 Ma intrusion ages from the Loch Borralan syenite (Goodenough et al. Reference Goodenough, Millar, Strachan, Krabbendam and Evans2011), and a minimum age on shearing is given by 40Ar–39Ar cooling ages on the mylonites of 430–420 Ma (Johnson et al. Reference Johnson, Kelley, Oliver and Winter1985; Kelley, Reference Kelley1988; Dallmeyer et al. Reference Dallmeyer, Strachan, Rogers, Watt and Friend2001).
4. Northern Highlands terrane (Moine Supergroup)
The Northern Highlands terrane, bounded by the Moine thrust zone in the west and the GGF in the SE, is comprised of rocks of the Moine Supergroup, a thick sequence of Neoproterozoic psammitic and semi-pelitic sedimentary rocks intruded by granites and affected by several phases of deformation and metamorphism (Peach et al. Reference Peach, Horne, Gunn, Clough and Hinxman1907; Ramsay, Reference Ramsay1958; Holdsworth, Reference Holdsworth1990; Friend et al. Reference Friend, Strachan, Kinny and Watt2003; Holdsworth et al. Reference Holdsworth, Alsop, Strachan, Ries, Butler and Graham2007; Strachan et al. Reference Strachan, Holdsworth, Krabbendam, Alsop, Law, Butler, Holdsworth, Krabbendam and Strachan2010; Cawood et al. Reference Cawood, Strachan, Merle, Millar, Loewy, Dalziel, Kinny, Jourdan, Nemchin and Connelly2015). Figure 5 shows a metamorphic map of northern Scotland showing the distribution of the Barrovian and Buchan facies metamorphic rocks, granites, syenites and gabbros (after Winchester, Reference Winchester1974; Fettes et al. Reference Fettes, Graham, Sassi and Scolari1976; Fettes, Reference Fettes, Harris, Holland and Leake1979; Tanner, Reference Tanner, Corfu, Gasser and Chew2014). Several inliers of Archaean Lewisian complex gneiss underlie the Moine metasedimentary rocks. Detrital zircon ages from the Moine Supergroup constrain deposition to <1000 Ma (Strachan et al. Reference Strachan, Harris, Fettes, Smith and Trewin2002; Cawood et al. Reference Cawood, Nemchin, Strachan, Kinny and Loewy2004, Reference Cawood, Strachan, Merle, Millar, Loewy, Dalziel, Kinny, Jourdan, Nemchin and Connelly2015), suggesting that the Moine rocks could be time-equivalent to the unmetamorphosed Sleat and Torridon groups in the foreland (Geikie, Reference Geikie1884, Reference Geikie1893; Soper et al. Reference Soper, Harris and Strachan1998; Stewart, Reference Stewart2002; Krabbendam et al. Reference Krabbendam, Prave and Cheer2008). Details of the Precambrian stratigraphy of the Northern Highlands and Grampian terranes are given in Holdsworth et al. (Reference Holdsworth, Strachan, Harris, Gibbons and Harris1994, Reference Holdsworth, Alsop, Strachan, Ries, Butler and Graham2007), Strachan et al. (Reference Strachan, Harris, Fettes, Smith and Trewin2002), Leslie et al. (Reference Leslie, Smith, Soper, Higgins, Gilotti and Smith2008), Chew et al. (Reference Chew, Daly, Magna, Page, Kirkland, Whitehouse and Lam2010) and Chew & Strachan (Reference Chew, Strachan, Corfu, Gasser and Chew2014). Moine sedimentary rocks have been stratigraphically subdivided into three main groups, the oldest Morar Group overlain by the Glenfinnan Group, and the youngest Loch Eil Group. The sedimentary rocks are all shallow marine deposits formed in graben systems, and in general becoming more distal towards the east, consistent with sedimentation on a passive continental margin during Iapetus ocean spreading. Soper et al. (Reference Soper, Harris and Strachan1998) mapped two major half-grabens with E-dipping normal faults, similar to some of the Torridon Group half-grabens on the Lewisian foreland. Detrital zircon ages suggest that the Morar Group was deposited after c. 1000–980 Ma and the Glenfinnan and Loch Eil sediments were deposited after 900–870 Ma (Cawood et al. Reference Cawood, Strachan, Merle, Millar, Loewy, Dalziel, Kinny, Jourdan, Nemchin and Connelly2015). There are no young (Cambrian – Lower Ordovician) protoliths recorded in the Moine Supergroup, as seen in the Dalradian, probably because the Moine rocks were situated in a more hinterland position relative to the more continental margin setting of the Dalradian and Highland Border complex. The youngest Loch Eil Group is intruded by the 870 Ma West Highland granitic gneiss, showing that the Moine sedimentation was older than development of the Iapetus passive margin.
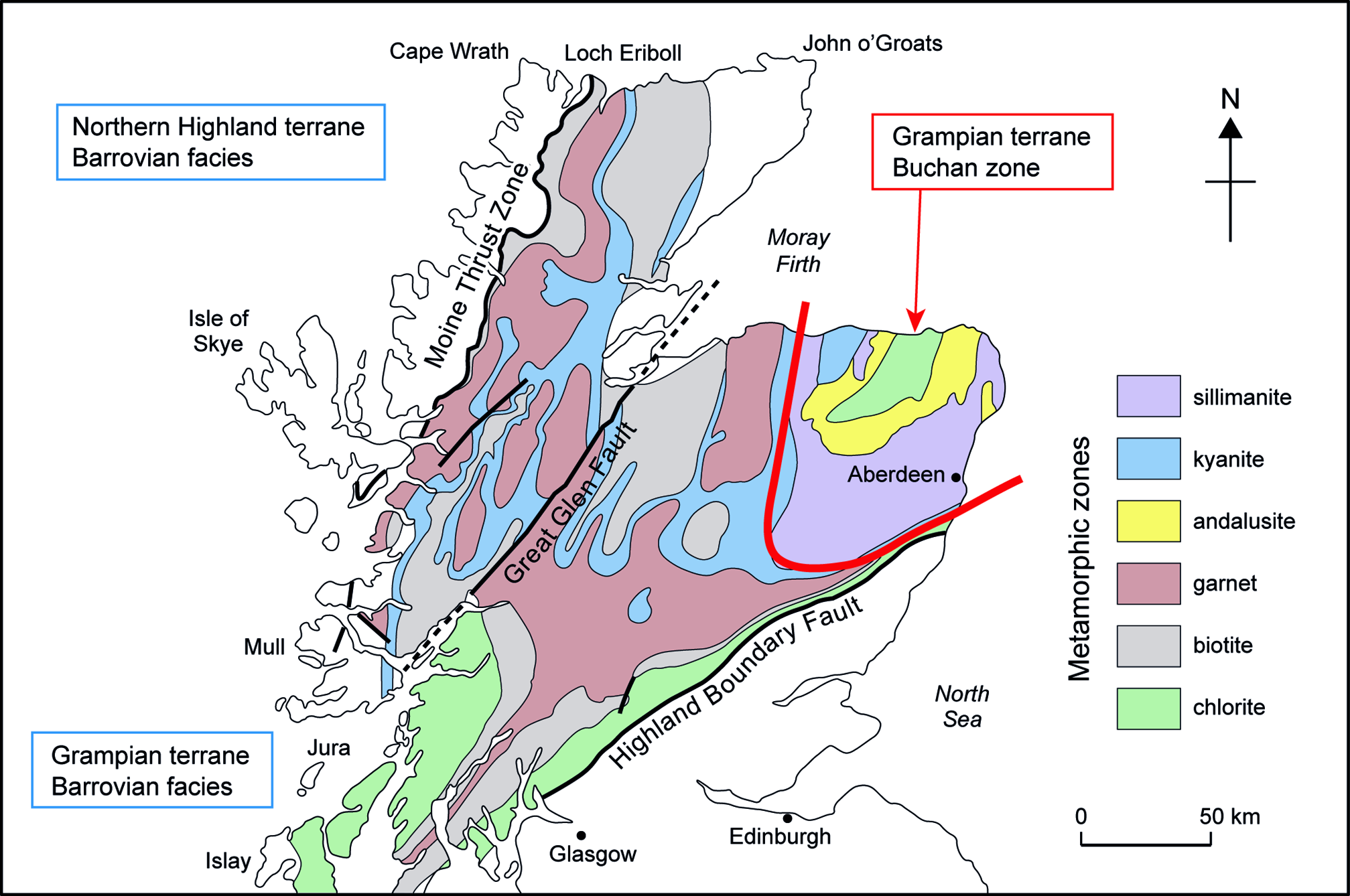
Fig. 5. Metamorphic map of northern Scotland, after Winchester (Reference Winchester1974) and Gillen (Reference Gillen1982), showing facies across the Northern Highlands and Grampian terranes, after Fettes (Reference Fettes1970, Reference Fettes, Harris, Holland and Leake1979), Harte & Hudson (Reference Harte, Hudson, Harris, Holland and Leake1979), Stephenson et al. (Reference Stephenson, Mendum, Fettes and Leslie2013a,b) and Tanner (Reference Tanner, Corfu, Gasser and Chew2014) for the Dalradian, and Thigpen et al. (Reference Thigpen, Law, Loehn, Strachan, Tracy, Lloyd, Roth and Brown2013), Ashley et al. (Reference Ashley, Thigpen and Law2015) and Mako et al. (Reference Mako, Law, Caddick, Thigpen, Ashley, Cottle and Kylander-Clark2019, Reference Mako, Law, Caddick, Kylander-Clark, Thigpen, Ashley, Mazza and Cottle2021) for the Northern Highlands (Moine) terranes.
A suite of felsic igneous rocks was intruded into the Moine sedimentary rocks at ∼870 Ma, and a possible amphibolite-facies metamorphism and deformation event, referred to as the ‘Knoydartian event’ and bracketed between ∼840 and 790 Ma, has been proposed (Vance et al. Reference Vance, Strachan and Jones1998; Rogers et al. Reference Rogers, Hyslop, Strachan, Paterson and Holdsworth1998; Tanner & Evans, Reference Tanner and Evans2003; Cutts et al. Reference Cutts, Hand, Kelsey and Strachan2009, Reference Cutts, Kinny, Strachan, Hand, Kelsey, Emery, Friend and Leslie2010; Cawood et al. Reference Cawood, Strachan, Merle, Millar, Loewy, Dalziel, Kinny, Jourdan, Nemchin and Connelly2015). Late Proterozoic granites such as the Carn Chuinneag and Inchbae granites dated by U–Pb zircon at 594 ± 11 Ma (Oliver et al. Reference Oliver, Wilde and Wan2008) intrude the Moine sedimentary units. U–Pb zircon ages of 503 ± 2 Ma on trondhjemitic gneisses from the calc-alkaline Strathy complex within the Moine Supergroup have suggested an intra-Moine Cambrian subduction zone (Dunk et al. Reference Dunk, Strachan, Cutts, Lasalle, Storey, Burns, Whitehouse, Fowler, Moreira, Dunlop and Pereira2019). It is also notable that some Moine-like sedimentary rocks are present SE of the GGF (Glen Banchor – Dava groups; Stephenson et al. Reference Stephenson, Mendum, Fettes and Leslie2013a,b; Badenoch Group; Cawood et al. Reference Cawood, Strachan, Merle, Millar, Loewy, Dalziel, Kinny, Jourdan, Nemchin and Connelly2015) in the Central Highlands (Grampian) terrane making the separate origin of the Dalradian and Moine supergroups (e.g. Bluck, Reference Bluck1985) questionable.
Figure 6 shows a structural cross-section across the SW part of the Moine, from the Moine thrust in the west to the GGF in the SE, after Powell & Glendinning (Reference Powell, Glendinning, Allison, May and Strachan1988) and Soper et al. (Reference Soper, Harris and Strachan1998), modified by Strachan et al. (Reference Strachan, Harris, Fettes, Smith and Trewin2002), with a simplified restoration of the major folds only (Fig. 6b). The absolute minimum amount of crustal shortening along this profile is ∼25 km, but in reality it is probably considerably more. In the northern part of the Moine, shortening is much greater with exhumation of high-grade kyanite and sillimanite-grade rocks along the hangingwall of the Naver thrust and Sgurr Beag thrust (Ashley et al. Reference Ashley, Thigpen and Law2015; Mako et al. Reference Mako, Law, Caddick, Thigpen, Ashley, Cottle and Kylander-Clark2019, Reference Mako, Law, Caddick, Kylander-Clark, Thigpen, Ashley, Mazza and Cottle2021). As in all high-grade metamorphic terranes characterized by extensive ductile folding and shearing, it is not possible to balance or restore cross-sections with any accuracy, but a restoration of major folds, shear zones and thrusts can provide an estimate of the minimum amount of crustal shortening.
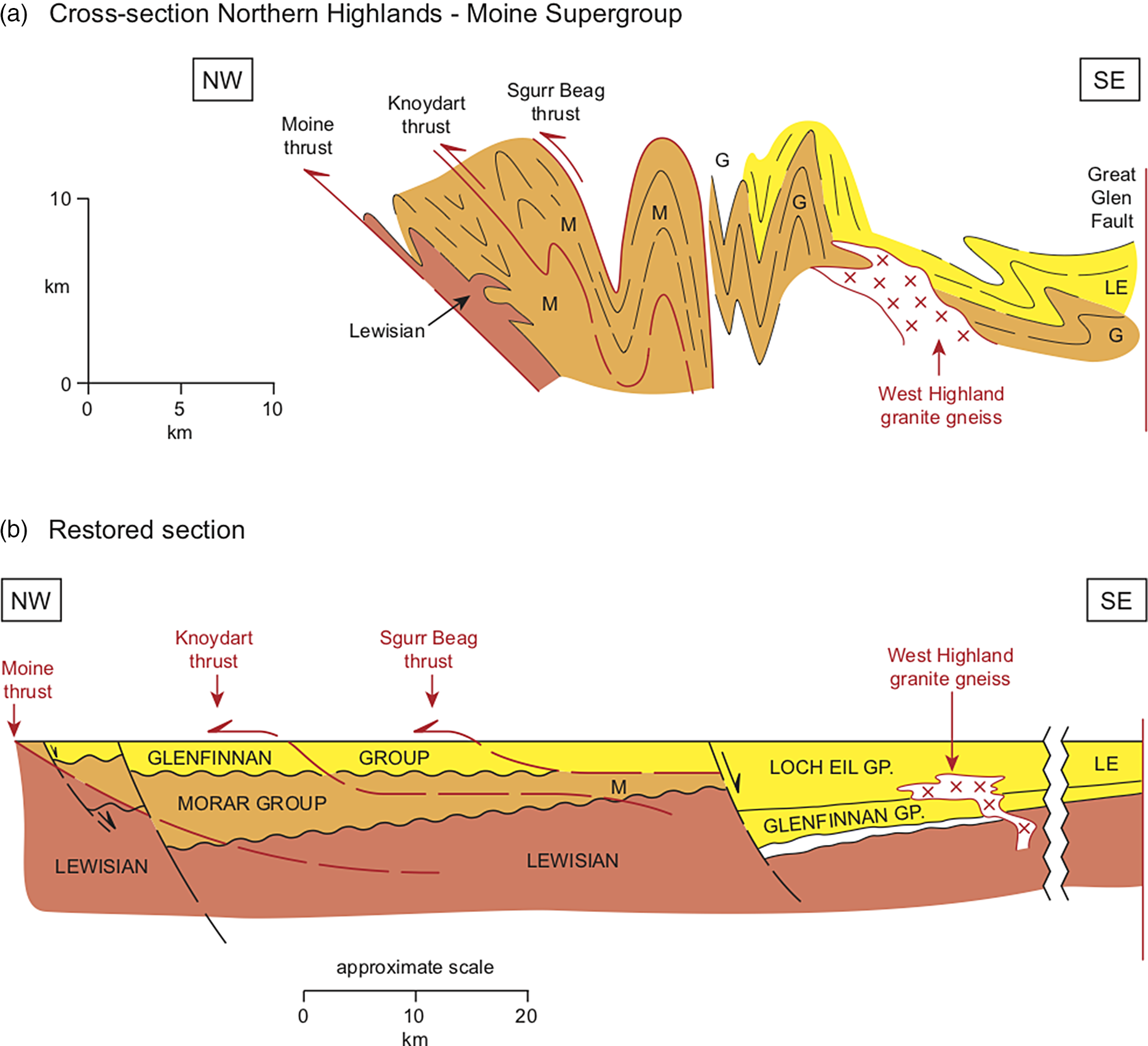
Fig. 6. (a) Simplified cross-section across the Northern Highlands terrane Moine Supergroup, after Powell & Glendinning (Reference Powell, Glendinning, Allison, May and Strachan1988), Soper et al. (Reference Soper, Harris and Strachan1998) and Strachan et al. (Reference Strachan, Harris, Fettes, Smith and Trewin2002). The restoration (b) shows the approximate positions of the Sgurr Beag thrust, Knoydart thrust, and Moine thrust sheets in the Northern Highlands terrane.
