Introduction
Dietary fat intake is one of the major modifiable risk factors implicated in the causation of CHD. The influence of dietary fatty acid chain length, degree of unsaturation and geometry of double bonds (cis and trans) on the atherosclerotic and thrombotic pathways that lead to CHD are well documented(Reference Lefevre, Kris-Etherton and Zhao1, Reference Sacks and Katan2). However, until recently, little attention has been given to the position of fatty acids in the TAG molecule. In vegetable fats and oils, SFA are found predominantly in the outer positions and unsaturated fatty acids in the middle position of the TAG, whilst in animal fats, the middle position of the TAG contains a high proportion of SFA. It is plausible that differences in the positional composition between animal and plant fats may account for differences in their effects on plasma lipids and CHD risk.
The impact of the positional composition of TAG on human nutrition is of increasing relevance with the growing commercial use of the process of interesterification, which changes the specific melting characteristics of a fat without the generation of trans-fatty acids by redistributing the position of fatty acids within the TAG. Commonly used interesterified fats, which provide suitable functionality for the food industry, include fats that are rich in the long-chain SFA, palmitic acid (16 : 0) and stearic acid (18 : 0), but few studies have investigated the acute and chronic health effects of these fats in human adults. The effect of TAG positional composition on the biochemical and physical characteristics of the fat may influence their absorption from the gut, metabolism in enterocytes, subsequent chylomicron metabolism and distribution into tissues. These may have subsequent implications for the risk of CHD via effects on energy balance, insulin sensitivity, fasting and postprandial lipids.
The major SFA in the Western diet are palmitic and stearic acids, which are present in both plant and animal products. In the UK approximately 17·6 g palmitic acid and 8·5 g stearic acid are consumed daily, accounting for 50 and 24 % of SFA intake respectively. It is generally believed that palmitic acid accounts for most of the cholesterol-raising effect of diets rich in SFA(Reference Grande, Anderson and Keys3, Reference Grundy and Denke4). Palmitic acid raises total and LDL-cholesterol concentrations compared with PUFA, MUFA and carbohydrates(Reference Grande, Anderson and Keys3, Reference Mattson and Grundy5). However, palm oil (about 44 % palmitic acid) does not raise cholesterol concentrations to the degree predicted by the equations of Mensink(Reference Mensink6) based on its SFA content (about 52 %). It is hypothesised that these differences may be explained by the position of palmitic acid in palm oil TAG ( < 10 % of total palmitic acid in the middle position). This may also be true for stearic acid-rich fats and may explain why the early equations of Hegsted et al. (Reference Hegsted, McGandy and Myers7), utilising cocoa butter (rich in stearic and palmitic acids in the outer position of the TAG(Reference Bracco8)), demonstrated that stearic acid had a neutral effect on serum cholesterol concentrations(Reference Denke9, Reference Kritchevsky10). However, there have been few studies in the human adult to investigate the chronic effects of differences in TAG structure and interesterification on fasting lipid levels or energy balance. The postprandial effects of fats with differing TAG structure are better characterised but there is no consensus between studies. The aim of the present review is to summarise the effects of TAG structure and interesterification on the physical properties of fats and subsequent effects on metabolism, and to review the literature relating to TAG structure and CHD risk factors (fasting and postprandial) in adult humans.
TAG structure
TAG consists of a glycerol moiety and three fatty acids esterified to the carbon-hydroxyl groups of the glycerol (Fig. 1). Each TAG may contain a mixture of different fatty acids or may contain three of the same fatty acids. These fatty acids are esterified to three distinct positions on the glycerol which is described by a stereospecific numbering system (sn); sn-1, sn-2 and sn-3. The sn refers to the position of the carbon atoms on the glycerol; the carbon atom that is at the top of the Fischer projection that has a vertical carbon chain with the hydroxyl group at carbon-2 orientated to the left is referred to as sn-1 (outer position). sn-2 Refers to the position at carbon-2 (middle position) and sn-3 (outer position) refers to the position below sn-2. The term molecular species describes the specific make-up of the TAG. For example, a TAG with palmitic acid at sn-1, oleic acid (18 : 1n-9) at sn-2 and stearic acid at sn-3 is referred to as the molecular species 1-palmitoyl 2-oleoyl 3-stearoylglcerol or in short-hand POS (where P is palmitic acid, O is oleic acid and S is stearic acid). A TAG mixture with just five different fatty acids can therefore exist as 105 different TAG molecular species (TAG-MS) according to differences in positional composition. For example, TAG containing just two fatty acids, palmitic acid (P) and oleic acid (O), can exist as eight different TAG-MS (POO, POP, POO, OOP, OPO, PPO, OPP, PPP, OOO). However, TAG are synthesised in nature by enzyme systems (acyl tranferases) which have specificity for different positions; therefore in plants and animals specific fatty acids occupy specific positions on the TAG molecule depending upon the species. The positional composition of some common vegetable and animal fats rich in long-chain SFA are shown in Table 1. In vegetable fats and oils, SFA are found predominantly in the external sn-1 and sn-3 positions and unsaturated fatty acids in the sn-2 position of the TAG. Conversely, in animal fats, the sn-2 position of the TAG contains a high proportion of SFA.

