Introduction
Safe and adequate nutrition is crucial for the proper development of children. Other than breast milk, infant formulas and baby foods are the most important part of a baby's diet in the first year of life. Infant formulas, available in powder, liquid-concentrate and ready-to-feed forms, are artificial substitutes mimicking human breast milk. They are based on cows' milk or soya milk, designed for the consumption of infants. The medical community considers infant formulas as nutritionally acceptable for infants under the age of 1 year when breast-feeding is not possible. Infant formulas can be modified based on scientific evidence about the nutrient needs of infants(1, 2). Baby food is any food given to infants, with a soft, liquid texture, or that is chewed, from the age of 6 months to 2 years(2–Reference Joeckel and Phillips4). Baby foods are also consumed for 3 years as a part of the child's diet(1, 2). Baby foods include several baby purées made from fruits, vegetables or vegetables plus meat (beef, chicken or fish) sold commercially.
Formulation, handling and storage of baby foods are important for the foods' nutritional quality and physico-chemical properties(Reference Nasirpour, Scher and Desobry3). There are several nutrients that an infant formula must contain according to the Food and Drug Administration through the advice of the American Academy of Pediatrics; it is also necessary to set upper limits for a nutrient in the formula(Reference O'Connor5, Reference Wharton6). On the other hand, infant formulas or baby foods can contain several contaminants that carry potential risk during the first years of life. A contaminant is an impurity; any material of an extraneous nature associated with a chemical, a pharmaceutical preparation, a physiological principle, or an infectious agent. Several contaminants, which can come from different stages of production and storage, may be present in infant formulas and baby foods. Food consumption is an important route of human exposure to contaminants such as acrylamide, pesticides and industrial pollutants(Reference Turconi, Guarcello and Livieri7, Reference Booker8). As acrylamide is an anticipated contaminant of baby foods and infant formulas, and as children generally have acrylamide intakes that are two to three times those of adults when expressed on a body-weight (BW) basis, we will focus on acrylamide and its presence in baby foods and infant formulas in the present review(9).
Properties of acrylamide
Acrylamide (acrylic amide; International Union of Pure and Applied Chemistry (IUPAC) name 2-propenamide), an α,β-unsaturated (conjugated) reactive molecule, has the chemical formula C3H5NO(10, Reference Fohgelberg, Rosén and Hellenäs11). It has a high mobility in soil and groundwater and is biodegradable(Reference Friedman12). It is also used in industry to manufacture polyacrylamide or its co-polymers(Reference Friedman12, Reference Wenzl, De La Calle and Anklam13).
Acrylamide is inadvertently found in starchy foods such as crisps, chips and bread(Reference Miller, Carter and Sipes14). Its discovery in heated foods has received a high level of attention from the public and the medical community. Acrylamide in fried and baked foods is produced by the reaction between asparagines and reducing sugars (fructose, glucose) or reactive carbonyls(Reference Viator and Muth15, Reference Choe and Min16). Coffee drinking and smoking are other major sources apart from the human diet(Reference Schettgen, Weiss and Drexler17, Reference Lantz, Ternité and Wilkens18). Boiling does not produce acrylamide, but baking, frying, deep-frying, over-cooking and microwaving produce large amounts of acrylamide in foodstuffs(Reference Miller, Carter and Sipes14). On the other hand, water is one of the major sources of exposure, as polyacrylamide is used mainly for the purification of drinking water as a flocculating agent(Reference Friedman12, Reference Wenzl, De La Calle and Anklam13).
Acrylamide is classified as ‘probably carcinogenic to humans (group 2A)’ by the International Agency for Research on Cancer. There is inadequate evidence in humans for the carcinogenicity of acrylamide(10).
Biotransformation of acrylamide
Absorption
According to data derived from animal studies, acrylamide is quickly absorbed by the skin and by the mucosa if inhaled. If taken by the oral route, it can diffuse evenly in the body because of its hydrosolubility(Reference Fohgelberg, Rosén and Hellenäs11).
Distribution
Tissue distribution is not significantly affected by dose or route of administration. The highest concentrations are found in erythrocytes. Despite the prominence of neurological effects, acrylamide is not concentrated in nervous system tissues(Reference Dearfield, Douglas and Ehling19). Acrylamide readily crosses the placenta(Reference Edwards20).
