Rivers are among the world's most important ecosystems, sustaining biodiversity and providing countless services, including some of the largest fisheries globally. They link vast landscapes through the delivery of water, sediment, organisms, and nutrients to floodplains, deltas, and coastal regions, benefiting billions of people (Lynch et al., Reference Lynch, Cooke, Deines, Bower, Bunnell, Cowx, Nguyen, Nohner, Phouthavong, Riley, Rogers, Taylor, Youn and Beard2016). The ability of rivers to sustain biodiversity and deliver many ecosystem services is governed by the degree to which their natural flow regime and connectivity are maintained. Defining free-flowing rivers (FFRs) as page 216: ‘rivers where ecosystem functions and services are largely unaffected by changes to fluvial connectivity,’ Grill et al. (Reference Grill, Lehner, Thieme, Geenen, Tickner, Antonelli, Babu, Borrelli, Cheng, Crochetiere, Macedo, Filgueiras, Goichot, Higgins, Hogan, Lip, McClain, Meng, Mulligan and Zarfl2019) mapped river connectivity across the globe. Fluvial connectivity is defined to extend in four dimensions: longitudinally (up- and downstream in the river channel), laterally (between main channel, floodplain, and riparian areas), vertically (between groundwater, river, and atmosphere) and temporally (natural flows that include seasonal variations, transport of sediment, and other organic materials) (Grill et al., Reference Grill, Lehner, Thieme, Geenen, Tickner, Antonelli, Babu, Borrelli, Cheng, Crochetiere, Macedo, Filgueiras, Goichot, Higgins, Hogan, Lip, McClain, Meng, Mulligan and Zarfl2019). They found that river connectivity has declined dramatically and that long FFRs (>1,000 km) are rare outside of the Arctic region and Amazon and Congo Basins.
Reconciling dams with freshwater system health remains one of the world's greatest conservation challenges and is fundamental to the maintenance and recovery of freshwater biodiversity and vital ecosystem services (Tickner et al., Reference Tickner, Opperman, Abell, Acreman, Arthington, Bunn, Cooke, Dalton, Darwall, Edwards, Harrison, Hughes, Jones, Leclère, Lynch, Leonard, McClain, Muruven, Olden and Young2020b). There has been a long history of conflicts, studies, and debate over how to both protect rivers and develop them sustainably. The World Commission on Dams (2000) brought together diverse perspectives and stakeholders to advance the dialogue, and while the political, economic, and social context has evolved since then and some progress has been made, the debate has continued (Schulz & Adams, Reference Schulz and Adams2019). Dam construction during the past century is the primary cause of decline in river connectivity and number of FFRs (Grill et al., Reference Grill, Lehner, Thieme, Geenen, Tickner, Antonelli, Babu, Borrelli, Cheng, Crochetiere, Macedo, Filgueiras, Goichot, Higgins, Hogan, Lip, McClain, Meng, Mulligan and Zarfl2019). Worldwide, there are an estimated >58,000 large dams (ICOLD, 2017). Data on smaller dams and barriers are scarce, but likely amount to several millions (Couto & Olden, Reference Couto and Olden2018; Lehner et al., Reference Lehner, Liermann, Revenga, Vörösmarty, Fekete, Crouzet, Döll, Endejan, Frenken, Magome, Nilsson, Robertson, Rödel, Sindorf and Wisser2011). Dams and associated reservoirs have historically been a cornerstone of economic development in numerous countries, and they provide many benefits – including water supply for human populations and irrigated agriculture, flood risk reduction, and hydropower production. However, dams also fragment rivers, inundate habitat, disrupt sediment and nutrient transport, alter flow regimes, and block migration and dispersal routes for numerous species and can be a source of social and geopolitical conflict. Alterations of water and sediment flows can affect the shape of river beds, which can in turn trigger increased vulnerability to floods and droughts, especially in densely populated large tropical deltas (Edmonds et al., Reference Edmonds, Caldwell, Brondizio and Siani2020). While hydropower dams are also often seen as a low-carbon source of energy, concerns have been raised about their greenhouse gas emissions, particularly in the tropics (Abril et al., Reference Abril, Guérin, Richard, Delmas, Galy-Lacaux, Gosse, Tremblay, Varfalvy, M. A. and Matvienko2005; de Faria et al., Reference de Faria, Jaramillo, Sawakuchi, Richey and Barros2015; Keller et al., Reference Keller, Marcé, Obrador and Koschorreck2021). The proliferation of dams has been a major factor in the continuing decline of aquatic biodiversity and inland fisheries, and has worsened the sinking of deltas, which host half of the world's mega-cities, and erosion of river banks and coasts due to upstream sediment retention (Anthony et al., Reference Anthony, Brunier, Besset, Goichot, Dussouillez and Nguyen2015; Dias et al., Reference Dias, Tedesco, Hugueny, Jézéquel, Beauchard, Brosse and Oberdorff2017). An estimated 40–80 million people have been directly displaced by dams and a potentially even higher number of river-dependent people have been impacted downstream of dams by changes to flow patterns, floodplain agriculture, and fisheries (Richter et al., Reference Richter, Postel, Revenga, Scudder, Lehner, Churchill and Chow2010; World Commission on Dams, 2000). Recent trends, suggest that dam development is slowing (IEA, 2020). The unfolding economic crisis resulting from the coronavirus pandemic is likely to further perpetuate this trend at least in the near term. The current situation provides an opening to rethink future and existing dams and ensure that the full suite of development options and trade-offs across energy, food, water, and ecosystems are better considered.