Fig. 1 Structure of TAG molecule. sn, Stereospecific numbering position; R, fatty acid.
Table 1 Positional distribution of fatty acids (mol %) in TAG of some common fats and oils (adapted from Berry & Sanders(Reference Berry and Sanders97))

sn, Stereospecific numbering position. P, palmitic acid; O, oleic acid; S, stearic acid; L, linoleic acid; B, butyric acid; C, capric acid.
* Major molecular species are in parentheses.
Interesterified fats and their physical characteristics
The role of stereospecific location of dietary fats can be studied using the process of interesterification. Interesterification is the shuffling of fatty acids moieties within (intra-esterification) and among (interesterification) TAG molecules until a thermodynamic equilibrium is reached. There are two types of interesterification: chemical interesterification (which can be random or directed) and enzyme directed interesterification(Reference Marangoni and Rousseau11). In the process of random chemical interesterification the fat is heated (at about 100–140°C) for a short period of time, in the presence of a catalyst (usually sodium methoxide; about 0·05–0·15 %). The fatty acids are hydrolysed from the glycerol moiety and rearrange in a random manner, approximately equally on each position (sn-1, sn-2, sn-3), such that one-third of each fatty acid is on the sn-1 position, one-third on the sn-2 position, and one-third on the sn-3 position of the TAG. Three types of reactions can be used: acidolysis (fatty acid with TAG), glycerolysis (glycerol with TAG) and transesterification (TAG with TAG). Random interesterification of palm oil (primary TAG-MS; POP, POO and POL (where L is linoleic acid, 18 : 2n-6)) would therefore produce a whole variety of TAG-MS (such as PPO, OPO, PPL, PPP, LPO, OLL, etc) and increase the proportion of palmitic acid of the total palmitic acid in the sn-2 position from about 10 % to about 33 %. In contrast, enzyme-directed interesterification is used to create TAG with specific positional composition using enzymes (derived from bacterial, yeast and fungal sources, such as Candida rugosa and Mucor miehei) with regiospecificity to direct the fatty acid to a specific position within the TAG.
Interesterification allows the comparison of the nutritional effects of diets with identical fatty acid composition but different positional composition and is also used by the food industry to alter the melting and crystallisation characteristics of fats to attain certain functionality, such as spreadability, a particular melting point or solid fat content–temperature profile. Interesterification influences the physical properties of fats due to differences in the individual melting and crystalline properties of TAG-MS (Table 2). As well as achieving suitable melting properties, interesterification also optimises crystallisation behaviour to generate more stable crystalline forms. SFA-rich TAG can exist in more than one crystalline form (polymorphs), which results in different patterns of molecular packing in the crystals and multiple melting points. The three basic polymorphs are referred to as α, β′ and β. The α form is the least stable with the lowest melting point, and β the most stable with the highest melting point. For example, the melting point of the α, β′ and β polymorphic forms of the TAG-MS SOS are 22·4, 36·5 and 41·6°C respectively(Reference Lavery12). During interesterification, the β′ polymorphic form is commonly generated, thus improving the stability and granularity of the fat.
Table 2 Melting temperatures of some TAG molecular species of different polymorphic forms (adapted from Small(Reference Small44), Anonymous(98), Wesdorp(Reference Wesdorp99) and Berry & Sanders(Reference Berry and Sanders97))

S, stearic acid; P, palmitic acid; O, oleic acid; NA, not available; L, linoleic acid.
The random chemical interesterification of cocoa butter illustrates the extent to which interesterification can alter the physical properties of a fat, as outlined in Table 3. Random chemical interesterification of cocoa butter (primary TAG-MS: SOS and POS) generates a fat with a variety of mono-saturated (OSO, SOO), di-saturated (PSO, OSS) and tri-saturated (PSS, SPS) TAG-MS, each with different melting properties. The overall effect is the generation of a hard fat from a soft fat, which is illustrated by the increased proportion of solid fat content at different temperatures (measured by pulsed NMR analysis). For example, the proportion of solid fat at body temperature (37°C) in the un-interesterified (referred to as native fats for the purpose of the present review) cocoa butter and randomly interesterified cocoa butter is 1 and 37 % respectively. The differences in the melting profiles of the native v. randomly interesterified cocoa butter fats are also illustrated using differential scanning calorimetry (Fig. 2), which shows a major melting peak at 36–37°C for the native cocoa butter (hence why cocoa butter and chocolate have the ‘melt in the mouth’ feel) and a broad melting peak between 30 and 55°C for the randomly interesterified cocoa butter.
Table 3 TAG structure and physical characteristics of native cocoa butter and interesterified cocoa butter(Reference Sanders, Berry and Miller90)

sn, Stereospecific numbering position; P, palmitic acid; O, oleic acid; S, stearic acid.
* Melting temperature of β polymorphism given in parentheses.
† Measured with slow method.

Fig. 2 Differential scanning calorimetry plot of native cocoa butter (a) and interesterified cocoa butter (b)(Reference Sanders, Berry and Miller90). Adapted from Berry & Sanders(Reference Berry and Sanders97).