Metabolism
In blood, acrylamide has a half-life of approximately 2 h. In tissues, total acrylamide (parent compound and metabolites) exhibits biphasic elimination with an initial half-life of approximately 5 h and a terminal half-life of 8 d. Acrylamide does not accumulate in the body(Reference Miller, Carter and Sipes14, Reference Edwards20, Reference Bergmark, Calleman and He21).
At low doses 50 % of acrylamide is oxidised to a DNA-reactive epoxide, glycidamide, by cytochrome 2E1 (CYP2E1). CYP2E1 polymorphisms in mice can cause different amounts of glycidamide to be formed. Wild-type mice have been found to metabolise 50 % of the administered dose of acrylamide to glycidamide; however, CYP2E1-null mice could not metabolise acrylamide to glycidamide(Reference Jägerstad and Skog22). Glycidamide can be metabolised by epoxide hydrolase or can undergo conjugation with glutathione (GSH)(Reference Friedman12, Reference Bergmark, Calleman and He21, Reference Sumner, Fennell and Moore23–Reference Carere25).
Excretion
The major pathway of metabolism for acrylamide is its conjugation with reduced GSH by glutathione S-transferase. Conditions such as malnutrition, oxidative stress and liver disease (alcoholic hepatitis, cirrhosis, and other malignant liver disorders) can decrease the GSH content of liver. Since a child's liver cannot carry as high a burden as an adult liver, especially under such conditions, a higher toxicity of acrylamide might be anticipated in children. Elimination occurs mainly in the urine as mercapturic acid conjugates. Greater than 90 % of absorbed acrylamide is excreted in the urine as metabolites. Less than 2 % is excreted as unchanged acrylamide. Smaller amounts are excreted in the bile and faeces. Approximately 60 % of an administered dose appears in the urine within 24 h(Reference Miller, Carter and Sipes14). Biotransformation of acrylamide is summarised in Fig. 1.
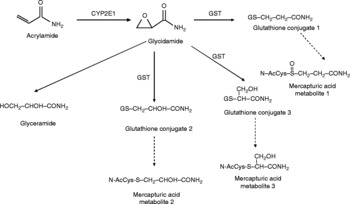
Fig. 1 Biotransformation mechanism of acrylamide. CYP2E1, cytochrome 2E1; GST, glutathione S-transferase.
Toxicity of acrylamide
There are several health risks caused by the intake of acrylamide from several routes. Smoking by the mother is a major way by which the neonate is exposed to acrylamide(Reference Hilbig, Freidank and Kersting26). In addition, contaminated foodstuffs and drinking water are two other main ways to expose the baby or child to acrylamide. As children, especially those under the age of 2 years, are the most sensitive population, acrylamide intake levels must be monitored particularly in infant formulas and baby foods. On the other hand, mothers should be attentive while choosing the water with which they prepare food for their child. The health risks of acrylamide can be classified as follows.
Mutagenic, genotoxic and carcinogenic properties of acrylamide
The genotoxic, mutagenic and carcinogenic potentials of acrylamide have been studied extensively. Acrylamide itself reacts rapidly with thiol (–SH) and amino groups; this explains why its primary targets are proteins(Reference Carere25). Acrylamide has been shown to bind DNA by a Michael-type process in vitro with low activity(Reference Bergmark, Calleman and He21, Reference Carere25). Nevertheless, there is sufficient evidence in the literature that both acrylamide and its metabolite glycidamide are mutagenic and clastogenic in mammalian cells(Reference Dearfield, Douglas and Ehling19, Reference Jägerstad and Skog22). Data suggest that mice are more vulnerable to acrylamide tumorigenicity. The metabolic activation of acrylamide is more efficient and the detoxification process is poorer in mice than rats, since mice have higher levels of glycidamide and lower levels of GSH–glycidamide conjugates(Reference Sumner, Selvaraj and Nauhaus27).