The Moine Supergroup sedimentary rocks have been affected by Precambrian (Knoydartian) metamorphism at 820–720 Ma (Vance et al. Reference Vance, Strachan and Jones1998; Kinny et al. Reference Kinny, Friend, Strachan, Watt and Burns1999; Friend et al. Reference Friend, Jones and Burns2000; Cutts et al. Reference Cutts, Hand, Kelsey and Strachan2009, Reference Cutts, Kinny, Strachan, Hand, Kelsey, Emery, Friend and Leslie2010), and by regional Barrovian facies metamorphism during the Ordovician – early Silurian Caledonian orogeny across the Northern Highlands terrane (Mako et al. Reference Mako, Law, Caddick, Kylander-Clark, Thigpen, Ashley, Mazza and Cottle2021). The hangingwall of the Naver thrust in the east consists of sillimanite-bearing migmatites that preserve P–T conditions of c. 8–9 kbar and 600 °C with decompression and heating to conditions of 6–7 kbar and 700 °C (Kinny et al. Reference Kinny, Friend, Strachan, Watt and Burns1999; Friend et al. Reference Friend, Jones and Burns2000; Ashley et al. Reference Ashley, Thigpen and Law2015; Mako et al. Reference Mako, Law, Caddick, Thigpen, Ashley, Cottle and Kylander-Clark2019; Dunk et al. Reference Dunk, Strachan, Cutts, Lasalle, Storey, Burns, Whitehouse, Fowler, Moreira, Dunlop and Pereira2019). In the southern part of the Northern Highlands terrane, the Sgurr Beag thrust may be the southern continuation of the Naver thrust. P–T conditions decrease westwards, and structurally downward, to 4.8–7.2 kbar and ∼550–680 °C in the hangingwall of the Moine thrust (Ashley et al. Reference Ashley, Thigpen and Law2015; Mazza et al. Reference Mazza, Mako, Law, Caddick, Krabbendam and Cottle2018; Mako et al. Reference Mako, Law, Caddick, Thigpen, Ashley, Cottle and Kylander-Clark2019, Reference Mako, Law, Caddick, Kylander-Clark, Thigpen, Ashley, Mazza and Cottle2021). U–Pb monazite and xenotime ages range between 430 and 415 Ma, although two samples are older at 445–440 Ma (Fig. 4; Mako et al. Reference Mako, Law, Caddick, Thigpen, Ashley, Cottle and Kylander-Clark2019). The latter, defined as the ‘Grampian II event’, are similar to Lu–Hf garnet ages in the Moine, Ben Hope and Naver (+ Sgurr Beag) thrust sheets (Bird et al. Reference Bird, Thirlwall, Strachan and Manning2013). Syn-tectonic granite intrusions in the Naver thrust sheet are 430–420 Ma (Fig. 4; Kinny et al. Reference Kinny, Strachan, Friend, Kocks, Rogers and Paterson2003; Strachan et al. Reference Strachan, Alsop, Ramezani, Frazer, Burns and Holdsworth2020), and confirm the presence of high temperatures in the middle and lower crust during this period. These rocks have been affected by ductile shear zones that have emplaced higher grade rocks above lower grade rocks (Ashley et al. Reference Ashley, Thigpen and Law2015). This inverted metamorphism appears to have formed by post-peak metamorphic thrusting (Mazza et al. Reference Mazza, Mako, Law, Caddick, Krabbendam and Cottle2018), rather than by any channel flow type mechanism. The regional structure and metamorphism of the Moine Supergroup is comparable to the Greater Himalayan Sequence metamorphic rocks above the Main Central thrust (Searle et al. Reference Searle, Law, Godin, Larsen, Streule, Cottle and Jessup2008; Streule et al. Reference Streule, Strachan, Searle, Law, Law, Butler, Holdsworth, Krabbendam and Strachan2010). Both regions show extensive staurolite, kyanite and sillimanite-grade metamorphic rocks with sillimanite + K-feldspar migmatites at higher structural levels, and an inverted metamorphic field gradient down-structural section. The Himalaya contain a far greater volume of partial melt migmatites and leucogranites (with garnet, muscovite, tourmaline and cordierite), so the Himalayan channel flow model, lubricated by extensive melting (e.g. Searle et al. Reference Searle, Law, Jessup, Law, Searle and Godin2006, Reference Searle, Law, Dewey, Streule, Law, Butler, Holdsworth, Krabbendam and Strachan2010 a), is unlikely to be applicable to the Moine metamorphism. However, the time scales of metamorphism are similar (lasting ∼45–55 m.y.), in both the Caledonian and Himalayan orogenies.
5. Grampian Highlands terrane (Dalradian Supergroup)
The Grampian terrane is bounded by the GGF in the NW and the Highland Border complex and HBF zone in the south (Bailey, Reference Bailey1922; Stephenson & Gould, Reference Stephenson and Gould1995; Stephenson et al. Reference Stephenson, Mendum, Fettes and Leslie2013a,b). Like the Moine metasedimentary rocks, the lower part of the Dalradian metasedimentary sequence is mostly Precambrian and unfossiliferous. Both the Moine and Dalradian supergroups have similar detrital zircon ages, but the upper part of the Dalradian has fossil evidence that suggest protolith rocks could be as young as Lower Ordovician (Stephenson et al. Reference Stephenson, Mendum, Fettes and Leslie2013a,b; Tanner, Reference Tanner, Corfu, Gasser and Chew2014). Detrital zircon ages from the Dalradian Supergroup show a similar age range as rocks from East Greenland and Newfoundland, confirming a Laurentian plate origin (Cawood et al. Reference Cawood, Nemchin, Smith and Loewy2003, Reference Cawood, Nemchin, Strachan, Prave and Krabbendam2007; McAteer et al. Reference McAteer, Daly, Flowerdew, Whitehouse and Monaghan2014). Five main stratigraphic groups have been defined, the Grampian Group at the base overlain by the Appin, Argyll and Southern Highlands groups, overlain in turn by the partially fossiliferous Trossachs Group, of oceanic affinity, at the top (Cawood et al. Reference Cawood, Strachan, Merle, Millar, Loewy, Dalziel, Kinny, Jourdan, Nemchin and Connelly2015). The latter may be continuous with the Highland Border complex along the southern margin of the Grampian terrane; see Stephenson & Gould (Reference Stephenson and Gould1995), Tanner & Sutherland (Reference Tanner and Sutherland2007), Tanner (Reference Tanner2008, Reference Tanner, Corfu, Gasser and Chew2014) and Stephenson et al. (Reference Stephenson, Mendum, Fettes and Leslie2013a,b) for comprehensive reviews.
Palaeoproterozoic basement units are present in the form of the Rhinns complex syenitic gneisses on Islay. Most clasts within the basal conglomerates of the Iona Group in the Inner Hebrides are Archaean–Palaeoproterozoic gneisses, similar to the Lewisian basement in the foreland and in the Northern Highlands terrane (McAteer et al. Reference McAteer, Daly, Flowerdew, Whitehouse and Monaghan2014). Neoproterozoic rocks at the base of the Dalradian, or sub-Grampian Group (Glen Banchor, Dava Group) rocks, are possible lateral equivalents to the Moine Supergroup in the Northern Highlands terrane. The Appin Group comprises the Ballachulish, Lochaber and Blair Atoll formations, with ages ranging from ∼800 to 660 Ma (Prave et al. Reference Prave, Fallick, Thomas and Graham2009), and is overlain by the Argyll Group, which includes the 600 Ma old Tayvallich volcanics, and intruded by ∼590 Ma granites, notably the Ben Vurich and Portsoy granites (Tanner et al. Reference Tanner, Leslie and Gillespie2006; Tanner, Reference Tanner, Corfu, Gasser and Chew2014). The youngest protolith sedimentary rocks in the Trossachs Group contain trilobite faunas, dated as Arenig, ∼478–468 Ma (Tanner, Reference Tanner, Corfu, Gasser and Chew2014). Sedimentation associated with the Ballantrae ophiolite also continued up to late Arenig time (∼470–466 Ma; Tanner, Reference Tanner, Corfu, Gasser and Chew2014), correlative with the younger Dalradian sediments to the NW. In the southern part of the Grampian Highlands terrane, ophiolite complexes (Bute, Tyrone ophiolites) were obducted from the oceanic Midland Valley terrane NW onto the Laurentian southern passive margin (protolith of the Dalradian metasediments) between ∼490 and 470 Ma (Dewey & Shackleton, Reference Dewey and Shackleton1984; Chew et al. Reference Chew, Daly, Magna, Page, Kirkland, Whitehouse and Lam2010; Chew & Strachan, Reference Chew, Strachan, Corfu, Gasser and Chew2014). The Unst ophiolite in the Shetland Islands has a comparable age with a U–Pb zircon date on the metamorphic sole of 484 ± 4 Ma (Crowley & Strachan, Reference Crowley and Strachan2015), but it is uncertain how the Shetland Islands geometrically fits with the Northern Highlands (Moine Supergroup) and Grampian Highlands (Dalradian Supergroup) structures. The GGF has been correlated northwards to the WBF on Shetland, but the two faults do not appear to link on seismic profiles in the Moray Firth and across the northern North Sea (Stoker et al. Reference Stoker, Hitchen and Graham1993; Chadwick & Evans, Reference Chadwick, Evans and Boldy1995). By 470 Ma Dalradian Supergroup sedimentation had ceased, and crustal thickening, regional metamorphism and S-type granite intrusion began (Fig. 4).
Metamorphism of the Dalradian Supergroup (Figs 4, 5) is complex involving two facies series, a regional Barrovian-type metamorphism characterized by biotite, garnet, staurolite, kyanite and sillimanite in pelitic rocks (Barrow, Reference Barrow1893, Reference Barrow1912), and a localized LP–HT Buchan facies series in the NE characterized by LP–HT andalusite, cordierite and sillimanite index minerals in pelites (Read, Reference Read1923, Reference Read1955; Fettes, Reference Fettes1970; Fettes et al. Reference Fettes, Long, Bevins, Max, Oliver, Primmer, Thomas, Yardley and Harris1985; Harte, Reference Harte, Harris and Fettes1988). Buchan-type metamorphism is spatially associated with a suite of gabbro and diorite intrusions (Fettes, Reference Fettes1970; Harte & Hudson, Reference Harte, Hudson, Harris, Holland and Leake1979; Dempster et al. Reference Dempster, Rogers, Tanner, Bluck, Muir, Redwood, Ireland and Paterson2002), but appears to be continuous with, and overprinted by the high-grade regional Barrovian facies series (Fettes, Reference Fettes1970; Dempster & Harte, Reference Dempster and Harte1986). Dempster et al. (Reference Dempster, Hudson and Rogers1995, Reference Dempster, Rogers, Tanner, Bluck, Muir, Redwood, Ireland and Paterson2002) described the overprinting of low-pressure andalusite-bearing Buchan assemblages with Barrovian facies kyanite-grade metamorphism. The additional heat and lower pressure conditions of Buchan metamorphic rocks are probably accounted for by high-level gabbroic intrusions that are only present in the NE Grampian Highlands (Fig. 4). Johnson et al. (Reference Johnson, Kirkland, Reddy, Evans and McDonald2016) published U–Pb zircon ages of 486 ± 9 Ma and 493 ± 8 Ma for diorites within the Portsoy gabbro, ages that are broadly similar to the youngest preserved fossiliferous sediments in the Trossachs Group (Dalradian), and also to the age of ophiolite obduction (Fig. 4).
Originally thought be a short-lived (<10 m.y.; 470–460 Ma) metamorphism associated with ophiolite obduction (Dewey, Reference Dewey2005), or alternatively with crustal extension (Viete et al. Reference Viete, Oliver, Fraser, Forster and Lister2013; Viete & Lister, Reference Viete and Lister2016), recent radiometric age dating casts doubt on this model. Granitic and gabbroic intrusions in the Dalradian have emplacement ages of ∼493–440 Ma (Fig. 4), dates that are roughly concomitant with the metamorphic ages (Oliver et al. Reference Oliver, Chen and Buchwaldt2000; Appleby et al. Reference Appleby, Graham, Gillespie, Hinton and Oliver2008; Johnson et al. Reference Johnson, Kirkland, Reddy, Evans and McDonald2016). Baxter et al. (Reference Baxter, Ague and DePaolo2002) and Bird et al. (Reference Bird, Thirlwall, Strachan and Manning2013) reported Sm–Nd garnet whole-rock dates of Dalradian pelites starting at 473–467 Ma and lasting at least until 464.8 ± 2.7 Ma, interpreted as dating prograde metamorphism. Bird et al. (Reference Bird, Thirlwall, Strachan and Manning2013) also published Lu–Hf garnet ages, 470.8 ± 3.2 Ma from the Leven schist and 463.2 ± 1.7 Ma from metabasic intrusions within the Dava succession, that confirm the contemporaneous metamorphism of the Moine and Dalradian supergroups during the older Grampian metamorphic episode (Fig. 4). So far, the younger metamorphic ages from Sm–Nd and Lu–Hf garnet (Bird et al. Reference Bird, Thirlwall, Strachan and Manning2013) and U–Pb monazite (Mako et al. Reference Mako, Law, Caddick, Thigpen, Ashley, Cottle and Kylander-Clark2019) Silurian ages from the Moine Supergroup have not been replicated in the Dalradian Supergroup. However, extensive high 87Sr/86Sr S-type granites in the Dalradian, spanning 475–440 Ma (Oliver et al. Reference Oliver, Wilde and Wan2008), and the Silurian – Early Devonian ‘Newer Granites’ of Pankhurst & Sutherland (Reference Pankhurst, Sutherland and Sutherland1982), attest to temperatures high enough for crustal melting during this time. On the basis of the garnet and monazite ages, as well as the dating of S-type granites, it seems more likely that post-accretion and syn-collision crustal thickening resulted in a single extended period of regional metamorphism, including both the earlier, localized Buchan event, and the overprinting and longer-lasting Barrovian sequences, with dates spanning 478–420 Ma, rather than two separate short-lived ‘events’ (Strachan et al. Reference Strachan, Harris, Fettes, Smith and Trewin2002; Dewey, Reference Dewey2005; Johnson & Strachan, Reference Johnson and Strachan2006; Viete et al. Reference Viete, Oliver, Fraser, Forster and Lister2013; Viete & Lister, Reference Viete and Lister2016). The more localized LP–HT Buchan facies series can be spatially related to the gabbro intrusions in the NE part of the Grampian Highlands (Dempster et al. Reference Dempster, Hudson and Rogers1995, Reference Dempster, Rogers, Tanner, Bluck, Muir, Redwood, Ireland and Paterson2002; Baxter et al. Reference Baxter, Ague and DePaolo2002).