Commercially, interesterification has been used by the food industry as an alternative to hydrogenation to alter the physical characteristics of fats. Interesterified fats for commercial use are usually generated from the random interesterification of a liquid oil with a fully hydrogenated (tri-saturated) fat, or blends of palm-stearin fractions with lauric (12 : 0) acid-rich fats such as coconut oil or palm kernel stearin. Therefore most commercially available interesterified fats, which have the functionality desired by the food industry, will consist predominantly of lauric, palmitic, stearic, oleic and linoleic acids. Chemical random interesterification was first used commercially in the 1930s to modify the spreadability and baking properties of lard, by reducing the solid fat content at 20°C and changing the crystalline polymorphic forms. Unilever Ltd was one of the first companies to use enzyme-directed interesterification, in 1980, for the production of cheaper confectionery fat substitutes for use in place of cocoa butter, using lipase-catalysed interesterification of POP (from palm oil mid-fraction) with stearic acid, by 1,3-specific lipase to result in the synthesis of POS and SOS, the main TAG-MS of cocoa butter. Since then, interesterified fats have become more widely used in the manufacture of shortenings, margarines and spreads to improve their textural properties, modify their melting behaviour and improve their stability. Interesterification is also used to produce fats with specific properties for use in parenteral, enteral and infant feeding. For example, Salatrim™ is an interesterified mixture of stearic, acetic, propionic and butyric acid and is used as a lower-energy fat(Reference Finley, Klemann and Leveille13) due to the presence of medium-chain TAG (33·5 kJ/g; 8 kcal/g) and the position of the stearic acid in the outer positions (sn-1 and sn-3) of the TAG molecule. In contrast, Betapol™ (Lipid Nutrition B. V., Wormerveer, the Netherlands), produced by position-specific enzyme-directed interesterification, is used in infant feeding to mimic human breast milk fat composition (45 % palmitic acid in the sn-2 position) and facilitate a greater fat absorption (discussed in detail later).
Due to the increasing awareness of the negative health effects of partially hydrogenated fats(Reference Mozaffarian, Katan and Ascherio14) and the removal of trans-fatty acids from food products, alternative processes to produce high-melting-point TAG are of increasing relevance. The process of interesterification, which preserves the level of unsaturated fatty acids and negates the need for higher SFA-rich fractionated fats, is an ideal substitute for partially hydrogenated fats. Therefore its health effects are of increasing relevance to human nutrition.
Overview of influence of TAG structure on dietary fat metabolism
In addition to chain length and degree of saturation of fatty acids, it is now recognised that the positional composition of TAG may be an important determinant of digestibility and metabolism. This is because pancreatic lipase(Reference Yang and Kuksis15) and lipoprotein lipase(Reference Nilsson-Ehle, Egelrud and Belfrage16) preferentially hydrolyse the fatty acids in the sn-1 and sn-3 positions of TAG, leaving the fatty acid in the sn-2 position as a 2-monoacylglycerol (MAG). The positional specificity of pancreatic lipase may be advantageous for the absorption of SFA located in the sn-2 position due to the better absorption of SFA as a 2-MAG (due to its polar (glycerol) and non-polar (fatty acid) moieties) rather than as NEFA. Furthermore, long-chain SFA present in the intestine as NEFA (hydrolysed from the sn-1 and sn-3 position of TAG) have high individual melting points (stearic acid, 69°C; palmitic acid, 64°C) and tend to form insoluble soaps with Ca and Mg in the gut(Reference Carnielli, Luijendijk and van Goudoever17). It is therefore plausible that long-chain SFA are better absorbed if situated in the sn-2 position than if in the sn-1 and sn-3 positions, as shown in rat studies(Reference Mattson, Nolen and Webb18, Reference Tomarelli, Meyer and Weaber19) and human infants(Reference Carnielli, Luijendijk and van Beek20, Reference Filer, Mattson and Fomon21).
MAG provide the basic structure for the resynthesis of chylomicron-TAG in the enterocyte; therefore upon absorption the fatty acids in position sn-2 of the dietary TAG are retained(Reference Berry, Miller and Sanders22, Reference Berry, Woodward and Yeoh23) and may influence subsequent metabolism of chylomicrons and chylomicron remnants(Reference Yang and Kuksis15, Reference Innis, Dyer and Nelson24), which are formed following chylomicron-TAG hydrolysis. Indeed, palmitic and stearic acids in the sn-2 position of chylomicron-TAG have been shown to slow down TAG lipolysis(Reference Mortimer, Simmonds and Joll25, Reference Redgrave, Kodali and Small26) and the clearance of chylomicrons and chylomicron remnants in rat studies compared with when they are in the sn-1 or sn-3 positions(Reference Mortimer, Simmonds and Joll25–Reference Mortimer, Simmonds and Cockman29).
This evidence would suggest that eating dietary fats containing a predominance of sn-2 saturated TAG might be more completely digested, absorbed more rapidly and cleared from the circulation more slowly than TAG containing SFA in the sn-1 and sn-3 positions. Furthermore, fatty acids attached to the sn-2 position may be preferentially transported to the liver rather than adipose tissue due to the positional specificity of lipoprotein lipase for the sn-1 and sn-3 positions of TAG. Therefore, because the hepatocyte is the major site of action of fatty acids on LDL metabolism, SFA in the sn-2 position of dietary TAG may elevate LDL concentrations more than the same fatty acid in the sn-1 or sn-3 positions. In addition, a delayed chylomicron remnant clearance may influence atherosclerosis due to their potent atherogenic properties(Reference Botham30). Therefore differences in digestion and metabolism may have implications for energy balance, fasting lipid concentrations, postprandial lipaemia and subsequent CVD risk.