1. An uncertain future for rivers
Countries that ratified the sustainable development goals (SDGs) and the Convention on Biological Diversity have committed to halting global declines in freshwater biodiversity and ecosystem services. This effort requires policy and management solutions that balance a range of resource management objectives (water supply, irrigation, and energy) with climate resilience and ecosystem conservation and restoration. Recognizing that all water and energy development options have some level of social and environmental impact, achieving this balance necessitates more strategic decision-making that fully considers the long-term costs and benefits across a range of possible development or management scenarios. In many parts of the world, planning processes and social and environmental impact assessments for dams have been largely performed at the individual project level (Hanna et al., Reference Hanna, Vanclay, Langdon and Arts2014; Vanclay, Reference Vanclay2020). Recognizing the limitations of this approach, strategic or regional impact assessments have become more common (Fundingsland Tetlow & Hanusch, Reference Fundingsland Tetlow and Hanusch2012). Even so, many regulatory frameworks do not require assessments of the collective social and environmental impacts and trade-offs from multiple dams and associated operations. These assessments also often lack serious consideration of alternative development options that may be less impactful, or they are undertaken pro-forma to meet legislative requirements but not rigorously or with the intent of implementation (e.g. IUCN, 2019). At the same time, global climate change, energy, food and water commitments through the SDGs and the Paris Agreement may provide renewed momentum to increase dam development. While all types of dams affect river connectivity, hydropower dams are a primary threat to long FFRs and they are a critical component of energy scenario planning to meet SDG 7 while staying within climate limits (IEA, 2019; Teske, Reference Teske2019; Winemiller et al., Reference Winemiller, McIntyre, Castello, Fluet-Chouinard, Giarrizzo, Nam, Baird, Darwall, Lujan, Harrison, Stiassny, Silvano, Fitzgerald, Pelicice, Agostinho, Gomes, Albert, Baran, Petrere and Sáenz2016).
More than 1.6 M kilometers of rivers worldwide have lost their free-flowing status and only about one-third of rivers >1,000 km currently remain free-flowing (Grill et al., Reference Grill, Lehner, Thieme, Geenen, Tickner, Antonelli, Babu, Borrelli, Cheng, Crochetiere, Macedo, Filgueiras, Goichot, Higgins, Hogan, Lip, McClain, Meng, Mulligan and Zarfl2019). In the absence of consistent global data for other dam types, we calculated the potential future loss of FFRs from new hydropower development alone by intersecting 3,700 under-construction and proposed hydropower dams (>1 MW) (Zarfl et al., Reference Zarfl, Lumsdon, Berlekamp, Tydecks and Tockner2015) with the global river network and assessed the projected change in connectivity status. Our analysis shows that over 260,000 km of rivers, including the Amazon, Congo, Irrawaddy, and Salween mainstem rivers, would lose their free-flowing status if all these hydropower dams were built (Figure 1, Supplementary Tables S1–S3). These numbers are underestimates, as data for small dams and dams built for purposes other than hydropower are not included. Collectively, the hydropower capacity of dams proposed to be built on FFRs (1,809 dams) amounts to 212 GW, or approximately 1,114 TWh of energy generation per year (assuming a capacity factor of 60%). While there are regional variations, this level of energy generation represents less than 2% of the projected worldwide generation from renewables in 2050 necessary to maintain a global temperature increase below 1.5 °C (Teske, Reference Teske2019). Thus, proposed hydropower expansion would result in significant losses of the remaining FFRs and their globally significant biodiversity and associated ecosystem services for a relatively minor contribution toward the needed increase in renewable energy capacity.
