Introduction
Over 50 000 plants are edible, but very few of them make any significant contribution to the human food supply. Cereals such as maize, rice and wheat together make up about 60 % of the world’s energy intake(1). Currently, the average annual global production of wheat is about 734 million tonnes(2). Only maize has, at 1·14 million tonnes, a higher global production.
Cereals and cereal products have a long history of use by humans. Cereals make significant contributions to dietary energy and nutrient requirements and are sometimes referred to as a ‘dietary staple’, along with milk, fruit and vegetables. Cereals underpin food-based dietary guidance globally. Scientific discussion regarding cereals has typically related to quantity and quality within the human diet, for example, low- or high-carbohydrate diets, whole or refined cereal. However, there has been some recent debate regarding level of processing as a descriptor to define food products, including cereal-based ones. The aim of this paper is to shed light on the fundamental processes for cereal-based foods as currently consumed and any implications for nutritional value in the context of a healthy diet. Using ready-to-eat breakfast cereals (RTEC) and breads as examples of globally consumed cereal-based food products, the review will compare the processes used in their generation and outline their importance in making nutrients available and helping to achieve dietary balance.
History of cereal processing and consumption by humans
The inclusion of cereals in the diet was an important step in human evolution as it is an example of how humans were able to increase the supply of digestible carbohydrates by creating solutions to the technical complexity and culinary manipulation needed to change raw cereals into palatable and nutritious foods or staples. Most scientists believed that the domestication of grains began about some 10 000 years ago, but more recent findings may put this time point substantially earlier. An ancient site in Israel contained a collection of cereals, which were dated to about 23 000 years ago(Reference Weiss, Kislev and Simchoni3), suggesting that cereal storage preceded domestication by about 10 000 years. The first processing of cereals might date back even further as evidence suggests that some early Homo sapiens were eating starchy, cereal-based snacks as early as 105 000 years ago(Reference Mercader4). For example, grass seed residue, mainly sorghum, has been found on ancient African stone tools, which in turn suggests an early application of processing to make the seeds consumable. More recently, about 30 000 years ago, plant food processing including the production of flour was a common practice across Europe(Reference Revedin, Aranguren and Becattini5). As early as about 7000 BC people in Greece were cultivating wheat, barley and oats(Reference Beetz6). Anecdotal evidence suggests that the troops of Hannibal, the Carthaginian general, ate porridge before and during their famous crossing of the Alps (218 BC).
Rationale for food processing
Simplistically, food processing can be described as any deliberate change in a food that occurs before it is available to people in a form and time that it is required(Reference Augustin, Riley and Stockmann7,Reference Floros, Newsome and Fisher8) . Food processing is therefore critical for food consumption as it typically turns inedible raw materials into more useful, shelf-stable and palatable foods or drinks fit for human consumption(9). This basic need as well as safety was one of the key drivers of the creation of food processing. Food processing has since evolved to satisfy more modern-day purposes which can be expanded as follows: (1) to make food edible; (2) to ensure safety of food; (3) to increase palatability (better tasting and greater access to nutrients)(Reference Knorr and Watzke10); and (4) to produce convenient foods(Reference Knorr and Watzke10).
Positive outcomes of food processing therefore include an increase in the useful life of foods, destruction of food-borne microbes and toxins, optimised nutrient availability, improved characteristics of food quality such as sensory characteristics and functional properties, increased convenience and reduced losses and waste(Reference Augustin, Riley and Stockmann7,Reference van Boekel, Fogliano and Pellegrini11,Reference Welch and Mitchell12) . Ultimately this provides greater choice and variety of safe products for the consumer. There may, however, be less desirable outcomes following processing, one example being an increased availability of starch which can facilitate rapid digestion and blood glucose elevation, especially if grain products are consumed as refined-grain products(Reference Grundy, Edwards and Mackie13). To prevent such downsides, an opportunity exists to optimise grain processing to improve nutritional value and make whole grains easier to use in food manufacturing.
Currently, there are a myriad of definitions about how to describe or classify processed foods, which have been described in detail elsewhere(Reference Jones14). These include descriptions by scientific authorities, for example, the European Food Safety Authority (EFSA), where processed food is simply defined within the definition of ‘food’(15) to more complex descriptions by non-profit organisations (for example, International Food Information Council(9)) or groups of scientists (for example, NOVA) where more detailed but differing categorisations have been developed(Reference Monteiro16,Reference Monteiro, Cannon and Levy17) . This may have contributed to the current standing where despite the long tradition of humans processing food, particularly cereals, today there is some confusion or uncertainty around processed foods and about what processing of food means among a number of scientists as well as some consumers. In fact, many food processing techniques are common to both homes and food manufacturers (Fig. 1).

Fig. 1. Most of the common food processing techniques are similar in home and industrial manufacturing (modified from the European Food Safety Authority(79)).
Main aspects of cereal processing
Processing of cereals is required before human consumption, as Rubel(Reference Rubel18) explained:
Humans are not equipped to live on raw grain as we have neither the teeth or the stomachs for it. We must transform grains into food through cooking, with basic methods of sprouting, fermenting, roasting, boiling and baking. Grinding and baking into breads radically increases a grain’s glycaemic index releasing otherwise unavailable carbohydrates.
In principle, cereal processing is essentially a physical process whereby the kernel is cleaned, adjusted to an appropriate moisture content and then mechanically reduced to the desired particle size to produce a flour and by-products (for example, bran). Where appropriate, flour production also involves fractionation – not only to separate bran, germ and endosperm from each other but also to assure the correct particle size of the milled endosperm. There are a number of processing steps for cereals between harvest and consumption. While each type of cereal requires specific treatment steps, the FAO suggest three distinct areas such as (1) preparation of the cereal for storage; (2) primary cereal processing to remove inedible fractions; and (3) secondary processing to transform cereals into ‘consumer-facing’ products (see Fig. 2)(19). Generally speaking, cereal products undergo all three processing steps before being consumed by humans.

Fig. 2. Principal processing steps of grains, modified from the FAO(19).
At a European level, the European Union (EU) Commission(20) mentions processing but only in the context of establishing maximal levels of certain contaminants within a foodstuff whereby:
“First-stage processing” shall mean any physical or thermal treatment, other than drying, of or on the grain. Cleaning, sorting and drying procedures are not considered to be “first-stage processing” insofar as no physical action is exerted on the grain kernel itself and the whole grain remains intact after cleaning and sorting. In integrated production and processing systems, the maximum level applies to the unprocessed cereals in case they are intended for first-stage processing.
