Introduction
Strongyloidiasis is a globally distributed, but neglected disease caused by Strongyloides stercoralis, which is more common in tropical and subtropical regions of Africa, South-East Asia and Latin America in conditions of poor sanitation (Levenhagen & Costa-Cruz, Reference Levenhagen and Costa-Cruz2014). This disease is especially dangerous for immunosuppressed hosts due to their high risk of developing hyperinfection syndrome, a condition in which proliferation of larvae in various organs culminates in death (Greaves et al., Reference Greaves, Coggle, Pollard, Aliyu and Moore2013). Strongyloides stercoralis larvae can also infect sites that are not part of their usual life cycle and cause disseminated strongyloidiasis (Puthiyakunnon et al., Reference Puthiyakunnon, Boddu, Li, Zhou, Wang, Li and Chen2014). Treatment of these severe forms of strongyloidiasis in patients who are immunocompromised and/or have reduced intestinal absorption of oral antiparasitic drugs is still controversial (Moura et al., Reference Moura, Maia, Ghazi, Amorim and Pinhati2012).
The indiscriminate use of anthelmintic drugs has favoured the development of parasite resistance (Carvalho et al., Reference Carvalho, Chagas, Cotinguiba, Furlan, Brito, Chaves, Stephan, Bizzo and Amarante2012), which reduces the effectiveness of drugs therapies (Grove, Reference Grove1996) and prompts the continuous search for complementary anthelmintic drugs (Kumarasingha et al., Reference Kumarasingha, Karpe and Preston2016). In this context, medicinal plant research is a rich source of knowledge on the potential action of plant extracts in treating human and animal diseases (Carvalho et al., Reference Carvalho, Chagas, Cotinguiba, Furlan, Brito, Chaves, Stephan, Bizzo and Amarante2012), including the control of intestinal nematodes through their anthelmintic properties (Wangchuk et al., Reference Wangchuk, Pearson, Giacomin, Becker, Sotillo, Pickering, Smout and Loukas2016).
Siparuna guianensis, which belongs to the Siparunaceae family, is a typical bush of the Brazilian Cerrado known as ‘negramina’, though this popular name varies according to the region or country where it occurs (Valentini et al., Reference Valentini, Rodríguez-Ortíz and Coelho2010). Siparuna guianensis leaves and flowers are used in Brazilian folk medicine due to their aromatic, stimulatory, anti-inflammatory, diuretic, anti-dyspeptic, anti-rheumatic and carminative properties (Aguiar et al., Reference Aguiar, Santos, Morgado, Ascencio, Lopes, Viana, Didonet and Ribeiro2015).
As there are no reports on the anthelmintic activity of S. guianensis, the present study examined the action of ethanol extracts, fractions, essential oil and major constituent α-bisabolol derived from it against Strongyloides venezuelensis eggs and larvae in vitro.
Materials and methods
Strongyloides venezuelensis eggs and larvae
The strain of S. venezuelensis was maintained by serial passage of infective larvae (L3) in gerbils (Meriones unguiculatus). Faeces from infected animals were used for egg collection and vermiculite culture (72 h at 28°C) to obtain infective (L3) larvae (Rugai et al., Reference Rugai, Mattos and Brisola1954).
Plant material
Aerial parts of S. guianensis were collected in May 2014 at Jataí, Goiás, Brazil, within the Cerrado. The voucher specimen (Herbário Jataiense 1997) was deposited at the herbarium of the Universidade Federal de Jataí, Goiás, Brazil.
Preparation of extracts and fractions
Leaves of S. guianensis were washed with distilled water and dried for three days in an oven at 40–45°C. The dried material was powdered, submitted to ultrasonic extractions with ethanol for five times and filtered. The filtrate was rotary evaporated at 50–55°C to yield the ethanol extract. This extract was dissolved in an ethanol/water (3:7) solution and partitioned by liquid–liquid extraction with hexane and ethyl acetate. The resulting hexane, ethyl acetate and aqueous fractions were concentrated and stored at −20°C until used.
