Canine cognitive dysfunction (CCD) and Alzheimer's disease (AD) are the most common age-related neurodegenerative disorders affecting millions of pets and people worldwide. It is now well established that both oxidative stress and Aβ peptide have been implicated in the pathogenesis of CCD and AD( Reference Schütt, Helboe and Pedersen 1 ). Aβ peptide is formed and released after the sequential cleavage of the amyloid precursor protein by β- and γ-secretases, respectively. Aβ peptide, a 40–42 amino acid-long peptide, is an important component of senile plaque formation in AD brain( Reference Mattson 2 ) and its toxicity is mediated through the generation of hydrogen peroxide (H2O2)( Reference Behl, Davis and Lesley 3 , Reference Opazo, Huang and Cherny 4 ). Reactive oxygen species (ROS), on the other hand, generate highly electrophilic α,β-unsaturated carbonyl derivatives including acrolein, 4-hydroxy-2-nonenal, and 4-oxononenal from the peroxidation of membrane lipids( Reference LoPachin, Gavin and Petersen 5 ). In AD brain, levels of acrolein were found to be significantly higher in several brain regions such as in the hippocampus, amygdala, middle temporal gyrus and cerebellum( Reference Williams, Lynn and Markesbery 6 , Reference Bradley, Markesbery and Lovell 7 ). In primary neuronal cultures from the hippocampus, the toxicity of acrolein was higher than 4-hydroxy-2-nonenal( Reference Lovell, Xie and Markesbery 8 ). We have recently established on a neuronal cell line SK-N-SH the details of the multiprocess step of the toxicity of acrolein( Reference Thanh Nam, Arseneault and Zarkovic 9 ). We showed that, in addition to being a byproduct of lipid peroxidation, acrolein could also potentiate oxidative damage and activate several redox-sensitive pathways.
Incidence rates of age-specific AD in rural India are at least three times lower than those of an age-matched American reference population( Reference Chandra, Pandav and Dodge 10 ), which could be attributed to genetic, environmental or dietary causes. Withania somnifera (WS) is one of the most important medicinal plants used in the Indian system of traditional medicine as a nootropic agent and brain tonic to restore age-related decline in mental abilities( Reference Ven Murthy, Ranjekar and Ramassamy 11 ). The major biochemical constituents of WS are steroidal alkaloids and steroidal lactones saponins, together called withanolides( Reference Elsakka, Grigorescu and Stănescu 12 ). Presently more than twelve alkaloids and forty withanolides have been identified and characterised from the leaves, roots and berries of Withania species which either exist in free form, i.e. withanone, withaferin A, withanolide A, withanolides D-M, withanolides I–III, or in glycosidic form, i.e. withanosides I–VI( Reference Mirjalili, Moyano and Bonfill 13 ). Recently WS extract has been shown to protect against Aβ peptide- and H2O2-induced toxicities( Reference Kuboyama, Tohda and Komatsu 14 – Reference Kumar, Seal and Howes 16 ). In spite of its widespread use as a brain tonic, its neuroprotective activity against acrolein remains poorly investigated.
Since multi-factorial causes have been recognised in CCD and AD as well as in other neurodegenerative disorders, CCD and AD will require multiple drug therapy to address the varied pathological aspects. Even if the strategy of combining drugs with different therapeutic targets is workable, the new pharmacological approach is to develop a multi-functional compound or extract to target multiple sites in the brain. Thus, the purpose of the present investigation was to demonstrate if a treatment with a standardised extract of WS can protect the human neuroblastoma cell line SK-N-SH against Aβ peptide- and acrolein-induced toxicity, decrease ROS levels and inhibit acetylcholinesterase (AChE) activity.
