Introduction
In a world of rapid changes, the use of biotechnology offers a solution to meet the challenges in medicine and those related to agriculture, food and renewable energies. Among the different biotechnology, blue biotechnology that focuses on the exploration and exploitation of marine organisms in order to develop new products (turning aquatic biomass into novel foods, feed, energy, packaging…) is booming. One major field of application is the use of seaweed and microalgae in agriculture. They can elicit defensive responses resulting in protection against pathogen or insect damage, and can be used as biofertilizers (Chatzissavvidis and Therios, Reference Chatzissavvidis and Therios2014). Biofertilizers are gaining significance in sustainable agriculture as a means of enhancing crop productivity, in an environmentally friendly and economically viable manner, and reducing the polluting effects of synthetic fertilizers (Renuka et al., Reference Renuka, Guldhe, Prasanna, Singh and Bux2018; Nosheen et al., Reference Nosheen, Ajmal and Song2021). Among the various types of biofertilizers, formulations based on photosynthetic organisms, including eukaryotic microalgae and cyanobacteria, are gaining importance because of their significant contributions, particularly to the maintenance of soil fertility and enhancing crop yield (Li et al., Reference Li, Tao, Ling and Chu2017; Guo et al., Reference Guo, Wang, Wang, Zou, Liu, Hao, Alam, XU and Wang2020).
The loss of yield from agricultural production due to the presence of pests has been treated over the years with synthetic pesticides, but the use of these substances negatively affects the environment and presents health risks for consumers and animals. Natural compounds derived from plants such as Rhus muelleri, Aquilegia vulgaris (commonly called European crowfoot and granny's bonnet) can treat fungal infections. Phytopathogens, which remain current as one of the most common plant diseases, also represent one of the most significant issues for agricultural companies (Jimenez-Reyes et al., Reference Jimenez-Reyes, Carrasco, Olea and Silva-Moreno2019). P. sarmentosum (wild pepper) extract bioformulations can for example potentially be applied as bio-pesticides for the management of rice diseases (Syed-Ab-Rahman and Omar, Reference Syed-Ab-Rahman and Omar2018).
Researchers are also focusing on potential biological control microbes as viable alternatives for the management of pests and plant pathogens. In fact, there is a growing body of evidence that notes the potential of leaf and root-associated microbiomes to increase plant efficiency and yield in cropping systems (Syed Ab Rahman et al., Reference Syed Ab Rahman, Singh, Pieterse and Schenk2018). A variety of endophytic bacteria and free-living rhizobacteria on the root surface and rhizosphere utilize the nutrients released from the host, as well as secrete metabolite substances to the soil that aid in controlling plant diseases caused by fungi or bacteria. Besides, microalgae, especially cyanobacteria, are able to biosynthesize a number of secondary metabolites with potential biopesticidal action and can be considered potential biological agents for the control of harmful organisms to soils and plants (Costa et al., Reference Costa, Freitas, Cruz, Silveira and Morais2019; Gonçalves, Reference Gonçalves2021). These activities are attributed to phenolic compounds, polyphenols, tocopherols, saponins, nitrogen-rich peptides and sesquiterpenes.
Plant pathogens are forming a huge problem on the economic and life stability. They cause diseases for leaf, stem, root, vascular system and fruit indeed. They comprise viruses, bacteria, fungi, nematodes and parasitic plants, and induce diseases for leaf, stem, root, vascular system and fruit. The plant pathogen attacks the plant by using some mechanisms that are responsible for increasing the disease and appearance of the symptoms. New strategies not only directly protect plants against pathogens but can also induce enhanced immunity that permanently protects against pathogenic strains. For example, Chlorella fusca protects the host plant against pathogenic fungi Colletotrichum orbiculare (anthracnose) and Botrytis squamosa (leaf blight of onion) in cucumber and Chinese chive (Kim et al., Reference Kim, Shim, Kim, Ko, Park and Hwang2018).
Agriculture is also dealing with major issues to ensure food production quantities and constant evolution of quality standards (pesticide residues, GMOs-free, organoleptic properties). This is the goal of the current European Green Deal project: becoming climate neutral by 2050 by turning the political commitment into a legal obligation and a trigger for investment (European Commission, 2019). As a turning point in this project, concrete changes are observed in regulations in order to reduce our reliance on hazardous chemicals and invest in environmentally friendly technologies. Careful consideration of all available methods to ensure the success of cultures and their integration to discourage the development of populations of harmful organisms is of prime importance (Barzman et al., Reference Barzman, Bàrberi, Birch, Boonekamp, Dachbrodt-Saaydeh, Graf, Hommel, Jensen, Kiss, Kudsk, Lamichhane, Messéan, Moonen, Ratnadass, Ricci, Sarah and Sattin2015). Seaweed and microalgae are one of the solutions to overcome this challenge along with plant and microbial extracts (Jamiołkowska, Reference Jamiołkowska2020; Morales-Cedeño et al., Reference Morales-Cedeño, Orozco-Mosqueda, Loeza-Lara, Parra-Cota, de los Santos-Villalobos and Santoyo2021). One of the best historical examples of an algal product to protect crops is the use of purified laminarin extracted from Laminaria digitata, as an elicitor of plant defences (Klarzynski et al., Reference Klarzynski, Plesse, Joubert, Yvin, Kopp, Kloareg and Fritig2000). Currently, many other algal bioproducts have reached the market, exploiting the enormous diversity of potential modes of action they offer (Ronga et al., Reference Ronga, Biazzi, Parati, Carminati, Carminati and Tava2019).
The present review aimed at the interest of blue biotechnology in agronomy, with a specific focus on microalgae, their biological activities and their possible application in agriculture as a potentially sustainable alternative for enhanced crop performance, nutrient uptake and resilience to environmental stress.
Biotechnology
Biotechnology is defined as the application of science and technology related to living organisms, in order to modify living or non-living materials for the production of knowledge, goods and services (Organisation for Economic co-operation and Development, 2005). This includes microorganisms (microalgae, bacteria and fungi), algae and invertebrates. For more than three decades the field of biotechnology has had an extraordinary impact on science, law, regulatory environment and business. Since 2010, progress for biotechnology has increased quickly, with a focus in the pharma industry for science and health care (Niosi and McKelvey, Reference Niosi and McKelvey2018).
Historically, blue technology is linked to the health domain. For example, in the 1950's antiviral drug development was based on the identification of nucleosides in Tectitethya crypta (Demospongiae) by Bergman and Feeney (Reference Bergman and Feeney1951). Zidovudine (AZT) and Acyclovir drugs stem directly from this development. These authors also studied marine products against microbes. A few years later, in the 1960's, the first marine antibiotic (pentabromopseudilin) was described from the marine bacterium Pseudomonas bromoutilis (Uzair et al., Reference Uzair, Mahmood and Tabassum2011).