Deformation of the Dalradian Supergroup in the Grampian Highlands terrane is also extremely complex. Figure 7a shows a simplified cross-section across the SW part of the terrane (after Roberts & Treagus, Reference Roberts and Treagus1977; Stephenson et al. Reference Stephenson, Mendum, Fettes and Leslie2013a,b; Tanner, Reference Tanner, Corfu, Gasser and Chew2014), with a simplified restoration of the major structures shown in Figure 7b. The overall structure is a complex one showing a major NW-facing recumbent fold (Islay anticline) in the NW, and a huge SW-facing recumbent fold, the Tay nappe, along the SE margin (Shackleton, Reference Shackleton1958; Krabbendam et al. Reference Krabbendam, Leslie, Crane and Goodman1997). A minimum shortening of ∼65 km occurs simply by restoring the major fold structures (Fig. 7b), although the true amount of crustal shortening is likely double this.

Fig. 7. (a) Simplified cross-section across the Grampian Highlands Dalradian Supergroup, after Roberts & Treagus (Reference Roberts and Treagus1977), Stephenson et al. (Reference Stephenson, Mendum, Fettes and Leslie2013a,b) and Tanner (Reference Tanner, Corfu, Gasser and Chew2014). (b) Restoration of the major fold structures in the Dalradian, showing minimum amounts of crustal shortening.
6. Great Glen fault (mainland Scotland) and Walls Boundary fault (Shetland)
In mainland Scotland, the GGF is the major NE–SW-aligned strike-slip fault that separates the Moine Supergroup in the Northern Highlands terrane to the NW from the Dalradian Supergroup in the Grampian Highlands terrane to the SE (Fig. 1). The first recognition of strike-slip motion along the GGF was by Kennedy (Reference Kennedy1946) who proposed 65 miles (∼100 km) of sinistral offset, based on the offset of the Strontian granite (location 10 on Fig. 8; 425 ± 3 Ma; Rogers & Dunning, Reference Rogers and Dunning1991) and Foyers granite (location 20 on Fig. 8; 424 ± 4 Ma; Oliver et al. Reference Oliver, Wilde and Wan2008), and the offset of metamorphic zones in the Northern Highlands and those in the Grampian Highlands (Winchester, Reference Winchester1974). Since the Devonian Old Red Sandstone is also affected by the fault in places, Kennedy (Reference Kennedy1946) proposed that slip occurred post-Devonian, but pre-late Carboniferous. Both the Strontian and Foyers granites show a zonation from tonalite–granodiorite–granite towards the core, and a broadly similar geochemical evolution and zircon inheritance, confirming a strong affinity, but not necessarily proof that they were once the same pluton (W. E. Stephens, pers. comm. 2021).

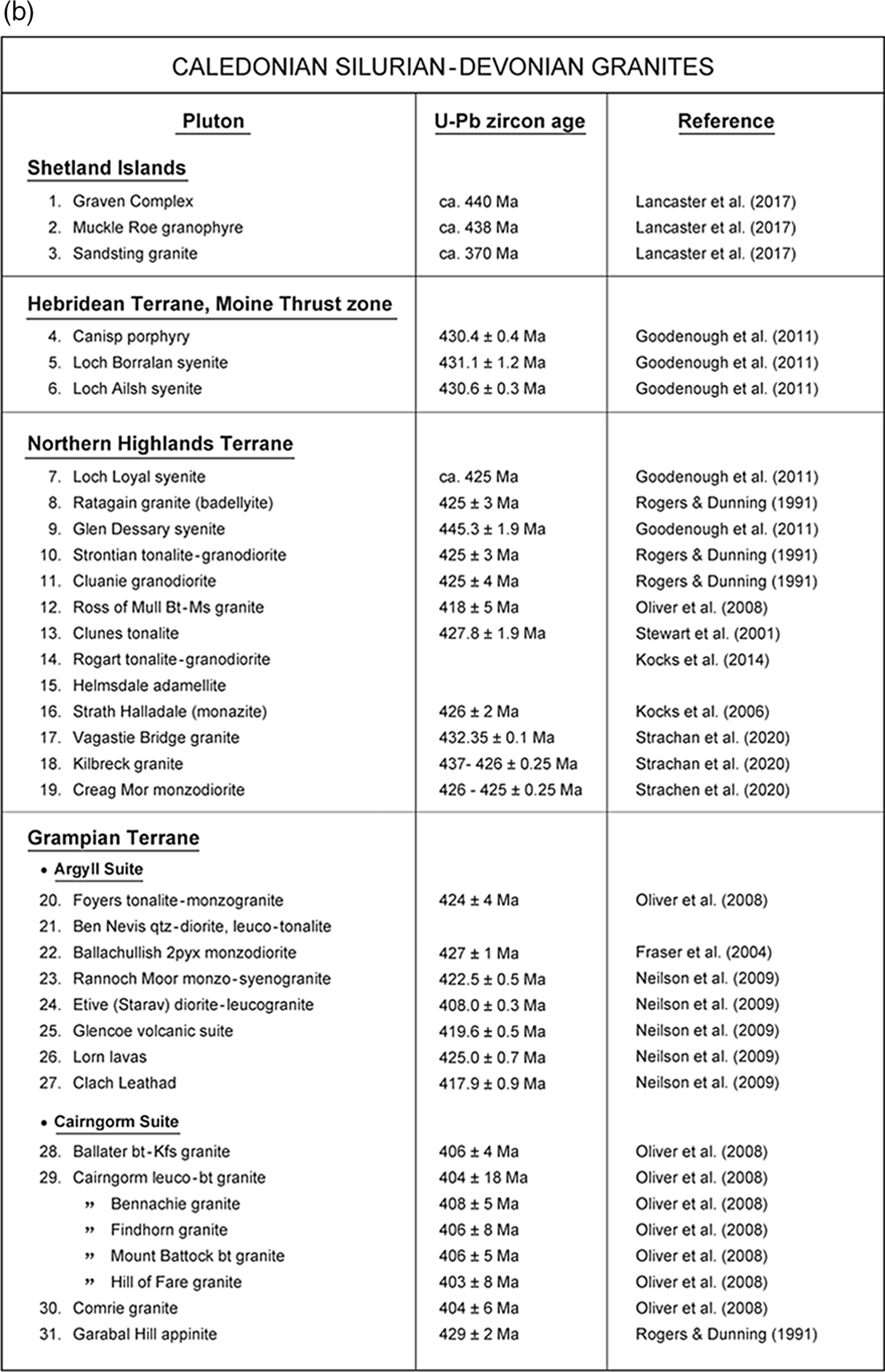
Fig. 8. Map of Scotland, northern Ireland, and northern England, showing locations of the Silurian – early Devonian granites. U–Pb ages for each dated granite pluton are shown in the key.
Post-Caledonian motion along the GGF is mainly dextral or extensional, with the GGF and Helmsdale fault clearly cutting Jurassic rocks onshore and Cretaceous rocks offshore in the Moray Firth (Chadwick & Evans, Reference Chadwick, Evans and Boldy1995). Rogers et al. (Reference Rogers, Marshall and Astin1989) documented 25–29 km of dextral displacement along the GGF between late Frasnian and early Permian times based on offset of mapped Old Red Sandstone on the Scottish mainland. Dextral displacement of ∼8 km has been proposed, based on the offset of a Permian–Carboniferous dyke swarm in Argyll (McQuillan et al. Reference McQuillan, Donato and Tulstrup1982). Some authors have proposed a dominant normal faulting component, down-to-SE during the Mesozoic period forming a boundary of the North Sea extensional graben systems to the west (Bacon & Chesher, Reference Bacon and Chesher1975).
Determining older Caledonian motion along the GGF is more difficult. The fault is poorly exposed, running mainly along the deep glaciated valleys of Loch Linnhe and Loch Ness. The GGF cuts metamorphic fabrics in the Moine Supergroup to the NW and those in the Dalradian Supergroup to the SE, as well as cutting several granitic plutons. Phyllonites, mylonites and cataclasites showing sinistral kinematic indicators crop out near Fort William, and provide clear evidence of sinistral displacement (Stewart et al. Reference Stewart, Strachan and Holdsworth1997). Left-lateral shear along the GGF clearly occurred after metamorphism in the Moine (c. 478–420 Ma), and after metamorphism and crustal melt granite formation in the Dalradian (472–435 Ma). The ‘Newer Granites’, intruded into the Dalradian Supergroup between c. 435 and 405 Ma, are present across the whole Grampian terrane, as well as parts of the Northern Highlands terrane, and are not spatially associated with strike-slip faults. The GGF cuts two such granites to the NW along its trace, the Strontian granite (location 10 on Fig. 8) and the Clunes tonalite (location 13 on Fig. 8; 427.8 ± 1.9 Ma; Stewart et al. Reference Stewart, Strachan, Martin and Holdsworth2001), and one granite, the Foyers granite to the SE (location 20 on Fig. 8). The Glenelg–Ratagain granite (location 8 on Fig. 8; U–Pb zircon age 425 ± 3 Ma; Rogers & Dunning, Reference Rogers and Dunning1991; Hutton & McErlean, Reference Hutton and McErlean1991) is cut along the south side by the Strathconon fault, which runs parallel to the GGF within the Moine Supergroup. A swarm of lamprophyre dykes dated at 435–410 Ma (Rock & Hunter, Reference Rock and Hunter1987) is also cut by the GGF. The ductile shear fabrics in the granites were clearly formed after crystallization of the intrusive granite (<425 Ma), not during granite emplacement, as proposed by Hutton (Reference Hutton1988), Hutton & McErlean (Reference Hutton and McErlean1991) and Hutton & Reavy (Reference Hutton and Reavy1992). The ductile shear fabrics associated with, and parallel to, the GGF must have been imposed after 424 Ma, the youngest emplacement age of the granite, or after 410 Ma, the youngest age of lamprophyre dykes cut by the GGF, and likely before c. 405 Ma, the base of the unconformably overlying Old Red Sandstone (Fig. 4). Since the Old Red Sandstone is also offset by limited amounts along the GGF, some limited motion continued after this time, into Devonian time.
The GGF was previously thought to continue NE to connect with the WBF in the Shetland Islands (Flinn, Reference Flinn1992; Watts et al. Reference Watts, Holdsworth, Sleight, Strachan and Smith2007; Dewey et al. Reference Dewey, Dalziel, Reavy and Strachan2015). However, the two faults are not directly connected on seismic reflection profiles across the northern North Sea (Chadwick & Evans, Reference Chadwick, Evans and Boldy1995) and the GGF seems to have a different kinematic history (Armitage et al. Reference Armitage, Watts, Holdsworth and Strachan2021). The Shetland Islands expose Lewisian-type Neoarchaean basement and overlying Precambrian–Cambrian metasedimentary rocks (Sand Voe, Yell Sound and Westing groups and East Mainland succession), in turn overlain by Devonian sedimentary and volcaniclastic successions (Armitage et al. Reference Armitage, Watts, Holdsworth and Strachan2021). Neoproterozoic to Devonian rock units are present both west and east of the WBF. Late Ordovician (c. 465 Ma) to Late Devonian (c. 360 Ma) granites intrude both west and east of the WBF (Lancaster et al. Reference Lancaster, Strachan, Bullen, Fowler, Jaramillo and Saldarriaga2017). On the islands of Unst and Fetlar, a late Cambrian to Early Ordovician ophiolite sequence has a U–Pb zircon age of 484 ± 4 Ma from the metamorphic sole (Crowley & Strachan, Reference Crowley and Strachan2015), similar timing as the Ballantrae ophiolite south of the Midland Valley arc, and the Highland Border ophiolites (Bute ophiolite) (Fig. 4). On Shetland, Armitage et al. (Reference Armitage, Watts, Holdsworth and Strachan2021) documented intense dextral late Carboniferous transpressional fabrics, including a 2 km wide zone of cataclasites, fault gouge and local pseudotachylytes that formed during dextral strike-slip shearing. Dextral motion along the WBF is constrained as being post-370 Ma, the age of granites cut by the fault, and prior to overlying Permian sedimentary rocks at c. 330–300 Ma (Armitage et al. Reference Armitage, Watts, Holdsworth and Strachan2021). Dewey et al. (Reference Dewey, Dalziel, Reavy and Strachan2015) proposed at least 500–700 km of sinistral motion along the GGF–WBF between 428 and 385 Ma. There are, however, no clear pinning points either side of the GGF, so these amounts are only speculation. Dewey & Strachan (Reference Dewey and Strachan2003) proposed that the GGF, together with other similar strike-slip faults, shows up to 1200 km of sinistral displacement, during the period 435–395 Ma, during which the GGF juxtaposed the Dalradian Supergroup rocks in the SE next to the Moine Supergroup rocks in the NW. If this model was correct the obvious question arises: what lay in the space 700–1200 km long, between the Moine and Dalradian terranes prior to slip along the GGF? These very large strike-slip offsets must imply either that the Moine and Dalradian supergroups were separate ‘blocks’ or ‘exotic terranes’, separated by some unknown oceanic tract, or that very large lateral strike-slip motion occurred along the Laurentian continental margin at the end of the Caledonian orogeny.
7. Silurian: Devonian granites and volcanic rocks
A large volume of granites was emplaced into the crust during late Silurian – early Devonian times (435–408 Ma) across northern Scotland on both sides of the GGF, as well as in the Southern Uplands suture zone and northern England Avalonian terrane, the latter called the Trans-suture granite suite (Read, Reference Read1961; Stephens & Halliday, Reference Stephens and Halliday1984; Atherton & Ghani, Reference Atherton and Ghani2002; Fowler et al. Reference Fowler, Kocks, Darbyshire and Greenwood2008; Neilson et al. Reference Neilson, Kokelaar and Crowley2009; Miles et al. Reference Miles, Woodcock and Hawkesworth2016). Figure 8a shows the locations of the Silurian – early Devonian granites across Scotland, northern Ireland and northern England, together with a key showing U–Pb ages and references from sources of data (Figure 8b). Figure 9 is a compilation of all the U–Pb zircon and monazite ages as well as Sm–Nd and Lu–Hf garnet ages of granitoids and metamorphic rocks across the Northern Highlands and Grampian Highlands terranes.