Given this EU focus is directed at contamination of food rather than processing per se, for the scope of the present review we adhere to the more targeted FAO definition and discuss the primary and secondary processing steps of two key cereal products: flaked RTEC and bread. We acknowledge that there are many types of breakfast cereals with a varying range of processing steps. We have chosen flaked RTEC as an example of grain products since their production process demonstrates a variety of processing steps. Further, there is a significant history of humans eating both foods. Bread making is one of the oldest but also the most common processing technique for cereals, with reports of bread consumption by ancient Greeks, Egyptians and people belonging to ancient Rome and Babylon(Reference Pétel, Onno and Prost21). While breakfast cereals in the form of porridge have a similar long history to bread, there is a much shorter history of use for RTEC, but their presence on the market is nonetheless significant, with the first RTEC appearing over 100 years ago in the late nineteenth century(Reference Gibney, Barr and Bellisle22). The per capita consumption of RTEC in 2020 stands at 3·7 kg in France, 6·1 kg in the UK, 8·8 kg in Ireland, 6·5 kg in the USA and 5·2 kg in Canada(23).
Ready-to-eat breakfast cereals and breads: processing steps involved
In general, all cereal-based foods have common primary processing steps. They involve cleaning, sorting and removal of the inedible fractions from the cereals followed by milling. In contrast, secondary processing of cereals (or ‘adding value’) is the utilisation of the primary products (whole grains, flakes or flour) to enhance shelf-stability of cereal foods and make them sensorially more attractive for consumers. Any processing should be used as an opportunity to improve recipe formulations such as replacing refined grain with whole grain and reducing composition of added sugar, fat and salt, thereby helping products attain a healthier profile. The fundamental technology applied to both RTEC and bread is heat treatment in various forms to both RTEC and bread. Details of the main steps relevant to breads and flaked RTEC and their purpose are summarised below. Processing steps vary according to desired criteria of the endproduct. Describing the entire spectrum of processes would be beyond the scope of the present review; hence we confine ourselves to the principal processes involved in flaked RTEC and bread.
Flaked breakfast cereals
Depending on the desired properties of the endproduct breakfast cereal, the processes involved may vary considerably. Examples of processes include mixing, cooking, extruding, drying, cooling, tempering, flaking, drying/toasting and packaging. The US Environmental Protection Agency has schematically outlined the basic processing steps for flaked cereals and extruded flaked cereals as adapted here (Fig. 3).

Fig. 3. Traditional processes for flaked cereals (left panel) and extruded flake production (right panel), modified from the Ministry of Health of Brazil(116).
The initial preparation of the cereals for this process depends on the starter grain. For example, maize requires dry milling to remove the germ and the bran from the kernel, leaving the endosperm. Wheat kernels are first steamed and then gently passed through a pair of rolls to break open the kernels without producing flour at this point. Rice, on the other hand, requires only milling to form the polished head rice that is the normal starting material. The physical processes of flaking, puffing, shredding, extruding and expanding are sometimes described as ‘texturising’.
An important texturising step for flaked cereals is extrusion, a process which increasingly replaces the more traditional cooking and delumping steps. However, it is often overlooked that extrusion is widely used in the production of other foods, such as pasta, hence here we discuss extrusion for RTEC and pasta together. Since the 1930s, pasta and breakfast cereals have been produced using extrusion processes(Reference Akdogan24). In simple terms, for RTEC, extrusion and expansion alter the surface:volume ratios to allow for shaping and production of crisp, crunchy products, which addresses consumer demands. For pasta, extrusion enables a variety of different shapes that consumers can purchase, for example, spaghetti, campanelle, chifferi, farfalle, conchiglie and many others. The effectiveness of the extrusion step for either food form is dependent upon factors such as nutritional composition (for example, protein, fibre, carbohydrate content), structure (for example, particle size, proportion of bran) and moisture content achieved in the upstream processes. In general, cooking, drying and toasting, which are applied in traditional as well as extrusion manufacturing, introduce thermal energy to the products, with texturising operations applied during extrusion exerting additional mechanical energy on the dough(Reference Campbell, Mateos-Salvador, Klemeš, Smith and Kim25). Nowadays, those processes are often combined in one manufacturing (extrusion) line. Extrusion cooking of RTEC provides several advantages over traditional methods. Extrusion cooking allows for faster processing times, lower processing costs, and greater flexibility leading to greater variability of endproducts(Reference Alam, Kaur and Khaira26). From a consumer perspective, extrusion cooking creates cereals that are easy to chew and come in different shapes. During the manufacture of RTEC, extrusion cooking uses temperature, pressure and shear in varying intensities, depending on the types of ingredients and the desired characteristics of the endproducts(Reference Bartsch27). A modern manufactory line for extruded RTEC is shown in Fig. 4. These manufacturing lines allow very precise control of process parameters while ensuring high efficiency and variability in output, i.e. selection of RTEC to meet consumers’ demands. The effects of extrusion on nutritional quality are complex. Like many other processes that involve heating food at a high temperature before consumption, such as baking or frying, the nutritional quality may change during extrusion. As a principle, just like with pasta, the extrusion process for RTEC starts with mixing cereal grains into a dough. High temperature extrusion for a short time may minimise losses in vitamins and amino acids and improve protein quality and digestibility(Reference Harper28). On the other hand, fragmentation of proteins and starches may occur(Reference Camire29), the latter being implicated in an increased glycaemic response, possibly resulting in blood glucose elevation. In simplified terms, nutritional quality has been reported to improve with short duration, high moisture and low temperature, whereas negative effects on nutritional quality have been reported with high temperature (>200°C) and low moisture (<15 %)(Reference Singh, Gamlath and Wakeling30). More recently, it has been shown that introducing amaranth or buckwheat, as well as whole grain, significantly reduces the rate of carbohydrate hydrolysation in the human digestive tract, which positively affects the glycaemic index of the extruded RTEC or pasta(Reference Brennan, Menard and Roudaut31,Reference Brennan, Monro and Brennan32) . The glycaemic index is considered a marker for nutritional quality of carbohydrate-rich foods.

Fig. 4. Modern breakfast cereal manufacturing (provided courtesy of Bühler AG, Switzerland).
For RTEC, extrusion is often followed by coating, a process where for example heat-liable vitamins and/or sugar are added. Adding sugar during processing is fundamental for many different functions, not limited to caramelisation, and is responsible for inter alia colour and flavour aspects. Whilst such added sugar has a variety of purposes during processing, its excessive consumption has been implicated with dental caries and with increased risks for various chronic diseases including obesity, diabetes and CVD. The strongest body of evidence relates dental caries and frequency of intake of sugar-containing foods(33). However, to date, there is no firm conclusion that added sugar is related to the above metabolic diseases(Reference Rippe and Angelopoulos34), with the strongest available evidence for sugar-containing beverages rather than sugar per se (Reference Rippe and Angelopoulos34). Nonetheless, it would be prudent to consume added sugars in moderation.