Currently, marine biotechnology contributes to several applications. Among them, a sustainable blue economy, the core idea of which is to draw economic benefit from marine and freshwater environments without sacrificing their ecological resilience, has emerged (Similä et al., Reference Similä, Soininen and Paukk2020).
Focusing on algae, they can produce biodiesel, bioethanol through fermentation, biomethane by anaerobic digestion and biohydrogen (Jayaseelan et al., Reference Jayaseelan, Usman, Somanathan, Palani, Muniappan and Jeyakumar2021). Biodiesel is made from algal biomass including oil extraction, knowing that microalgae oil contains more free fatty acids compared to the oil from terrestrial plants.
Microalgae and seaweeds have also been used for various purposes that range from food sources to drug development . They, in fact, represent an abundant unexplored source of highly diverse phytochemicals that possess various biological functions. Thousands of novel compounds and their metabolites, with diverse biological activities ranging from anticancer to antiviral, have been isolated since the 1990s (Yasuhara-Bell and Lu Reference Yasuhara-Bell and Lu2010). These compounds are rich in metabolites such as carotenoids, polysaccharides, terpenes, phenol derivatives, nitrogen-containing compounds and alkaloids which have huge potential for the cure of diseases (Abd El-Hack et al., Reference Abd El-Hack, Abdelnour, Alagawany, Abdo, Sakr, Khafaga, Mahgoub, Elnesr and Gebriel2019). Because of their healthy and bioactive compounds such as carotenoids, phycobilins, fatty acids, polysaccharides, vitamins and sterols, microalgae have been widely used in the health food market. Microalgal fat is also a vegetarian source of n-3 fatty acids [eicosapentaenoic acid (EPA), docosahexaenoic acid (DHA), γ-linolenic acid (GLA)], and show a high protein content. Multiple nutritional evaluations demonstrated the suitability of algae biomass as a valuable feed supplement or substitute for conventional protein sources (soybean meal, fish meal, rice bran, etc.). Besides the use of algae animal feed as a protein source for livestock, many of the health benefits also apply to animals (improved immune response, improved fertility, better weight control) (Remize et al., Reference Remize, Brunel, Silva, Berthon and Filaire2021). However, the technology to produce microalgae is still immature, even innovation has been done and continues on cultivation systems (Kratzer and Murkovic Reference Kratzer and Murkovic2021).
Microalgae have also received interest among agrochemical industries, through their capabilities to increase seed germination, seedling growth, crop productivity, as well as tolerance to abiotic stressors. However, compared to green biotechnology related to agricultural (Barkha et al., Reference Barkha, Barthi and Anita2016), the field for blue biotechnology can still be considered underdeveloped and future prospects will contribute significantly to tomorrow's agriculture (Barre and Bates, Reference Barre and Bates2018; Rizwan et al., Reference Rizwan, Mujtaba, Memon, Lee and Rashid2018). It appears that this domain is currently offering the best possibilities for future developments (Rizwan et al., Reference Rizwan, Mujtaba, Memon, Lee and Rashid2018). The phylogenetic diversity is significantly more important in water than on land. Life appeared in the water around 4 billion years ago and many phyla are present only in the marine environment. By linking all these features, it is easy to consider the potential of research dealing with marine organisms, the fruits of lengthy evolutionary processes and potentially full of unique metabolic specificities. It is also interesting to note that marine environments present highly contrasting conditions of life (deep seas, hydrothermal sources, streams…), increasing the possibility of encountering novelties and revealing new marine biotechnology applications (Costello and Chaudhary, Reference Costello and Chaudhary2017).
The agronomical use of blue biotechnology
The potential of seaweed or microalgae in agricultural applications is used since antiquity (Nabti et al., Reference Nabti, Jha and Hartmann2017). They have been used since ancient times directly or in composted form as a soil amendment to improve the productivity of crops in coastal regions (Craigie, Reference Craigie2011). It is also reported that in the second half of the first century, seaweed manure had widely been used (Metting et al., Reference Metting, Zimmerman, Crouch, Van Staden and Akatsuka1990).
Out of more than 800 000 species of algae that exist in nature, only 5000 have been characterized to date. Out of 5000 species, only a small number of the algae species have been selected to determine their potential applications in plant growth under defined growth conditions (Nabti et al., Reference Nabti, Jha and Hartmann2017; Lee and Ryu Reference Lee and Ryu2021). It is important to note the real growing interest in this field over the past 20 years, studies having almost doubled in the last 5 years (Lee and Ryu, Reference Lee and Ryu2021). This is mainly due to the fact that the current trend for agricultural practices is to reduce pesticides and fertilizers for the benefit of alternatives with a lower impact on human and environmental health. The use of genetically selected plants for their aptitude to resist biotic and abiotic stresses, mechanical weeding, physical barriers to avoid pest problems, cultural practices, adjustments and the use of chemical (as a last alternative) or biological solutions (Barzman et al., Reference Barzman, Bàrberi, Birch, Boonekamp, Dachbrodt-Saaydeh, Graf, Hommel, Jensen, Kiss, Kudsk, Lamichhane, Messéan, Moonen, Ratnadass, Ricci, Sarah and Sattin2015; Lamichhane et al., Reference Lamichhane, Dachbrodt-Saaydeh, Kudsk and Messéan2015).
The use of plants genetically selected for their ability to resist biotic and abiotic stresses, mechanical weeding, physical barriers to avoid pest problems, and the use of biological solutions were means to reduce the use of chemical solutions (Barzman et al., Reference Barzman, Bàrberi, Birch, Boonekamp, Dachbrodt-Saaydeh, Graf, Hommel, Jensen, Kiss, Kudsk, Lamichhane, Messéan, Moonen, Ratnadass, Ricci, Sarah and Sattin2015; Lamichhane et al., Reference Lamichhane, Dachbrodt-Saaydeh, Kudsk and Messéan2015). In this respect, blue biotechnology and more precisely the use of seaweed and microalgae enter the last category: biological solutions. They are a source of diverse natural substances and microorganisms, able to achieve plant stimulation and/or pest and pathogen biocontrol effects (Nabti et al., Reference Nabti, Jha and Hartmann2017; Righini et al., Reference Righini, Roberti, Varma, Tripathi and Prasad2019; Ronga et al., Reference Ronga, Biazzi, Parati, Carminati, Carminati and Tava2019; Stirk et al., Reference Stirk, Rengasamy, Kulkarni and van Staden2020). For example, cyanobacteria are regarded as the main biological pest control agents that control the various diseases in the plants, by producing various bioactive compounds against fungi, nematodes and other diseases (Thirumurthy and Mol, Reference Thirumurthy and Mol2020). The algal polysaccharide has biostimulant activities of plant growth, nutrients uptake and biotic and abiotic stresses tolerance (Zou et al., Reference Zou, Lu, Zhao, Yuan, Meng, Zhang and Li2019). Microalgae act also against phytopathogens. A general summary of the involvement of blue biotechnology in agricultural practices is presented in Fig. 1. More details on the latest advances and further prospects are discussed later on in the present paper.