Acrylamide causes induction of the following genotoxic effects(Reference Rice28, Reference Hashimoto and Tanii29):
(a) gene mutations and chromosomal aberrations in germ cells of mice in vivo;
(b) chromosomal aberrations in germ cells of rats in vivo;
(c) chromosomal aberrations in somatic cells of rodents in vivo;
(d) gene mutations and chromosomal aberrations in cultured cells in vitro;
(e) cell transformation in mouse cell lines;
(f) somatic mutation in the spot test in vivo;
(g) heritable translocation and specific locus mutations in mice and dominant lethal mutations in both mice and rats;
(h) unscheduled DNA synthesis in rat spermatocytes in vivo; but not in rat hepatocytes. However, glycidamide induces unscheduled DNA synthesis in rat hepatocytes.
The studies on mutagenic, genotoxic and carcinogenic properties of acrylamide and glycidamide are summarised in Table 1(Reference Hashimoto and Tanii29–Reference Mei, McDaniel and Dobrovolsky43).
Table 1 Summary of literature on mutagenicity, genotoxicity, carcinogenicity, neurotoxicity, reproductive and developmental toxicity of acrylamide

CHO, Chinese hamster ovary; CYP450, cytochrome 450; ppm, parts per million; GD, gestation day.
Adduct formation with Hb
Acrylamide forms different protein adducts, the most important of which are Hb adducts extensively formed at –SH groups and on the amino groups of the N-terminal valines in erythrocytes. The measurement of Hb adducts can give an integrated measure of the exposure in the previous 3–4 months, since the lifespan of erythrocytes is about 4 months(Reference Carere25, 44).
Adduct formation with acrylamide shows a linear dose–response relationship and glycidamide adducts generate a concave curve. This shows that the percentage of acrylamide metabolised to glycidamide is inversely proportional to the administered dose of acrylamide(Reference Calleman, Stern and Bergmark45, Reference Bergmark, Calleman and Costa46).
Neurotoxic effects of acrylamide
Early morphological studies suggested that neurological defects caused by acrylamide intoxication are mediated through distal axonal degeneration (also known as ‘dying-back’ neuropathy) in the peripheral nervous system and central peripheral nervous system(Reference Höke47, Reference Murray48). Later studies showed that nerve terminals and Purkinje neurons are the targets of acrylamide(Reference Cavanagh49). Nowadays, there are two important hypotheses regarding acrylamide neurotoxicity: (a) inhibition of kinesin-based fast axonal transport; (b) direct inhibition of neurotransmission(Reference LoPachin, Jones and Patterson50, Reference LoPachin51).
Acrylamide, the parent compound, is a soft electrophilic neurotoxicant, reacting on thiol groups of proteins (cysteine, homocysteine) and GSH as well as protein-bound –SH groups (kinesin, dynein), whereas the metabolite glycidamide is a harder electrophilic compound, reacting with nucleophilic centres of adenine and guanine in the DNA(Reference Ma, Wang and Yang37–Reference Wolf, Niehaus-Rolf and Banduhn41). It has been shown that acrylamide inhibits the action of brain glutathione S-transferase and reduces the levels of brain GSH(Reference LoPachin, Ross and Reid52). It has also been suggested that acrylamide neurotoxicity is caused by its effects on heavy- and medium-weight neurofilaments, the change it causes on neurotransmitter receptor expression and through inhibition of neurotransmission(Reference Mei, McDaniel and Dobrovolsky43–Reference Calleman, Stern and Bergmark45). Electrophilic neurotoxins, including acrylamide, may cause protein structure and function changes by oxidation and this may lead to pathway failure and finally nerve cell damage. Therefore such chemicals at low doses and long-term exposure might be a cause of neurodegenerative diseases such as Alzheimer's disease(Reference Friedman12, Reference Fu, Von Tungeln and Hammons35, Reference LoPachin and DeCaprio53, Reference LoPachin and Gavin54). The studies on neurotoxic effects of acrylamide and glycidamide are summarised in Table 1(Reference Hashimoto and Tanii29, Reference Fu, Von Tungeln and Hammons35, Reference Burek, Albee and Beyer55–Reference Ghanayem, Bai and Kissling57).
Reproductive and developmental toxicity of acrylamide
Reproductive toxicity has also been observed in laboratory animals exposed to high levels of acrylamide(Reference Burek, Albee and Beyer55). Several studies have been conducted on reproductive toxicity; these are presented in Table 1(Reference Zenick, Hope and Smith58–Reference Tyl, Marr and Myers62).