Bread
Globally, the techniques for making bread can vary but essentially use four essential ingredients: flour, water, yeast and salt(Reference Buttriss35). Nonetheless, bread making is also a complex process that involves many physiochemical and structural changes to yield the final aerated baked product(Reference Ktenioudaki, Alvarez-Jubete and Gallagher36). Fig. 5 provides a general outline of key steps involved.

Fig. 5. Traditional process for bread making (sourced from BAKERpedia(126)).
Within the bread-making processes differences can also exist, particularly with respect to extraction rates during milling and fermentation processes used. A higher extraction rate allows for a higher percentage of outer grain layers to be retained with 100 % extraction rates yielding whole-grain flours. In contrast, white flours typically have lower extraction rates, as low as 70–72 %(Reference Ktenioudaki, Alvarez-Jubete and Gallagher36,Reference O’Connor37) .
Differences in fermentation can also arise depending on bread type (for example, sourdough) or whether mainstream mixing and fermentation processes are used. Sourdoughs (a commonly consumed bread type globally) are created when flour and water are mixed and then fermented with yeast and with homo- and hetero-fermentative lactic acid bacteria, increasing the lactic and acetic acid concentrations and resulting in a sour-taste product. The final taste can also vary depending on the cereal used (wheat, rye, oat, barley or maize), the microbial population present and ratio of bacteria:yeast used(Reference Gobbetti, De Angelis and Di Cagno38,Reference Vrcek, Cepo and Rasic39) . Some other popular types of bread globally include baguettes, ciabattas, various flat breads, rye or maize breads(Reference Gobbetti, De Angelis and Di Cagno38). However, in some countries, such breads may be viewed as artisan, where the term ‘bread’ is typically associated with white or brown pre-packaged or pre-sliced loafs or pans. Currently, bakeries and food business operators can use two main methods to make such prepacked bread which differ only with respect to their mixing and fermentation stages; the bulk fermentation process (BFP) and the Chorleywood bread process (CBP)(40). The BFP is the more traditional method where ingredients are mixed and the dough left to ferment for up to 3 h. During this fermentation phase, the mixture changes to form an elastic dough. In contrast, the CBP uses mechanical energy in the form of high-speed mixing as a type of kneading to develop the dough for proving and baking. A little fat or emulsifier and a treatment agent (for example, ascorbic acid) are typically added as an improver to aid this process. Soya flour may also be added to soften the bread, provide food for the yeast, hold water, keep the bread moist and help improve the natural colour of the bread. First developed in the 1960s in Chorleywood, UK, this CBP method was highly successful as it enabled a much faster production of bread to satisfy consumer need(40). Hence, the processes of making bread are complex and varied but ultimately result in a processed cereal product which is commonly consumed.
Comparison of processing of bread and ready-to-eat breakfast cereals
There are many steps common to the processing of both RTEC and bread. Using ‘wheat’ as an example, it is evident that the majority of generic processes for making a wheat-based flaked RTEC or bread are very similar (Table 1). Notable differences do exist such as fermentation for bread or steps like puffing, flaking, roasting or batch cooking which are applied to RTEC, or steps like extrusion to achieve the desired product characteristics. Extrusion is not applied in bread making (Table 1). One additional step which can be common to both cereal products involves the addition of vitamins and/or minerals to the mix or blend either as a restorative function to replace losses during extraction or as fortificants. We acknowledge that there are of course many variations to processing steps not only depending on the final product characteristics but also types of cereals used as raw materials. For example, traditional milling of oats first requires a thermal treatment to inactivate endogenous enzymes and prevent rancidity, followed by dehulling (dry-shelling)(Reference Papageorgiou, Skendi and Galanakis41). The more modern ‘green-shelling’ process, however, requires the oats to be shelled before kiln-drying(Reference Kent and Evans42). Furthermore, the nature of the endproduct will define which processes can be applied, for example, shredded cereals require different processing steps to the flake cereals described here. Nevertheless, when comparing the generic processing steps for wheat bread and flaked RTEC as illustrated here, it is evident that considerable similarities exist, with thirteen of the seventeen generic processing steps common to wheat-based bread and RTEC (Table 1).
Table 1. Overview of the generic processing steps for wheat-based cereals, highlighting those steps used in the manufacture of ready-to-eat breakfast cereal (RTEC) flakes and bread*
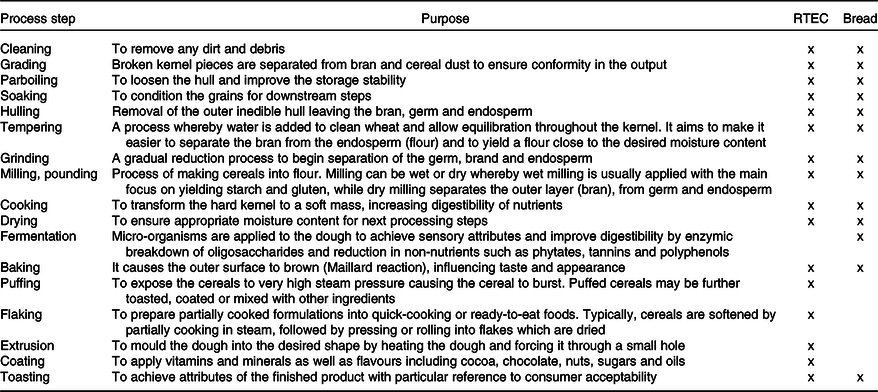
* Depending on the desired product, not every step will necessarily be involved in the manufacture of breads or RTEC.
Impact of processing on the nutrient and non-nutrient composition of bread and ready-to-eat breakfast cereals
The nutritional composition of the main types of whole cereal grains consumed by humans is shown in Table 2. In general, raw whole grains contain typically about 60–80 % carbohydrate along with some fat and protein. Whole cereal grains also contain considerable levels of many nutrients and non-nutrients due to the presence of functional molecules, dietary fibre, minerals, vitamins, lignans, phyto-oestrogens and phenolic compounds in their outer layers (bran, germ and endospermic tissue)(Reference Fardet43). Furthermore, whole-grain products contain more vitamins and minerals than their refined-grain counterparts. Processing of cereals and the associated removal of outer layers can alter their nutritional value; however, there are more facets to processing. The following subsections highlight the impact on composition following processing of cereals to bread and RTEC in more detail. Table 3 provides an overview of how the nutrient and non-nutrient composition may be altered during the manufacture of RTEC and bread and is described in brief below for energy and macronutrients and in the ‘Impact on micronutrients and non-nutrient compounds’ section.
