Biological tissues are marvelous examples of adaptive, self-healing materials. However, the tissues exhibit complex biochemical and mechanical behaviors that are often challenging to understand and predict. Wound healing in epithelial tissue is a multicellular remodeling process particularly relevant to health and disease states.
In a recent study by an interdisciplinary team of scientists (representing 10 research institutions across six countries), key mechanistic features of epithelial wound healing have been elucidated. The findings are published in the July 9 online edition of Nature Communications (DOI: 10.1038/ncomms8683). The report clarifies the roles of two gap-closing mechanisms—cell crawling and purse-string dynamics—and describes how the relative contributions of the two mechanisms are affected by gap geometry.“Our paper uncovers a purely mechanical interaction between the two mechanisms,” said first author Andrea Ravasio. “Importantly, this interplay and the resulting speed of movement are functions of the local curvature.”
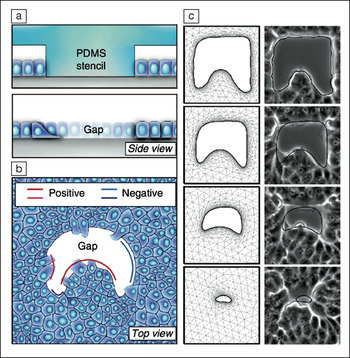
(a) Schematic overview of polydimethylsiloxane (PDMS) stencil approach to creating gaps of defined geometries within confluent epithelial cells; (b) schematic overview highlighting regions of positive and negative curvature associated with gap geometries; (c) time-lapse micrographs (right column; the initial gap is ∼100-µm wide) and results of finite element modeling (left column) show good agreement in gap closure dynamics. Credit: Nature Communications.
In tissue regions of positive curvature (e.g., the tips of finger-like structures pointing into the gap; see Figure), cells crawl across the substrate to close the tissue gap and drive wound healing. In areas of negative curvature (i.e., where the gap bows into the tissue; see Figure), purse-string closure—a gap-closing process in which cells constrict an actomyosin cable (a structural polymer used by the cells)—and crawling operate additively to fill the gap, leading to faster tissue velocity in regions of negative curvature.
To untwine these gap-filling dynamics, the researchers experimentally implemented microscopic gaps of defined geometries into confluent epithelial sheets (derived from a canine kidney cell type). Gap creation was achieved using either silicone (polydimethylsiloxane) stamps (which the cells initially grew around before their removal to expose the gap) for in vitro closure studies, or by etching through laser ablation for in vivo studies. Upon exposure to the gap, tissue recovery dynamics were monitored by optical microscopy (to record gap-edge velocity), fluorescence microscopy (to track immunostained cytoskeletal features), or traction force microscopy (to assess force dipole orientations across regions of positive and negative curvature). Forces tended to be perpendicular to edges with positive curvature, indicating cell crawling activity, and tangential to edges with negative curvature, indicating purse-string dynamics.
In a telling experiment, the actomyosin cable running along the gap edge was site-specifically severed by laser ablation, thereby mechanically disentangling regions of positive and negative curvature. Under this condition, tissue edges advanced at similar rates across regions of both positive and negative curvature. With an intact actin cable, however, regions with negative curvature moved relatively faster. Complementary experiments with drug inhibitors were able to isolate each closure mechanism by inactivating either purse-string closure or cell crawling independently. This revealed that purse-string closure speed depends on gap curvature (moving faster toward the gap with more negative curvature, and exhibiting edge retraction with positive curvature), while cell crawling speed remained largely unaffected by curvature.
The experimental insights obtained by the researchers allowed them to develop a mathematical model describing gap closure dynamics. The resulting simulations closely agreed with experimental results (see Figure c). The model incorporates the relative influence of cell crawling, purse-string action, and tissue boundary curvature in a general way such that in addition to accurately describing gap closure in the present study, the model further predicts relative contributions of crawling and purse-string mechanisms for other modes of motility such as cell extrusion and tissue expansion. Describing motility dynamics through such an insightful mechanistic framework is an important step toward more fully understanding epithelial tissues as complex natural biomaterials, and should ultimately allow improved designs of synthetic biomaterials that can interface with such tissues to accelerate wound healing in biomedical contexts.