There seems to be a relationship between the neurotoxicity and reproductive toxicity of acrylamide(Reference Chapin, Fail and George56, Reference Tyl, Marr and Myers62–Reference Miller, Mulholland and Vogl65). One theory is that reproductive toxicity is related to neurotoxicity, as neurotoxicity influences mating behaviour. Several studies have clarified that some of the neurotoxic effects of acrylamide in rats are a weakness of the hind-limbs, reduced hind-limb grip strength, and increased foot splay(Reference Tyl, Marr and Myers62, Reference Sakamoto and Hashimoto63, Reference Miller, Mulholland and Vogl65). This reduced hind-limb function could cause impairment in mounting responses, copulatory behaviour, and intromission (entry)(Reference Zenick, Hope and Smith58). Dysfunctional intromission could also have an impact on the proper deposition of sperm in the vagina and uterus and subsequent hormonal events that cause the stimulation of reproductive hormones and implantation. In addition, erectile function could be decreased due to nerve damage in the penis(Reference Tyl and Friedman66). Another theory is that both types of toxicity are mediated through effects on the kinesin motor proteins(Reference Tyl, Marr and Myers62). These kinesin proteins are found in the flagella of sperm as well as the nervous system and other tissues(Reference Miller, Mulholland and Vogl65). Interference with these proteins could reduce sperm motility and fertilisation events(Reference Tyl, Marr and Myers62, Reference Tyl and Friedman66, Reference Friedman, Tyl and Marr67–Reference Sickles, Brady and Testino70).
Other mechanisms of acrylamide on reproduction in rodents could be from the alkylation of sulfhydryl groups on unique proteins, such as protamine, in the sperm head and tail(Reference Jägerstad and Skog22, Reference Sega, Alcota and Tancongco71, Reference Sega72). This could affect sperm penetration and induce the pre-implantation losses seen in some dominant lethal studies(Reference Tyl, Marr and Myers62, Reference Lähdetie, Suutari and Sjöblom73).
No human data are present regarding the reproductive and developmental toxicity of acrylamide. However, data are sufficient to conclude that acrylamide is a reproductive and developmental toxicant in rodents.
Dose–response and dose–effect relationships of acrylamide
Numerous investigators have looked at dose–response and dose–effect relationships in a variety of animal models. There do not appear to be significant differences between the mammalian species studied. The lethal dose, 50 % (LD50) levels of acrylamide through various routes in different rodents are given in Table 2(Reference Manson, Brabec and Buelke-Sam74).
Table 2 Lethal dose, 50 % (LD50) levels of acrylamide through different routes in different rodents

The WHO states that acrylamide belongs to the group of chemicals thought to have no reliably identifiable ‘threshold’ of effects, meaning that very low concentrations will also result in very low risks, but not in zero risk: some risk is always present when the chemical is ingested. However, for carcinogens such as acrylamide, risk is thought to increase with increasing exposure(75).
In June 2002, the FAO of the UN and the WHO issued a report about the health implications of acrylamide in food. The Consultation concluded that the no observed adverse effect level (NOAEL) for acrylamide neuropathy is 0·5 mg/kg BW per d and the NOAEL for fertility changes is four times higher than for peripheral neuropathy and 2000-fold greater than estimated dietary exposures(Reference Dybing and Sanner24, Reference Konings, Baars and van Klaveren76, 77). The NOAEL for reproductive toxicity was estimated to be 2–5 mg/kg BW per d depending on the endpoint of fertility or embryonic death(77). No reproductive toxicities have been reported in humans. Therefore, it is highly unlikely that any reproductive toxicity in humans would result from dietary exposure to acrylamide, although concerns about the cumulative effects of low-level chronic exposure are increasing.