Extraction of S. guianensis essential oil
Leaves of S. guianensis were crushed and hydrodistilled in a Clevenger-type apparatus for 4 h. The oil was collected with the hydrolate and extracted with dichloromethane (3 × 10 ml) in a separatory funnel. The organic phases were dried with anhydrous magnesium sulphate and rotary evaporated at 40°C, under low pressure. The resulting essential oil was weighed in an analytical balance and the extraction yield was expressed as mg/g plant dry mass. Dry matter was determined by drying three aliquots of approximately 10 g in an oven at 103 ± 2°C to constant mass. The essential oil was stored in sealed tubes at −20°C until used.
Isolation of α-bisabolol
The S. guianensis essential oil was fractionated on a silica gel chromatographic column (36 cm × 18 mm) according to the method reported by Diniz (Reference Diniz2014). Briefly, 0.2 g of essential oil was applied onto the top of the column and eluted with a hexane/ethyl ether gradient in the following ratios: 10:0, 9:1, 8:2, 7:3, 6:4 and 5:5. The fractions were analysed by thin-layer chromatography, using hexane/ethyl ether (7:3) as the mobile phase and phosphomolybdic acid as the developer. α-bisabolol was identified by gas chromatography–tandem mass spectrometry, and compared with an α-bisabolol standard previously characterized by the Laboratory of Analysis and Synthesis of Agrochemicals at the Universidade Federal de Viçosa, Viçosa, Minas Gerais, Brazil (Diniz, Reference Diniz2014). The α-bisabolol fraction was stored in a stoppered sample vial at −20°C until used.
Dilution of S. guianensis samples
The S. guianensis extract and fractions were dissolved in 1% dimethyl sulfoxide (DMSO) to achieve final concentrations of 0.2–1.0 mg/ml and 0.05–0.8 mg/ml, respectively. The essential oil and isolated α-bisabolol were diluted in 1% DMSO to provide 0.2–1.0 mg/ml solutions. All the solutions were stored under refrigeration until used.
Phytochemical analysis
Qualitative phytochemical characterization and analysis by thin-layer chromatography were performed according to the protocol reported by Barbosa et al. (Reference Barbosa, Quignard, Tavares, Pinto, Oliveira and Oliveira2004). The ethanol extract and ethyl acetate and aqueous fractions were screened for the presence of tannins, phenolic compounds, flavonoids and saponins. Aliquots of the extract and fractions were applied to silica gel plates, eluted with a mixture of solvents with different polarities (hexane, ethyl acetate, acetone and methanol) and revealed using ultraviolet light (254–360 nm) and acid vanillin.
Egg hatching test
The egg hatching test was performed using the procedure reported by Coles et al. (Reference Coles, Bauer, Borgsteede, Geerts, Klei, Taylor and Waller1992), with some modifications. Faeces from S. venezuelensis-infected gerbils were homogenized in water and filtered through a 50-μm sieve. Fifty microliters of the filtrate containing 50 eggs were added to 50 µl of each S. guianensis test-sample (extract, fractions, essential oil and isolated compound). After 48 h of incubation at 28°C, egg hatching and larvae from hatchings were quantified using an optical microscope. Albendazole solution (0.025 mg/ml) was used as the positive control (Carvalho et al., Reference Carvalho, Chagas, Cotinguiba, Furlan, Brito, Chaves, Stephan, Bizzo and Amarante2012), and water and 1% DMSO were used as the negative controls.
Larval motility test
The larval motility test was performed according to the method described by Cordeiro et al. (Reference Cordeiro, Athayde, Vilela, Costa, Silva, Araújo and Rodrigues2010), with some modifications. Fifty microliters of a solution containing 50 infective larvae (L3) were mixed with 50 µl of each S. guianensis test-sample (extract, fractions, essential oil and isolated compound). After 24, 48 and 72 h of incubation at 28°C, larval motility was analysed and quantified using an optical microscope. Ivermectin solution (0.316 mg/ml) was used as the positive control (Rebollo et al., Reference Rebollo, Taira, Ura and Williams2003), and water and 1% DMSO were used as the negative controls. All the tests were performed in triplicate for each concentration.