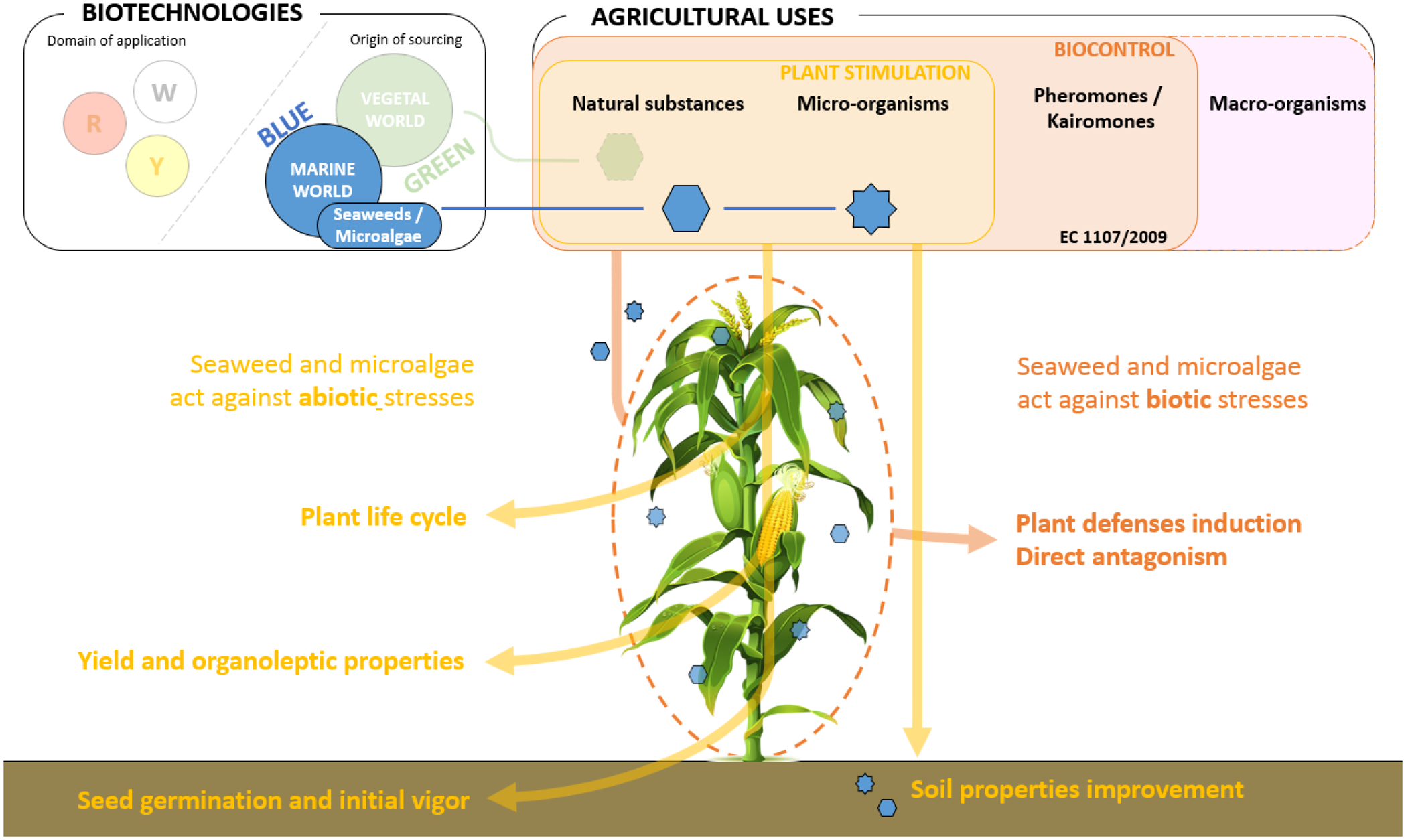
Fig. 1. Colour online. Action of blue technology on agricultural uses, seaweed and microalgae represent natural substances and micro-organisms both involved in plant stimulation and pest and pathologies biocontrol.
A plant biostimulant is ‘any substance or micro-organism, in the form in which it is supplied to be user, applied to plants, seeds or the root environment with the intention to stimulate natural processes of plants benefiting nutrient use efficiency and/or tolerance to abiotic stress, regardless of its nutrients content, or any combination of such substances and/or microorganisms intended for this use’ (European Commission, 2014). Starting in 2022, the EU market will open the single market for fertilizing products and aye down common rules on safety, quality and labelling requirements for fertilizing products.
Biological control is a strategy that was proposed half a century ago (Alabouvette et al., Reference Alabouvette, Olivain and Steinberg2006). Biocontrol solutions protect plants against biotic stresses (diseases, insects and other harmful organisms, weeds). They use natural mechanisms and interactions. According to the International Biocontrol Manufacturers Association (IBMA), the principle of biocontrol is based on the management of stresses and natural balances of the populations of aggressors rather than on their suppression. Biocontrol solutions are regulated in Europe the same way as chemical pesticides under the regulation EC 1107/2009 (Anonymous, 2009). In order to be competitive on these aspects of plant protection (against abiotic and/or biotic stresses), seaweed and microalgae offer a large range of features (Chanda et al., Reference Chanda, Merghoub and Arroussi H2019).
Known products and attributes of seaweed and microalgae in agriculture
Plant stimulation compounds
Plant growth is marked by its adaptability to continuous changes in the environment and led by the distribution of growth substances in the whole organism. These substances are produced by the plant, but they may also be brought exogenously by other organisms. They have been investigated in order to elucidate their mode of action in microalgae and higher plants (Lu and Xu Reference Lu and Xu2015). Phytohormones, including auxin (IAA), abscisic acid (ABA), cytokinin (CK), ethylene (ET) and gibberellins (GAs) are therefore described for their beneficial actions on major traits in phototrophic organisms. The presence and role of active hormones have been long speculated in algae, but the first proof of the bioactive forms of auxin, cytokinin, Gibberellic Acid (GA), brassinosteroid, Abscisic Acid (ABA), Jasmonic Acid (JA) and polyamines came from the work of Tarakhovskaya et al. (Reference Tarakhovskaya, YuI and Shishova2007).