Table 2. Nutritional composition of different whole grains and refined grains (per 100 g)*

* Obtained from the United States Department of Agriculture nutrient database(127).
Table 3. Overview of the influence of some processing steps on nutrient and non-nutrient content, highlighting steps in the manufacturing of ready-to-eat breakfast cereals (RTEC) and bread(Reference Alam, Kaur and Khaira26,Reference Camire29,Reference Oghbaei and Prakash44,Reference Slavin, Jacobs and Marquart48-Reference García-Estepa, Guerra-Hernández and García-Villanova50,Reference Suma, Sheetal and Jyothi56-Reference Thomson61)

* Typically including fractionation.
Impact on energy and macronutrient content
The endosperm, the large central part of the grain, is mostly composed of carbohydrates in the form of starch. Hence with milling and subsequent removal of the grain’s fibre fraction, this main constituent of cereals (starch) can become more easily accessible and digestible(Reference Oghbaei and Prakash44) and can lead to an increased glycaemic response following hydrolysis in the upper gastrointestinal tract. Only a fraction of starch in foods is not digested and absorbed in the small intestine, reaching the colon, where it is fermented to a variable extent by the microbiome. This fraction is called resistant starch. Resistant starch acts as a type of dietary fibre and has the ability to contribute to a healthy gut microbiota as described elsewhere(Reference Asp45-Reference Lockyer and Nugent47).
All cereals used to make RTEC and breads undergo milling to various extents (for example, whole grain, refined grains) and so final quantities of energy and macronutrients present are more influenced by the extent of milling and extraction rather than belonging to a particular commodity group. Cereals also contain protein and fat in addition to carbohydrates, but this is relatively less than other dietary staples, for example, dairy products or meat. Nevertheless, macronutrient composition can be affected by the level of processing. For one example, as part of the Maillard reaction the essential amino acid lysine has been described to become potentially unavailable when the dough is heated in the presence of reducing sugars, thereby altering protein amino acid profile(Reference Slavin, Jacobs and Marquart48). This would make an argument for using whole grains as the cereal source, because whole grain contains more protein than refined grain.
Impact on micronutrients and non-nutrient compounds
Although nutrients are found to varying degrees throughout the cereal, there is a higher concentration of micronutrients and fibre in the bran. Polyphenols such as ferulic acid or cinnamic acid are also present mainly in the outer layers of cereal bran, but phytosterols are reported to accumulate in both the bran and germ fractions(Reference Luithui, Baghya Nisha and Meera49). Hence, removal of the bran fraction during the primary processing step, milling, can result in reductions in a number of nutrients and non-nutrients present, for example, dietary fibre, tannins, polyphenols, minerals and some vitamins(Reference García-Estepa, Guerra-Hernández and García-Villanova50), the concentration of nutrients decreasing with the degree of milling(Reference Ramberg and McAnalley51). For example, Oghbaei & Prakash(Reference Oghbaei and Prakash52) reported a loss of vitamins in the range of 70–80 % when rice is milled. Alterations in dietary fibre composition and content will also depend on the level and type of processing and affect both soluble (for example, β-glucans) and insoluble forms (for example, arabinoxylans)(Reference Luithui, Baghya Nisha and Meera49). Although bran fibre can be added back in later as an ingredient, such losses may provide an argument to prefer whole-grain products or products with added bran fibre over refined-grain products. In contrast, the presence of naturally occurring inhibitors in whole grains such as phytic acid can render the bioavailability of divalent minerals (for example, Fe, Zn or Ca) low because of the formation of insoluble complexes(Reference Coulibaly, Kouakou and Chen53). However, phytic acid has also been recognised as a natural antioxidant(Reference Graf, Empson and Eaton54) which may potentially explain the protective effect of whole grain for colorectal cancer(Reference Aune, Chan and Lau55). Hence, the ‘right’ amount and method of processing can reduce or prevent this anti-nutritional activity(Reference Luithui, Baghya Nisha and Meera49) and perhaps confer protection for colonic health.
As mentioned, secondary processing techniques generally involve heat in some manner and are used to ‘add value to goods’ or variety to the diet. As evident in Table 3, the impact on nutrient and non-nutrient content is often dependent on the individual processing technique and while some are common to breads and RTEC, others are commodity and product specific. For example, the process of flaking has the potential to alter the content of P and dietary fibre but not Fe and Ca: using rice flakes as a medium, fibre and P content decreased in proportion to the thickness of the flakes, while Fe and Ca contents were reported as unaffected(Reference Suma, Sheetal and Jyothi56). In contrast, the process of fermentation in bread making (used to break down the tough outer hulls and increase cereal digestibility) may increase the content of phenolic compounds and enhance bioavailability(Reference Sandhu, Punia, Kaur, Gahlawat, Salar, Siwach, Duhan, Kumar and Kaur57). Furthermore, fermentation in combination with hydrothermal treatment and particle size reduction has been shown to reduce phytic acid(Reference Majzoobi, Pashangeh and Farahnaky58), which in turn could increase the availability of minerals that are otherwise bound by phytic acid. Fermentation is widely used in bread making. Other processes like soaking and germination reduce the phytic acid content in cereals(Reference Gupta, Gangoliya and Singh59). Extrusion seems to have no effect on mineral bioavailability(Reference McKevith60) or retention of vitamins, respectively(Reference Alam, Kaur and Khaira26). Another important mineral, iodine, appears relatively stable throughout the process chain, as it was retained to 100 % in the final products when applied as salts(Reference Thomson61). Salt is a key vehicle for fortification in some countries. Ferulic acid is the most abundant phenolic acid in wheat. There is evidence that ferulic acid increase up to 3-fold(Reference Alam, Kaur and Khaira26), while others point to the potentially increased antioxidant activities rather than content(Reference Ramberg and McAnalley51,Reference Nayak, Liu and Tang62) .