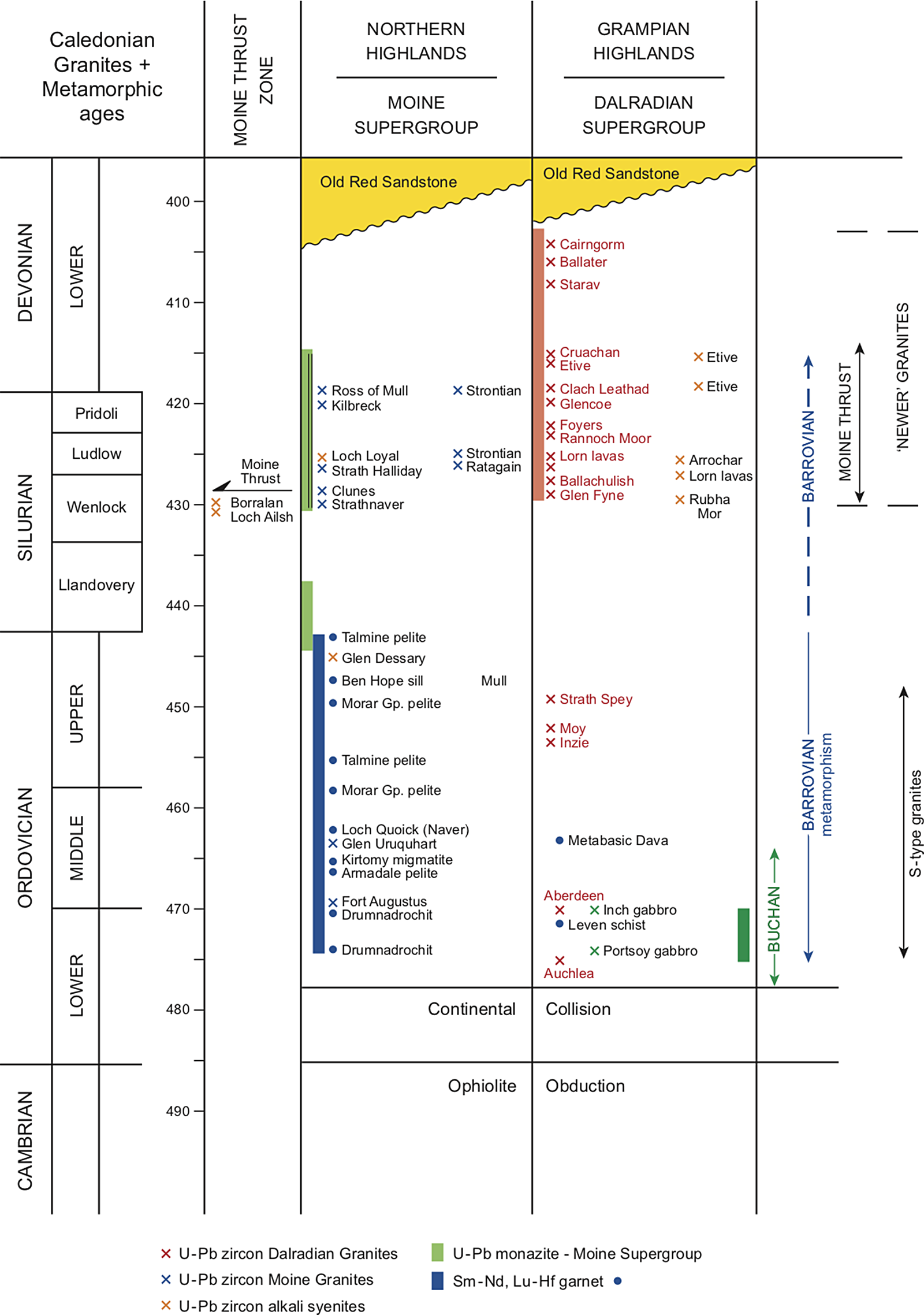
Fig. 9. Lower Palaeozoic time chart showing the age ranges of granites (U–Pb zircon) and metamorphic rocks (U–Pb monazite, Sm–Nd and Lu–Hf garnet) dates. U–Pb zircon age data is compiled from Rogers & Dunning (Reference Rogers and Dunning1991), Kinny et al. (Reference Kinny, Friend, Strachan, Watt and Burns1999, Reference Kinny, Strachan, Friend, Kocks, Rogers and Paterson2003), Dempster et al. (Reference Dempster, Rogers, Tanner, Bluck, Muir, Redwood, Ireland and Paterson2002), Oliver et al. (Reference Oliver, Wilde and Wan2008), Neilson et al. (Reference Neilson, Kokelaar and Crowley2009) and Goodenough et al. (Reference Goodenough, Millar, Strachan, Krabbendam and Evans2011). U–Pb monazite age data from the Moine is from Mako et al. (Reference Mako, Law, Caddick, Thigpen, Ashley, Cottle and Kylander-Clark2019). Lu–Hf and Sm–Nd garnet age data is from Baxter et al. (Reference Baxter, Ague and DePaolo2002), Cutts et al. (Reference Cutts, Kinny, Strachan, Hand, Kelsey, Emery, Friend and Leslie2010), Bird et al. (Reference Bird, Thirlwall, Strachan and Manning2013). Ages are based on the International Chronostratigraphic Chart 2016/04.
The ‘Newer Granites’ (Read, Reference Read1961) have been divided into the compositionally distinct Argyll and Northern Highlands suite, and low-Ba and Sr granites of the Cairngorm suite (Stephens & Halliday, Reference Stephens and Halliday1984). Stephens & Halliday (Reference Stephens and Halliday1984) recognized three different suites, all geochemically distinct: (a) the Argyll suite, (b) the Cairngorm suite, both in the Dalradian, and (c) the South of Scotland suite, or the so-called Trans-suture granites across the Southern Uplands into northern England (Shap, Weardale, Skiddaw granites). The Argyll suite is characterized by hornblende-bearing granites and diorites, with high Ba, Sr, K2O, Na2O and La/Yb ratios, and are closely associated, both spatially and temporally, with appinites and lamprophyres, similar to the Silurian plutons north of the GGF (Stephens & Halliday, Reference Stephens and Halliday1984). The Cairngorm suite is composed mainly of silicic granites with high Nb, Rb and Th, and low Ba and Sr (Stephens & Halliday, Reference Stephens and Halliday1984). The Trans-suture granites have U–Pb zircon ages between 426 and 395 Ma, overlapping with ages of the ‘Newer Granites’ in the Dalradian Supergroup (Soper, Reference Soper1986; Miles et al. Reference Miles, Woodcock and Hawkesworth2016).
The younger granites intruding Dalradian rocks of the Grampian Highlands have U–Pb zircon ages spanning 430–405 Ma (Fig. 9). Re–Os molybdenite ages of the Ballachulish (433.5 ± 1.8 Ma) and Kilmelford (425.8 ± 1.7 Ma) igneous suites overlap with the U–Pb zircon ages (Conliffe et al. Reference Conliffe, Selby, Porter and Feely2010). Similar age granites occur in the Donegal pluton in western Ireland (Pitcher, Reference Pitcher1972; Pitcher & Berger, Reference Pitcher and Berger1972) and in northern England (e.g. Shap, Weardale, Skiddaw granites; Miles et al. Reference Miles, Woodcock and Hawkesworth2016). U–Pb zircon ages of granites intruding the Moine Supergroup in the Northern Highlands have a similar span of ages from c. 430–418 Ma. These include the Strath Naver, Klibreck, Clunes tonalite, Ratagain, Strontian and Ross of Mull granites (Rogers & Dunning, Reference Rogers and Dunning1991; Stewart et al. Reference Stewart, Strachan, Martin and Holdsworth2001; Kinny et al. Reference Kinny, Strachan, Friend, Kocks, Rogers and Paterson2003; Oliver et al. Reference Oliver, Wilde and Wan2008; Strachan et al. Reference Strachan, Alsop, Ramezani, Frazer, Burns and Holdsworth2020). The ages of the ‘Newer Granites’ suite are also similar to the large syenite intrusions in the Assynt area (Canisp, Loch Borralan, Loch Ailsh syenites), which are 431–429 Ma (Goodenough et al. Reference Goodenough, Millar, Strachan, Krabbendam and Evans2011; Fox & Searle, Reference Fox and Searle2021).
The Silurian – Early Devonian granites in the Northern Highlands and Central Highlands (Grampian) terranes have been used as evidence that subduction dipped NW beneath Scotland during this (Scandian) period (Atherton & Ghani, Reference Atherton and Ghani2002; Neilson et al. Reference Neilson, Kokelaar and Crowley2009). However, the granites are not restricted to the Moine–Dalradian terranes as would be expected in this Andean-type model, but are also present across the suture zone, and in the Avalon terrane in northern England. Silurian–Devonian granites are not similar to Andean granites, in that the late Caledonian granites are generally not associated with gabbros, or related volcanic rocks, and they have a more alkaline geochemistry (Pitcher, Reference Pitcher1972; Chappell & Stephens, Reference Chappell and Stephens1988; Atherton & Ghani, Reference Atherton and Ghani2002; Neilson et al. Reference Neilson, Kokelaar and Crowley2009). Andean granites have only a minor S-type component (e.g. Cordillera Blanca granites, Peru; Pitcher, Reference Pitcher1972), again unlike the Caledonian granites, and they are not associated with regional lamprophyric magmatism as seen across northern Scotland. The classic I–S classification of Chappell & White (Reference Chappell and White1974, Reference Chappell and White2001) works well for end-members such as typical Andean granites (I-type) or typical Himalayan crustally derived leucogranites (S-type), but the Caledonian granites do not fit either category easily. A-type granite magmatism (syenites, syenogranites, etc) is the most controversial category. These granites include associated ultrapotassic lamprophyres, shoshonites and adakites, and typically occur in late, post-collision settings or anorogenic ‘within-plate’ settings (Pearce et al. Reference Pearce, Harris and Tindle1984; Whalen & Hildebrand, Reference Whalen and Hildebrand2019). Many of the Caledonian granites have compositional and temporal similarities with associated appinite and lamprophyre magmas.
Most of the Silurian – Early Devonian granites in Scotland are medium to high-K calc-alkaline monzodiorite, granodiorite and monzogranite of intermediate to silicic compositions, with a great variation in incompatible trace elements and light rare earth elements (LREEs). They have an enriched lithospheric mantle signature and 87Sr/86Sr initial ratios of <0.708, and are associated with small-volume appinite–lamprophyre intrusions (Halliday, Reference Halliday1984; Rock & Hunter, Reference Rock and Hunter1987; Shand et al. Reference Shand, Gaskarth, Thirlwall and Rock1994; Neilson et al. Reference Neilson, Kokelaar and Crowley2009; Miles et al. Reference Miles, Woodcock and Hawkesworth2016). Appinites can range from ultramafic to felsic in composition, with extensive metasomatic fluid infiltration resulting in geochemical characteristics indistinguishable from low-K, calc-alkalic arc magmatism (Murphy, Reference Murphy2020). In Scotland, appinites are melanocratic hornblende-rich syenites, monzonites and diorites that span alkaline to calc-alkaline trends. Lamprophyres are small-volume alkaline, silica-undersaturated, mafic and ultramafic rocks derived from deep and hot mantle melting. They include biotite- and hornblende-phyric minettes, vogesites and alkali feldspar-bearing micro-syenites and rhyolites (Rock, Reference Rock1984; Rock & Hunter, Reference Rock and Hunter1987). The appinite–lamprophyre intrusions have high Ni, Cr, V and MgO with high large-ion lithophile elements (LILE) and LREE abundances, consistent with plagioclase-absent fractional crystallization (Neilson et al. Reference Neilson, Kokelaar and Crowley2009). Lamprophyre magmatism is a common late orogenic occurrence, for example across Tibet (Chung et al. Reference Chung, Chu, Zhang, Xie, Lo, Lee, Lan, Li, Zhang and Wang2005), but they do not require crustal thinning or any form of slab break-off to form.
Volcanic rocks, mainly hypabyssal to extrusive basalt–andesite–trachyte–rhyolites, are associated with some of the Silurian – Early Devonian granites and are mainly present across the Central Highlands Grampian terrane (Figs 8, 9). They include the large Rannoch Moor (422.5 ± 0.5 Ma), Ben Nevis, Cruachan, Starav–Etive (414.9 ± 0.7 Ma) and Ballachulish (419.6 ± 5.4 Ma) granites in the Dalradian Supergroup, and the Loch Linne and Strontian granites NW of the GGF in the Moine Supergroup (Neilson et al. Reference Neilson, Kokelaar and Crowley2009). The Caledonian volcanic rocks include the Lorn–Etive volcanic sequence and the Glencoe caldera volcanoes, which are the extrusive equivalents of the large-scale Lochaber batholith (Kokelaar & Moore, Reference Kokelaar and Moore2006). The Lorn lavas (location 26, Fig. 8; U–Pb zircon ages 426–425 Ma) around Oban are andesites and dacites erupted some 5 m.y. before the major Glencoe caldera lavas and the Etive dyke swarm (location 24, Fig. 8; U–Pb zircon ages 420–415 Ma; Neilson et al. Reference Neilson, Kokelaar and Crowley2009). The andesites and trachyandesites in the Grampian Highlands were erupted continuously over a period of ∼22 m.y. from c. 430 to 408 Ma, and were concomitant with emplacement of shoshonites and lamprophyres within the Lorn–Etive lava pile, dated at 430–415 Ma (Kokelaar & Moore, Reference Kokelaar and Moore2006; Neilson et al. Reference Neilson, Kokelaar and Crowley2009). The temporal and spatial association of the volcanic rocks with shoshonites and lamprophyres is similar in many respects to the extensive, but small-volume, post-collisional melts exposed across the Tibetan plateau (Chung et al. Reference Chung, Chu, Zhang, Xie, Lo, Lee, Lan, Li, Zhang and Wang2005; Searle, Reference Searle and Watts2015). The Grampian granite suite, including the appinites and the lamprophyric dykes, were intruded at a similar time as the Assynt alkaline suite (Canisp, Loch Borralan, Loch Ailsh; 431–429 Ma; Goodenough et al. Reference Goodenough, Millar, Strachan, Krabbendam and Evans2011; Fox & Searle, Reference Fox and Searle2021) in the Moine thrust zone, suggesting a far more widespread regional alkaline igneous event during this time.
There are no subduction-related, Andean-type granites emplaced in the Grampian terrane during subduction accompanying the Early to Middle Ordovician closure of the Iapetus ocean along the Southern Uplands suture zone, as would be expected from an Andean analogy (Chappell & Stephens, Reference Chappell and Stephens1988). Miles et al. (Reference Miles, Woodcock and Hawkesworth2016) noted that there was an absence of magmatic activity during Iapetus subduction between c. 455 and 425 Ma. Instead, the ‘Newer Granites’ generally have younger U–Pb zircon ages between c. 430 and 408 Ma, and have an origin related to partial melting of a LILE- and LREE-enriched mantle (Neilson et al. Reference Neilson, Kokelaar and Crowley2009). The ubiquitous lamprophyres associated with the granites may relate to a deeper, hotter, possibly asthenospheric mantle origin. The granites are scattered throughout the Grampian terrane and the southern part of the Northern Highlands terrane, as well as the Southern Uplands and northern England (Trans-suture granites). They are unrelated, either geographically or temporally, to strike-slip faulting, either along the GGF or other strike-slip faults, most of which were active later (<405 Ma). The generation of these granites remains a puzzle, but we can rule out models involving an Andean-type, subduction-related origin and intrusion along strike-slip faults. Their origin appears more related to late or post-collision alkaline A-type syenite, syeno-granite and monzonite suites, ultimately derived from partial melts of enriched (K-amphibole, phlogopite-bearing) sub-continental lithospheric mantle combined with lower crust melting and some possible assimilation (Neilson et al. Reference Neilson, Kokelaar and Crowley2009).
8. Highland Boundary fault
The HBF dividing the Central (Grampian) Highlands to the NW from the Midland Valley to the SE is a major geological feature running from Stonehaven on the east coast to the island of Bute on the west coast of Scotland (Fig. 1), and further SW across northern Ireland (Chew et al. Reference Chew, Daly, Magna, Page, Kirkland, Whitehouse and Lam2010). The Clew Bay complex in western Ireland is the youngest lower Palaeozoic part of the Dalradian Supergroup (Chew et al. Reference Chew, Daly, Magna, Page, Kirkland, Whitehouse and Lam2010) and the Trossachs Group on Arran (Tanner & Sutherland, Reference Tanner and Sutherland2007). It is not clear where the fault runs across the island of Arran, partly because of the intrusion of the Paleocene Arran granite across the westward extension of the fault zone. The Highland Border complex is a zone less than 1 km across that contains a diverse sequence of mudstones, limestones, cherts, pillow lavas and highly altered serpentinites. A series of highly deformed ophiolitic rocks crop out along the Highland Boundary, notably the Bute ophiolite (Ayrshire), Unst ophiolite (Shetland Islands) and the Tyrone ophiolite in Ireland (Chew et al. Reference Chew, Daly, Magna, Page, Kirkland, Whitehouse and Lam2010; Tanner, Reference Tanner, Corfu, Gasser and Chew2014). The Highland Border complex was initially interpreted as an exotic terrane juxtaposed next to the Grampian terrane by strike-slip motion along the HBF (Bluck, Reference Bluck1984, Reference Bluck1985). It has also been interpreted as a major Caledonian sinistral strike-slip fault (Woodcock & Strachan, Reference Woodcock and Strachan2012) and a NW-dipping thrust (Tanner, Reference Tanner2007, Reference Tanner2008). However, it now known that the sub-ophiolite sediments form a continuous succession with the Dalradian rocks, and the Grampian terrane was overthrust by the Highland Border ophiolites during late Cambrian – Early Ordovician times (Tanner & Sutherland, Reference Tanner and Sutherland2007; Chew et al. Reference Chew, Daly, Magna, Page, Kirkland, Whitehouse and Lam2010; Cawood et al. Reference Cawood, Strachan, Merle, Millar, Loewy, Dalziel, Kinny, Jourdan, Nemchin and Connelly2015). The Highland Border ophiolites were initially obducted to the NW during the period 510–485 Ma and later steepened and overturned to dip steeply NW (Fig. 10). The HBF is interpreted here as a steep, NW-dipping (back)thrust fault that cuts structures in the Dalradian Tay nappe to the NW (Harris et al. Reference Harris, Bradbury and McGonigal1976), and also cuts Lower Devonian rocks in the Midland Valley terrane to the SE. Earlier Cambrian ophiolite obduction structures, both in the Highland Border remnant ophiolites and the underlying Dalradian Tay nappe, have been overprinted by large-scale refolding and backthrusting. Lateral displacements are limited, less than 30 km (Tanner, Reference Tanner2008).