Triacylglycerol structure and digestibility
Studies investigating the effect of the positional composition of stearic and palmitic acid-rich TAG on digestibility suggest that the position of the fatty acid within the TAG may be a major determinant of its digestibility. An early study in rats by Hoagland & Snider(Reference Hoagland and Snider31) reported that the digestibility of cocoa butter (rich in stearic and palmitic acids in the sn-1 and sn-3 positions) was much lower than that of maize oil, with digestibility values of 63–82 and 97–98 % respectively. Similar findings have since been reported by others(Reference Apgar, Shively and Tarka32), and are attributed primarily to a reduction in stearic and palmitic acid absorption(Reference Apgar, Shively and Tarka32–Reference Bonanome and Grundy34) from the sn-1 or sn-3 position.
Mattson et al. (Reference Mattson, Nolen and Webb18) observed that stearic acid absorption was decreased in rats fed the TAG-MS SOS compared with SSO. Following diets rich in the TAG-MS SOO, OSO, SOS, SSO and OOO, absorption of stearic acid was 98 and 99 % for OSO, 55 and 96 % for SOO, 37 and 70 % for SOS and 59 and 60 % for OSS in the presence and absence of Ca and Mg respectively(Reference Mattson, Nolen and Webb18). In a subsequent study(Reference Brink, Haddeman and de Fouw35), rats were fed diets containing a fat blend high in SOS or OSS given at low (0·3 g/100 g) or high (1·0 g/100 g) Ca concentrations. Total fat absorption was lower in rats fed SOS (93 and 73 %; low and high Ca respectively) compared with OSS (97 and 89 %; low and high Ca respectively), and the absorption of stearic acid was also lower at low and high Ca concentrations after SOS (stearic acid 85 and 46 % respectively) compared with SSO (stearic acid 93 and 80 % respectively). Both these studies are in agreement with the hypothesis that long-chain SFA are better absorbed when esterified to the sn-2 position and demonstrate that stearic acid esterified to the sn-1 or sn-3 position is released as non-esterified stearic acid and in the presence of Ca and Mg is poorly absorbed.
Similar results have been observed for palmitic acid. Studies in animals(Reference Tomarelli, Meyer and Weaber19, Reference Aoe, Yamamura and Matsuyama36, Reference Smink, Gerrits and Hovenier37) and human infants(Reference Carnielli, Luijendijk and van Beek20, Reference Filer, Mattson and Fomon21, Reference Lucas, Quinlan and Abrams38) have observed a greater fat absorption when palmitic acid was present in the sn-2 position (as in human breast milk, native lard, enzyme-directed and randomly chemically interesterified plant fats) compared with the sn-1 and sn-3 positions (bovine milk, randomly chemically interesterified lard or native palm oil). It was found that the relative absorption of palmitic acid(Reference Tomarelli, Meyer and Weaber19) and total fat(Reference Carnielli, Luijendijk and van Goudoever39) was linearly related to the proportion of palmitic acid in the sn-2 position of the TAG in human infants. Studies in rats comparing the effects of feeding native palm oil v. randomly interesterified palm oil observed a reduced palmitic acid and total fat excretion(Reference Smink, Gerrits and Hovenier37, Reference Lien, Yuhas and Boyle40, Reference Renaud, Ruf and Petithory41) and greater proportion of palmitic acid in adipose tissue(Reference Smink, Gerrits and Hovenier37) following the randomly interesterified palm oil compared with the native palm oil. This enhanced absorption of palmitic acid in the sn-2 position of TAG has been utilised by food manufacturers who have manufactured a palmitic acid-rich fat with a high proportion of palmitic acid esterified to the sn-2 position of the TAG to produce a ‘vegetable equivalent to human breast milk fat’ called Betapol™; human milk fat contains approximately 65 % of its palmitic acid in the sn-2 position, whereas bovine milk has approximately 32 % palmitic acid in the sn-2 position(Reference Jensen42). The effect of TAG structure on digestibility in animals and human infants is reviewed in more detail elsewhere(Reference Kritchevsky43–Reference Decker45).
Although animal and human infant data indicate that differences in TAG structure influence long-chain SFA absorption, TAG structure does not appear to influence absorption and digestion in human adults. Recent studies suggest that the human adult can absorb efficiently most dietary fatty acids whether as NEFA or as 2-MAG. Results from infant studies must be interpreted with caution as pancreatic lipase activity is low in newborn infants, which would affect fat digestibility compared with that in human adults. Furthermore, many of the animal studies have a high Ca:fat ratio in the diets and data in human subjects would suggest that at physiological levels, Ca does not affect long-chain SFA absorption(Reference Finley, Klemann and Leveille13).