Evidence of neurological effects has been observed following single oral doses of 126 mg/kg in rats and rabbits and 100 mg/kg in dogs(Reference McCollister, Oyen and Rowe78, Reference Kuperman79). Using chronic dosing schedules, it has been observed that cumulative oral doses of 500–600 mg/kg using daily doses of 25–50 mg/kg per d are required to produce ataxia in rats, dogs and baboons(Reference McCollister, Oyen and Rowe78, Reference Thomann, Koella and Krinke80, Reference Hopkins81). Smaller daily doses do not produce a clinical effect until a larger cumulative dose is attained. It has been found that the administration of acrylamide at daily doses of 6 to 9 mg/kg does not produce evidence of neurotoxicity in rats until a cumulative dose of 1200 to 1800 mg/kg is attained, and that doses of up to 3 mg/kg per d for 90 d administered to rats do not result in adverse effects(Reference McCollister, Oyen and Rowe78, Reference Fullerton and Barnes82). Spencer et al. (Reference Spencer, Sabri and Schaumburg83) reported that Rhesus monkeys fed up to 2 mg/kg per d did not show any adverse clinical effects at 325 d.
Acrylamide appears to be a multi-organ carcinogen in rodents. This is consistent with its distribution throughout the whole body. However, it is noteworthy that there are no corresponding target organs in mice and rats. Rat thyroid follicular cell tumours and mammary tumours from two studies are considered of possible relevance for human health. Modelling of these data has allowed the determination of benchmark doses and benchmark dose lower confidence limits(Reference Johnson, Gorzinski and Bodner84, Reference Friedman, Dulak and Stedham85). For a 10 % CI, the results were in the range of 300–1100 μg/kg per d for the mammary tumours and between 630 and 930 for the thyroid tumours using the Environmental Protection Agency's dose–response modelling for determination of a point of departure for a risk of 10 % lower exposure dose (LED10)(Reference Parzefall86, Reference Shipp, Lawrence and Gentry87). Relating the combined tumour data to Hb adduct data of acrylamide and glycidamide the doses 440 or 950 μg/kg per d were determined, respectively, which are close to the benchmark dose lower confidence limits(Reference Parzefall86–Reference Exon88).
The estimated average chronic human dietary intake was calculated crudely as 0·3–0·7 μg/kg BW per d in 2002. However, the Food and Drug Administration calculated the exact intake as 0·4 μg/kg BW per d in 2003 and this value remained the same when the Food and Drug Administration updated this subject in 2004(89). This increase of acrylamide intake by age seems to reflect the increased consumption of fast food together with potato crisps or French fries.
As the NOAEL for neuropathy is given as 0·5 mg/kg BW per d, a woman weighing 132 lb (60 kg) could safely consume 30 mg acrylamide daily; a man weighing 180 lb (82 kg) could consume about 41 mg(Reference Rosén and Hellenäs90). On the other hand, if the same calculation is applied to the daily intake of a child, a child weighing 20 lb (9 kg) can consume food including 4·5 mg acrylamide. Whether it is appropriate to judge the daily intake of a child with the same calculation is a matter of debate considering that the susceptibility of a child towards several chemicals is much higher than that of an adult. It is anticipated that children will generally have intakes that are two to three times those of adults when expressed on a BW basis.
Studies on acrylamide contamination in baby foods and infant formulas and estimated daily exposures of acrylamide are given in Table 3(Reference Hilbig, Freidank and Kersting26, Reference Alexy, Sichert-Hellert and Kersting91–94).
Table 3 The daily intake of acrylamide of children in several European countries

BW, body weight; SCF, Scientific Committee on Food.
Determination of acrylamide and limits in foods
Potentially toxic acrylamide is largely derived from heat-induced reactions between the amino group of the free amino acid asparagine and carbonyl groups of glucose and fructose in cereals, potatoes, and other plant-derived foods(Reference Friedman and Levin95). Several methods have been described that determine acrylamide in several foodstuffs and water. The first reported data on acrylamide in foods available on the market were developed by the Swedish National Food Administration using liquid chromatography/tandem mass spectroscopy (LC/MS/MS)(96). Since then, several analytical procedures have been developed, including LC/MS/MS and GC/MS with different sensitivity, cost, speed and applicability(Reference Rosén and Hellenäs90).
There are a limited number of studies concerning the daily intake of acrylamide from foodstuffs. In the year 2002, the Swedish National Food Agency showed that certain fried, baked and deep-fried foods and coffee had high concentrations of acrylamide(96). In a Norwegian study, acrylamide exposure from foods and coffee was estimated to be 0·49 and 0·46 μg/kg BW in males and females, respectively(97). The New Zealand National Nutrition Survey showed that with a typical Western diet, including hot chips (French fries) and potato crisps, the calculated daily intake of acrylamide is 0·3 μg/kg BW, which is below the NOAEL(98).