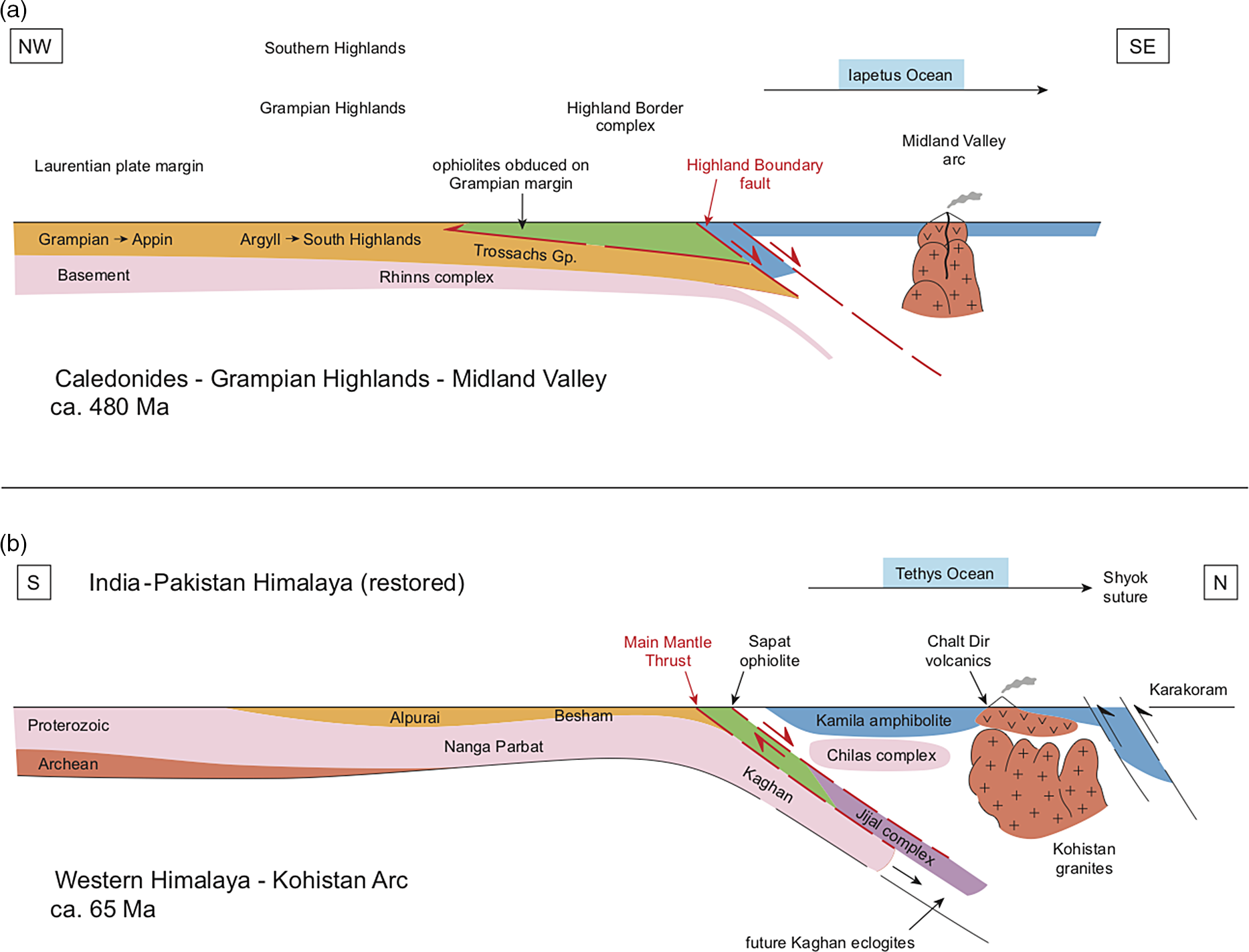
Fig. 10. (a) Reconstruction of the Laurentian margin at c. 480 Ma showing geometry of the margin, the Dalradian continental margin, the Highland Border ophiolite suites, and the Midland Valley arc, after Chew et al. (Reference Chew, Daly, Magna, Page, Kirkland, Whitehouse and Lam2010). (b) Reconstruction of the Indian plate continental margin in the western Himalaya, showing the geometry of similar thrust sheets related to obduction of the Late Cretaceous Sapat ophiolite, accretion of the large-scale Kohistan island arc, with suture zone rocks along both margins; after Searle (Reference Searle and Watts2015).
The major point of contention now lies in whether the Buchan and early Barrovian metamorphic facies series in the Dalradian (Grampian phase of the orogeny) was a result of the obduction of ophiolites (Dewey & Shackleton, Reference Dewey and Shackleton1984; Dewey et al. Reference Dewey, Dalziel, Reavy and Strachan2015), or whether the metamorphism was related to a later, Himalayan-style crustal thickening event. Despite some models for Himalayan crustal thickening being related to ophiolite obduction (Spontang ophiolite; Searle & Treloar, Reference Searle, Treloar, Kusky, Zhai and Xiao2010), most U–Pb monazite ages and high-temperature geochronology suggest that Himalayan metamorphism was related to post-obduction, continental collision-related crustal thickening and internal heat generation during middle Eocene to Miocene (∼45–20 Ma) crustal thickening and shortening (Searle, Reference Searle and Watts2015). The main difference is that the Buchan metamorphism in the Dalradian Grampian Highlands was associated with syn-metamorphic gabbroic intrusions, whereas in the Himalaya, LP–HT metamorphism (andalusite, sillimanite, cordierite) was associated with extensive migmatite and crustal melt leucogranite, and followed the regional staurolite–kyanite–sillimanite regional Barrovian-type metamorphism (see Searle, Reference Searle and Watts2015 for a review). In both the Himalaya and the Caledonian orogenies, ophiolite obduction was followed very closely by arc accretion (Kohistan island arc in the Himalaya; Midland Valley arc in the Caledonides), and continental collision, resulting in crustal thickening, regional Barrovian facies metamorphism and lower crustal melting forming S-type granites. In both orogenic belts the suture zone region was steepened and overturned, as seen along the Indus suture in the Himalaya and the Highland Boundary in Scotland (Fig. 11).
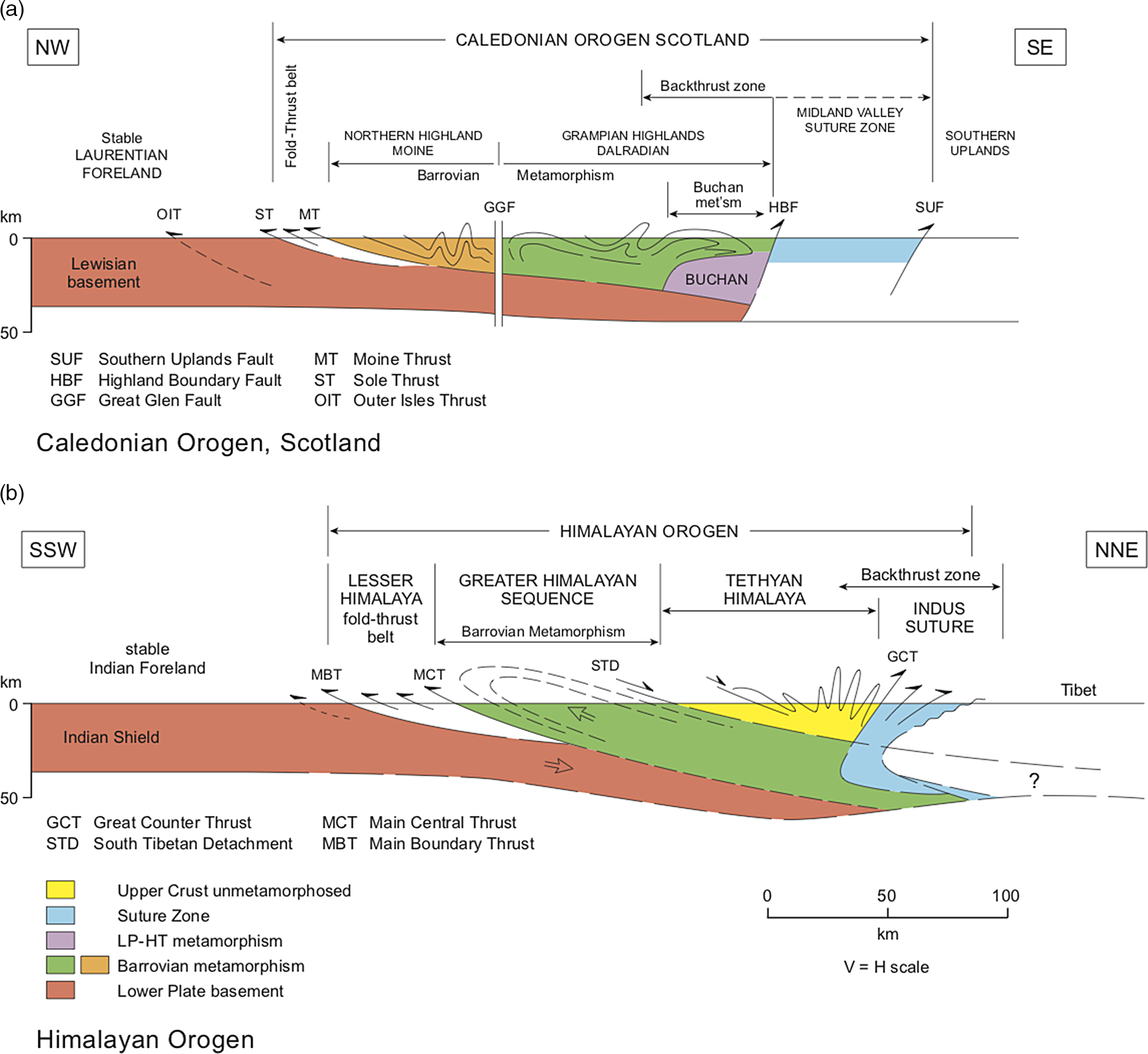
Fig. 11. Simplified cross-sections of (a) the Scottish Caledonides from the Laurentian foreland to the Highland Border and Midland Valley, combining structure of the Moine Thrust zone, Northern Highlands (Moine Supergroup) and Grampian Highlands (Dalradian Supergroup) rocks. (b) Simplified cross-section of the Indian plate Himalaya (after Corfield & Searle, Reference Corfield, Searle, Khan, Treloar, Searle and Jan2000; Searle, Reference Searle and Watts2015) from the Indian foreland to the Indus–Tsangpo suture zone. Note the similarities in scale and fold vergence–facing directions across the Tay nappe backfold and the backfolds across the northern margin of Indian and the Indus–Tsangpo suture zone.
9. New model for Caledonian orogeny
Many published tectonic models invoke a SE-directed subduction during the Grampian ‘orogeny’ that was dominated by NW-directed ophiolite obduction, followed by a subduction switch to NW-dipping subduction during Late Ordovician – Silurian times (Fig. 2; Dewey, Reference Dewey1969; Dewey & Shackleton, Reference Dewey and Shackleton1984; Dewey et al. Reference Dewey, Dalziel, Reavy and Strachan2015). An alternative model presented in this paper is that the entire Caledonian orogeny in Scotland, comprising ophiolite obduction (490–475 Ma) and accretion of the Midland Valley terrane, crustal thickening, regional metamorphism and partial melting (480–420 Ma in the Northern Highlands; 480–435 Ma in the Grampian Highlands), can be explained by continuous SE-directed subduction and NW- to WNW-vergent foreland-directed thrusting. The geometry and time scales of orogeny are closely comparable to the Indian plate – Kohistan island arc – Asia collision zone along the Himalaya. The early parts of the orogenic process (Fig. 10) involve accretion of a large island arc (Kohistan in the Himalaya; Midland Valley arc in the Caledonian orogeny) and ophiolite obduction onto a previously passive continental margin (Indian plate; Laurentian plate margin). Following ophiolite obduction, continental collision-related crustal thickening resulted in widespread Barrovian metamorphism (Greater Himalayan Sequence; Dalradian, Moine metamorphism). The latest stages involved foreland-propagating thrusting (Lesser Himalaya and Main Central Thrust zone in the Himalaya; Moine thrust zone in Caledonides). The orogenic wedge from the Moine thrust zone in the WNW to the HBF in the ESE shows a gradual thickening towards the ESE, consistent with this geometric model. In this model, the Tay nappe and the Highland Border ophiolite are in a backthrust zone (Fig. 11), a similar structure as that seen along the Insubric Line and the SE hinterland margin of the Alps (Schmid et al. Reference Schmid, Pfiffner, Froitzheim, Schönborn and Kissling1997), and also similar to the backthrusting and backfolding mapped along the Indus–Tsangpo suture zone in the India–Asia collision today (Searle, Reference Searle and Watts2015; Corfield & Searle, Reference Corfield, Searle, Khan, Treloar, Searle and Jan2000).
The separation of the Moine and Dalradian supergroups seems unnecessary given the similarities in protolith ages, metamorphic and crustal melt granite dates, and structures. Seismic surveys suggest that the GGF extends to the base of the crust (McBride, Reference McBride1994), but there is no evidence from seismic tomography that it is a plate boundary suture zone (Nicolson et al. Reference Nicolson, Curtis and Baptie2014). There are no ophiolites, deep-sea sedimentary rocks, mélanges or (ultra)high-pressure rocks exposed along the GGF. It is therefore suggested here that the Northern Highlands terrane (Moine Supergroup) and Grampian Highlands terrane (Dalradian Supergroup) were all originally part of one contiguous hyper-extended continental margin along the eastern margin of Laurentia during Neoproterozoic to Ordovician times (Fig. 3), and later deformed and metamorphosed during a single long-lived metamorphic event from c. 470 to 420 Ma (Fig. 4). It is also proposed that late Caledonian offsets along the GGF were far less than those widely accepted, and that the fault post-dates late Silurian – Early Devonian granite emplacement in both the Northern Highlands and Central (Grampian) Highlands terranes. The GGF can only be traced offshore north as far as east of the Orkney Islands, and is not directly connected to the WBF on the Shetland Islands.
10. Discussion
Several contentious issues are apparent from the regional review of the stratigraphy, structure, magmatism, metamorphism and geochronology and the new tectonic model for the evolution of the Scottish Caledonides, presented above.
10.a. Linking protoliths across the Moine and Dalradian supergroups
Restoration of the Moine thrust zone has previously suggested a correlation of the Neoproterozoic Torridon Group sediments on the Laurentian foreland with the protoliths of the Morar and Glenfinnan groups (Moine Supergroup) above the Moine thrust in the Northern Highlands (Geikie, Reference Geikie1893; Turnbull et al. Reference Turnbull, Whitehouse and Moorbath1996; Searle et al. Reference Searle, Cornish, Heard, Charles and Branch2019). Several stratigraphic and igneous correlations can be documented from the Northern Highlands terrane across to the Central Highlands (Grampian) terrane (Figs 3, 4). Both terranes have Neoproterozoic protoliths (Morar/Glenfinnan and Loch Eil groups in the Moine Supergroup; Glen Banchor – Dava groups in the Dalradian Supergroup). These older metasedimentary rocks are cut by the ∼870 Ma West Highland granite gneiss (Leslie et al. Reference Leslie, Smith, Soper, Higgins, Gilotti and Smith2008). Both terranes have evidence of an 840–790 Ma metamorphic event (Knoydartian gneisses and migmatites). Both terranes have a suite of 600–590 Ma granite intrusions (Carn Chuinneag, Inchbae granites in the Moine; Ben Vurich, Portsoy granites in the Dalradian). Following the Knoydartian crustal thickening and metamorphism (c. 840–790 Ma), the Northern Highlands terrane was sub-aerial, and shallow marine sedimentary rocks were deposited across the Dalradian (Argyll, Appin, Southern Highlands and Trossachs groups) supergroups on a hyper-extending passive continental margin, along the margin of the Iapetus ocean, with deeper water facies to the south. The model presented here for the Cambrian passive margin is very similar to that presented by Cawood et al. (Reference Cawood, Nemchin, Strachan, Prave and Krabbendam2007). Along the Highland Border, ophiolites (e.g. Ballantrae, Bute, Unst, Shetland and Tyrone ophiolites) were obducted from SE to NW along this margin (Dewey & Shackleton, Reference Dewey and Shackleton1984; Chew et al. Reference Chew, Daly, Magna, Page, Kirkland, Whitehouse and Lam2010; Chew & Strachan, Reference Chew, Strachan, Corfu, Gasser and Chew2014) in a tectonic setting similar to the Oman ophiolite emplacement onto the previously rifted passive margin of Arabia (Searle & Cox, Reference Searle and Cox1999) and the emplacement of ophiolites and arc accretion in the western Himalaya (Fig. 10).