In contrast to the animal studies, most human studies have reported cocoa butter to be well absorbed(Reference Mitchell, McMahon and Shively33, Reference Bonanome and Grundy34, Reference Bonanome and Grundy46, Reference Denke and Grundy47). Shahkhalili et al. (Reference Shahkhalili, Duruz and Acheson48) reported that following cocoa butter or maize oil (31 g/d) fed to male subjects for 2 weeks, there was no difference in the faecal fat excretion (mean 4·55 g/d) and digestibility relative to maize oil (99 %), although the proportion of stearic acid in the faeces during the cocoa butter period (1·9 g/d) was higher than during the maize oil period (41 v. 27 % respectively), but was compensated for by a decrease in the proportion of other faecal fatty acids(Reference Shahkhalili, Duruz and Acheson48). Denke & Grundy(Reference Denke and Grundy47) observed that following diets rich in beef tallow, native cocoa butter, butter fat and olive oil (23·6, 40·9, 12·4 and 3·7 g stearic acid/d respectively) using liquid formulated diets containing 40 % of energy from the test fat in ten male subjects for 3 weeks, the level of stearic acid excreted from beef tallow, native cocoa butter, butter fat and olive oil was within the usual range (1·4, 3·1, 1·2 and 1·2 g/d respectively), despite differences in the position of stearic acid in the dietary TAG. Our group also recently reported stearic acid to be well absorbed whether in the sn-1, sn-3 position or the sn-2 position(Reference Berry, Miller and Sanders22). In a cross-over design study, sixteen healthy male subjects consumed 30 g fat/d as native shea butter (stearic acid in the sn-1 and sn-3 positions) or randomly chemically interesterified shea butter for 3 weeks. At the end of each 3-week feeding period, 3 d faecal collections were made and total fat (excretion 3·5–3·9 g/d) and stearic acid (excretion 1·9–2·1 g/d) were found to be well absorbed from both fats with no differences in digestibility between fats. Others have also reported stearic acid to be well digested whether distributed in the sn-1 and sn-3 positions of the dietary TAG(Reference Dougherty, Allman and Iacono49) or as a randomly interesterified stearic acid-rich fat(Reference Bonanome and Grundy46, Reference Baer, Judd and Kris-Etherton50–Reference Tuomasjukka, Viitanen and Kallio52). Human studies have also shown that the digestibility of palmitic acid is high in human subjects when in the outer positions(Reference Denke and Grundy47, Reference Shahkhalili, Duruz and Acheson48), or randomly distributed on all three positions of the glycerol(Reference Snook, Park and Williams51).
The above studies demonstrate that in human adults, stearic and palmitic acid are well digested independently of their position in the TAG. However, few studies have made head-to-head comparisons of fats with identical fatty acid composition, which differ only with respect to their positional composition. Some of the chronic studies listed in Table 4 investigating the chronic effects of TAG structure on fasting plasma lipids measured body weight but not digestibility. A study comparing a fat containing palmitic acid in the sn-2 position (Betapol™) v. the sn-1 and sn-3 position (native palm oil) reported a higher body weight following 3 weeks of the Betapol™ intervention compared with the native palm oil (by 0·1 (SD 0·8) kg); however, CI were not given and statistical results were not reported, so the significance of this very small difference is unclear(Reference Zock, de Vries and de Fouw53). Other studies comparing native palm oil v. interesterified palm oil(Reference Nestel, Noakes and Belling54), native shea butter v. interesterified shea butter(Reference Berry, Miller and Sanders22) and a high stearic and palmitic acid-rich fat blend v. its interesterified counterpart(Reference Meijer and Weststrate55) for 3-week periods reported no change in body weight.
Table 4 Chronic intervention studies in human subjects on effects of TAG structure of stearic and palmitic acid-rich fats on blood lipids

P, palmitic acid; sn, stereospecific numbering position; O, oleic acid; L, linoleic acid; E, energy; S, stearic acid; EL, elaidic acid; My, myristic acid; HDL-C, HDL-cholesterol.
* Lipid Nutrition B. V., Wormerveer, the Netherlands.
In summary, the positional composition of stearic and palmitic acid-rich TAG influences digestibility in animals and human infants but there is little evidence to suggest it affects digestibility or energy balance in adult humans. Further chronic feeding studies are needed to compare interesterified fats with their native counterpart for longer intervention periods. When considering energy balance any acute effects resulting from differences in the rates of absorption on satiety signals and gut hormones should also be considered.
Fasting lipids and atherogenicity
The effects of TAG structure on the metabolism of long-chain SFA-rich fats may have subsequent effects on atherogenicity and fasting plasma lipids. This was first brought to light by McGandy et al. (Reference McGandy, Hegsted and Myers56) who reported that stearic acid was hypercholesterolaemic when fed as an interesterified fat in contrast to findings by Hegsted et al. (Reference Hegsted, McGandy and Myers7) who reported that stearic acid had a neutral effect on cholesterol concentrations when provided as native cocoa butter (stearic acid in the sn-1 and sn-3 positions). It was hypothesised that these differences were due to differences in the TAG structure of the fats fed, and that stearic acid in the sn-2 position was hypercholesterolaemic but when in the sn-1 or sn-3 positions had a neutral effect on cholesterol. This may also be the case for palmitic acid; native palm oil (about 44 % palmitic acid, mostly in the sn-1 and sn-3 positions) does not raise cholesterol concentrations to the degree predicted by the equations of Mensink(Reference Mensink6) based on its SFA content (about 52 %)(Reference Mensink6). A plausible mechanism to support this hypothesis is that fatty acids in the sn-2 position may be preferentially transported to the liver, which is the major site of action of fatty acids on LDL metabolism. Therefore changing the structure of fats, such that SFA are in the sn-2 position, may increase the delivery of SFA to the liver, which would up-regulate cholesterol production. Furthermore, sn-2 MAG delivered to the liver from remnant chylomicrons may serve as the backbone for gut or liver phospholipids(Reference Jung, Turner and Neese57) exerting downstream effects which may affect plasma lipid levels.