Materials and methods
Drugs and chemicals
A standardised extract of WS was kindly provided by Natural Remedies Private Limited, Bangalore, India. WS extract was certified to contain 2·6 % withanolides including withanosides IV (0·87 %) and V (0·65 %), withaferin A (0·56 %), withanolide A (0·20 %) and B (0·06 %), and 12-deoxy withastramonolide (0·26 %) as determined by HPLC. Dulbecco's minimum essential medium (DMEM), fetal bovine serum, penicillin/streptomycin, acrolein, acetylthiocholine iodide, neostigmine bromide, 5,5′-dithiobis-2-nitrobenzoic acid (DTNB), H2O2 and TOX-2 (XTT based) were obtained from Sigma-Aldrich Inc. The cytotoxicity detection kit based on the lactate dehydrogenase (LDH) assay was from Roche Diagnostics and 2′,7′-dichlorofluorescein diacetate (DCF-DA) was from Invitrogen. β-Amyloid peptide(25–35) was purchased from American Peptide.
Aβ25–35 preparation
The synthetic peptide Aβ25–35 was dissolved in sterilised tap water and incubated at 37°C for 72 h for fibrillisation before use as previously described( Reference Longpré, Garneau and Christen 17 ). Although both soluble and fibrillar Aβ peptide can induce oxidative stress, the latter is more efficient in causing lipid peroxidation( Reference Melo, Sousa and Garção 18 ).
Cell culture and treatment
For neuroprotective effects of WS, SK-N-SH cells, a human neuroblastoma cell line from American Type Cell Culture (ATCC), were maintained in DMEM supplemented with 10 % (v/v) fetal bovine serum, 100 U/ml penicillin and 100 µg/ml streptomycin. SK-N-SH cells were plated at a density of 1·5 × 104 cells/well in ninety-six-well-plates (Corning) and incubated at 37°C. After 24 h of plating, the medium was completely removed and cells were kept in DMEM with antibiotics but without serum. Cells were then treated with toxic doses of Aβ (50 µg/ml) for 48 h or with acrolein (20 µm) for 24 h.
Cell viability assays
Cytotoxicity and cell survival were measured by LDH and XTT assays by using commercial kits as previously described( Reference Singh, Murthy and Ramassamy 19 ). The XTT assay measures mitochondrial dehydrogenases activity and may reflect the cellular metabolic state and serves as an indicator of cell survival. Because of the proliferative effects of the WS extract, cells may have higher dehydrogenases activity and the XTT test alone would not be sufficient to measure the neuroprotective effects. To further confirm the protective effects of the WS extract, we employed the LDH test; which measures cellular membrane integrity and is a mean of quantifying dead cells.
Intracellular reactive oxygen species level
The antioxidant activity of WS was confirmed by measuring its ability to scavenge ROS in SK-N-SH cells by using the fluorescent dye DCF-DA as previously described( Reference Singh, Murthy and Ramassamy 19 ). DCF-DA, a cell0permeable dye, is enzymically converted to a strongly fluorescent compound DCF in the presence of cellular ROS and the fluorescent intensity is directly proportional to the intracellular ROS level.
Acetylcholinesterase inhibition
The relative AChE- inhibitory activity of WS extract was determined in rat brain homogenates by a spectrophotometric method( Reference Ellman, Courtney and Andres 20 , Reference Singh and Rishi 21 ). The reaction mixture containing 50 µl of DTNB (3 mm), 25 µl WS extract, 25 µl brain homogenate from a pool of several Sprague–Dawley rats (100 µg total protein) and 100 µl of phosphate buffer. The reaction was started with the addition of 50 µl acetylthiocholine iodide (15 mm). Enzymic hydrolysis of acetylthiocholine iodide releases thiocholine, which forms a yellow 5-thio-2-nitrobenzoate anion as a result of the reaction with DTNB. The plate was read kinetically at λ 412 nm for 300 s and change in optical density/min was calculated. Neostigmine bromide (6·25 nm) was taken as a positive control.
Data analysis
All results were confirmed in four or five separate independent experiments with at least three technical replicates each time and expressed as means with their standard errors. The GraphPad InStat3 program (GraphPad Software) was used for data analysis. Statistical analysis was done by one-way ANOVA followed by Dunnett's multiple means comparison test and significance was considered when P was <0·05.