Auxins are a class of plant hormones, essential for plant growth. They were the first class of plant hormones to be discovered. Indole-3-acetic acid (IAA) is the most abundant and the basic auxin natively occurring and functioning in plants. Auxin content in plant parts is variable depending on its needs and the associated development of the plant from the single cell to the whole organism (Li et al., Reference Li, Xie, Hu and Zhang2016). They act quickly on gene expression and regulation, and a large set of candidate genes that are potentially regulated by auxins have been identified in the last three decades (Di et al., Reference Di, Zhang, Luo, An and Guo2016). Environmental conditions are linked to the pattern of auxin distribution within the plant, modifying the development of plant organs. Thus, plants react to external factors and adapt their needs through the active transport of auxin molecules.
The presence and/or functions of auxins (i.e. indole-3-acetic acid, IAA; indole-3-butyric acid, IBA; phenylacetic acid, PAA) were confirmed in different microalgal species from Chlorella, Scenedesmus and Acutodesmus genus (Žižková et al., Reference Žižková, Kubes, Dobrec, Pribyl, Simura, Zahajska, Drabkova, Novak and Motyka2017; Piotrowska-Niczyporuk et al., Reference Piotrowska-Niczyporuk, Bajguz, Kotowska, Bralska and Talarek-Karwel2018). Nannochloropsis oculata, Nannochloropsis oceanica and Dunaliella salina also present bioactive forms of auxins (Han et al., Reference Han, Zeng, Bartocci, Fantozzi and Yan2018).
These phytohormones are the most essential endogenous molecules for modifying physiological and molecular reactions and are critically required by the plant for its survival under heavy metal stress. They act as chemical messengers and, under a complex regulation, allow plants to sustain abiotic stresses such as excess heavy metal ions (Piotrowska-Niczyporuk et al., Reference Piotrowska-Niczyporuk, Bajguz, Kotowska, Kotowska and Zambrzycka-Szelewa2020). Recent sequencing efforts have shown that the indole-3-pyruvic acid pathway, one of the primary biosynthetic pathways in land plants, is also found in the red, brown and green algae (Morffy and Strader, Reference Morffy and Strader2020). Generally speaking, various concentrations of IAA or its derivatives are identified in microalgae extracts and supernatants, implementing both stimulatory and inhibitory effects on the growth and metabolism of higher plants and microalgae (Han et al., Reference Han, Zeng, Bartocci, Fantozzi and Yan2018).
Cytokinins are a class of plant hormones with many physiological processes, including stimulation of cell division, differentiation and growth, regulation of seed dormancy and germination, and inhibition of the root apical meristem (Wybouw and De Rybel, Reference Wybouw and De Rybel2019). There are two types of cytokinins: adenine-type and phenylurea-type cytokinins, with the first being the only one retrieved in plants (Mok, Reference Mok2019). In microalgae, it had been speculated that the levels of active cytokinins are controlled by de novo biosynthesis (Sakakibara, Reference Sakakibara2006). For instance, Nayar (Reference Nayar2021) showed that the direct activation of cytokinins is functional in Chlorella variabilis. Cytokinins are also present in Acutodesmus obliquus, Chlorella protothecoides, Dunaliella salina, Chlorella vulgaris and Gracilaria caudata (Han et al., Reference Han, Zeng, Bartocci, Fantozzi and Yan2018).
Occurrence in brown algae is well described and cytokinins can be found in several genus, such as Ascophyllum, Cystoseira, Ecklonia, Fucus, Macrocystis and Sargassum (Nabti et al., Reference Nabti, Jha and Hartmann2017), Ascophyllum nodosum extracts being used as biostimulant to promote growth and productivity in a number of agricultural production systems. Ecklonia maxima extracts present a high potential for application in agriculture due to its high content of several plant hormones (Nabti et al., Reference Nabti, Jha and Hartmann2017).
Gibberellins (Gas) are a class of plant hormones, essential for plant development, including stem elongation, dormancy, flowering and senescence (Hedden and Sponsel, Reference Hedden and Sponsel2015). They induce seed germination by breaking the seed's dormancy and acting as a chemical messenger. Hundreds of gibberellins have been discovered, however, only a few of them are recognized as bioactive (GA1, GA3, GA4 and GA7). GAs are be found in microalgae, such as Nannochloropsis oceanica (Lu and Xu Reference Lu and Xu2015) and Chlorella Sorokiniana. Recently, Do et al. (Reference Do, Tran, Le and Nguyen2020) determined that auxins (IAA and IPA) and gibberellins (GA4 and GA7) were endogenous phytohormones produced by C. sorokiniana TH01 under both phototrophic and mixotrophic cultivation modes (Dumale et al., Reference Dumale, Gamoso, Manangkil and Divina2018). Green algae such as Trebouxiophyceae, Ulvophyceae and Charophycea also possess functional phytohormone metabolic pathways (Žižková et al., Reference Žižková, Kubes, Dobrec, Pribyl, Simura, Zahajska, Drabkova, Novak and Motyka2017).
Abscisic acids (ABA) are a class of plant hormones, involved in plant development including dormancy, cell size and stomatal closure. Abscisic acids are also linked to plant responses against environmental stresses like heavy metal stress, drought, thermal or heat stress, high level of salinity, low temperature and radiation stress (Vishwakarma et al., Reference Vishwakarma, Upadhyay, Kumar, Yadav, Singh, Mishra, Kumar, Verma, Upadhyay, Pandey and Sharma2017). A comprehensive review of abscisic acids on plants from Chen et al. (Reference Chen, Li, Bressan, Song, Zhu and Zhao2020) highlighted the importance of their role for plant growth and development, while leaving many unanswered questions on the associated mechanisms.
The presence of ABA in seaweed has been identified in Chlorophyta species such as Chara foetida, Draparnaldia mutabilis, Dunaliella parva, Ulva lactuca, Stigeoclonium tenuen (green algae) and in Phaeophyta species such as A. nodosum and three Laminaria species (brown algae). It is also important to note that when it comes to algae, ABA has been reported to mitigate stresses for cyanobacteria (salt stress) and algae (salt, osmotic, oxidative, drought and nutrient stresses) (Lu et al., Reference Lu, Tarkowská, Turecková, Luo, Xin, Li, Wang, Jiao, Strnad and Xu2014). Therefore, it has been proposed that the cultivation of microalgae can lead to more high-valued bioproducts with the combination of exogenous ABA and stress.