A modern-day example of a similar hyper-extended passive continental margin is provided by the modern Grand Banks – Newfoundland margin, eastern Canada, that shows similar proportions and structures as the restored Neoproterozoic Moine–Dalradian margin proposed here. Using multi-channel seismic reflection profiles, Lau et al. (Reference Lau, Louden, Deemer, Hall, Hopper, Tucholke, Holbrook and Larsen2006) showed that thinned continental crust characterized by extensional faults extends at least 150 km offshore the Newfoundland shelf margin, with 50–60 km of ‘transitional crust’ outboard, before oceanic crust appears some 200+ km offshore. A restoration of the Moine and Dalradian structures (Fig. 3) would reveal a passive continental margin of similar proportions to the modern-day Grand Banks – Newfoundland margin.
10.b. Location of the Iapetus suture: role of the Highland Boundary fault
Suture zones separating tectonic plates are rarely narrow faults, and are usually zones containing rocks that formed within the intervening ocean, notably ophiolites and deep-sea sedimentary rocks, as well as high-pressure blueschists and (ultra)high-pressure eclogites, and frequently post-collision molasse basins. Sometimes, island arcs are preserved within suture zones, such as the Late Cretaceous – Eocene Kohistan island arc, sandwiched between the Indian and Asian plates in the Indus–Tsangpo suture zone (Fig. 10b). Most of these rock types occur along both the Midland Valley and Southern Uplands terranes of Scotland, suggesting that the Iapetus suture can be delineated as the terrane south of the HBF and north of the English Avalon terrane. This definition of the Iapetus suture includes the buried Midland Valley island arc and the ophiolite complexes to the north and south (Tanner, Reference Tanner, Corfu, Gasser and Chew2014; Chew & Strachan, Reference Chew, Strachan, Corfu, Gasser and Chew2014). This interpretation is supported by Rayleigh wave tomographic imaging across the British Isles, which shows a major change across the Highland Boundary, abruptly separating high velocities across the Scottish Highlands (Moine and Dalradian) from low velocities across the Midland Valley (Nicolson et al. Reference Nicolson, Curtis and Baptie2014). Deep crustal xenoliths in Carboniferous–Permian volcanic rocks in the Midland Valley show evidence of a buried Late Ordovician arc beneath the suture zone and also evidence of buried Late Silurian – Devonian ‘Newer Granites’ (Badenszki et al. Reference Badenszki, Daly, Whitehouse, Kronz, Upton and Horstwood2019). This same suture zone can be traced across to northern Ireland (Clew Bay zone; Dewey & Shackleton, Reference Dewey and Shackleton1984; Chew et al. Reference Chew, Daly, Magna, Page, Kirkland, Whitehouse and Lam2010; Chew & Strachan, Reference Chew, Strachan, Corfu, Gasser and Chew2014; Dewey et al. Reference Dewey, Dalziel, Reavy and Strachan2015) and central Newfoundland (Baie Verte oceanic track to the Dover Fault; van Staal et al. Reference van Staal, Barr and Murphy2012), but it cannot be traced to the north, where it must lie somewhere beneath the Atlantic, east of Greenland and west of Norway.
10.c. Role of the Great Glen fault
The GGF is critical to models of different evolution for the Northern Highlands Moine and the Dalradian Grampian terranes. Offset and timing of slip along the GGF are poorly known, as there are no definitive pinning points on either side of the fault. In the Dewey & Shackleton (Reference Dewey and Shackleton1984), Dewey & Strachan (Reference Dewey and Strachan2003) and Dewey et al. (Reference Dewey, Dalziel, Reavy and Strachan2015) model, the GGF has 500–700 km of sinistral displacement in late Scandian time, possibly as much as 1200 km when combined with estimated slip along other strike-slip faults (Dewey & Strachan, Reference Dewey and Strachan2003). This large-scale orogen-parallel strike-slip motion was required in order for the Grampian terrane to escape Scandian metamorphism and deformation. However, although there are, as yet, no Scandian-age U–Pb monazite or Sm–Nd, Lu–Hf garnet dates from the Dalradian rocks, there is abundant evidence of crustally derived S-type granites in the Grampian Highlands (Oliver et al. Reference Oliver, Wilde and Wan2008) during this time.
The model of large-scale strike-slip motion along the GGF is partly based partly on the presumed Silurian – Early Devonian age of sinistral fabrics affecting the Strontian granite (425 ± 3 Ma; Rogers & Dunning, Reference Rogers and Dunning1991), the Clunes tonalite (427.8 ± 1.9 Ma; Stewart et al. Reference Stewart, Strachan, Martin and Holdsworth2001) and the Glenelg–Ratagain granite (425 ± 3 Ma; Rogers & Dunning, Reference Rogers and Dunning1991; Hutton & McErlean, Reference Hutton and McErlean1991). The U–Pb zircon ages of the granites clearly date the crystallization and emplacement age of the granite, but they only give a maximum possible age of the fabrics that were imposed after crystallization and during shearing. The high-temperature fabrics could have formed soon after the granite emplacement, but they could also have been superimposed much later. There are no definitive Caledonian pinning points either side of the GGF. Some models have also linked the GGF to the WBF on the Shetland Islands (Flinn, Reference Flinn1992; Dewey & Strachan, Reference Dewey and Strachan2003; Dewey et al. Reference Dewey, Dalziel, Reavy and Strachan2015), but seismic data in the Moray Firth and offshore Shetland show that the two faults are not linked. The Carboniferous age of dextral motion along the WBF in Shetland (Armitage et al. Reference Armitage, Watts, Holdsworth and Strachan2021) is also incompatible with the presumed Silurian syn-granite shearing (Hutton & McErlean, Reference Hutton and McErlean1991; Hutton & Reavy, Reference Hutton and Reavy1992) proposed for the GGF.
Similar discussions on syn- and post-emplacement shearing fabrics of granites along major strike-slip faults in Tibet have occurred, notably along the right-lateral Karakoram Fault (Phillips & Searle, Reference Phillips and Searle2007; Searle & Phillips, Reference Searle and Phillips2007), and the left-lateral Red River fault in Yunnan and Vietnam (Searle et al. Reference Searle, Yeh, Lin and Chung2010 b). In both cases, shear fabrics along the ductile strike-slip fault were superimposed after initial crystallization of the leucogranites, so that the U–Pb zircon ages of the granites only provide a maximum age of shearing. It is suggested that the Strontian, Clunes and Ratagain granites were all emplaced in the Northern Highlands (Moine) terrane during late Silurian time, along with all the other ‘Newer Granites’ in the Grampian terrane, and the ductile fabrics were superimposed during high-temperature deformation sometime after 415 Ma, and before deposition of the overlying Old Red Sandstone at ∼405 Ma. The amount of offsets and early motion of slip along the GGF remains highly speculative. Offsets of c. 100 km could be interpreted from the offset of the Strontian granite along the NW side of the GGF, and the Foyers granite in the Dalradian to the SE, as originally suggested by Kennedy (Reference Kennedy1948). Both granites have similar ranges of compositions, and they also have similar U–Pb zircon ages, of 425 ± 3 Ma and 424 ± 4 Ma, respectively (Rogers & Dunning, Reference Rogers and Dunning1991; Oliver et al. Reference Oliver, Wilde and Wan2008).
10.d. Grampian: Scandian metamorphism, one event or two?
Peak metamorphic ages in both the Moine and Dalradian supergroups started at ∼475 Ma indicating that continental collision, immediately following accretion of the Midland Valley arc–microcontinent, was at least of this age. Both Moine and Dalradian rocks were subjected to compressional folding, ductile thrusting and MP–HT metamorphism from 475 Ma to at least 430–420 Ma (Fig. 4). The older S-type granites in the Grampian terrane (e.g. Auchlea, Aberdeen granites) have similar ages to those of the gabbros (e.g. Inch, Portsoy gabbros) in the Buchan zone (Oliver et al. Reference Oliver, Wilde and Wan2008). Previous interpretations of two short-lived orogenies, the Ordovician Grampian and Silurian Scandian, were based on sparce, early geochronological data. The span of ages relating to peak metamorphism (Lu–Hf, Sm–Nd garnet; U–Pb monazite) as well the dates of crustal melt granites across the Grampian Dalradian series and the Northern Highlands Moine series overlap (Fig. 4), ranging from ∼470 to 420 Ma (Rogers et al. Reference Rogers, Hyslop, Strachan, Paterson and Holdsworth1998; Kinny et al. Reference Kinny, Friend, Strachan, Watt and Burns1999; Baxter et al. Reference Baxter, Ague and DePaolo2002; Oliver et al. Reference Oliver, Wilde and Wan2008; Bird et al. Reference Bird, Thirlwall, Strachan and Manning2013; Mako et al. Reference Mako, Law, Caddick, Thigpen, Ashley, Cottle and Kylander-Clark2019). It is suggested here that these data require one semi-continuous, long-lived crustal thickening and regional metamorphic event, rather than two separate events. Metamorphic facies in the Northern Highlands Moine Supergroup are similar to those in the Barrovian part of the Dalradian Supergroup. Buchan metamorphism is restricted to the NE part of the Dalradian terrane and is spatially and temporally related to intrusion of gabbroic rocks, such as the Early Ordovician Portsoy gabbros (Fettes, Reference Fettes1970; Harte, Reference Harte, Harris and Fettes1988; Johnson et al. Reference Johnson, Kirkland, Reddy, Evans and McDonald2016). Buchan metamorphism occurred during the late stages of ophiolite obduction and was a precursor to, and then continuous with, Barrovian kyanite-grade metamorphism, which overprinted early lower pressure andalusite-bearing assemblages (Harte & Hudson, Reference Harte, Hudson, Harris, Holland and Leake1979). Compressional folds, thrusts and the regionally extensive metamorphism in both the Dalradian and Moine terranes suggest that post-accretionary and syn-collisional regional crustal thickening was the dominant cause of metamorphism, and not lithospheric extension as suggested by Viete et al. (Reference Viete, Richards, Lister, Oliver, Banks, Law, Butler, Holdsworth, Krabbendam and Strachan2010, Reference Viete, Oliver, Fraser, Forster and Lister2013) and Viete & Lister (Reference Viete and Lister2016). Pre-, syn- and post-metamorphic folding is widely documented across the Northern Highlands Moine and Grampian Highlands Dalradian supergroups, testifying to continuous compression during Ordovician and Silurian times.
10.e. Alkaline and calc-alkaline magmatism
A further similarity between the Moine and Dalradian rocks is the younger post-collision, late orogenic, Late Silurian – Early Devonian ‘Newer Granites’. Locations of the major late orogenic, Silurian–Devonian granite plutons across the Scottish Highlands, together with their U–Pb dates are shown in Figure 8. It is noteworthy that these granites span all terranes from the Laurentian foreland and Moine thrust zone, across the Moine and Dalradian terranes to the Southern Uplands and the northern part of the Avalonia terrane in northern England. They cannot therefore be related to any NW-dipping slab break-off model (Atherton & Ghani, Reference Atherton and Ghani2002), otherwise they would be restricted to the Moine and Dalradian terranes. They are spatially and temporally related to appinites and lamprophyres, and also do not show a typical linear batholith-like outcrop pattern as seen along the Andes. Inherited zircon ages of both Laxfordian (1.8–1.6 Ga) and Grenvillian (c. 1.0 Ga) show multiple source rocks and periods of reworked or ‘re-magmatized’ granites (Chappell & Stephens, Reference Chappell and Stephens1988).
These late orogenic granites, intruded between ∼430 and 405 Ma (Oliver et al. Reference Oliver, Wilde and Wan2008; Fowler et al. Reference Fowler, Kocks, Darbyshire and Greenwood2008; Neilson et al. Reference Neilson, Kokelaar and Crowley2009; Miles et al. Reference Miles, Woodcock and Hawkesworth2016), are associated with an up to 2 km thick pile of andesite–trachyte volcanic rocks (Lorn lavas, Etive dyke swarm), with contemporaneous emplacement of shoshonite and lamprophyre dykes in the Grampian terrane (Kokelaar & Moore, Reference Kokelaar and Moore2006; Neilson et al. Reference Neilson, Kokelaar and Crowley2009). Similar late Silurian granites intrude the Moine Supergroup north of the GGF, notably the Strontian, Loch Linne, Ratagain and Clunes granites and tonalites. These granites are not geochemically similar to Andean granites (Pitcher & Berger, Reference Pitcher and Berger1972; Pitcher, Reference Pitcher1972; Atherton & Ghani, Reference Atherton and Ghani2002; Neilson et al. Reference Neilson, Kokelaar and Crowley2009), and were intruded some 30–35 m.y. after initial terrane accretion and continental collision (Miles et al. Reference Miles, Woodcock and Hawkesworth2016). They are associated more with alkaline appinite, shoshonite and lamprophyre intrusions, similar to magmatism seen today on the Tibetan plateau (Chung et al. Reference Chung, Chu, Zhang, Xie, Lo, Lee, Lan, Li, Zhang and Wang2005). The Glen Dessary syenite (location 9 on Fig. 8; U–Pb age 445.3 ± 1.9 Ma; Goodenough et al. Reference Goodenough, Millar, Strachan, Krabbendam and Evans2011) and the Ratagain monzogranite (location 8 on Fig. 8; U–Pb age 425 ± 3 Ma; Rogers & Dunning, Reference Rogers and Dunning1991) are both alkaline intrusions, indicating a wide extent of this ‘intra-plate’ type magmatism related to melting of enriched lithospheric mantle (Halliday et al. Reference Halliday, Aftalion, Parsons, Dickin and Johnson1987) during early–middle Silurian times. Many granites of the Cairngorm suite in the Grampian Highlands (Dalradian Supergroup) have A-type geochemical affinities (Stephens & Halliday, Reference Stephens and Halliday1984). The Silurian – Early Devonian granites and syenites in the Scottish Highlands are best explained by continuous magmatic underplating and melting of heterogeneous mafic lower crust, rather than any subduction–slab break-off or delamination model. The granites and syenites do not require an Andean-type subduction zone in order to explain their geochemistry (Atherton & Ghani, Reference Atherton and Ghani2002; Neilson et al. Reference Neilson, Kokelaar and Crowley2009), and their timing is at least 30 m.y. later than the timing of Iapetus subduction (Miles et al. Reference Miles, Woodcock and Hawkesworth2016). The high Ba and Sr granites in the Argyll suite and Northern Highlands ‘Newer Granites’ are not typical subduction-related granites, and their geochemistry and isotopic compositions show no geographical trend away from the trench (Halliday et al. Reference Halliday, Stephens, Hunter, Menzies, Dicken and Hamilton1985).
