Introduction
Hiärneite, (Ca,Na,Mn2+)2(Zr,Mn3+)5(Sb,Ti,Fe3+)2O16, was described as a new mineral (Holtstam, Reference Holtstam1997) from the famous Fe–Mn–(Ba–Pb–As–Sb–Be–B) deposit at Långban, in Värmland, Sweden (Holtstam and Langhof, Reference Holtstam and Langhof1999). It occurs in a phlogopite-rich skarn with magnetoplumbite, fluorapatite, oxyplumboroméite, pyrophanite and jacobsite (Fig. 1), and was interpreted as a product of metasomatism and regional metamorphism. Hiärneite was the first mineral known to have an essential combination of Zr and Sb in its formula (the second one is usturite, a member of the garnet supergroup; Grew et al., Reference Grew, Locock, Mills, Galuskina, Galuskin and Hålenius2013).

Fig. 1. Microphotograph in polarised light of a thin section with poikiloblastic hiärneite (orange) and magnetoplumbite/jacobsite (opaque) in a fine-grained phlogopite matrix. Field of view = 1 mm width. Specimen GEO-NRM #19920776.
Hiärneite, having a tetragonal unit cell with a = 15.264(1), c = 10.089(2) Å, space-group symmetry I41/acd, was inferred to be isostructural with calzirtite, ideally Ca2Zr5Ti2O16 (Holtstam, Reference Holtstam1997). The crystal structure was, however, not studied in detail at the time of discovery. We have now collected single-crystal diffraction data and refined the crystal structure of type hiärneite (sample from the mineral collection of the Swedish Museum of Natural History, GEO-NRM #19920776).
X-ray diffraction data and refinement
X-ray diffraction data of hiärneite were obtained with an Oxford Diffraction Xcalibur 3 diffractometer (MoKα radiation, λ = 0.71073 Å) fitted with a Sapphire 2 CCD detector (see Table 1 for details). Intensity integration and standard Lorentz-polarisation corrections were done with the CrysAlis RED (Oxford Diffraction, 2006) software package. Crystal shape and dimension optimisation were performed with X-shape (Stoe and Cie, 1996), based on the Habitus program (Herrendorf, Reference Herrendorf1993). The set of reflections was corrected for absorption via a Gaussian analytical method and averaged according to the 4/mmm point group. Following an absorption correction, the merging R for the data set decreased from 0.081 to 0.054. The analysis of the reflection conditions unequivocally led to the choice of the space group I41/acd. Given the close similarity in terms of unit-cell dimensions and crystal symmetry, the crystal structure was refined starting from the atomic coordinates of calzirtite, ideally Ca2Zr5Ti2O16 (Rossel Reference Rossell1982; Sinclair et al., Reference Sinclair, Eggleton and McLaughlin1986; Rastsvetaeva et al., Reference Rastsvetaeva, Pushcharovskii, Spiridonov and Gekimyants1995; Jafar et al., Reference Jafar, Phapale, Achary, Mishra and Tyagi2016).
Table 1. Crystal and diffraction experimental data.

*Weighting scheme: w=1/[σ2(Fo2)+(0.0441P)2+4.9070P] where P = (Fo2+2Fc2)
The site occupancy factor (s.o.f.) at the cation sites was allowed to vary (Mn vs. structural vacancy, Sb vs. structural vacancy, Ca vs. structural vacancy and Zr vs. structural vacancy for M1, M2, M3 and M4, respectively) using scattering curves for neutral atoms taken from the International Tables for Crystallography (Ibers and Hamilton, Reference Ibers and Hamilton1974). The mean electron numbers obtained for each site were modelled considering the observed bond distances and the electron microprobe analysis (see below). These proportions were then fixed in subsequent refinement cycles (see Table 2 – constrained refinement). The full-matrix least-squares program Shelxl-97 (Sheldrick, Reference Sheldrick2008) was used for the refinement of the structure. Convergence was easily achieved up to R 1 = 0.046. Atomic coordinates and bond distances are given in Tables 2 and 3, respectively. The crystallographic information file has been deposited with the Principal Editor of Mineralogical Magazine and is available as Supplementary material.
Table 2. Fractional atomic coordinates, site occupancies and equivalent isotropic displacement parameters (Å2) for hiärneite.

Table 3. Selected bond distances (Å) in hiärneite.