Atherogenicity
Animal studies have shown that palmitic and stearic acids in the sn-2 position of the TAG may render that TAG more atherogenic than when in the sn-1 or sn-3 positions. Kritchevsky et al. (Reference Kritchevsky, Tepper and Chen58) undertook a series of experiments to investigate the atherogenicity of fats with different positional composition in rabbits(Reference Kritchevsky, Tepper and Chen58). The fats tested contained the TAG species SOS, SSO, POP and PPO. Atherosclerosis was similar following SOS and SSO, but more severe following PPO compared with POP, suggesting that the positional composition of palmitic acid-rich TAG, but not stearic acid-rich TAG, determines their effects on atherogenicity. Kritchevsky et al. (Reference Kritchevsky, Tepper and Kuksis59) also observed that randomly chemically interesterified palm oil was more atherogenic in rabbits compared with native palm oil. Furthermore, lard, which contains virtually all its palmitic acid in the sn-2 position, was found to be more atherogenic in rabbits than beef tallow which only has a small proportion of palmitic acid the sn-2 position(Reference Kritchevsky, Tepper and Bises60), and randomisation of lard rendered it less atherogenic(Reference Kritchevsky and Tepper61). It is noteworthy that in these studies the atherogenic potential of the fats was not related to changes in plasma lipids. Indeed, van Jaarsveld et al. (Reference van Jaarsveld, Smuts and Benadé62) observed that native palm oil reduced atherogenesis compared with lard despite having similar effects on cholesterolaemia in monkeys(Reference van Jaarsveld, Smuts and Benadé62). It is plausible that the fats containing palmitic acid in the sn-2 position, which may be removed from the circulation more slowly, would result in increased exposure of the endothelium to SFA and/or chylomicron remnants. Indeed, chylomicron remnants are toxic to the endothelium(Reference Botham30) and the endothelium has been shown to be impaired following a diet rich in SFA v. MUFA or a high-carbohydrate diet(Reference Keogh, Grieger and Noakes63, Reference Sarabi, Vessby and Millgard64). It is likely that the mechanisms behind this are related to effects on the release on inflammatory and haemostatic mediators rather than fasting lipids.
These findings support the theory that palmitic acid esterified to the sn-2 position is more atherogenic than when esterified to the sn-1 and sn-3 positions. However, there are no studies to date in adult human subjects looking at end-point measures of CVD following TAG of different structure, and there are only a small number of studies investigating effects on fasting plasma lipids, outlined below.
Fasting lipids
Few studies in adult human subjects have made direct comparisons between native and interesterified fats of similar fatty acid composition and animal studies are inconclusive. Studies in rats and rabbits have reported no significant effects of the positional distribution of palmitic acid on plasma cholesterol concentrations(Reference Renaud, Ruf and Petithory41, Reference Kritchevsky, Tepper and Bises60, Reference de Fouw, Kivits and Quinlan65), whilst in piglets a higher plasma total cholesterol concentration has been observed with higher proportions of palmitic acid in the sn-2 position(Reference Innis, Quinlan and Diersen-Schade66). In contrast, Reena & Lokesh(Reference Reena and Lokesh67) reported a cholesterol-lowering effect (lower total cholesterol, LDL-cholesterol and TAG) following interesterified fats (with more long-chain SFA in the sn-2 position) compared with their native counterparts in rats, despite a lack of difference in food intake or growth(Reference Reena and Lokesh67). A study in human infants fed formula for 4 months containing 25–27 % of palmitic acid, with about 30 % (synthesised TAG), about 57 % (breast milk) or about 5 % (standard formula) of the palmitic acid at the sn-2 position, observed no differences in weight or growth following the different diets(Reference Nelson and Innis68). However, infants fed the standard formula and synthesised formula had lower plasma total cholesterol concentrations compared with those fed breast milk, whilst those fed the synthesised formula had a lower HDL-cholesterol concentration compared with those fed breast milk or standard formula. These results suggest that in infants, a higher proportion of palmitic acid in the sn-2 position may be hypercholesterolaemic, but the effect of positional composition on HDL-cholesterol is unclear.