Granites and syenogranites emplaced in the Moine and Dalradian series have similar ages as the large alkaline syenitic complexes along the Laurentian foreland, such as the Canisp porphyry (location 4 on Fig. 8), the Loch Borralan and Loch Ailsh intrusions (locations 5 and 6 on Fig. 8) in the Moine thrust zone, Assynt and the Loch Loyal syenite (location 7 on Fig. 8) in the Moine Supergroup (Searle et al. Reference Searle, Law, Dewey, Streule, Law, Butler, Holdsworth, Krabbendam and Strachan2010 a; Goodenough et al. Reference Goodenough, Millar, Strachan, Krabbendam and Evans2011; Fox & Searle, Reference Fox and Searle2021). Therefore, it is suggested that this Silurian alkaline intrusive region was far more extensive than previously thought, and is linked temporally and spatially to intrusion of the ‘Newer Granites’ across the Moine and Dalradian zones, which extends right the way across all the Caledonian orogenic terranes from the Laurentian foreland to the Midland Valley, and even across to the northern England Avalonia plate (Trans-suture granite suite).
10.f. Timing of the Moine thrust zone thrusting
The timing of shearing along the Moine thrust zone is constrained by the U–Pb zircon 431–429 Ma dates on the two large syenite intrusions that lie between, and are cut by, prominent thrusts (Goodenough et al. Reference Goodenough, Millar, Strachan, Krabbendam and Evans2011; Fox & Searle, Reference Fox and Searle2021). The Loch Ailsh intrusion is cut by the Moine thrust above and carried on the Ben More thrust below (Elliott & Johnson, Reference Elliott and Johnson1980; Coward, Reference Coward1985; Searle et al. Reference Searle, Law, Dewey, Streule, Law, Butler, Holdsworth, Krabbendam and Strachan2010 a). Peralkaline micro-syenite (nordmarkite) dykes are numerous within the Ben More thrust sheet, and one of them cuts across mylonites along the Moine thrust zone east of Loch Ailsh (Law et al. Reference Law, Casey and Knipe1986), but does not appear to intrude across up into non-mylonitized Moine schists. 40Ar–39Ar cooling ages of Moine mylonites of 430–420 Ma record the exhumation of the mylonites (Freeman et al. Reference Freeman, Butler, Cliff and Rex1998), and probably date the transition from ductile to brittle deformation in the Moine thrust sheet. Surprisingly, U–Pb monazite dates from the Moine schists above the Moine thrust, interpreted as dating high-temperature metamorphism, continue until 420 Ma (Mako et al. Reference Mako, Law, Caddick, Thigpen, Ashley, Cottle and Kylander-Clark2019), suggesting some out-of-sequence motion to account for the younger ages of peak metamorphism in the Moine and Ben Hope thrust sheets above.
10.g. Southern Uplands: SE-vergent accretionary prism or backthrust zone?
The Southern Uplands has been interpreted as a classic example of a subduction–accretionary wedge. This is a series of NW-dipping imbricate thrust faults cut through a complete Middle Ordovician to middle Silurian (∼458–425 Ma) sequence of southward-younging deep-water turbidites (McKerrow et al. Reference McKerrow, Leggett and Eales1977; Leggett et al. Reference Leggett, McKerrow and Eales1979, Reference Leggett, McKerrow and Soper1983; Dewey & Mange Reference Dewey, Mange, MacNiocaill and Ryan1999; Dewey et al. Reference Dewey, Dalziel, Reavy and Strachan2015). Dewey (Reference Dewey1969, Reference Dewey1971) and Dewey et al. (Reference Dewey, Dalziel, Reavy and Strachan2015) proposed a flip in subduction polarity from SE-dipping beneath the Midland Valley (ophiolite obduction) to NW-dipping beneath the Grampian terrane at ∼456 Ma (N. gracilis graptolite zone). The source of the turbiditic sedimentary rocks was the Grampian terrane and the Laurentian basement to the NW (Dewey & Mange Reference Dewey, Mange, MacNiocaill and Ryan1999; Waldron et al. Reference Waldron, Floyd, Simonetti and Hearman2008), suggesting they were likely the deeper water sediments deposited outboard of the shallow shelf sediments of the Laurentian foreland and Grampian Highlands (Trossachs Group, Highland Border complex). It would appear that the major structural break lies along the Midland Valley and Southern Uplands terranes, rather than the GGF. It remains difficult to reconcile the age of sedimentation along the Southern Uplands with Middle Ordovician – Silurian crustal thickening and regional metamorphism in the Northern Highlands (Moine) and Grampian Highlands (Dalradian) terranes. It is possible that the Midland Valley terrane was a microcontinent rather than simply a volcanic arc, and the Laurentia–Midland Valley continental collision during Early Ordovician time was the cause of crustal thickening and regional metamorphism along the Laurentian margin. The Southern Uplands terrane remained an oceanic realm to the south of the Midland Valley microcontinent, and had little effect on the tectonic evolution of the Moine or Dalradian rocks.
10.h. Scottish Caledonides: Himalaya comparison
Figure 11a shows a generalized cross-section of the Scottish Caledonides across the Laurentian foreland, Moine thrust zone, Northern Highlands and Grampian Highlands terranes to the Highland Border and the Midland Valley, combining data from Figure 6a across the Moine Supergroup (Strachan et al. Reference Strachan, Harris, Fettes, Smith and Trewin2002) and Figure 7a across the Dalradian Supergroup (Leslie et al. Reference Leslie, Smith, Soper, Higgins, Gilotti and Smith2008). Figure 11b shows a cross-section across the Cenozoic Himalayan orogenic belt, from Searle (Reference Searle and Watts2015). The two orogenic belts show many similarities in geometry and scale. Both show a tapering orogenic wedge with the youngest thrusts in the foreland (Sole thrust in Moine thrust zone; Main Boundary thrust in the Himalaya). Both orogenic belts thicken towards the hinterland with a regional metamorphic core (Moine, Dalradian metamorphic rocks; Greater Himalayan Sequence). Both show an inverted metamorphic field gradient placing hotter rocks over colder rocks as seen in the metamorphic rocks above the Moine Thrust (Thigpen et al. Reference Thigpen, Law, Lloyd and Brown2010a,b; Ashley et al. Reference Ashley, Thigpen and Law2015; Mazza et al. Reference Mazza, Mako, Law, Caddick, Krabbendam and Cottle2018; Mako et al. Reference Mako, Law, Caddick, Thigpen, Ashley, Cottle and Kylander-Clark2019) and the metamorphic rocks along the Main Central Thrust zone in the Himalaya (Searle et al. Reference Searle, Law, Godin, Larsen, Streule, Cottle and Jessup2008, Reference Searle, Law, Dewey, Streule, Law, Butler, Holdsworth, Krabbendam and Strachan2010 a). Both orogenic belts show a foreland-younging metamorphic sequence spanning c. 45 m.y. Both orogenic belts show dominant foreland-directed thrusting with a steepening and then overturning towards the back of the orogenic wedge, resulting in a backthrust zone along the suture zone in the Caledonides (Midland Valley, Highland Border complex) and the Himalaya (northern part of Tethyan Himalaya and the Indus–Tsangpo suture) (Corfield & Searle, Reference Corfield, Searle, Khan, Treloar, Searle and Jan2000). Both show post-orogenic continental sandstones and conglomerates (molasse) deposited in a hinterland basin. In the Scottish Caledonides, the Old Red Sandstone unconformably overlies the Moine, Dalradian and Midland Valley sequences, and in the Himalaya the Indus Group molasse overlies the north Indian shelf sequence and Indus–Tsangpo suture zone. Both zones have been affected by oversteepening and backthrusting during the late stages of orogeny, a geometry similar also to that seen along the Alps (Insubric Line; Schmid et al. Reference Schmid, Pfiffner, Froitzheim, Schönborn and Kissling1997).
The entire orogenic time span of the Scottish Caledonides lasted ∼65 m.y. (from c. 480–415 Ma), and in the Himalaya lasted ∼55 m.y. but remains ongoing. It is suggested that the Scottish Caledonides originated from a two-continent collision (Laurentia–Avalonia) with an intervening island arc or microcontinent (Midland Valley arc) in a fashion similar to the Cenozoic India – Dras/Kohistan arc – Asia collision, and formation of the Himalaya (Searle, Reference Searle and Watts2015). In both Caledonian and Himalayan orogenies, ophiolite obduction was followed immediately by arc accretion, and then continental collision. In both the Caledonian and Himalayan orogenies, metamorphism was dominantly post-collisional and not related to any pre-collision, hot, back-arc extension, as for example in the Rocky Mountain belt comparison (Hyndman, Reference Hyndman2019).
Aspects of the Scottish Caledonides that do not fit a Himalayan-type model are (1) the evolution of granite petrogenesis (lack of late orogenic I-type or A-type granites across the Himalaya), (2) the gabbroic intrusions and Buchan metamorphism in the early stage of Caledonian metamorphism are missing in the Himalaya, and (3) the NW-dipping accretionary prism structure in Ordovician – early Silurian oceanic rocks of the Southern Uplands (McKerrow et al. Reference McKerrow, Leggett and Eales1977; Dewey & Mange, Reference Dewey, Mange, MacNiocaill and Ryan1999). Crustal melt S-type granites in the Grampian Highlands span c. 475–435 Ma (Oliver et al. Reference Oliver, Wilde and Wan2008), and Caledonian ‘Newer Granites’ are generally later, between c. 435 and 400 Ma (Fig. 4). The change has previously been thought to be related to a flip in subduction direction and initiation of NW-dipping subduction making the Silurian Scandian metamorphism approximating to an Andean-type analogue. Although the Andes are associated with widespread I-type magmatism and volcanism, there are few A-type granites, no appinite–lamprophyre–shoshonite-type magmatism, and there is no equivalent of a regional Barrovian metamorphism as seen in the Scottish Highlands exposed in the Andes (Pitcher, Reference Pitcher1972; Pitcher & Berger, Reference Pitcher and Berger1972). Timing of granite emplacement in the Scottish Caledonides (late Silurian – Early Devonian) does not coincide with timing of supposed Iapetus subduction (Miles et al. Reference Miles, Woodcock and Hawkesworth2016). Himalayan granites are entirely syn-orogenic crustal melt S-type leucogranites with garnet, tourmaline, muscovite ± cordierite that are concomitant with sillimanite + muscovite metamorphism and muscovite breakdown (sillimanite + K-feldspar) melting at high structural levels of the Greater Himalayan Sequence (Searle et al. Reference Searle, Law, Dewey, Streule, Law, Butler, Holdsworth, Krabbendam and Strachan2010 a). Migmatites are present along the hangingwall of the Naver (+ Sgurr Beag) thrust sheet (Ashley et al. Reference Ashley, Thigpen and Law2015; Mako et al. Reference Mako, Law, Caddick, Thigpen, Ashley, Cottle and Kylander-Clark2019), but there are no large Himalayan-type leucogranites in the hinterland of the Naver thrust sheet. In the Himalaya, subduction-related I-type granites are entirely pre-collisional along the Kohistan–Ladakh–Gangdese batholith on the Asian upper plate, and are not related to any exposed regional metamorphic rocks (Searle, Reference Searle and Watts2015).
Buchan metamorphism in the NE part of the Grampian terrane is associated spatially and temporally with several early Early–Middle Ordovician gabbro and diorite intrusions such as the Portsoy gabbro dated at ∼475 Ma (Oliver et al. Reference Oliver, Wilde and Wan2008), or 486 ± 9 Ma and 493 ± 8 Ma (Johnson et al. Reference Johnson, Kirkland, Reddy, Evans and McDonald2016). The latter ages push the age of Buchan metamorphism back to latest Cambrian – earliest Ordovician, a similar timing as formation of the Unst and Ballantrae ophiolites. It is possible that gabbro intrusions and early Buchan LP–HT metamorphism was somehow related to late stages of ophiolite emplacement rather than subsequent crustal thickening processes. The transition from Buchan to Barrovian metamorphism in the Dalradian at c. 470 Ma (Fig. 4) marks the transition from ophiolite obduction to collision-related crustal thickening. Although sillimanite- and cordierite-bearing LP–HT metamorphic rocks occur at mid-crustal levels of the Greater Himalaya, they are associated with migmatites and leucogranites during the later stages of Himalayan metamorphism (Searle et al. Reference Searle, Law, Dewey, Streule, Law, Butler, Holdsworth, Krabbendam and Strachan2010 a), not with earlier gabbroic intrusions as in the Buchan zone.
One other complication is that in the Himalaya, the Indus–Tsangpo suture zone shows an abrupt change from marine to continental sedimentation at the time of India–Asia collision (∼50 Ma), whereas in Scotland marine sedimentation along the Southern Uplands apparently continues until late Silurian time (Cocks & Fortey, Reference Cocks and Fortey1982; Dewey et al. Reference Dewey, Dalziel, Reavy and Strachan2015). The structure of the Southern Uplands has traditionally been associated with a NW-dipping subduction zone along the suture zone, with an accretionary prism dipping to the NW, and younging to the SE (McKerrow et al. Reference McKerrow, Leggett and Eales1977; Leggett et al. Reference Leggett, McKerrow and Eales1979; Dewey & Mange, Reference Dewey, Mange, MacNiocaill and Ryan1999). However, the Southern Uplands is 80–100 km SSE of the ophiolite complexes along the HBF and had little to do with the Midland Valley terrane – Laurentia collision, which resulted in accretion of the arc, and then crustal thickening along the Dalradian and Moine supergroups. The Tay nappe in the SE part of the Dalradian (Shackleton, Reference Shackleton1958; Tanner, Reference Tanner2008, Reference Tanner, Corfu, Gasser and Chew2014) and the northern part of the Midland Valley terrane are the oversteepened and backthrust zones, similar to the structure seen along the hinterland of the Alps (Insubric Line) and Himalayan suture zone. It is noteworthy that in East Greenland, the northern continuation of the Scottish Caledonides, a large-scale E-dipping high-velocity slab has been inferred from receiver function analysis of broadband seismometers that is interpreted as a relict subduction zone composed of eclogitized crust (Schiffer et al. Reference Schiffer, Balling, Jacobsen, Stephenson and Nielsen2014). This would support the model preferred in this paper of a single SE-dipping subduction zone for Scotland.
11. Conclusions
The two-stage model of Caledonian orogeny in Scotland involving: (a) collision of Laurentia with an oceanic arc (Midland Valley) resulting in the Ordovician Grampian orogeny, and (b) collision of Laurentia and Baltica resulting in the Silurian Scandian orogeny (e.g. Dewey & Shackleton, Reference Dewey and Shackleton1984; Dewey & Strachan, Reference Dewey and Strachan2003; Oliver et al. Reference Oliver, Wilde and Wan2008; Dewey et al. Reference Dewey, Dalziel, Reavy and Strachan2015), is reviewed in the light of recent data, including recent U–Pb zircon and monazite ages (Kinny et al. Reference Kinny, Friend, Strachan, Watt and Burns1999, Reference Kinny, Strachan, Friend, Kocks, Rogers and Paterson2003; Oliver et al. Reference Oliver, Wilde and Wan2008; Johnson et al. Reference Johnson, Kirkland, Reddy, Evans and McDonald2016; Mako et al. Reference Mako, Law, Caddick, Thigpen, Ashley, Cottle and Kylander-Clark2019), and Sm–Nd and Lu–Hf garnet ages (Baxter et al. Reference Baxter, Ague and DePaolo2002; Cutts et al. Reference Cutts, Kinny, Strachan, Hand, Kelsey, Emery, Friend and Leslie2010; Bird et al. Reference Bird, Thirlwall, Strachan and Manning2013). These data lead to a simpler solution to explain the tectonic evolution of the Caledonian orogeny that follows a classic orogenic cycle from ophiolite obduction and arc accretion (c. 499–475 Ma), through continental collision (Midland Valley – Laurentia), regional crustal thickening and metamorphism, and crustal melting (c. 470–420 Ma). It is suggested here that the Laurentian foreland, Northern Highlands (Moine) and Grampian Highlands (Dalradian) sequences were all part of the same, conjoined, hyper-extended passive continental margin, possibly over 400 km wide (Fig. 3). The sedimentary environments of Moine and Dalradian protolith rocks and the dimensions of the restoration are very similar to modern, magma-poor, hyper-extending, rifted continental margins such as the Grand Banks – Newfoundland margin offshore eastern Canada (Lau et al. Reference Lau, Louden, Deemer, Hall, Hopper, Tucholke, Holbrook and Larsen2006). It is also suggested that the Grampian and Scandian metamorphic events were part of a single continuous prograde regional metamorphic event rather than two separate orogenies (Fig. 4). The Scottish Caledonides are interpreted therefore as a NW-vergent pro-wedge, with subduction along the Midland Valley suture zone dipping to the SE. Late-stage backfolding and backthrusting along the Tay nappe and HBF has a geometry very similar to that seen along the northern margin of the Himalaya and Indus suture zone. The Caledonian orogeny in Scotland lasted for ∼60 m.y. (from ∼475 to 415 Ma) and was an orogenic event of similar dimension and time scale as the Himalaya.