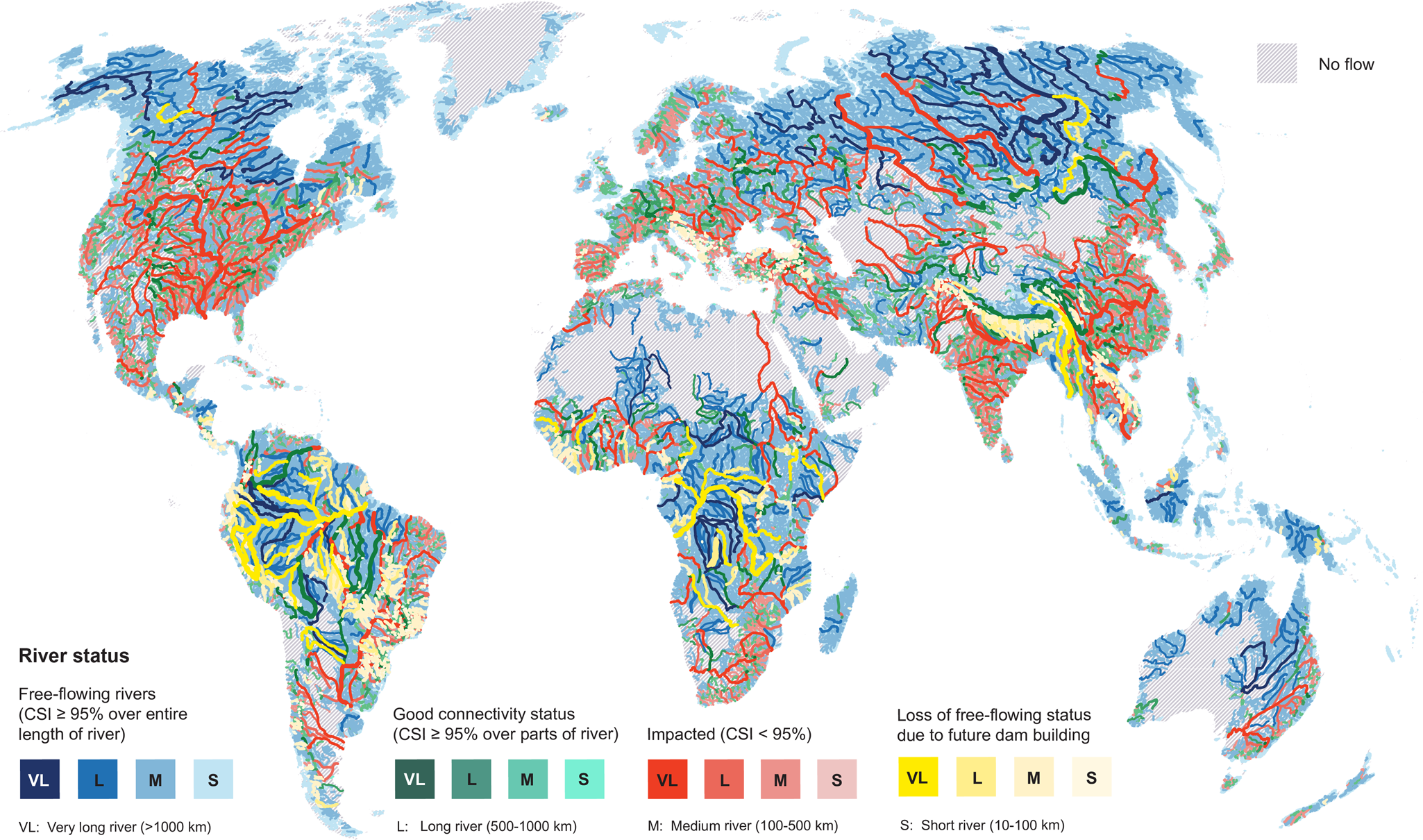
Fig. 1. Loss of FFRs. Global river connectivity status and distribution of rivers that lose their free-flowing status between current and future dam scenarios. Current dam portfolio as well as methods to calculate connectivity status and FFRs are described in Grill et al. (Reference Grill, Lehner, Thieme, Geenen, Tickner, Antonelli, Babu, Borrelli, Cheng, Crochetiere, Macedo, Filgueiras, Goichot, Higgins, Hogan, Lip, McClain, Meng, Mulligan and Zarfl2019). Dam build-out adds 3,700 hydropower dams (>1 MW capacity) (Zarfl et al., Reference Zarfl, Lumsdon, Berlekamp, Tydecks and Tockner2015). A list of rivers with status change longer than 500 km is given in Supplementary Table S3.