Results
Withania somnifera extract protects SK-N-SH cells against Aβ peptide- and acrolein-induced toxicity
Results in Tables 1 and 2 show that treatment with the WS extract protects SK-N-SH cells against Aβ peptide- and acrolein-induced toxicity. This protection was assessed 48 h after the addition of Aβ peptide and was significant from 1·0 and 5·0 µg/ml for the LDH and XTT assays, respectively (Table 1). Interestingly, treatment with the WS extract could also protect SK-N-SH cells against toxicity induced by acrolein from 12·5 µg/ml of WS and reached a maximum at 25 µg/ml (Table 2). To rule out any false-positive effects and interference with the XTT and LDH assays, absorbance was read and no interference was observed with the different doses of WS tested.
Table 1. Effect of treatment with Withania somnifera (WS) on SK-N-SH cell survival after 24 h with Aβ peptide (50 µg/ml) using lactate dehydrogenase (LDH) and XTT assays†
(Mean values with their standard errors of four or five separate experiments performed in triplicate at least in each group)

Mean value was significantly different from that of the Aβ group: * P < 0·05, ** P < 0·01.
† Results are expressed as percentage of control (taken as 100 %).
Table 2. Effect of treatment with Withania somnifera (WS) on SK-N-SH cell survival after 24 h with acrolein (20·0 µm) using lactate dehydrogenase (LDH) and XTT assays†
(Mean values with their standard errors of four or five separate experiments performed in triplicate at least in each group)

** Mean value was significantly different from that of the acrolein group (P < 0·01).
† Results are expressed as percentage of control (taken as 100 %).
Withania somnifera extract decreases intracellular reactive oxygen species level
Since ROS and oxidative damages are involved in the toxicity of Aβ peptide and acrolein, we investigated the antioxidant activity of WS. The WS extract was able to decrease the levels of ROS in SK-N-SH cells treated with H2O2 (500 µm), with a significant effect observed from 5·0 µg/ml (Supplementary Fig. S1).
Withania somnifera extract has potent acetylcholinesterase-inhibitory activity
The so-called cholinergic hypothesis of AD proposed that degeneration of cholinergic neurons in the basal forebrain and the associated loss of cholinergic neurotransmission in the cerebral cortex and other areas contribute significantly to the deterioration in cognitive function seen in patients with AD( Reference Francis, Palmer and Snape 22 ). AChE is the key component of cholinergic synaptic transmission and plays a major role in the termination of impulse transmission by rapid hydrolysis of the neurotransmitter acetylcholine. This serves as a rationale for the use of three Food and Drug Administration-approved AChE inhibitors in the symptomatic treatment of AD( Reference Cummings 23 ). We thus investigated the effect of WS on the activity of AChE on brain homogenates. As shown in Fig. 1, AChE activity was reduced by 30 % using 12·50 µg/ml of WS and reached about 50 % of inhibition at 50 µg/ml of WS.
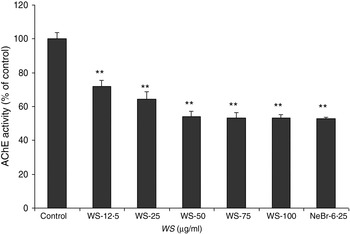
Fig. 1. Effect of Withania somnifera (WS) extract on rat brain acetylcholinesterase (AChE) activity. Results are expressed as percentage of control (taken as 100 %). Neostigmine bromide (NeBr; 6·25 nm) was taken as a positive control. Values are means, with standard errors represented by vertical bars, of four or five separate experiments performed in triplicate at least in each group. ** Mean value was significantly different from that of the control group (P < 0·01).