Specific conclusions from these data are the following:
-
(1) The youngest protolith sedimentary rocks in the Dalradian Supergroup are the upper Cambrian – Lower Ordovician Trossachs Group (Tanner & Sutherland, Reference Tanner and Sutherland2007). Ophiolites formed at c. 499–480 Ma were emplaced towards the NW above the Dalradian continental margin sediments. Cooling ages continuing until c. 488 Ma are interpreted as dating the obduction event (Chew et al. Reference Chew, Daly, Magna, Page, Kirkland, Whitehouse and Lam2010). Diorites and gabbros in the Buchan zone (NE part of the Dalradian) have U–Pb zircon ages of c. 493–486 Ma (Johnson et al. Reference Johnson, Kirkland, Reddy, Evans and McDonald2016), and these rocks, together with the concomitant LP–HT Buchan metamorphism, could be related to the obduction of ophiolites and the accretion of the Midland Valley arc. There is no evidence of any extension during this period. Instead, compressional tectonics (NW-vergent folds, thrusts) related to ophiolite obduction gave way to collision-related folding, thrusting and crustal thickening, leading to regional Barrovian metamorphism during Early Ordovician time.
-
(2) Sm–Nd and Lu–Hf garnet ages (Baxter et al. Reference Baxter, Ague and DePaolo2002; Cutts et al. Reference Cutts, Kinny, Strachan, Hand, Kelsey, Emery, Friend and Leslie2010; Bird et al. Reference Bird, Thirlwall, Strachan and Manning2013) and U–Pb monazite ages (Kinny et al. Reference Kinny, Friend, Strachan, Watt and Burns1999, Reference Kinny, Strachan, Friend, Kocks, Rogers and Paterson2003; Friend et al. Reference Friend, Jones and Burns2000; Mako et al. Reference Mako, Law, Caddick, Thigpen, Ashley, Cottle and Kylander-Clark2019) from the Northern Highlands terrane (Moine metamorphic rocks) span ∼475–420 Ma indicating a continuum of crustal thickening and regional metamorphism throughout this time. The ‘Grampian orogeny’ and ‘Scandian orogeny’ are both part of one continuous orogenic event, caused by collision of Laurentia with the Midland Valley arc terrane, during Early–Middle Ordovician times continuing through Silurian to Early Devonian times. There is no need to invoke two orogenic events with different collisions (first with an arc, then with Baltica). The time span of metamorphism in the Scottish Caledonides (∼475–420 Ma) is similar to the time span of regional Barrovian metamorphism of ∼40–50 m.y. known from the Himalaya.
-
(3) Dalradian metamorphic rocks in the Grampian terrane, SE of the GGF, have 470–460 Ma Lu–Hf and Sm–Nd garnet growth ages, similar to the Northern Highlands terrane, but so far lack the younger Silurian (Scandian) ages (Baxter et al. Reference Baxter, Ague and DePaolo2002; Bird et al. Reference Bird, Thirlwall, Strachan and Manning2013). However, decompression melting resulting in S-type granites, typically containing garnet, muscovite and occasional tourmaline, in the Dalradian rocks of the Grampian terrane span ∼478–435 Ma ages (Oliver, Reference Oliver2001; Oliver et al. Reference Oliver, Wilde and Wan2008), and must be related to crustal thickening and partial melting during this time. The late Silurian – Early Devonian granites spanning 430–405 Ma have high 87Sr/86Sr ratios (0.712; Pankhurst & Sutherland Reference Pankhurst, Sutherland and Sutherland1982; Harmon et al. Reference Harmon, Halliday, Clayburn and Stephens1984) indicating widespread crustal melting concomitant with regional metamorphic and magmatic ages in the Northern Highlands terrane. The Ratagain, Strontian and Clunes granites in the Northern Highlands terrane were intruded during this period, as were the Strath Naver, Ben Klibreck and Ross of Mull granites (Figs 8, 9).
-
(4) The Northern Highlands Moine rocks show many features in common with the Grampian Highlands Dalradian rocks (Figs 3, 4). Both have Proterozoic protoliths, although the younger Cambrian sediments are not present in the Moine and are shallow marine sediments deposited on a hyper-extending continental margin (Stephenson et al. Reference Stephenson, Mendum, Fettes and Leslie2013a,b). Both terranes have evidence of Neoproterozoic Knoydartian metamorphism (∼870–800 Ma; Cawood et al. Reference Cawood, Strachan, Merle, Millar, Loewy, Dalziel, Kinny, Jourdan, Nemchin and Connelly2015). Both terranes have evidence of 610–590 Ma granite intrusions, the ‘Older Granites’ of Barrow (Reference Barrow1893), including the Carn Chuinneag – Inchbae granites in the Moine, and the Ben Vuirich – Portsoy granites in the Dalradian (Oliver et al. Reference Oliver, Wilde and Wan2008). Both share a common Early–Middle Ordovician ‘Grampian’ orogenic metamorphism (Fig. 4). Both terranes also have Silurian – Early Devonian granite–syenite emplacement (Canisp, Loch Borralan, Loch Ailsh syenites, Glen Dessary syenite, Ratagain monzogranite–syenite in the foreland and Moine; Argyll and Cairngorm granites in the Dalradian). These factors all imply that the two were contiguous parts of the Laurentian passive margin separated by unknown, limited, late to post-orogenic sinistral offset, possible Late Devonian – Carboniferous limited dextral motion and minor post-Jurassic dextral strike-slip motion along the GGF.
-
(5) Metamorphic grade across the Northern Highlands terrane (Moine) increases up-structural section from the Moine thrust sheet to the Ben Hope thrust sheet, and the Naver thrust sheet sillimanite-bearing migmatites, indicating inverted metamorphism (Thigpen et al. Reference Thigpen, Law, Loehn, Strachan, Tracy, Lloyd, Roth and Brown2013; Ashley et al. Reference Ashley, Thigpen and Law2015; Mako et al. Reference Mako, Law, Caddick, Thigpen, Ashley, Cottle and Kylander-Clark2019). The inversion probably formed by thrusting hot rocks over colder rocks during late Silurian crustal shortening, rather than a Himalayan channel flow type process. Late Silurian migmatites above the Naver thrust were emplaced to the west during the period of ∼430–420 Ma. This age span coincides with motion along the Moine thrust, suggesting simultaneous, or possibly some out-of-sequence, motion along the Naver thrust (Mako et al. Reference Mako, Law, Caddick, Thigpen, Ashley, Cottle and Kylander-Clark2019; Strachan et al. Reference Strachan, Alsop, Ramezani, Frazer, Burns and Holdsworth2020).
-
(6) Intrusion of the Assynt alkaline suite (Canisp porphyry, Loch Ailsh and Borralan syenites, peralkaline rhyolite dykes and lamprophyre sills) at 431–429 Ma (U–Pb zircon ages, Goodenough et al. Reference Goodenough, Millar, Strachan, Krabbendam and Evans2011) occurred immediately prior to thrusting along the Moine thrust zone (Searle et al. Reference Searle, Law, Dewey, Streule, Law, Butler, Holdsworth, Krabbendam and Strachan2010 a; Fox & Searle, Reference Fox and Searle2021). Widespread alkaline magmatism from the stable foreland across the restored Moine thrust zone (Assynt minor intrusions including lamprophyres, peralkaline rhyolites, micro-syenites), as well as the alkaline–lamprophyric affinity of many Caledonian granites across both Moine and Dalradian metamorphic terranes, suggest a hot mantle anomaly across much of northern Scotland during Silurian time.
-
(7) Scottish granites are not similar to subduction-related Andean-type granites, either geochemically or structurally. Middle Silurian to Early Devonian magmatism (c. 430–405 Ma) occurs right across both the Northern Highlands (Moine) and Grampian Highlands (Dalradian) terranes (as well as south across the Southern Uplands and northern England), and does not intrude as linear, Andean-type batholiths. These granites are transitional from calc-alkaline to alkaline, and intrusion was not related, neither temporally nor spatially, to Andean-type subduction beneath the Northern Highlands. Late Silurian granites intruding the Moine (430–418 Ma) and the ‘Newer Granites’ intruding the Dalradian (430–405 Ma) are associated closely with appinites, lamprophyres and shoshonites, and have a distinct geochemistry with notably high Ba, Sr, Zr, Na and K, and high 87Sr/86Sr ratios (Atherton & Ghani, Reference Atherton and Ghani2002; Fowler et al. Reference Fowler, Kocks, Darbyshire and Greenwood2008; Miles et al. Reference Miles, Woodcock and Hawkesworth2016). They are more related to melting of an enriched lithospheric mantle (Halliday et al. Reference Halliday, Stephens, Hunter, Menzies, Dicken and Hamilton1985) with multiple sources and reworking of older suites (Chappell & Stephens, Reference Chappell and Stephens1988). The Silurian – Early Devonian Caledonian granites intrude across all zones from the Moine, Dalradian, Midland Valley, Southern Uplands and northern England (Avalonia) terranes, and are related to melting of hot, phlogopite-bearing, metasomatized sub-continental mantle (Stephens & Halliday, Reference Stephens and Halliday1984; Whalen & Hildebrand, Reference Whalen and Hildebrand2019).
-
(8) WNW- or W-directed thrusting along the Moine thrust zone and the Northern Highlands across a >120 km width of northern Scotland indicates regional SE-directed subduction of the Iapetus ocean beneath the Midland Valley, and underthrusting of the Laurentian plate beneath the Northern Highlands from at least 490–420 Ma. The >120 km width of the W-vergent fold and thrust system (from the Sole thrust of the Moine thrust zone to the central Grampian Highlands, Dalradian terrane) is far too great to be a backthrust zone, or ‘retro-wedge’, as previous models proposed. Alternatively, a SE-vergent backthrust zone could lie along the Tay nappe (SE part of the Dalradian), the Highland Boundary complex (Tanner & Sutherland, Reference Tanner and Sutherland2007; Leslie et al. Reference Leslie, Smith, Soper, Higgins, Gilotti and Smith2008) and the Midland Valley arc, similar in location and proportions to the backthrust zones along both the Alps and the northern Himalaya and Indus–Tsangpo suture zone.
-
(9) Crustal shortening estimates across the Moine thrust zone (>80 km; Elliott & Johnson, Reference Elliott and Johnson1980), Northern Highlands Moine (>110 km) and Grampian Highlands Dalradian (∼100 km) during the period 470–420 Ma are minimum crustal shortening values. The Devonian Old Red Sandstone was deposited unconformably across the Northern Highlands, Grampian Highlands and Midland Valley terranes from ∼410–400 Ma to at least 380 Ma, a similar timing to that in East Greenland, marking the ending of the Caledonian orogeny in Scotland. Acadian deformation is regarded as post-Caledonian and unrelated to the Ordovician–Silurian Caledonian orogeny sensu stricto (Thirlwall, Reference Thirlwall1988; Woodcock et al. Reference Woodcock, Soper and Miles2019).
-
(10) The GGF dividing the Northern Highlands terrane (Moine) from the Grampian terrane (Dalradian) is not a plate boundary, and has an unknown, but limited amount, of post-Scandian sinistral offset. U–Pb zircon ages of granites along the fault, and related parallel faults, including the Glenelg–Ratagain monzogranite (425 ± 3 Ma; Rogers & Dunning, Reference Rogers and Dunning1991), Strontian (425 ± 3 Ma; Rogers & Dunning Reference Rogers and Dunning1991) and the Clunes tonalite (∼428 Ma; Stewart et al. Reference Stewart, Strachan, Martin and Holdsworth2001), date the intrusion of the granite, only providing a maximum age of sinistral shearing along the fault. Magmatic and deformation fabrics in the igneous bodies swing into alignment with the fault approaching the fault contact, clearly showing a later imprint, some unknown time after igneous crystallization and intrusion. Brittle slip along the GGF also offsets post-Caledonian Devonian Old Red Sandstone and Jurassic rocks. The GGF does not connect north to the WBF in the Shetland Islands, based on offshore seismic profiles. Sinistral late Caledonian offsets along the GGF remain unknown, but were probably relatively minor. Post-Caledonian (< ∼400 Ma) dextral offsets during Late Devonian – Carboniferous times and even younger post-Jurassic–Cretaceous offsets were also minor.
-
(11) Timings of major tectonic events in Scotland have many similarities to those in East Greenland (Strachan et al. Reference Strachan, Nutman and Friederichsen1995; Higgins & Leslie, Reference Higgins and Leslie2000; Watt et al. Reference Watt, Kinny and Friderichsen2000; Leslie & Nutman, Reference Leslie and Nutman2003; Gilotti et al. Reference Gilotti, Jones, Elvevold, Higgins, Gilotti and Smith2008; Leslie et al. Reference Leslie, Smith, Soper, Higgins, Gilotti and Smith2008; Corfu & Hartz, Reference Corfu and Hartz2011). The Moine thrust is a similar structure and shows a similar timing to the Caledonian sole thrust of East Greenland (Leslie et al. Reference Leslie, Smith, Soper, Higgins, Gilotti and Smith2008). A fossil E-dipping subduction zone is present beneath East Greenland (Schiffer et al. Reference Schiffer, Balling, Jacobsen, Stephenson and Nielsen2014), supporting the model of a single SE-dipping subduction zone in Scotland.
-
(12) Timings of major tectonic events in Scotland have little similarity with those of Baltica, in Western Norway. Norway shows long periods (∼70 m.y) of ultrahigh-pressure metamorphism and deep crustal W-dipping subduction, spanning 478–450 Ma (upper allochthon), 470–430 Ma (middle allochthon) and 420–405 Ma (lower allochthon; Western Gneiss region) (e.g. Terry et al. Reference Terry, Robinson, Hamilton and Jercinovic2000; Hacker & Gans, Reference Hacker and Gans2005; Kylander-Clark et al. Reference Kylander-Clark, Hacker, Johnson, Beard, Mahlen and Lapen2007; Gordon et al. Reference Gordon, Whitney, Teyssier, Fossen and Kylander-Clark2016; Jamtveit et al. Reference Jamtveit, Moulas, Andersen, Austrheim, Corfu, Petley-Ragan and Schmalhotz2018). Subduction direction was consistently towards the west throughout Ordovician to Early Devonian times (Andersen et al. Reference Andersen, Jamtveit, Dewey and Swensson1991), opposite to the proposed SE-directed subduction in Scotland and East Greenland. The long-lasting ultrahigh-pressure metamorphism in Western Norway reflects continuous, W-directed continental subduction along the margin, without any of the typical Andean or Tibetan-type geology exposed, as would be expected if Baltica had collided with Laurentia–Avalonia.
Acknowledgements
I thank the reviewers, Peter Cawood, Graham Leslie, and especially Rob Strachan for their very detailed reviews, and suggestions which greatly improved the paper. I especially thank them for not wholly rejecting my sometimes heretical new model for the Scottish Caledonian orogeny, and fully acknowledge that they may not be entirely in agreement with several of my conclusions. Thanks also to Rick Law, John Dewey, Chris Hawkesworth, Ed Stephens, Marc St-Onge, Bruce Levell and Tony Watts for many animated discussions on Scottish geology. Thanks also to David Sansom for quality drafting, and editor Olivier Lacombe for his patience.