On the other hand, as babies and children are a more sensitive population than adults, their consumption of foods including acrylamide must be limited. The studies calculating the daily intake of acrylamide of children in several European countries are summarised in Table 4(Reference Fohgelberg, Rosén and Hellenäs11, Reference Rosén and Hellenäs90, Reference Jiao, Zhang and Ren99–103).
Table 4 Studies on acrylamide contamination in baby foods and infant formulas and estimated daily exposures of acrylamide

LC/MS/MS, liquid chromatography/tandem mass spectroscopy.
The European Union gives high importance to acrylamide contamination in food. After the Commission Recommendation 2007/331/EC, twenty-one member states of the European Union presented the results of acrylamide in several foodstuffs (presented under the titles of ‘French fries’, ‘potato crisps’, ‘potato products for home cooking’, ‘bread’, ‘breakfast cereals’, ‘biscuits’, ‘roasted coffee’, ‘jarred baby foods’, ‘processed cereal-based baby foods’ and ‘other products’) to the European Food Safety Authority in 2007. There were 2715 results reported for foods sampled in 2007, with a minimum of seventy-six reported for ‘processed cereal-based baby foods’ and a maximum of 854 reported for ‘other products’. The arithmetic mean acrylamide content ranged from 44 μg/kg for ‘jarred baby foods’ to 628 μg/kg for ‘potato crisps’, with the equivalent geometric means of 31 and 366 μg/kg. The 2007 results were compared with results collected by the European Commission Joint Research Centre's Institute for Reference Materials and Measurements in the years 2003 to 2006. There were 9311 results reported for foods sampled in the 4 years; however, there were only eight results reported for the food category ‘jarred baby foods’ and they were not included in the comparison. The arithmetic mean of acrylamide content ranged from 55 μg/kg for ‘cereal-based baby foods’ to 678 μg/kg for ‘potato crisps’, with the equivalent geometric means of 35 and 514 μg/kg. The highest 95th percentile value was reported for ‘potato crisps’ at 1718 μg/kg and the highest maximum for ‘other products’ at 7834 μg/kg(102).
Conclusion
Infants and children are susceptible towards the effects of several xenobiotics. The knowledge of all factors that affect the formation of glycidamide is important with respect to estimating risk from this known carcinogen. It is known that the metabolism and clearance of drugs are higher in children because of the larger liver:BW ratio and the higher blood flow through the liver compared with those of older adults(Reference Greim and Snyder104). As the major metabolite of acrylamide, glycidamide, is formed through biotransformation, it is likely that glycidamide can be formed at an higher rate than adults in children. In addition, the detoxification pathway through conjugation with GSH may be less functional due to low levels of GSH in a child's liver. Therefore, it is possible that the toxicity of acrylamide might be higher in children.
Children and adolescents should eat a balanced and assorted diet, which includes plenty of fruit and vegetables but also cereals. Also, parents should moderate their children's consumption of fried and fatty foods. Such a diet would also reduce the risk of high exposures to acrylamide, since acrylamide has not been determined in unheated or boiled foods(Reference Tareke, Rydberg and Karlsson105). Acrylamide presumably will also be present in low levels in a nutritionally balanced diet; however, it is in the power of parents to feed their children with more healthy foods. On the other hand, for small children who are merely fed with infant formula and baby food, it is obligatory for governments to take serious measures to monitor contaminants(89).
Taking into account all the knowledge given above, baby food and infant formulas should be routinely tested before use for the detection of acrylamide using improved techniques. Strict law enforcement should be undertaken regarding the detection of all the contaminants in infant formulas and baby foods. Furthermore, producers should be warned by regulatory authorities regarding good manufacturing practices to decrease the levels of contaminants, particularly acrylamide.
Acknowledgements
The present review was not supported by any funding.
P. E. and T. B. are working as faculty staff in the Department of Toxicology, Hacettepe University Faculty of Pharmacy and prepared this review together with equal contribution to the text.
There are no conflicts of interest.