Crystal structure
The crystal structure (Fig. 2) is a derivative of the fluorite-type structure, with a tetragonal symmetry. Here we use the same site nomenclature previously used for calzirtite. The cations are arranged on planes parallel to (010) and constitute double heteropolyhedral layers (at y = ⅙, ⅓) containing the M2, M3, and M4 sites (with 8-, 6- and 7-fold coordination, respectively), intercalated between Ml + M4 layers (at y = 0, ½). A special feature of the calzirtite structure is the splitting of the Zr-dominated M1 site (Zr–Zr contact 0.54 Å) within a distorted cube of O atoms. In hiärneite, metal atoms occupying the 4- to 8-coordinated site M1 are fixed at (0, ¼, ⅛) without any spreading of electron density. For the remaining parts, calzirtite has an identical structural topology as hiärneite. Bond distances are the same within 0.02 Å as obtained for previous studies of the calzirtite structure, except for average M1–O distances that are slightly longer in hiärneite (2.31 Å vs. 2.26 Å).

Fig. 2. The crystal structure of hiärneite seen along [001].
Chemical composition
Calzirtite is normally chemically relatively pure, with minor Nb and Ta substituting for Ti, Hf for Zr and Fe for Ca (Bellatreccia et al., Reference Bellatreccia F., Caprilli E, Williams and Parodi1999); it may also host rare earth elements and actinides at low concentrations (Pascal et al., Reference Pascal, Di Muro, Fonteilles and Principe2009). As the concentration of common Pb is usually negligible, calzirtite has proven to be useful for 207Pb–206Pb age determination (Wu et al., Reference Wu, Yang, Mitchell, Bellatreccia, Li and Zhao2010). Hiärneite is more complex in composition (Table 4, data from Holtstam, Reference Holtstam1997).
Table 4. Compositional data for hiärneite based on electron-microprobe point measurements*.
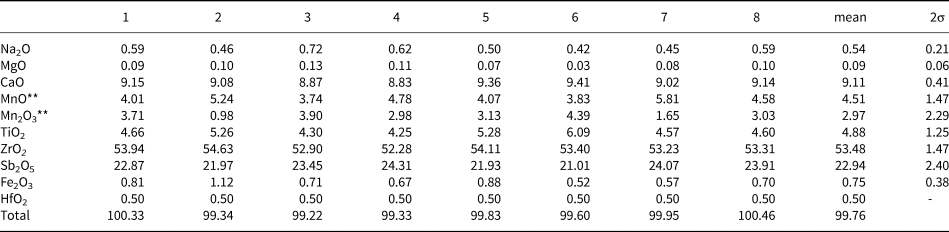
*Analytical conditions: wavelength-dispersive spectroscopy, 20 kV accelerating voltage and 12 nA beam current. HfO2 concentration is estimated from energy-dispersive X-ray spectrometry. Data from Holtstam (Reference Holtstam1997), reproduced with permission.
**Calculated from stoichiometry (9 cations, 16 oxygen atoms).
The distribution of the cations present at the structural sites of hiärneite is based primarily on ionic radii and electronic charge. Calcium, Na and a fraction of Mn2+ are assigned to M3. The M2 site is a mixed Sb, Ti site with minor Fe3+. The M4 site, as for calzirtite, is dominated totally by Zr (+ Hf). The M1 site hosts mainly Mn and a small fraction of Zr. These cation assignments are supported by refined site-scattering values (Table 5) and bond-valence sums (Table 6). The deviation of 2% in the overall mean electron number is acceptable for an Sb-bearing compound. The corresponding empirical formula of hiärneite is M 3(Ca1.57Na0.17Mn2+0.26)Σ2.00 M 4(Zr3.98Hf0.02)Σ4.00M 1(Mn3+0.36Mn2+0.35Zr0.21Fe3+0.05Mg0.02)Σ1.00M 2(Sb1.37Ti0.59Fe0.04)Σ2.00O16. The presence of Mn3+ in hiärneite is confirmed by optical absorption spectroscopy (Holtstam, Reference Holtstam1997). Ordering of Mn3+ to a normally Zr-dominated site is a very rare phenomenon, but the similar ionic radii (0.65 and 0.72 Å, respectively) and electronic charges make it feasible. The assignment of Mn3+ to the M1 site, and the presence of both Sb5+ and Ti4+ at the M2 site, form the basis for a definition of the ideal formula of hiärneite: Ca2Zr4Mn3+SbTiO16. The formula is expressed in this way to conform with the listed International Mineralogical Association (IMA) formula of calzirtite (from which its name is directly derived, and where Zr5 represents the combined contents of M1+M4). The point-analysis closest to the hiärneite end-member represented here (no. 6 in Table 4) has the composition M 3(Ca1.61Na0.13Mn2+0.26)Σ2.00M 4(Zr3.98Hf0.02)Σ4.00M 1(Mn3+0.53Mn2+0.26Zr0.17Fe0.03Mg0.01)Σ1.00 M 2(Sb1.24Ti0.73Fe0.03)Σ2.00O16.
Table 5. Site-scattering (s.s.) values (epfu) for hiärneite.