2. Solution pathways to navigate trade-offs of dams and river conservation
A better balance between economic development goals and sustaining healthy rivers and the communities that depend on them can be achieved through expansion of existing policy and finance mechanisms at national, regional and basin scales. Here, we provide five examples from basins around the world that demonstrate solution pathways to meet economic goals while maintaining a broad range of benefits from rivers. We frame this set of potential solutions within the mitigation hierarchy (avoid, minimize, restore, offset). Most of the examples provided focus on energy generation. However, similar or other innovative approaches that enhance design and management of dams for water storage for irrigation agriculture or municipal supply could be deployed.
2.1. Avoid
There are two significant mechanisms to avoid impacts to river ecosystems. These are avoidance through formal protection, and avoidance of immediate threats through exploration of alternative development options, including reduction of demand and nature-based solutions, which could provide less impactful options for meeting climate resilience, water, food, and energy security goals (UNEP, 2007).
2.1.1. River protections
Numerous types of protection mechanisms for high-conservation value rivers exist around the world – from inclusion of rivers within national parks to designated Ramsar sites, water resource protection areas or water reserves. Wherever rivers are designated as protected, dam construction should be avoided – although that is often not the case (Thieme et al., Reference Thieme, Khrystenko, Qin, Golden Kroner, Lehner, Pack, Tockner, Zarfl, Shahbol and Mascia2020). A widely recognized and explicit protection for rivers is the Wild and Scenic Rivers designation in the USA, which provides protection for wild, FFRs and their immediate environments with outstanding scenic, recreational, geologic, fish and wildlife, historic, cultural, or other conservation values and prohibits dam construction. As one example, the designation of the Clarks Fork of the Yellowstone River in 1990 has permanently protected the river and immediate surroundings from federally licensed dams and other development (Moir et al., Reference Moir, Thieme and Opperman2016). System-scale planning (see below) can identify specific river basins, or portions of basins, which should be prioritized for protection and that development should avoid. In Myanmar, the recent Strategic Environmental Assessment for hydropower recommended keeping about 4,100 km of mainstem rivers connected (including the last two very long FFRs in Southeast Asia, the 1,500 km Irrawaddy, and 1,200 km Salween) and protecting 10 sub-basins, covering 24% of Myanmar (IFC et al., 2018). While not a formal declaration of river protection, if implemented, these recommendations would effectively act to safeguard these rivers and basins from hydropower development. Policy interventions that affect entire rivers or basins, like the Myanmar example, are more effective than those focused on certain sections given the underlying value of water and sediment flows in shaping the ecology and health of the system (Acreman et al., Reference Acreman, Hughes, Arthington, Tickner and Dueñas2020). System-wide sediment budgets and associated protections for natural sediment source areas and flows from source to sink are also critically needed (Syvitski et al., Reference Syvitski, Vorosmarty, Kettner and Green2005; Walling, Reference Walling2006).
2.1.2. Explore alternative options
Options for new energy sources are now available for many countries as costs have declined for non-hydropower renewables and battery storage over the past decade. Hydropower may be better deployed to complement and support the deployment of low-impact wind and solar. The 2,750 MW HidroAysen project in southern Chile stalled in 2012 due to citizen protests opposing damming of the Pascua and Baker FFRs and construction of a 3,000 km transmission line. The dams were cancelled, and subsequently Chilean policy enabled the installation of 2,300 MW of solar PV and 1,300 MW of wind between 2012 and 2018, equivalent to 90% of the country's incremental energy needs during this period (Comisión Nacional de Energía, 2019; Opperman et al., Reference Opperman, Hartmann, Lambrides, Carvallo, Chapin, Baruch-Mordo, Eyler, Goichot, Harou, Hepp, Kammen, Kiesecker, Newsock, Schmitt, Thieme, Wang, Weatherby and Weber2019a). While hydropower can provide dispatchable generation that can support expansion of variable renewable energy sources, governments should seize the opportunity presented by the dramatically dropping costs of wind, solar and battery storage to develop power systems that are both low carbon and low cost and with potentially lower conflicts with communities, rivers, and other ecosystems (Aggarwal & O'Boyle, Reference Aggarwal and O'Boyle2020), especially as they build back their economies post-pandemic. Solar and wind projects have also been shown to be able to generate more jobs (IRENA, 2020; Montt et al., Reference Montt, Maître and Amo-Agyei2018) and have lower cost overruns and delays compared to hydropower (Ansar et al., Reference Ansar, Flyvbjerg, Budzier and Lunn2014; Plummer Braeckman et al., Reference Plummer Braeckman, Disselhoff and Kirchherr2020).