Data analysis
The percentage of inhibition of eggs hatching (IEH) was calculated as reported by Wood et al. (Reference Wood, Amaral and Bairden1995), who followed the Guidelines of the World Association for the Advancement of Veterinary Parasitology (WAAVP):

The percentage of inhibition of larval motility (ILM) was calculated using the formula reported by Al-Rofaai et al. (Reference Al-Rofaai, Rahman and Abdulghani2013):

Data were analysed using the GraphPad Prism 5.0 software. The half-maximal inhibitory concentration of each test-sample (IC50) was calculated. The experimental, positive and negative control groups were compared using Fisher's exact test. Comparison of mobile and immobile larvae at the different times of analysis and between the different concentrations tested was performed by one-way analysis of variance (ANOVA) and the Kruskal–Wallis test. Data from the extracts and fractions at the same dilution were compared using the Mann–Whitney test. P < 0.05 was considered statistically significant.
Results and discussion
The S. guianensis samples differed in their ability to inhibit S. venezuelensis egg hatching (table 1; see supplementary table S1). None of the ethanol extract concentrations tested suppressed egg hatching as effectively as the positive control; however, those from 0.6 to 1.0 mg/ml suppressed eggs hatching more strongly than the negative controls (P < 0.05). The inhibitory effect of the ethyl acetate and aqueous fractions, essential oil and isolated α-bisabolol on egg hatching only differed from the negative controls at the highest concentrations tested (1.0 mg/ml; P < 0.05). The ethyl acetate and aqueous fractions suppressed egg hatching in a concentration-dependent manner, their inhibitory effect increasing from 71.2% and 70.7% at 0.05 mg/ml, respectively.
Table 1. Inhibition of S. venezuelensis egg hatching after 48 h of treatment with S. guianensis samples.

Letters indicate significant difference (P < 0.05) from the controls, awater and cdimethyl sulfoxide (DMSO), for the Fisher's exact test.
There are few studies on the in vitro anthelmintic activity of plants against Strongyloides spp., and most of these are related to other nematodes, such as Haemonchus contortus (Macedo et al., Reference Macedo, Bevilaqua and Oliveira2009, Reference Macedo, Bevilaqua, Oliveira, Camurça-Vasconcelos, Morais, Machado and Ribeiro2012).The S. guianensis samples were effective against S. venezuelensis at concentrations lower than plant extracts from Eucalyptos globulus (Macedo et al. Reference Macedo, Bevilaqua and Oliveira2009), Alpinia zerumbet, Coriandrum sativum, Lantana camara and Tagetes minuta (Macedo et al., Reference Macedo, Bevilaqua, Oliveira, Camurça-Vasconcelos, Morais, Machado and Ribeiro2012) were against H. contortus.
In this study, we demonstrated that the negative controls (water and DMSO 1%) showed 62.7% and 69.4% inhibition of S. venezuelensis egg hatching, respectively; however, a higher number of larvae were observed in relation to other tested compounds of S. guianensis. These results were similar to the findings of negative controls in other IEH tests of the same species (Moraes et al., Reference Moraes, Levenhagen, Costa-Cruz, Costa Netto and Rodrigues2017). This study suggested that IEH caused by negative controls was due to the isolation of the eggs from another environment that was not faeces. A previous study showed that the viability of S. venezuelensis eggs was lower when isolated from saline solution compared to storage in faeces, and when exposed to different environmental conditions (Baek et al., Reference Baek, Islam and Kim1998). Compared with the negative controls, all the S. guianensis samples tested, ethanol extract, aqueous and ethyl acetate fractions, essential oil and isolated α-bisabolol, reduced the number of embryonated eggs and caused degradation of L1 larvae inside the egg. These larvae resembled a condensed mass, suggesting that the S. guianensis samples reached the interior of the egg and interfered with the blastomeration phase. All the samples caused morphological changes in S. venezuelensis eggs within the concentrations tested (fig. 1). They all suppressed egg hatching as effectively as the positive control, albendazole, at the highest concentrations tested, and caused morphological alterations in the eggs with degradation of L1. This was consistent with previous reports that the anthelmintic efficiency of a given compound relies on its ability to inhibit egg development and hatching during the first larval stage (Krychak-Furtado et al., Reference Krychak-Furtado, Negrelle, Miguel, Zaniolo, Kapronezai, Ramos and Sotello2005).