Although only a small number of studies in human adults have directly investigated the effects of TAG structure on plasma lipids, some conclusions can be drawn from studies that have compared interesterified fats or fats with SFA in distinct positions (sn-1 and sn-3 or sn-2) with habitual diets. Human studies investigating the effects of native cocoa butter(Reference Kris-Etherton, Derr and Mitchell69, Reference Connor, Witiak and Stone70), native shea butter(Reference Dougherty, Allman and Iacono49, Reference Tholstrup, Marckmann and Jespersen71) and beef tallow(Reference Denke and Grundy47), all of which have their stearic and/or palmitic acid predominantly in the sn-1 and sn-3 positions, have demonstrated a hypocholesterolaemic effect of these fats compared with habitual diets. Additionally, a study in 120 men fed native palm oil v. lard (24 % energy from test fat) for 6 weeks, reported lower total and LDL-cholesterol concentrations following the palm oil-rich diet compared with the lard diet(Reference Zhang, Ping and Chunrong72). It was proposed that the neutral effect observed was due to the positional composition of these fats, with the SFA eliciting a neutral effect when distributed in the sn-1 and sn-3 positions. However, studies utilising lard must be interpreted with caution due to its cholesterol content. Furthermore, Grande et al. (Reference Grande, Anderson and Keys3) found that native cocoa butter and an artificial interesterified TAG mixture with the same fatty acid composition as native cocoa butter produced similar serum cholesterol concentrations(Reference Grande, Anderson and Keys3), suggesting no effect of positional composition on lipids in humans. Others have reported that randomly interesterified fats rich in stearic acid lower cholesterol concentrations (mainly from LDL) compared with baseline diets(Reference Bonanome and Grundy46, Reference Snook, Park and Williams51, Reference Aro, Jauhiainen and Partanen73, Reference Nestel, Pomeroy and Kay74) in human subjects. Forsythe et al. (Reference Forsythe, French and Goh75) recently reported a lower total cholesterol concentration (by 0·2 mmol/l; P = 0·02) following palmitic acid-rich diets (8 % energy from palmitic acid) with palmitic acid in the sn-2 position (65 % palmitic acid in the sn-2 position; lard) compared with palmitic acid in the sn-1 and sn-3 positions (23 % palmitic acid in the sn-2 position; palm-stearin), but no differences in HDL- and LDL-cholesterol or TAG concentrations(Reference Forsythe, French and Goh75). However, the study duration was short (2 weeks with 2-week washout periods), the subject numbers small (eight males), treatment order was not randomised and the diets delivered a different fatty acid composition (lower palmitic acid and higher stearic acid content in the sn-2 diet) and cholesterol content (higher cholesterol content in the sn-2 diet).
In a recent study designed to test the effects of an interesterified fat v. native fats, volunteers (n 30) were fed whole diets for 4-week periods containing 31 % energy from fat of which 70 % came from the test fats, which were based on palm olein, a trans-rich partially hydrogenated fat and an interesterified stearic acid-rich fat(Reference Sundram, Karupaiah and Hayes76). The partially hydrogenated fat and the interesterified fat increased the LDL:HDL ratio, largely due to lower plasma HDL-cholesterol concentrations following the interesterified and partially hydrogenated fat. An increase in fasting plasma glucose and decrease in plasma insulin concentrations were also observed following the interesterified-rich fat compared with the palm olein. Although this study triggered much negative media attention regarding the deleterious effects of interesterified fats, it is noteworthy that the interesterified fat did not elicit any changes in total cholesterol, LDL-cholesterol or TAG concentrations, and comparisons with baseline values were not shown. Furthermore, the fats that were being compared were not matched in SFA and PUFA composition.
Five studies have been identified that have made head-to-head comparisons of fats of similar fatty acid composition that differ only with regard to their positional composition. These are summarised in Table 4. Zock et al. (Reference Zock, de Vries and de Fouw53) compared diets (3 weeks) rich in palmitic acid (providing 28 % energy from the test fat and 11 % energy from palmitic acid) in the sn-2 position (Betapol™; 66·9 % palmitic acid in the sn-2 position) with a diet rich in palmitic acid in the sn-1 and sn-3 positions (native palm oil fraction; 6·4 % palmitic acid in the sn-2 position) in sixty-three free-living, healthy subjects. Diets consisted of similar fatty acid composition and differed only in respect to the amount of palmitic acid esterified to the sn-2 position. Following the diets there were no significant differences in plasma lipids (total, LDL- and HDL-cholesterol and TAG). However, when the male subjects were analysed separately, a small but significant increase in total cholesterol (mean increase 0·10 (95 % CI 0·02, 0·18) mmol/l) and LDL-cholesterol (mean increase 0·08 (95 % CI 0·00, 0·15) mmol/l) was observed following the Betapol™ diet compared with the native palm oil diet. Although small changes in LDL- and total cholesterol can have large effects on overall CHD risk, the changes reported in this study are unlikely to have any significant health implications due to the very large changes in fat intakes that were made in order to produce this effect. Nestel et al. (Reference Nestel, Noakes and Belling54) reported similar findings in hypercholesterolaemic subjects (n 27) following 3-week diets rich in native palm oil v. a randomly chemically interesterified palm oil blend (providing 20 % energy from test fats), which produced no difference in cholesterol concentrations. However, in this study a high-linoleic, moderate-trans blend was also used and elicited a significantly lower total cholesterol, LDL-cholesterol and HDL-cholesterol v. the native palm oil blend and interesterified palm oil blend.