Cooking forms a central process for both RTEC and bread making, with cooking increasing the digestibility of nutrients and functional molecules(Reference Slavin, Jacobs and Marquart48). Dietary fibre has been shown to be relatively resistant to heat treatment(Reference Carnovale and Lintas63). The content of dietary fibre is hardly affected by extrusion cooking but a redistribution of insoluble to more soluble fractions has been reported(Reference Camire29) and increases with the intensity of the process conditions(Reference Björck, Nyman and Asp64). In addition, the content of resistant starch may be influenced by cooking processes. For example, a form of resistant starch called retrograded starch may be increased by the heat treatment and subsequent cooling(Reference Slavin, Jacobs and Marquart48). Optimal parameters for increasing this retrograded (resistant) starch in specific products, such as actual temperature, cooling temperature and duration of those steps, are not yet clearly defined. In addition, hydration may also affect the formation of resistant starch, by reducing its content. This is also true, in terms of extrusion cooking, where starch becomes fully gelatinised, resulting in a possible decrease in molecular entanglement and reduced resistant starch formation compared with other processing methods(Reference Vaidya and Sheth65). Approaches to modify the composition of resistant starch in products have been described elsewhere(Reference Ma and Boye66). Building on the point of replacing digestible starch with resistant starch, it has been demonstrated that processing techniques such as roasting, baking and boiling increased the content of resistant starch in cereal products, whereas steaming and frying had the opposite effect(Reference Vaidya and Sheth65). Also, the storage of different cereal products at 4°C up to 24 h significantly increased resistant starch content.
The ability of resistant starch to contribute to a healthy gut microbiota has been described in detail elsewhere(Reference Birt, Boylston and Hendrich46,Reference Lockyer and Nugent47) . Another key consideration relates to fortification. In countries where fortification is permitted, both RTEC and breads can contribute substantially to the intakes of certain nutrients. A final step in many bread-making steps is the addition of micronutrients to white flours either to restore those lost during extraction (restorative function) or as fortificants. For example, under UK law, white flours must be enriched with thiamin, niacin and Ca to combat nutrient losses in extracted white flours and to ensure minimum levels of these nutrients in the diet(67,68) . In the USA, thiamin, Fe and niacin must be added and since 1998 folic acid in a bid to reduce the occurrence of neural tube defects. Although no European countries currently fortify breads with folic acid, this is common in many other countries globally(Reference Crider, Bailey and Berry69). In contrast, RTEC, when fortified, may contain considerably greater numbers of vitamins and/or minerals and it is recognised that RTEC are one of the most highly fortified foods, helping to improve the overall nutritional status of consumers(Reference Caldwell, Kadan, Wrigley, Corke and Walker70). Their consumption with milk and milk products can also enhance nutritional intakes at meal times for key nutrients such as Ca, vitamin D (if fortified), riboflavin and K(Reference Priebe and McMonagle71).
Collectively, such processing techniques can increase or decrease the amounts and accessibility of a number of macro- and micronutrients in the two cereal products examined, even within categories. For example, using UK food composition data, consuming a breakfast consisting of one 40 g bowl of wholegrain fortified RTEC (for example, wheat biscuits, no milk) and two average slices of whole-grain bread (plain) would yield 293 kcal (1226 kJ), 60 g carbohydrates, 9·1 g fibre, 10·2 mg niacin, 318 mg K and 3·6 g of total sugars. On the other hand, choosing a fortified sugar-containing RTEC (for example, 35 g extruded type cereal, no milk) with white bread results in intakes of 297 kcal (1243 kJ), 64 g carbohydrates, 3·7 g fibre, 7·6 mg niacin, 175·8 mg K and 14·4 g of total sugars. Although energy profiles are similar, distinct differences with respect to fibre and some micronutrients exist. Simplistically, this implies that choice of products consumed over prolonged periods of time has significant potential to impact nutrient intakes at both an individual and population level, with healthier options available and needed across all categories to help individuals and populations meet dietary guidelines.
In conclusion, depending on the extent of primary and secondary processing steps involved, the final nutritional value of the by-products can vary greatly. While some common processes (for example, milling and refining) reduce the vitamin and mineral content of cereal, they can also enhance bioavailability of some nutrients as well as the digestibility and accessibility of phenolic compounds. Further, strategies can be employed to mitigate any risk of nutrient losses, for example, to choose whole-grain and bran-based products over refined-grain products, to use the processes of soaking, fermentation and germination to increase mineral bioavailability and reduce phytic acid content. Also, the addition of vitamins and/or minerals (restorative or fortification) will increase nutrient content. Collectively this suggests that by eating a wide variety of foods, as recommended as part of food-based dietary guidelines, we can benefit from a mix of nutrients which are bioavailable in different food forms. It also shows that there are no real differences in losses of nutrients depending on processing of the two major cereal types; both processes are similar.
The role of processing for safety in cereal-based food products
Generally, a fundamental reason for processing is food safety. Raw, unprocessed cereal grains can represent a substantial source of natural contaminants, specifically mycotoxins and heavy metals(72), with contaminants heterogeneously distributed within the kernel(Reference Alldrick, Wrigley and Batey73). Mycotoxins are poisons produced by fungi or can be toxins produced by moulds. There are many different mycotoxins that have been commonly found to contaminate cereal grains; these include aflatoxins, ochratoxin, fumonisins, deoxynivalenol, T-2 toxin, zearalenone and alkaloids. It has been pointed out that processing of cereal contributes to a substantial reduction in contaminants(Reference Kaushik74), where any risk to human safety is greatly lessened. In particular, large fractions of mycotoxins can be removed by appropriate post-harvest storage, sorting, cleaning, dehulling, and debranning reduction of damaged kernels, and fine material(Reference Cheli, Pinotti and Rossi75). These are processes common to cereals used for RTEC and bread. In addition, it has been suggested that the Se, Mg, Ca, Zn, Fe and dietary fibre, inherent in whole-grain RTEC and bread may have protective properties by reducing uptake and toxicity of heavy metals(Reference Thielecke and Nugent76). Whole-grain products with high levels of antioxidants, vitamins and carotenoids as well as superoxide anion scavengers, such as ferulic acid in whole-grain wheat, have the potential to reduce the impact of mycotoxins by protecting cell membranes from mycotoxin-induced damage(Reference Thielecke and Nugent76). Novel food processing techniques have been invented to further improve nutritional quality and advance production efficiency, while ensuring microbial safety. Grain-based foods can also become contaminated with plant toxins, for example, alkaloids or tropane alkaloids, but typically this is as a result of cross-contamination with toxin-containing plants rather than being integral to the grain itself. Processing to ensure safety of cereal-based products is predicted to become increasingly important in the context of climatic change, drive to organic sustainable farming and desire to abandon the use of chemicals in farming(Reference Alldrick, Wrigley and Batey77). Other examples of common toxins in food and present in the diet of most individuals are the process contaminants furan and acrylamide. The formation of both is linked to the Maillard reaction. This reaction enhances the taste of the cooked food items and often adds to the brownish colour of foods.