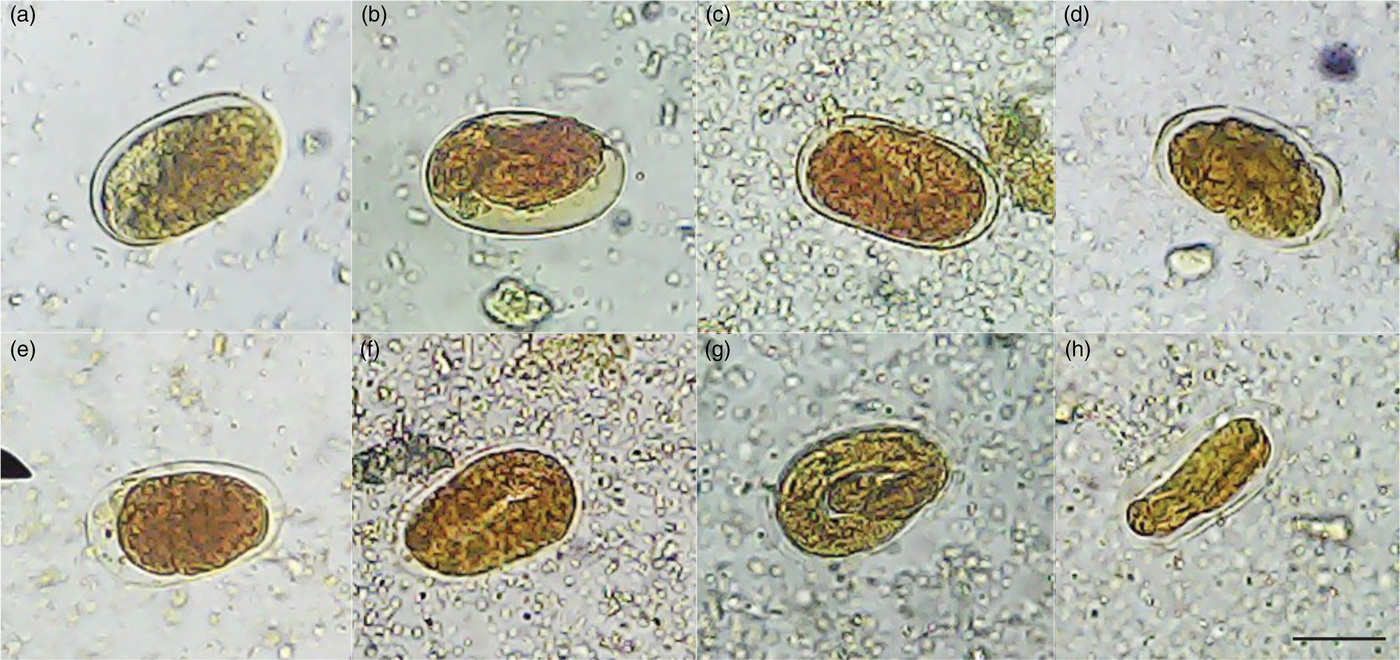
Fig. 1. Morphological changes in S. venezuelensis eggs after treatment with (a) an ethanol extract, (b) essential oil, (c) α-bisabolol, (d) ethyl acetate and (e) an aqueous fraction of S. guianensis leaves. Negative controls: (f) water and (g) 1% DMSO. Positive control: (h) 0.025 mg/ml albendazole. Note: there was no development of L1 larvae inside the eggs and only a condensed mass was visualized for treatments (a)–(e) and (h). Negative controls: (f) water and (g) 1% DMSO showed L1 larvae inside the eggs. Images were captured at 40× magnification. Scale bar 40 μm.
The inhibitory effect of the five S. guianensis samples on S. venezuelensis larval motility did not significantly differ across the treatment periods (24, 48 and 72 h; data not shown); however, they significantly differed from the negative controls (water and DMSO) and showed a concentration-dependent manner, except for α-bisabolol (table 2; see supplementary table S2). The ethanol extract suppressed larval motility by 88.4% at 0.2 mg/ml and by 100% at concentrations >0.8 mg/ml (table 2). At concentrations of 0.8 mg/ml, the ethyl acetate and aqueous fractions suppressed larval motility by 82.2% and 99.4%, respectively (see supplementary table S2). Essential oil and α-bisabolol at the range of concentrations tested, as well as ethyl acetate and aqueous fractions at concentrations <0.4 mg/ml, only weakly inhibited larval motility (table 2; see supplementary table S2). The ethanol extract was the S. guianensis sample that suppressed larval motility the most strongly, inhibiting larval motility by 88.4% at the lowest concentration tested. These findings were consistent with the inhibitory effects of Spondias lutea ethanol extract and fractions against S. venezuelensis adult females (Medeiros, Reference Medeiros2014), and Carica papaya latex and purified papain against L3 larvae (Moraes et al., Reference Moraes, Levenhagen, Costa-Cruz, Costa Netto and Rodrigues2017). WAAVP classifies an anthelmintic compound as highly and moderately effective when it inhibits >90.0% and 80.0–90.0% of parasite activity, respectively. Anthelmintic compounds kill worms by either starving or paralysing them, causing death in up to 24 h. The efficacy of S. guianensis ethanol extract against S. venezuelensis after only a 24-h treatment indicated its anthelmintic potential.