Discussion
There is growing interest in naturally derived bioactive compounds for pharmacological application for the treatment of CCD and AD. Different strategies are being investigated such as the neuroprotective therapeutic approach based on protection against Aβ-induced neurotoxicity and oxidative damage or inhibition of AChE. In the present study, our results showed that the WS extract could significantly protect SK-N-SH cells against Aβ- and acrolein-induced toxicity, decrease ROS levels in SK-N-SH cells and inhibit the activity of AChE. The protective effect against Aβ is consistent with other studies that have suggested that WS may be neuroprotective by mediating antioxidant effects. Similar to curcuminoids and ginkgolides, withanamides from WS fruit showed potent antioxidant activity as indicated by their ability to inhibit lipid oxidation( Reference Jayaprakasam, Strasburg and Nair 24 ). In our study, the neuroprotective effect against Aβ could not be attributed to the effects of WS on ROS scavenging as its effect on ROS levels was significant from 5 µg/ml while cytoprotection was observed at lower concentrations (1 µg/ml). Other mechanisms should be investigated such as the effects of WS on redox-sensitive pathways because it has been demonstrated that withaferin A, the major constituents of the extract, could inhibit the activity of the transcription factors NF-κB, or AP-1( Reference Kaileh, Vanden Berghe and Heyerick 25 ). Also, the WS extract was more effective than withanamide A, one of the major components of WS, against Aβ because withanamide A was inactive until 12·5 µg/ml( Reference Jayaprakasam, Padmanabhan and Nair 15 ). These results indicate that other components from the WS extract are implicated in the neuroprotective effect against Aβ such as sominone, the active metabolite of withanoside IV( Reference Kuboyama, Tohda and Komatsu 14 ).
The present study demonstrated the protective effect of WS against acrolein. This result is of great interest as acrolein has been shown to be elevated in the brain from AD, from mild cognitive impairment and from preclinical AD( Reference Singh, Nam and Arseneault 26 ) and could induce neuronal and glial toxicity( Reference Thanh Nam, Arseneault and Zarkovic 9 ). Different mechanisms could underlie this protection such as the antioxidant activity of WS because acrolein has been shown to increase the production of superoxide, activate NADPH oxidase activity and deplete reduced glutathione levels( Reference Ansari, Keller and Scheff 27 ). Moreover, among the α,β-unsaturated aldehydes, acrolein reacts 110–150 times faster with glutathione than 4-hydroxy-2-nonenal or crotonal( Reference Thanh Nam, Arseneault and Zarkovic 9 ). The effects of WS on the activity of redox-sensitive pathways could also be involved as we have recently shown that the toxicity of acrolein modulates different signalling pathways( Reference Thanh Nam, Arseneault and Zarkovic 9 ).
The inhibition of AChE activity by WS from 12·5 µg/ml described here is in line with the effects of withanolides on AChE with an half maximal inhibitory concentration (IC50) ranging between 20·5 and 49·2 µg/ml( Reference Choudhary, Nawaz and ul-Haq 28 ).
WS components have been shown to induce outgrowth of axons and dendrites, and memory enhancement. Tohda & Joyashiki( Reference Tohda and Joyashiki 29 ) have demonstrated that sominone, an active metabolite of withanoside IV, could induce the phosphorylation of RET (rearranged during transfection), a receptor for glial cell line induced neurotropic factor (GDNF). Pharmacokinetics studies in mice suggested rapid oral absorption of withanolides and revealed that withaferin A has one and half times more relative bioavailability as compared with withanolide A( Reference Patil, Gautam and Mishra 30 ). Recent studies have shown that WS extract reversed brain pathology in mouse models of Alzheimer's and amyotropic lateral sclerosis, thereby indicating that certain components are bioavailable and cross the blood brain–barrier( Reference Sehgal, Gupta and Valli 31 , Reference Dutta, Patel and Rahimian 32 ).
Thus, cholinesterase-inhibiting potential along with antioxidant ability and neurotrophic and neuroprotective activity against Aβ peptide and acrolein could make WS extract and its constituents possible therapeutic agents in CCD, AD and senile dementia. Our investigation further strengthens the traditional use of WS as a nootropic agent for restoring age-related decline in mental abilities. However, further exploratory animal studies are needed to optimise therapeutic doses, to find out the right therapeutic compounds that can cross the blood–brain barrier and the duration of WS treatments aiming to yield desired beneficial outcomes; this further represents challenges as some of the components such as withaferin A are potentially cytotoxic( Reference Wadhwa, Konar and Kaul 33 ).
Supplementary material
The supplementary material for this article can be found at https://doi.org/10.1017/jns.2017.48
Acknowledgements
Financial support was obtained from the Natural Sciences and Engineering Research Council of Canada (NSERC) for C. R.; M. S. gratefully acknowledges financial support from the Foundation Armand-Frappier.
There were no conflicts of interest.