The formation of acrylamide relies on the presence of carbohydrates (reducing sugars such as glucose and fructose) and the amino acid asparagine when cooked at high temperatures such as in frying, roasting, toasting and baking(Reference Krishnakumar and Visvanathan78). Therefore, a variety of food products including French fries (chips), potato crisps, bread, breakfast cereals (excluding porridge), fine bakery wares, coffee and coffee substitutes as well as ‘baby food’ and processed cereal-based food intended for infants and young children(79) can be sources of acrylamide. Levels of acrylamide in products can range substantially and depend not only on processing but on growing conditions of the crops (i.e. cereal type, variety, growing conditions, climate) and methods used for analysis. The sourcing of wheat with lower free asparagine is currently not a viable option because it is commercially not available. Therefore, mitigation of acrylamide formation is mainly achieved through changes in product composition and/or process conditions. For example, extended fermentation time has been shown to result in dough with lower levels of acrylamide in bread(Reference Krishnakumar and Visvanathan78). EU authorities have provided effective mitigation measures as well as regulatory benchmarks for acrylamide levels in food products including RTEC (150–300 µg/kg) and soft bread (50–100 µg/kg)(80). In comparison, the benchmarks for roast coffee is 400 µg/kg, French fries 500 µg/kg and potato crisps 750 µg/kg. The regulatory benchmarks are typically lower for bread and RTEC than for other commodities.
Furans are another class of contaminant, potentially formed in foods during thermal processing. The formation of furans requires several precursors such as ascorbic acid, amino acids, carbohydrates, unsaturated fatty acids and carotenoids, and they are found in a variety of foods including coffee and canned foods(Reference Knutsen, Alexander and Barregård81). The presence of furans in the diet, and any implication for public health are closely monitored by the authorities. For example, in Europe, EFSA has characterised that grain and grain-based products are chief sources of furans in children, but not in adults. In adults, coffee is the main contributor of furans(Reference Kettlitz, Scholz and Theurillat82). Mitigation strategies depend on the food product and include lowering the thermal load, and adding antioxidants that delay lipid oxidation(Reference Kettlitz, Scholz and Theurillat82). However, specific mitigation strategies are still under investigation(Reference Kettlitz, Scholz and Theurillat82).
Taken together, many of the core processes used to manufacture bread and RTEC can decrease the presence and/or amounts of contaminants, while best practices can mitigate the risk of forming any process-related undesired substances, for example, furans and acrylamide, thereby ensuring food safety.
The roles of ready-to-eat breakfast cereals and bread for the human diet
The contribution of processed foods in general, as defined by the International Food Information Council, to nutrient intake has been described(Reference Weaver, Dwyer and Fulgoni83). For example, in 2014 the American Society for Nutrition reported that, for the USA, processed foods contributed 57 % of energy, 55 % of dietary fibre, 48 % of Ca, 43 % of K, 34 % of vitamin D, 64 % of Fe, 65 % of folate and 46 % of vitamin B12 intakes. On the other hand, processed foods contributed 52 % of saturated fat, 75 % of added sugars and 57 % of Na. Within this, the American Society for Nutrition concluded that the level of processing was of secondary priority as long as nutrient-dense foods are selected(Reference Weaver, Dwyer and Fulgoni83).
For RTEC and bread (both processed foods), their role as part of healthy balanced diets has been acknowledged(Reference O’Connor37,Reference Thomson61,Reference McKevith and Jarzebowska84-Reference Weegels86) . For both foods, options that are made with whole grain and are high in fibres makes them a valuable source of key nutrients such as vitamins and minerals, antioxidants(Reference Toma and Curtis85,Reference Miller, Rigelhof and Marquart87) and other plant actives(Reference O’Connor37,Reference Ryan, Thondre and Henry88) . Therefore, whole grain should be the preferred option; however, in reality, although the available choices of whole-grain foods are increasing globally, the majority of bread and RTEC are still consumed as refined grain.
Contribution to nutrient intakes
Both RTEC and bread make significant contributions to population nutrient intakes. Healthy adult habitual breakfast cereal consumers are reported to have a higher proportional intake of energy from carbohydrates and lower intake of total and saturated fats than non-consumers(Reference Priebe and McMonagle71,Reference Weegels86) . Further, consumption of breakfast cereals has been associated with healthier dietary patterns in adults and children(Reference Priebe and McMonagle71) and to increase intake of associated foods such as milk and fruit(Reference Michels, De Henauw and Beghin89). Portion sizes associated with such benefits are typically moderate, for example, in a European cohort the average portion size of cereals consumed by adolescents was 35 (interquartile range 24–56) g(Reference Michels, De Henauw and Beghin89); this RTEC-consuming group also reported lower intakes of energy and fat but higher protein, carbohydrate and fibre when compared with adolescents consuming mostly bread for breakfast. Inclusion of RTEC in the diet may also yield higher intakes of vitamins and minerals and lower fat intakes but without influencing intakes of energy or Na(Reference Priebe and McMonagle71,Reference Laskowski, Gorska-Warsewicz and Rejman90,Reference Williams91) . In particular, frequent consumption of RTEC (> 5 times per week) has been associated with a lower risk of inadequate intakes of vitamin A, Ca, folate, vitamin B6, Mg and Zn. However, the nutritional merits of some RTEC have been questioned because of the content of sugar, salt and saturated fat in some products(Reference Williamson92), while RTEC consumption was also found to be associated with higher sugar intake(Reference Priebe and McMonagle71). Against this context, it is worth remembering that total dietary sugar intake will also be influenced by the intake of naturally occurring milk sugars following consumption of RTEC with milk, while the salt and added sugar composition of RTEC has decreased over the last decade(Reference Williamson92). On the other hand, RTEC can act as an important source of whole grain and fibre(Reference Devlin, McNulty and Gibney93), with some products providing about 50 % of its ingredients as whole grain. RTEC also have a substantial contribution to people’s nutrient intake; for example, in France, with a relatively low consumption of breakfast cereals, the overall contribution of breakfast cereals at breakfast alone is 14·7 % of energy, 19·2 % of carbohydrates, 7·9 % of protein, 20·3 % of fibres, 10·5 % Ca and 33 % Fe(Reference Bellisle, Hébel and Salmon-Legagneur94). However, in Canada, a country with a relatively high consumption of breakfast cereals, for an adult cohort, 9·3 % of the daily energy was reported from RTEC, this category also contributing 15·3 % carbohydrates, 5·7 % protein, 22·7 % fibres, 5·5 % Ca and 32·2 % Fe to the daily intake of these nutrients(Reference Vatanparast, Islam and Patil95).