In summary, this overview on plant growth substances potential highlights that both seaweed and microalgae represent excellent candidates for plant biostimulation purposes, based on their confirmed content in phytohormones. Their use can therefore be considered at a different time of the plant's development cycle (Fig. 2).

Fig. 2. Colour online. Known phytohormones produced by seaweed and microalgae and their potential impact on the life cycle of a crop. IAA, Auxins; CK, Cytokinins; GAS, Gibberellins and ABA, Abscisic Acid.
Complex molecules, macronutrients and micronutrients contribution
Seaweed and microalgae are naturally composed of a wide diversity of compounds which, once applied to soil and/or plants, may contribute to crop development. As for other living organisms, they contain variable amounts of proteins, carbohydrates, lipids, macronutrients (i.e. Na, Ca, K, P, Mn…) and micronutrients (B, Cl, Cu, Fe, Mn, Mo, Zn) essential for crop growth and health. Moreover, it is now observed that microalgae by-products (i.e. culture ‘juice’ after production, cell walls…), are also used as bioproducts for agriculture, because of their richness in a large diversity of elements (Daneshvar et al., Reference Daneshvar, Zarrinmehr, Kousha and Bhatnagar2020).
Proteins, carbohydrates and lipids represent a pool of organic matter, easily assimilable by other organisms including plants, when released by dead organisms. As many seaweed and microalgae bioproducts contain dead cells with grinded biomass and/or atomised cells, they are, therefore, a direct source of nutrition when used, leading to direct stimulation of plants. Moreover, these compounds often reach more than 50% (and up to 70%) of the fresh weight under optimal growth conditions (Barre and Bates, Reference Barre and Bates2018). As an example, a detailed representation of the composition of the microalgae Nannochloropsis occulata summarized from the available literature, is given in Fig. 3.

Fig. 3. Colour online. Detailed composition of the microalgae Nannochloropsis occulata. Mean of the available literature set proteins content to 37%, carbohydrate content to 30% and lipid content to 22% of total biomass. Ashes are mainly composed of sodium and chlorine.
Carbohydrates also act as plant biostimulants. Polysaccharides found in seaweed extracts (i.e. ulvans, alginates, fucoidans, laminarans, lichenan like glucans and fucose containing glucans), neutral sugars and sulphates, showed a positive correlation between the sulphate content of polysaccharide enriched extracts and shoot dry weight as well as chlorophyll content in tomato plants (Arioli et al., Reference Arioli, Mattner and Winberg2015; Mzibra et al., Reference Mzibra, Aasfar, El Arroussi, Khouloud, Dhiba, Kadmiri and Bamouh2018). Different environmental/abiotic stresses are known to induce overproduction of oxygen radicals and their derivatives, so-called reactive oxygen species (ROS), which are able to oxidatively injure cells. Microalgae polysaccharides can mitigate ROS toxicity by stimulating the antioxidant defence system. More precisely, Chanda et al. (Reference Chanda, Merghoub and Arroussi H2019) suggested that algal polysaccharides are perceived by membrane receptors as microbial associated molecular patterns (MAMPs) and thereby inducing the MAMPs-dependent signalling pathways which involves the activation of ROS scavenging enzymes.
Finally, the application of polysaccharide enriched extracts on soil proved to be more effective in improving plant growth parameters than direct foliar application (Mzibra et al., Reference Mzibra, Aasfar, Benhima, Khouloud, Boulif, Douira, Bamoud and Kadmiri2021).
Soil properties improvement
Seaweed and microalgae may also act indirectly on plant stimulation because of their beneficial effect on soil properties.
Nitrogen fixation
Arable soils are amended with organic and inorganic fertilizers yearly, to offset the losses due to crop rotation. According to the FAO forecast, global nutrient consumption exceeds 200 million tonnes in 2020 (FAO, 2020). Among microalgae, cyanobacteria can synthesize the enzyme nitrogenase and present the capacity to fix nitrogen. These microalgae develop non-dividing cells called heterocysts which contain the enzyme nitrogenase (Chen et al., Reference Chen, Li, Zhang, Chang, Liu, Wang and Li2014). This capacity is of major importance to agriculture because it represents the only way to add nitrogen to the soil without organic or mineral amendment. There is currently a trend to improve the use of nitrogen fertilizer as an agricultural management practice in order to avoid economic loss and environmental pollution (Martínez-Dalmau et al., Reference Martínez-Dalmau, Berbel and Ordóñez-Fernández2021). Therefore, this capacity to directly fix inorganic nitrogen from the air and to convert it into an organic and usable form in the soil has been estimated to save around 25–40% of chemical N fertilizers (Nabti et al., Reference Nabti, Jha and Hartmann2017; Renuka et al., Reference Renuka, Guldhe, Prasanna, Singh and Bux2018; Martínez-Dalmau et al., Reference Martínez-Dalmau, Berbel and Ordóñez-Fernández2021). This natural fixation, due to the capacity of microalgae to immobilize N through the formation of biological soil crusts, limits the impact of nitrogen on the environment (i.e. runoff and leaching) (Veluci et al., Reference Veluci, Neher and Weicht2006).
Organic matter in the soil
One of the key factors for the establishment and the development of plants in soils, is their content of organic matter. Manure and slurry are commonly used to enrich soils with organic matter, resulting in a cost for farmers. One way to minimize this economic issue is the use of seaweed and microalgae as they are autotrophic communities, able for plants to fix atmospheric carbon dioxide through photosynthesis (Singh and Ahluwalia, Reference Singh and Ahluwalia2013). Their contribution to the total soil carbon content is significant and thus, soils treated with algal biofertilizers are in better condition for plant development (Yilmaz and Sönmez, Reference Yilmaz and Sönmez2017; Gonçalves, Reference Gonçalves2021).
Nutrient availability
As for microbial organisms, microalgae possess a set of enzymatic features (i.e. siderophores, organic acids), able to directly contribute to the global soil biomineralization process (Rizwan et al., Reference Rizwan, Mujtaba, Memon, Lee and Rashid2018). Moreover, recent studies highlighted that these processes can also occur indirectly, with microalgae inoculation modulating the rhizosphere microbiome and changing the diversity and abundance of microorganisms involved in the nutrients availability (Manjunath et al., Reference Manjunath, Kanchan, Ranjan, Venkatachalam, Prasanna, Ramakrishnan, Hossain, Nain, Shivay, Rai and Singh2016; Prasana et al., Reference Prasanna, Kanchan, Ramakrishnan, Ranjan, Venkatachalam, Hossain, Shivay, Krishnan and Nain2016).