2.2. Minimize
2.2.1. Strategic or system-scale planning
Improved outcomes are possible through strategic planning approaches that focus on a system scale, which explore alternative development scenarios and identify combinations of projects that optimize investments for multiple environmental, social, and economic benefits (e.g. electricity generation, fisheries, and tourism). Several demonstration or proof of concept examples exist. These include modeling of hydropower expansion or reoperation in the Myitnge Basin in Myanmar, the Rufiji River in Tanzania, and the Tana River in Kenya (Geressu et al., Reference Geressu, Siderius, Harou, Kashaigili, Pettinotti and Conway2020; Hurford et al., Reference Hurford, McCartney, Harou, Dalton, Smith and Odada2020; TNC et al., 2016). The tools and capability exist to systematically bring these approaches into policy and investment planning processes, for example, through strategic social and environmental assessments or project preparation facilities.
2.2.2. Re-regulation of downstream flows
Much experience has been gained over the past decades in the mitigation of downstream flow alterations from dams. Options to minimize the effects of flow alteration include environmental flow releases (with several elements that can apply to any type of dam including minimum flows, ramp up/down rules, and the release of periodic high flows) and re-regulating reservoirs (Brown et al., Reference Brown, Zakaria, Joubert, Rafique, Murad, King, Hughes, Cardinale and Alonzo2019; King & Brown, Reference King and Brown2018; Tickner et al., Reference Tickner, Kaushal, Speed and Tharme2020a). Such reservoirs are sometimes built downstream of peaking power plants to reduce rapid flow fluctuations. For example, 20 such reservoirs exist in a US hydropower database (McManamay et al., Reference McManamay, Oigbokie, Kao and Bevelhimer2016). Examples from other countries include the Magat project in the Philippines, the Nam Ngiep 1 project in Laos, and the Cheves project in Peru. The most useful location for a re-regulating reservoir is at the bottom of a cascade of dams. In China, experiments with flow releases from Three Gorges Dam over a 5-year period have shown potential for partial mitigation of the decline in fish recruitment that followed construction of the dam. Continued monitoring, stakeholder engagement, and adaptive management will be critical to determine how successful mitigation has been, recognizing as well that this strategy only mitigates for changes in flow regime (Cheng et al., Reference Cheng, Opperman, Tickner, Speed, Guo and Chen2018).
2.3. Restore
2.3.1. Restoration of rivers through dam removal
Nearly 1,700 dams have been removed within the US and efforts are underway to continue this trend in the US, Europe and other regions (American Rivers, 2019). The Federal Power Act in Section 10(a)(1) requires the U.S. Federal Energy Regulatory Commission to give ‘equal consideration’ to river conservation and recreation alongside hydropower production (FERC, 2017). For example, using this policy, two aging dams were removed, and fish passage was improved on two other dams in the Penobscot River, beginning in 2011. As a result, migratory fish access increased by approximately 2,000 km, and numbers of river herring increased from 20,000 before dam removal to nearly 2 million in 2016. Meanwhile, a system-scale planning assessment recommended equipment and operational changes at several remaining dams. These changes were made and allowed total electricity generation from the Penobscot Basin to remain equal to the level prior to dam removal (Opperman et al., Reference Opperman, Kendy and Barrios2019b). Another example of regional efforts to reintroduce river connectivity is the EU Biodiversity strategy for 2030's goal to page 12: ‘restore at least 25,000 km of European rivers to a free-flowing state’ (European Commission, 2020).