For bread, it is reported that EU citizens consume approximately 50 kg of bread per person per year or about three to four slices of white bread per d, with citizens of Germany and Austria consuming slightly more and those of the UK and Ireland less(96). Outside of the UK (where vitamins and minerals are re-added to white flour after milling), breads made with whole-grain flours will generally yield a higher composition of fibre and vitamins (for example, thiamin, niacin, folate) and minerals (for example, Fe, Zn and Mg)(Reference O’Connor37). However, the exact contribution will depend on the type of flour used(Reference Weegels86,96) . For example, in the UK, total bread is reported to contribute approximately 12 % of total daily energy intake, 16–20 % of carbohydrate, 10–12 % of protein, up to 21 % of fibre intakes, 12–17 % of total Ca and 15–17 % of total Fe intakes(Reference Lockyer and Spiro97) (although the bioavailability of Fe powdered to fortify flour has been debated)(98). Similar contributions are reported for France, where bread contributes to 15·5 % of daily energy intake, 27·2 % of carbohydrates, 2·7 % of fat, 20·3 % of fibres, 3·5 % of Ca (only non-fortified sources) and 14·4 % of Fe to the daily intake of these nutrients(Reference Julia, Martinez and Alles99).
Some health benefits of eating ready-to-eat breakfast cereals and bread
There is a significant body of evidence researching the influence of RTEC and various health outcomes(Reference Williams91). For example, breakfast cereals have been reported to help lower cholesterol concentrations or improve bowel function, if high in oat, barley or fibre, respectively(Reference Williams91). Despite concerns that pre-sweetened breakfast cereals could increase the risk of overweight and obesity in children, this is not supported by current reviews(Reference Williams91). Such effects (positive or negative) primarily relate to the composition of the foods (nutrient or otherwise), with the role for processing simply to make healthy choices as palatable as possible. For bread, specifically wheat bread and wheat products, there have been concerns linking wheat products (as part of a Western diet and lifestyle) and increasing prevalence of obesity, type 2 diabetes, allergy and food intolerances(Reference Shewry and Hey100). Such views have been particularly popular in the press and media and are despite scientific reviews to the contrary(Reference Shewry and Hey100). There are also suggestions that modern bread wheat has different health benefits from traditional wheat types, but again there is, to date, insufficient evidence to confirm this theory(Reference Shewry101). Nonetheless, the consumption of bread, particularly whole-grain bread has been linked with reduced mortality(Reference Jacobs, Meyer and Solvoll102).
For both RTEC and bread, it is likely that the benefits arise from the composition of the grains and the nutrients and non-nutrients contained within the matrix. For example, benefits with respect to cholesterol or postprandial glucose concentrations may relate to the presence of β-glucans with associated health claims approved in Europe by the EFSA(103). Also, given that both foods can contain considerable amounts and types of dietary fibre, not least, arabinoxylan, resistant starch and lignin, this can have benefits with respect to gut health and can influence glycaemic response(Reference O’Connor37). Specifically, resistant starch has been consistently shown to reduce the glycaemic response, which resulted in an approved EFSA health claim(Reference Lockyer and Nugent47). Furthermore, EFSA also acknowledges health benefits of wheat bran fibre(104). On the back of considerable observational evidence showing that individuals who consume more whole grain have a lower risk of chronic disease(Reference Seal, Nugent and Tee105), this has encouraged countries/agencies to issue dietary intake recommendations for whole-grain options of both these processed dietary staples in an effort to improve diet quality and enhance health(Reference Seal, Nugent and Tee105).
Challenges of defining cereal-based products by level of processing
Current debate suggests grouping foods by level of processing(Reference Monteiro, Cannon and Levy17). In analyses of diet and health, grouping of foods and/or nutrients is common and has a strong historic background. For example, food groups are central to population dietary advice as part of food-based dietary guidance or as more complex food categorisations or coding systems within the scientific literature. There are many well-established examples of such coding systems, for example, the Langual (Langua alimentaria) system (first developed by the US Food and Drug Administration in the 1970s and which has since been modified and adopted by the EU consortium, Eurofir(Reference Becker, Møller and Ireland106,Reference Ireland and Møller107) ) and systems created by EFSA (Food Ex)(108) and the European Prospective Investigation into Cancer and Nutrition (EPIC) Consortium(Reference Slimani, Deharveng and Southgate109). Within these generally faceted systems comprehensive information on food descriptors is gathered, not least food source, cooking method, ingredients, use of fortificants and preservation technique (i.e. including some account of processing). Such coding systems are used to account for processing both with respect to influence of processing on exposure to food chemicals(108) or the role of nutrients or phytochemicals on disease risk(Reference Slimani, Deharveng and Southgate109).
Nevertheless it has been argued that the significance of processing and potential impact on health has been underestimated(Reference Monteiro16,Reference Monteiro, Cannon and Levy17) and hence alternative categorisations or descriptors have been developed which depend solely on the level of processing. For example, that of the International Food Information Council(9) which relates to five categories of processing (1) minimally processed, (2) processed to preserve or enhance nutrients or freshness, (3) combining foods with ingredients to enhance sensory and/or safety appeal, (4) ready-to-eat foods, (5) foods packaged to stay fresh and save time or the NOVA categorisation(Reference Monteiro16,Reference Monteiro, Cannon and Levy17) where foods can be divided into four categories (1) unprocessed and minimally processed, (2) processed culinary ingredients, (3) processed foods, (4) ultra-processed foods. Confusingly, depending on which categorisation scheme is used, a food may be listed as processed in one scheme but highly or ultra-processed using another coding system. There is currently no scientific consensus on how processing should be addressed as part of food coding systems, particularly with respect to method of processing (traditional, novel), number of ingredients, use of legally permitted food additives, etc. While such debate continues, it is unclear how this information can be used to scientifically measure trends in dietary quality or the relationship of diet with chronic disease(Reference Knorr and Watzke10,Reference Lecerf110) . Further, it is unclear how such newer categorisation systems satisfy the generally accepted principles underpinning general population dietary guidance are that they should be understandable, actionable, affordable and safe(Reference Jones14). There may be learnings on those principles from the French dietary guidelines, which recently discouraged the consumption of ultra-processed foods(111). As identified elsewhere, any shift in the use of food coding systems, particularly in studies relating to states of health or ill-health, should be accompanied by objective validation, use of biomarkers where possible and careful interpretation of results(Reference Leme, Fisberg and Thompson112).