Table 2. Inhibition of S. venezuelensis larval motility after 24 h of treatment with S. guianensis samples.

Letters indicate significant difference (P < 0.05) from the controls, awater, bivermectin and cdimethyl sulfoxide (DMSO), for the Fisher's exact test.
As the chemical components of S. guianensis grown in Jataí, Goiás, Brazil, had not previously been described, we qualitatively analysed the ethanol extract and ethyl acetate and aqueous fractions. The major classes of secondary metabolites identified were tannins, phenolic compounds and flavonoids (see supplementary table S3). Although flavonoids, including quercetin (Leitão et al., Reference Leitão, El-Adji, Melo, Leitão and Brown2005) and vicenin-2 (Negri et al., Reference Negri, Santi and Tabach2012), have been identified in S. guianensis leaf extracts from other Brazilian regions, chemical components of leaves, stem and fruits can vary according to geographic location and season (Valentini et al., Reference Valentini, Rodríguez-Ortíz and Coelho2010). The combined use of phytochemical screening and thin-layer chromatography analysis increases the probability of selecting bioactive secondary metabolites (Macedo et al., Reference Macedo, Bevilaqua, Oliveira, Camurça-Vasconcelos, Morais, Machado and Ribeiro2012).
We hypothesize that the ovicidal and larvicidal activity of the ethanol extract and fractions of S. guianensis was mediated by one or more classes of metabolites identified here. Previous studies have reported the presence of condensed tannins and different subclasses of flavonoids in plants with anthelminthic potential (Kozan et al., Reference Kozan, Anul and Tatli2013; Femex et al., Reference Femex, Díaz, Gives, Mora, Zamilpa and Cortazar2016). The mechanism of action of flavonoids is related to their ability to change the parasite's metabolic processes and/or enzymatic activities (Kerboeuf et al., Reference Kerboeuf, Riou and Guégnard2008), while the action of tannins depends on the place where they bind to cause structural changes, such as the external cuticle and the digestive or reproductive tracts (Hoste et al., Reference Hoste, Jackson, Athanasiadou, Thamsborg and Hoskin2006).
Taken together, the findings reported herein demonstrate the anthelmintic potential of S. guianensis, especially the strong efficacy of its ethanol extract and fractions when compared with the other samples tested. Such fractions show promise for the development of new drugs. Notably, the ethanol extract and fractions were not cytotoxic towards NIH/3T3 murine fibroblasts and Vero cells, at least at the concentrations that exhibited significant anthelmintic activity (data not shown). However, additional studies are required to isolate and identify the chemical constituents responsible for the anthelmintic activity of S. guianensis as well as to establish their effectiveness in in vivo tests.
Supplementary material
To view supplementary material for this article, please visit https://doi.org/10.1017/S0022149X19000282
Author ORCIDs
R. M. Rodrigues, 0000-0001-6156-3649
Acknowledgements
The authors are grateful to Professor Michelle Rocha Parise, PhD (Medical School, Universidade Federal de Goiás, Regional Jataí, Jataí, GO, Brazil) for her assistance in data interpretation, and to the laboratory technician Lilia Cristina de Souza Barbosa (Laboratory of Morphophysiology, Federal University of Goiás, Regional Jataí, Jataí, GO, Brazil) for her assistance in microscope image acquisition.
Financial support
This study was supported by Coordination for the Improvement of Higher Education Personnel (CAPES, Brazil, grant number 1550878) and the National Council for Scientific and Technological Development (CNPQ, Brazil, grant numbers 453817/2014-9).
Conflict of interest
None.