2.4. Offset
2.4.1. Biodiversity offset
While not yet widely used for mitigation, a case study from Costa Rica provides a compelling, and replicable example for mitigation of dam impacts. A 305 MW hydropower project was completed in 2016 in the lower reach of the Reventazón River. The project was located downstream from an existing dam cascade and was expected to exacerbate declines in migratory fish species. Costa Rica's government declared the adjacent Parismina River protected in perpetuity to ensure that river connectivity and flow on a similar river were maintained in the region. This policy guaranteed that page 59: ‘artificial modifications, including dams that would block migrations, will be prohibited and that the Parismina's natural flow pattern and its biological integrity will be preserved or restored where required’ (IADB, 2015). A new protected area encompassing a 100 km long corridor and buffer zone was established and funding provided to manage and restore the river and adjacent buffer (Moir et al., Reference Moir, Thieme and Opperman2016). Other recent examples include the protection and restoration of tributaries near the Chaglla project in Peru and the Nachtigal project in Cameroon (Hartmann et al., Reference Hartmann, Smith and Khalil2015).
3. Roles of different actors in deploying solution pathways
As these examples illustrate, avoiding or reducing impacts on rivers is possible – particularly when implemented at a system or regional scale – and can be achieved while maintaining or expanding benefits for water, food, and energy security. Dam financiers, developers and operators, research agencies, civil society, academia, and government ministries all have roles to play to avoid and reduce impacts on rivers (Table 1). Governance and financing mechanisms are critical now to scale-up implementation of these solutions – particularly with the opportunity presented by post-Covid economic recovery plans. Investments in wind, solar, and hydropower upgrades and flow management, along with dam removal, can contribute to economic growth and expansion of employment through power systems that are low carbon and consistent with maintaining and restoring FFRs and the values they provide (Opperman et al., Reference Opperman, Hartmann, Lambrides, Carvallo, Chapin, Baruch-Mordo, Eyler, Goichot, Harou, Hepp, Kammen, Kiesecker, Newsock, Schmitt, Thieme, Wang, Weatherby and Weber2019a). Government planning and permitting agencies need to have the mandate and capability to implement system-wide assessments, based on the mitigation hierarchy and that account for the full range of ecosystem services that FFRs provide. At the earliest stages of planning, multiple development scenarios need to be explored for relative risks and trade-offs, including options for increasing energy and/or water-use efficiency, which can lessen the need for new infrastructure. For instance, project preparation facilities can use scenario assessments to help to identify projects that support delivery of food, water, or energy with lower financial risks and reduced negative impacts for nature and people. Analytical frameworks that assess tradeoffs across a range of stakeholder values such as energy production, cost, water storage, fisheries, irrigation, biodiversity and river transportation are already available (Hurford et al., Reference Hurford, Huskova and Harou2014). Strategic dam upgrades and/or removal options to reconnect rivers should be considered for infrastructure systems that include aging dams with limited value, high maintenance costs, or structural failure risks. Where dam projects are ultimately developed, governance frameworks, such as free prior and informed consent (FPIC) and benefit sharing mechanisms that fund interventions to minimize, restore and offset impacts, are critical to ensure equitable outcomes for affected groups, including local communities and indigenous peoples.
Table 1. Actors and their potential role

Classification of actors and their potential roles and actions toward policy solutions.
4. Conclusions
Employing an early strategic approach and deploying the mitigation hierarchy within energy and water planning can avoid or reduce many negative social and environmental impacts, balance tradeoffs, enhance financial performance, and enable countries to reap the benefits of development. The examples featured here provide evidence of a variety of solutions that can contribute to meeting climate, food, and energy development goals while safeguarding FFRs to ensure a balance of benefits that rivers provide to society.
Supplementary material
The supplementary material for this article can be found at https://doi.org/10.1017/sus.2021.15
Acknowledgments
Funding for this study was provided in part by WWF in Washington, DC and by McGill University in Montreal, Quebec, Canada.
Author contributions
MT, DT, BL, GG, and JO conceived this paper. GG ran the analyses. MT, DT, and JO led the drafting of the paper and substantive contributions and reviews were provided by GG, JH, JPC, CN, MM, MG, CZ, KT, JH, and BL.
Conflicting interests
The authors declare that they have no conflicting interests.
Data and materials availability
The geometric dataset of the global river network and the associated attribute information for every river reach, i.e. the values of all pressure indicators as well as the main resulting indices of the study, including the connectivity and FFR status, are available from the figshare data repository at https://doi.org/10.6084/m9.figshare.14977305 (20XX) under the CC-BY-4.0 license. The databases of dams, required to calculate some of the pressure indices, are not part of the data repository due to licensing issues, but are freely available at http://www.globaldamwatch.org.