Finally, consideration must also be given to how such newer categorisation systems are communicated beyond scientific audiences. Consumer insights suggest that simple and realistic nutrition messages are welcome(Reference Webb and Byrd-Bredbenner113), and such new initiatives have led to economic opportunities for the development of phone-based applications and the creation of commercial companies (for example, https://siga.care/). However, given that there is no agreed consensus globally on ways to categorise foods such as cereals according to level of processing or more critically how any such categorisation could impact population dietary behaviour, care must be exercised to avoid confusion and distrust among consumers and to avoid any decreases in intakes of key nutrients which cereal products can provide, including those from fortified products.
Future considerations
An increasing focus of consumers as well as of public health authorities is sustainable diets. Indeed, the notion of shifting current dietary patterns towards more sustainable systems that carry environmental as well as health benefits has been favoured by the FAO as part of their 2010 definition of sustainable diets(114). Sustainability aspects have since been included in national dietary guidelines by countries such as Sweden(115), Brazil(116) and the Netherlands(117) with mention of similar discussions in the UK(Reference Steenson and Buttriss118). Parallel with this background of sustainability is forecasted world population growth, estimated to reach approximately 9·2 billion in 2050(119). Therefore, the world faces the challenge to meet increasing demands of key nutrients but in a sustainable manner. Food processing has traditionally represented an important link between production and consumption of food. In order to provide healthy diets and a maintainable food supply for an ever-growing population, all food processors (including those of grain products) must also consider the challenge of sustainable diets as a matter of concern and action.
Cereals, particularly whole grain, are nutrient-dense. They provide energy as well as significant contributions to population intakes of carbohydrate, protein, fibre and many micronutrients. This is often by virtue of their frequent consumption by large segments of the population. For example, in the UK, white bread, although containing about 155 mg Ca/100 g contributes approximately 12–17 % to population Ca intakes, particularly in groups such as children and adolescents(120). Hence, all cereals need to be regarded in helping meet increased demands for these key nutrients globally.
However, the environmental impacts of RTEC or bread and their role as part of future sustainable diets have not been assessed broadly in scientific papers. One paper, using the Dutch diet as the basic setting, applied a model comparing bread and RTEC, as well as dairy products and meat between 0 and 250 % of the current intake with the relationship between the quantity of these food products and the environmental impact as well as the nutrient balance of these diets examined(Reference Kramer, Martinez and Espinoza-Orias121). The authors found that RTEC and bread had a better environmental performance compared with dairy products or meat while still maintaining a high nutrient balance. Others assessed the influence of breakfast cereal production on land use and biodiversity; they identified cocoa cultivation as a major contributor to the loss of biotic production(Reference Leme, Fisberg and Thompson112), with maize and rice representing further factors. Also, using life-cycle assessment tools, the environmental impact of breakfast cereals, limited to breakfast cereals produced by Kellogg’s Europe, has been explored. Given the small quantities of cocoa across all Kellogg’s Europe breakfast cereals (<5 % by mass), the finding underpins the substantial impact of the use of cocoa in all food manufacturing, considering that cocoa is used in a variety of food categories other than RTEC(Reference Jeswani, Hellweg and Azapagic122). When it comes to assess sustainability of diets/food groups, the whole life cycle of a product, including, for example, packaging and distribution of products, needs to be considered. A comparison between the traditional (‘bag-in-box’ packages) and loose delivery (low-density polyethylene) revealed a potential of up to 85 % waste reduction(Reference Dolci, Nessi and Rigamonti123). However, boxes may serve many purposes and the choice of bag material to ensure consistent quality and safety of the products also needs to be taken into account. An interesting approach to further improve the carbon footprint of RTEC and bread, while maintain or increasing nutrient balance scores, involves the replacement of refined flours by pulses(Reference Chaudhary, Marinangeli and Tremorin124). When combining carbon footprint and nutrition into one score, the authors showed that yellow pea flour decreased the nutrition carbon footprint score by 15 % for pan bread and 90 % for breakfast cereal. This example for cereal-based food shows that there are opportunities to improve carbon footprint and nutrition value simultaneously.
To address the above demands the EAT–Lancet Commission suggests optimising diets for sustainable food systems predominantly by a shift of diets to plant-based diets(Reference Willett, Rockstrom and Loken125). Naturally, there are considerable challenges in shifting populations’ diets in this direction, costs of fruit and vegetables being one. Cereals already contribute significantly to the energy supply globally, and they can and should play a more dominant role in providing energy and nutrients in a sustainable way to meet demands for increasing global population.
Summary
Cereals have been consumed by humans since approximately 100 000 years ago. Cereals have to be processed in some way in order to be consumed by humans. Cereal processing is one of the oldest and given the role of cereals for its contribution to meeting nutrition requirements, one of the most important food technologies. Over the centuries, just like in any other food category, processing technologies have evolved in order to ensure safe products. Modern processes for RTEC and bread are efficient in providing sufficient products of good quality and to meet consumer expectations by providing a range of cereal foods that allow for a safe, varied and balanced diet. RTEC and bread share fundamental primary processing steps and many of the secondary processing steps. Potential reduction of nutrients can partly be balanced by choosing whole-grain varieties over refined-grain products as well as by fortification. On the other hand, processing enhances digestibility and bioavailability (for example, for minerals and phenolic acids) as well as reducing non-nutrients like phytic acid and contaminants. Based on the available scientific evidence to date and in comparison with more traditional approaches, the level of processing alone does not appear to act as a useful measure for the healthfulness of foods. Clearly, RTEC and bread play an important role in human nutrition by delivering important nutrients and are positively linked with intake of dietary fibre, certain vitamins and minerals, especially when consumed as whole grain. Therefore, whole-grain options should be the preferred choice. Cereal foods are part of the solution for sustainable diets as well as meeting the increasing global demand for energy and nutrients. From a public health perspective, it makes sense that food-based dietary guidelines in many countries already include cereal foods and specifically mention bread and breakfast cereals. It would be desirable to further strengthen the role of processed cereal foods by including more whole-grain, bran-based and fibre-rich options and developing universally agreed quantitative recommendations for whole grain consumption.
Acknowledgements
The present review was supported by CEEREAL e.V. who had no role in the design, analysis or writing of this review.
F. T. and A. P. N. conceived the idea of this review paper. F. T., A. P. N. and J.-M. L. wrote the review. All authors read and approved the final version of the manuscript.
F. T. is consultant to CEEREAL e.V. and received research financial support for conducting the literature search and drafting the manuscript. A. P. N. and J.-M. L. declare no conflicts of interest.