Pest and pathogen biocontrol modes of action
Two modes of action are recognized for pest and pathogen biocontrol achieved by seaweed and microalgae products, depending if they act directly against pathogens by direct antagonism or indirectly after being detected by plants resulting in the induction of their natural defences (Righini et al., Reference Righini, Roberti, Varma, Tripathi and Prasad2019; Gonçalves, Reference Gonçalves2021).
Direct antagonism
Microalgae can be considered one of the major biocontrol agents for fungi and soil-borne plant diseases issues (Lee and Ryu, Reference Lee and Ryu2021). This is mainly due to their capacity for secondary metabolites production expressing a wide range of actions i.e. antifungal, antibiotic, nematicide… (Gonçalves, Reference Gonçalves2021). The set of involved molecules is large, including carbohydrates, proteins, peptides, polyphenols, tocopherols, oils, saponins and sesquiterpenes (Gonçalves, Reference Gonçalves2021). They act directly on cell membrane with functional and structural modifications, disruption, enzyme inactivation, inhibition of protein synthesis (Lee and Ryu, Reference Lee and Ryu2021). Additionally, seaweed is a good source of bioactive compounds (i.e. fatty acids esters, phenolic compounds…) with a direct antagonistic effect against known pathogens, responsible for important crop diseases (Mukherjee and Patel, Reference Mukherjee and Patel2020).
Plant defence induction
Seaweed is used for its capacity to induce plant defences. Plants have developed a complete innate immunity to fight against plant diseases, with defence systems activated after the recognition of microbial components called pathogen-associated molecular patterns (PAMPs) (flagellin, lipopolysaccharides, chitin) (Choi and Klessig, Reference Choi and Klessig2016). A large set of seaweed polysaccharides and derived polysaccharides trigger plant defence induction against a range of pathogens by activating salicylic acid, jasmonic acid and/or ethylene signalling pathways at a systemic level (Sharma et al., Reference Sharma, Fleming, Selby, Rao and Martin2014; Gonçalves, Reference Gonçalves2021).
The immune system of a plant is also stimulated by microalgae. Polysaccharides produced by microalgae are perceived by a plant's membrane receptors as elicitors, and trigger a series of defence mechanisms (Chanda et al., Reference Chanda, Merghoub and Arroussi H2019). In a study by Farid et al. (Reference Farid, Mutale-Joan, Redouane, Mernissi Najib, Abderahime, Laila and Arroussi Hicham2019), polysaccharides extracted from Chlorella vulgaris and C. sorokiniana exhibited a significant increase in β-1,3-glucanase activity, that break down the cell wall components of pathogens.
Focus of studies realized since 2018 on algae and microalgae recorded on plants
Seed germination and initial vigour
As the first step in a plant's life, a good start in seed germination often leads to a vigorous plant with a better chance to achieve its complete life cycle. This includes the establishment of the plant's autotrophic functioning mode after the use of reserve resources contained in the seed, essential to the plant's emergence. Compounds such as minerals, nutrients, amino acids, vitamins, pigments and polysaccharides present in the seaweed may refine seed germination, growth and development by providing them as biofertilizers or biostimulants (Chanda et al., Reference Chanda, Merghoub and Arroussi H2019). The plant tolerance towards extreme environments like high heat and drought conditions becomes better. It also appears that seaweed liquid fertilizer can influence cellular metabolism and improve its yield (Rajan et al., Reference Rajan, Thriunavukkarasu, Joseph and Aruni2020). It contains plant growth regulators like cytokinin, auxin and gibberellins, abscisic acids, cytokinins and ethylene, and is also the supreme source of nitrogen, phosphorous, potassium, calcium and magnesium required for the normal growth of plants (Han et al., Reference Han, Zeng, Bartocci, Fantozzi and Yan2018).
Recent research focused on the tomato plant model highlighted that several seaweed extracts were able to promote seed germination. This is the case of neutral and alkaline seaweed extracts of U. lactuca and Padina gymnospora when used at 0.2% extracts were able to enhance germination parameters (Hernández-Herrera et al., Reference Hernández-Herrera, Santacruz-Ruvalcaba and Hernández-Carmona2019). Similarly, Mzibra et al. (Reference Mzibra, Aasfar, Benhima, Khouloud, Boulif, Douira, Bamoud and Kadmiri2021) observed a significant increase of germination and speed in tomatoes associated with a significant reduction of mean germination time of seeds treated with 0.02 mg/ml of seaweed polysaccharide enriched extracts obtained from Gigartina sp., Gigartina pistillata, Chondracanthus acicularis, Gelidium crinale, Schizymenia dubyi, Cystoseira foeniculacea and Fucus spiralis. Similar results were also obtained by application of higher polysaccharide enriched extracts concentration (0.1 mg/ml) extracted from Ulva rigida, Codium tomentosum, Codium decorticatum and Bifurcaria bifurcate (Mzibra et al., Reference Mzibra, Aasfar, El Arroussi, Khouloud, Dhiba, Kadmiri and Bamouh2018). Garcia-Gonzalez and Sommerfeld (Reference Garcia-Gonzalez and Sommerfeld2016) tested cellular extracts and dry biomass of the green algae Acutodesmus dimorphus on tomato seeds of Roma tomato plants. Treated seeds with extract concentrations at 750 mg/ml triggered faster seed germination, occurring 2 days earlier than the control group.
Comparable effects were recorded for an A. nodosum seaweed-based biostimulant on seed germination and seedling growth of sunflower, at a dosage of 15 mg/l (dos Santos et al., Reference dos Santos, Zabotto, Jordão, Boas, Broetto and Tavares2019) and of onion, at a dosage of 5500 ppm (Hidangmayum and Sharma, Reference Hidangmayum and Sharma2017). Besides, A. nodosum used in the early stages of plant growth and germination offer protection against salt stresses for avocado (Persea americana Mill.) and on the fruit yield (Bonomelli et al., Reference Bonomelli, Celis, Lombardi and Mártiz2018; Yildiztekin et al., Reference Yildiztekin, Tuna and Kaya2018).
Puglisi et al. (Reference Puglisi, Barone, Fragalà, Stevanato, Baglieri and Vitale2020) showed that low concentrations of Chlorella vulgaris and Scenedesmus quadricauda (the equivalent of 1–2 mg of organic carbon contained in the extracts) were able to significantly enhance sugar beet seed germination. Moreover, individual application of Chlorella vulgaris and Spirulina platensis (3 g/kg of soil) increased the rate of germination and seedling length of maize crops (Dineshkumar et al., Reference Dineshkumar, Subramanian, Gopalsamy, Jayasingam, Arumugam, Kannadasan and Sampathkumar2019).