* Wyckoff position
Table 6. Weighted bond-valence sums (in valence units) for hiärneite according to the parameters of Brese and O'Keffe (Reference Brese and O'Keeffe1991) for all the elements (site occupancies in Table 2) apart from Sb5+, which was calculated according to Mills et al. (Reference Mills, Christy, Chen and Raudsepp2009a).

In hiärneite, the average sum of trivalent ions at M1 (Mn3+ + Fe3+) is 0.41 atoms per formula unit (apfu), a marginally higher value than (Mn2+ + Mg) with 0.37 apfu. Minor changes in the chemical compositions could make the sum of divalent cations dominant instead, and thus the hypothetical end-member formula Ca2Zr4Mn2+Sb2O16 becomes valid according to the dominant-constituent rule. The application of the site-total-charge (STC) approach (Bosi et al., Reference Bosi, Hatert, Hålenius, Pasero, Miyawaki and Mills2019), however, gives a STC value of +2.81 for M1 and +9.33 for M2, close to +3 and +9, respectively. The dominant atomic arrangement is then Mn3+ at M1 and (SbTi)9+ at M2, giving the end-member formula presented here.
Classification
As calzirtite and hiärneite are nearly isostructural, and consist of similar chemical components, they constitute a mineral group (Mills et al., Reference Mills, Hatert, Nickel and Ferraris2009b). The calzirtite group was introduced informally by Strunz and Nickel (Reference Strunz and Nickel2001), and includes calzirtite, an orthorhombic modification, calzirtite-1O (described by Callegari et al., Reference Callegari, Mazzi and Ungaretti1997), hiärneite and the structurally closely related cubic mineral tazheranite (Zr,Ti,Ca)(O,□)2 (where □ = vacancy). Tazheranite is considered as a natural form of ZrO2, ‘cubic zirconia’, stabilised by impurity elements and oxygen vacancies (Rastsvetaeva et al., Reference Rastsvetaeva, Pushcharovskii, Spiridonov and Gekimyants1998; Konzett et al., Reference Konzett, Wirth, Hauzenberger and Whitehouse2013). Its structure is characterised by Zr and Ca, Ti etc. distributed randomly over the regular 8-coordinated sites in a fluorite-type Fm $\bar{3}$m unit cell, whereas the anion sites are only partially occupied for charge compensation (also shown for synthetic analogues; Howard et al., Reference Howard, Hill and Reichert1988). In calzirtite and hiärneite, the ordering of the cations over specific sites leads to a lower symmetry and a tripled a parameter and doubled c parameter (Table 7). More pure variants of cubic ZrO2 have also been reported to form from shock-metamorphism of zircon (e.g. Kenny and Pasek, Reference Kenny and Pasek2021). All of the group members belong to the Nickel-Strunz subdivision 4.DL (Strunz and Nickel, Reference Strunz and Nickel2001). The calzirtite group, with calzirtite, hiärneite and tazheranite, has been accepted by the Commission on New Minerals, Nomenclature and Classification of the IMA, along with the new formula of hiärneite presented here (proposal 21-G, Miyawaki et al., Reference Miyawaki, Hatert, Pasero and Mills2022).
Table 7. Minerals of the calzirtite group.

1 Sinclair et al. (Reference Sinclair, Eggleton and McLaughlin1986); 2this work; 3Rastsvetaeva et al. (Reference Rastsvetaeva, Pushcharovskii, Spiridonov and Gekimyants1998).
Supplementary material
To view supplementary material for this article, please visit https://doi.org/10.1180/mgm.2022.19
Acknowledgements
We thank CNMNC members for suggestions on the original proposal dealt with by the commission. Reviews by two anonymous reviewers and Structural Editor Peter Leverett improved the paper.