1. Introduction
Since the report of the first RT pulsed operation of nitride based laser diodes by researchers at Nichia Chemical Industries two years ago Reference Nakamura and Fasol[1] a handful of research groups in Japan Reference Akasaki, Sota, Sakai, Tanaka, Koike and Amano[2] Reference Itaya, Onomura, Nishio, Sugiura, Saito, Suzuki, Rennie, Nunoue, Yamamato, Fujimoto, Kokobun, Ohba, Hatakoshi and Ishikawa[3] Reference Domen[4] and recently Cree Research in collaboration with North Carolina State and Brown Universities in the United States have reported pulsed operation, and in the case of Cree, short lived (15 sec) continuous wave (CW) operation Reference Bulman, Doverspike, Sheppard, Weeks, Kong, Dieringer, Edmond, Brown, Swindell and Schetzina[5]. Nichia now reports RT CW operation with lifetimes in excess of 1,000 hours Reference Nakamura[6]. Despite this significant progress by Nichia and others, the actual lasing mechanism and its relationship to the structural and electrical properties of these materials is not well understood.
2. Experiment
The laser structure shown in Figure 1 was grown on c-plane sapphire using a combination of atmospheric and low pressure MOCVD in a two-flow horizontal reactor. The chemical precursors used were trimethylgallium (TMGa), trimethylindium (TMIn), trimethylaluminum (TMAl), ammonia, bis(cyclopentadienyl)magnesium (Cp2Mg) and disilane.

Figure 1. Epitaxial Structure
The lasers facets were formed by Cl2 reactive ion etching of 125 μm wide mesas of various lengths ranging from 400 μm to 2,000 μm. P-contact stripes were subsequently formed in the center of these large mesas with widths ranging from 3 μm to 20 μm. The n and p-contacts were formed by electron beam evaporation of Ti/Al/Ni/Au and Ni/Au respectively. Fabricated lasers were tested under pulsed operation with a duty cycle of about 0.025%.
3. Results
Figure 2 gives a photo of a 20×400 μm device operating above threshold showing the narrowed far field with some lateral interference due to multi-lateral mode operation. The projected far field pattern narrows abruptly at threshold. Using the central spot the vertical and lateral far-field half-angles were approximately 10° and 3°, respectively, for the 20 μm wide stripe.
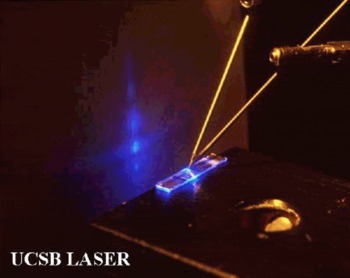
Figure 2. Photo of 20×400 μm device operating above threshold, showing elliptical far-field pattern.
A typical light vs. current (LI) curve is shown in Figure 3. The LI curve shows characteristic super linear behavior. The highest differential efficiency was 1.1%. Output powers of these devices were limited by heating during the pulses. Nevertheless, peak powers as high as 17.6 mW were obtained. Most devices gave outputs in excess of 10 mW. Device yield was well above 50%. The emission above threshold was strongly TE polarized with an extinction ratio in excess of 60 as shown in Figure 4.

Figure 3. Light vs. current (LI) for 800 μm long device with a 5 μm stripe

Figure 4. Light vs. Current (LI) with polarizer parallel and perpendicular to junction plane
Spectra were collected above and below threshold using an optical spectrum analyzer with a resolution of 0.1 nm. A strong, well-defined mode spectrum appears at threshold as shown in Figure 5. The resolution is not sufficient to resolve the expected individual mode spacing for the cavity lengths tested. As a result the width of the observed peaks corresponds to the analyzer resolution. The lasing spectrum does, however, show an envelope modulation with a peak spacing around 0.35 nm similar to that observed by Nakamura et al. Reference Nakamura and Fasol[1]. The origin of this modulation is still unclear.

Figure 5. Above threshold spectrum for 400 μm long device with a 5 μm stripe width
Diodes of different length and stripe width gave a range of threshold current densities as shown in Figure 6, Figure 7 and Figure 8. As indicated the threshold current density decreased for increasing width and length in rough agreement with the expected reduction in cavity loss. With increasing cavity length the threshold current density approaches a minimum, indicating that internal losses (as opposed to mirror losses) dominate the cavity loss. The lowest threshold current density observed was 12.7 kA/cm2 for a 10×1200 μm laser bar with uncoated facets at room temperature. Devices longer than 1200 μm could not be tested due to limitation in the pulse supply

Figure 6. Jth vs. laser length for 3 μm stripe width

Figure 7. Jth vs. laser length for 5 μm stripe width

Figure 8. Jth vs. laser length for 10 μm stripe width
A lifetime measurement done on a single device gave a lifetime greater than 6 hours. A 10×400 μm device was operated pulsed above threshold at a power of 2.6 mW. LI curves taken at intervals during the test are shown in figure 9. Initially the LI curves improve (lower threshold.) This is most likely due to annealing of the contacts and or thermal activation of the p-type dopant due to heating during operation. After 4 hours the threshold current density increases slightly until the device fails catastrophically between 6 and 8 hours due to shorting of the p-n junction.

Figure 9. LI curves taken at various time interval during continuous pulsed operation
4. Conclusion
Pulsed operation of 420 nm blue laser diodes with a threshold current density as low as 12.7 kA/cm2, output powers as high as 17.6 mW and lifetimes in excess of 6 hours are demonstrated. The power output of these devices is limited by heating during pulses. The lasing spectrum shows a “mode like” envelope modulation of unknown origin.
Acknowledgments
This work was supported by: DARPA (Dr.Anis Husain, Program Manager),ARO,NSF, HP Labs and Philips. The authors would like to acknowledge Daniel Cohen, Evelyn Hu, Milan Minsky, James Speck, X. Wu, John Bowers, Kehl Sink of UCSB and Bruce Young of Philips Research Labs for useful inputs and discussions.