Plant development
The most common and generalized use of seaweed and microalgae products for biostimulation purposes is the application during vegetative stages of plant development. Foliar application of seaweed extracts enhance nutrient uptake, growth promotion and root development in different agricultural crops, such as maize, tomato, grape and broccoli (Mukherjee and Patel, Reference Mukherjee and Patel2020). Castellanos-Barriga et al. (Reference Castellanos-Barriga, Santacruz-Ruvalcaba, Hernández-Carmona, Ramírez-Briones and Hernández-Herrera2017) reported that low concentrations (0.2%) of acid-extracts obtained from U. lactuca can enhance growth parameters in mung bean (plumule and radicle length, shoot and root length as well as fresh and dry weight). Similarly, 3% extract of the brown seaweed Sargassum swartzii enables improvement in the shoot length and the number of leaves in Vigna inguiculata plants (Vasantharaja et al., Reference Vasantharaja, Abraham, Inbakandan, Thirugnanasambandam, Senthilvelan, Jabeen and Prakash2019). During the onion life cycle, the application of a 0.55% extract of A. nodosum proved to increase leaf number, plant height, crop growth height bulb diameter and pigment content (Hidangmayum and Sharma, Reference Hidangmayum and Sharma2017). Mahmoud et al. (Reference Mahmoud, Salama, El-Tanahy and Abd El-Samad2019) studied the effect of the commercial product ACTION® containing the seaweed Sargassum vulgare on red radish plants. The most effective treatment was found to be seaweed-soaked seeds before sowing and foliar sprayed seaweed extract at 3 mg/l once 20 days after sowing, with a greater number of roots and aerial parts compared to non-treated seeds and plants.
Garcia-Gonzalez and Sommerfeld (Reference Garcia-Gonzalez and Sommerfeld2016) tested cellular extracts and dry biomass of the green algae A. dimorphus as a foliar spray to evaluate plant growth and fruit production in Roma tomato plants. Aqueous extracts applied as foliar fertilizers at 3.75 g/ml resulted in increased plant height and greater numbers of flowers and branches per plant.
Yield and nutritional quality
Yield and nutritional quality are of major concern for farmers. The environmental factors affecting crop yield can be classified into abiotic and biotic constraints. Actually, these factors are more intensified with global warming which leads to climate change (Food and Agriculture Organization of the United Nations, 2017). Abiotic stresses adversely affect growth, productivity and trigger a series of morphological, physiological, biochemical and molecular changes in plants. A direct link between seaweed/microalgae and these parameters can be done, ensuring real interest in the use of these bioproducts in order to stabilize potential financial losses (Gonçalves, Reference Gonçalves2021). The effects of germination parameters (percentage index, mean time) and growth parameters (shoot and root length) of mung bean (Vigna radiata) treated with acid seaweed liquid of Ulva latucta extracts at 0.2% were evaluated by Castellanos-Barriga et al. (Reference Castellanos-Barriga, Santacruz-Ruvalcaba, Hernández-Carmona, Ramírez-Briones and Hernández-Herrera2017). Results showed that these extracts can significantly enhance seed germination rates. Production (fresh and dry weight) of mung bean was also significantly higher compared to the control treatments. Ascophyllum nodosum applied at a dosage of 0.5% is efficient to increase bulb diameter and fresh weight, in addition to sulphur and protein content of onion crops (Hidangmayum and Sharma, Reference Hidangmayum and Sharma2017). Concerning this nutritional quality, treatments with Ulva latucta extracts at 0.2% also significantly increased total chlorophyll concentration and protein contents (Castellanos-Barriga et al., Reference Castellanos-Barriga, Santacruz-Ruvalcaba, Hernández-Carmona, Ramírez-Briones and Hernández-Herrera2017). Treatment of Sargassum swartzii extract at 3% on Cowpea (Vigna unguiculata) increased not only total phenolic content, protein and flavonoids but also yield (Vasantharaja et al., Reference Vasantharaja, Abraham, Inbakandan, Thirugnanasambandam, Senthilvelan, Jabeen and Prakash2019).
Seaweed and microalgae are thus interesting candidates as biostimulation solutions. However, many studies have highlighted the importance to clearly establish dosage for optimal efficiency (Chanda et al., Reference Chanda, Merghoub and Arroussi H2019; Mukherjee and Patel, Reference Mukherjee and Patel2020). An excessive concentration of seaweed and microalgae extracts may lead to unwanted effects or decreasing trends in the measured parameters, such as toxic effects on shoots or impaired growth (Hidangmayum and Sharma, Reference Hidangmayum and Sharma2017).
Pest and pathogen biocontrol
Antimicrobial activity
The use of seaweed and microalgae as biocontrol agents of microbial plant pathogens is well known and described, and their use in commercial formulations already occurs on the market (Righini et al., Reference Righini, Roberti, Varma, Tripathi and Prasad2019). De Corato et al. (Reference De Corato, Salimbeni, De Pretis, Avella and Patruno2017) found that some crude extracts from brown (Laminaria digitata and Undaria pinnatifida) and red (Porphyra umbilicalis, Eucheuma denticulatum and Gelidium pusillum) seaweed achieved control of grey mould and brown rot on strawberry, peaches and lemons. The identified action was mainly due to direct pest control with the importance of fatty acids, supported by an induction of resistance elicited by carbohydrates.
From a more in vitro point of view, Mohy El-Din and Mohyeldin (Reference Mohy El-Din and Mohyeldin2018) explored the capacities of four brown seaweed extracts (Colpomenia sinuosa, Padina pavonia, Cystoseira barbata and S. vulgare) for their antifungal activities against Aspergillus niger, A. flavus, Penicillium parasiticus, Candida utilis and Fusarium solani. The originality of this study was to compare extracts prepared from seaweed harvested during different seasons with multiple extractants. Methanol extracts were the most efficient, and Cystoseira barbata was the best seaweed to achieve maximum biocontrol effects. This difference in the type of extracts, moreover with methanol, was also highlighted by Khan et al. (Reference Khan, Abid and Hussain2017) in their study using 32 seaweed extracts against Fusarium oxysporum. This inhibition effect was also observed for a 15% extract of the seaweed Tubinaria conoides, with 100% inhibition against the same pathogen (Ameer Junaithal Begum et al., Reference Ameer Junaithal Begum, Selvaraju and Vijayakumar2016). Sargassum wightii presented high levels of antioxidants with free radical scavenging ability, resulting in an inhibition of Gram-negative bacterial pathogen (Suganya et al., Reference Suganya, Varman, Masjuki and Renganathan2016).
Spirulina sp. and Nannochloropsis sp. extracts were able to be very efficient against Fusarium graminearum species complex because they have a high content in chlorogenic acid (Scaglioni et al., Reference Scaglioni, Pagnussatt, Lemos, Nicolli, Del Ponte and Badiale-Furlong2019). The advantages conferred by these microalgae extracts had led some companies to patent them, as the extracts obtained from the Amphidinium genus and their use as fungicidal and/or bactericidal activity on fungi, oomycetes and/or pathogenic bacteria of plants and crops seeds (Immunerise biocontrol patent, de la Crouee and Thomas, Reference de la Crouee and Thomas2019).
Microalgae may also trigger a better biocontrol effect of some microbial strains. This is the case of Trichoderma, a well-known biocontrol genus, whose efficiency is enhanced in the presence of Chlorella vulgaris extracts, to control the late wilt disease caused by Cephalosporium maydis in maize (Elshahawy and El-Sayed, Reference Elshahawy and El-Sayed2018).
Inhibition of other plant pests
Seaweed and microalgae also have potential roles in the control of insects or nematode populations involved in plant problematics (Renuka et al., Reference Renuka, Guldhe, Prasanna, Singh and Bux2018). In their study, Suganya et al. (Reference Suganya, Varman, Masjuki and Renganathan2016) evaluated the efficacy of two types of seaweed for their potential as an insecticide. Their ethanol extract of Sargassum wightii presented larvicidal activity against Aedes aegypti (dengue and Zika virus vector). Another insect problem, the Asian citrus psyllid Diaphorina citri, has been controlled by repellent and insecticidal effects of ethanolic extracts obtained from Caulerpa sertularioides, Laurencia johnstonii and Sargassum horridum seaweeds (González-Castro et al., Reference González-Castro, Muñoz-Ochoa, Hernández-Carmona and López-Vivas2019). A recent example of success against nematodes is the use of three microalgae (Scenedesmus obliquus, Chlorella vulgaris and Anabaena oryzae), alone or in combination, to control the root-knot nematode Meloidogyne incognita. All treatments significantly reduced the number of juveniles in soil and the triple mixture was the best at increasing total phenolic content and antioxidant amounts in plants (Hamouda, Reference Hamouda2017). The same observations occurred from the use of commercial seaweed products A. nodosum and Ecklonia maxima, which adversely affect hatching and sensory perception of the root-knot nematodes, Meloidogyne chitwoodi and Meloidogyne hapla, in in vitro assays on tomato (Ngala et al., Reference Ngala, Valdes, dos Santos, Perry and Wesemael2016).
Future challenges
Currently, one of the most important challenges is crop production. As the global population increases, agricultural production must also keep pace with it (Frona et al., Reference Frona, Szenderak and Harangi-Rakos2019). In the process to increase crop production, fungicides, nematicides, fertilizers, mainly synthetic chemicals, are being used. However, it is now well established that chemical products may be linked to environmental disasters and severe health issues. One way to reduce our reliance on synthetic chemicals is the use of solutions of natural origin. Among them, seaweed and microalgae extracts show high levels of promise, with a low or complete absence of harmful effects for the environment and human health and their first plan role to develop sustainable agriculture (Hamed et al., Reference Hamed, Abd El-Rhman, Abdel-Raouf and Ibraheem2018). This is also in line with the European Regulation EC 1107/2009, concerning the placing of plant protection products on the market and repealing Council Directives 79/117/EEC and 91/414/EEC- [2009, which recommends that priority should be given to non-chemical and natural alternatives wherever possible (Regulation (ec) no 1107/2009 of the European Parliament and of the Council, 2009)].
The democratization of biosolutions for agriculture and furthermore of seaweed and microalgae, remains marginal. Some limits can be identified to explain that:
(1) Characterization of the mode of action and the correct use of these products: Seaweed and microalgae present beneficial properties for agriculture, but only if they are correctly used. It is not always the highest concentration of the extracts that present the best results for plant simulation or biocontrol activity. Moreover, sometimes a wrong dosage leads to adverse or unwanted effects on plants (i.e. delay in its life cycle, toxic effect, death) (Hidangmayum and Sharma, Reference Hidangmayum and Sharma2017).
(2) Surpass current standards: The current use of seaweed and microalgae products often propose the same genus of organisms or by-products (A. nodosum and Ecklonia maxima as major biostimulant and laminarin as biocontrol product). However, the potential of these organisms is broad, and the diversity is not exploited. Moreover, the use of seaweed or microalgae is currently based on the direct use of biomass or enriched fractions. As our world becomes more sensitive to the sustainability of our production systems and the limitation of waste, it becomes interesting to re-think the global process of this industry and to increase the value of all its chain of production. One of the possibilities is the use of culture supernatant as a part of the formulation of the bioproduct. For microalgae production, these juices are usually thrown away after cultivation, despite their high content in nutrients and other molecules of interest such as IAA (Prieto et al., Reference Prieto, Cordoba, Montenegro and González-Mariño2011). An example of success for implementing this concept is the product Naneos (Greencell), a liquid including a base of A. nodosum and Nannochloropsis occulata extract.
(3) Beyond the laboratory: Even if there is a great potential offered by seaweed and microalgae to become tomorrow's phytosanitary product, the number of products exploiting these organisms on the market is still limited. One of the major challenges for the production of algal biopesticides is clearly to increase the number of studies in order to identify the substances with potential pesticidal activity and their mode of action (Costa et al., Reference Costa, Freitas, Cruz, Silveira and Morais2019). Many compounds with ‘cide’ activity (the meaning of this prefix is cutting or killing) have been discovered and some of them, patented, but still not released onto the market. We can also see that most studies relating to algae are mainly conducted on biofuel and other bioproducts that they produce. Global programmes are therefore of prime importance to accompany this essential transition in our future agricultural practices.
(4) Regulation of these products: Regulation on biofertilizers and biostimulants is not harmonized between countries. This will soon be the case in Europe with the EU 2019/1009 regulation entering in force in 2022. This regulation will open the algae market, as organic fertilizers will not require marketing authorization anymore, thus having a strong and positive impact on blue biotechnology development for the agronomical field.
Conclusions
Research on seaweed and microalgae are one of the keys for future agriculture development. Presently, the world possesses a large number of industrial sites with plant production capacities able to provide the quantities required to supply the market. Studies are increasing in number yearly and mechanisms behind each species and/or extracts are increasingly detailed. However, a gap still needs to be overcome in order to transform scientific research on seaweed and microalgae into concrete solutions for professional users, even if the biosolutions gain momentum every year in terms of total market share.
Financial support
This research received no specific grant from any funding agency, commercial or not-for-profit sectors.
Conflict of interest
The authors declare no conflicts of interest.
Ethical standards
Not applicable.