To investigate the effect of more nutritionally relevant quantities of interesterified fats, Meijer & Weststrate(Reference Meijer and Weststrate55) fed healthy human subjects (n 60) two different fat blends, in a parallel-design study, in the form of margarine, at 4 % (about 10 g/d) and 8 % (about 20 g/d) energy levels for three consecutive weeks. Both fat blends contained 22 % palmitic acid, and differed only with regard to the proportion of palmitic acid in the sn-2 position (control blend; 18 % palmitic in the sn-2 position v. randomly chemically interesterified blend; 7 % palmitic in the sn-2 position). The increase in the proportion of palmitic acid in the sn-2 position on the 4 and 8 % energy blend was small (about 0·25 and 0·5 % energy), but a realistic level of intake if such fats were to be incorporated into spreads and other food products. The fat blends did not cause any significant changes in lipids or haemostatic parameters except for D-dimer concentrations which were significantly lower (about 10 %) following the interesterified fat blend. Although increased D-dimer concentrations are associated with increased risk of CHD, it is unlikely that this small reduction following the interesterified fat would result in any significant health impact.
Christophe et al. (Reference Christophe, De Greyt and Delanghe77) also investigated the effects of feeding realistic levels of native and interesterified fats in healthy human subjects by comparing butter as the control (palmitic acid predominantly in the sn-2 position) with interesterified butter (increase in stearic and oleic acids in the sn-2 position and decrease in palmitic, lauric and myrisitic acids in the sn-2 position). Thirty-two male subjects were fed the control butter (about 22 g out of about 130 g total fat per d) in a free-living setting (replacing cooking and spreading fat) for a 4-week period. Following this control period, volunteers were allocated to 4 further weeks on the control butter or interesterified butter, in a parallel design. Following 8 weeks on the control butter, HDL-cholesterol decreased by 7 %, whereas subjects consuming the interesterified butter for 4 weeks had lower TAG (about 14 %) and HDL-cholesterol (7 %) concentrations compared with baseline values. However, there were no significant differences between groups.
Utilising stearic acid-rich fats, we recently reported no effect of TAG structure on plasma lipids when fed at nutritionally relevant levels. Following 3-week diets rich in stearic acid (30 g test fat/d; 6 % energy from stearic acid) administered as native or randomly chemically interesterified shea butter (3 and 23 % stearic acid in the sn-2 position respectively) there were no differences between diets on fasting plasma lipids, glucose or insulin in healthy male subjects (n 16)(Reference Berry, Miller and Sanders22).
In summary, the evidence supports a lack of effect of TAG structure and interesterification of palmitic and stearic acid-rich fats on fasting plasma lipids in adult humans. However, the studies are of short duration (maximum 4 weeks) and have small subject numbers. Therefore, with the increasing utilisation of interesterified fats by the food industry, long-term chronic studies with large subject numbers are required using nutritionally relevant fats at realistic doses.
Postprandial lipaemia
Whilst there is a paucity of information regarding the chronic effects of interesterified fats, the influence of TAG structure is better characterised in postprandial studies. The effects of TAG structure on postprandial lipaemia are particularly relevant, as although most epidemiological investigations of plasma lipids as risk factors for CHD have relied on fasting values, in reality most human populations eat three to six times daily, and therefore spend most of their day in the postprandial state. The hypothesis that postprandial lipoproteins are involved in atherogenesis in humans was proposed by Zilversmit(Reference Zilversmit78). Since then, studies have shown that the magnitude and the duration of the postprandial response is relevant to CHD risk via effects on endothelial dysfunction(Reference Vogel, Corretti and Plotnick79), activation of factor VII(Reference Miller80), elevated circulating chylomicron remnants(Reference Gianturco and Bradley81), generation of small dense LDL particles(Reference Patsch, Miesenbock and Hopferwieser82) and impaired insulin sensitivity.
The postprandial lipaemic phase is highly dynamic and involves the complex interaction of lipoproteins (namely chylomicrons and chylomicron remnants) and apolipoproteins involved in the exogenous pathway of lipid metabolism, which transports and metabolises dietary fat(Reference Lopez-Miranda, Williams and Lairon83). During the later stages of the postprandial response, lipoproteins (namely VLDL, intermediate-density lipoprotein and LDL) from the endogenous pathway of lipid metabolism, which transports and metabolises fat synthesised in the liver, also come into play. Therefore the postprandial response will be determined by numerous physiological, genetic and dietary factors that influence gastric emptying, intestinal absorption, chylomicron synthesis, lipolysis and receptor-mediated removal. The fat content and composition of the meal is a major determinant of the response; fatty acid chain length, degree and geometry of unsaturation and the positional composition of TAG influence the level of postprandial lipaemia.
Human studies investigating the postprandial effects of TAG structure and interesterification are outlined in Table 5. Studies were selected for inclusion that had similar fatty acid composition (rich in palmitic and stearic acids) and differed only with regard to their positional composition. There is no consensus between the ten studies outlined regarding the effects of TAG structure on postprandial lipaemia. Comparisons of test meals rich in palmitic acid by Zampelas et al. (Reference Zampelas, Williams and Morgan84), Summers et al. (Reference Summers, Fielding and Ilic85), Yli-Jokipii et al. (Reference Yli-Jokipii, Schwab and Tahvonen86) and Berry et al. (Reference Berry, Woodward and Yeoh23), and test meals rich in stearic acid by Summers et al. (Reference Summers, Fielding and Herd87) and Berry et al. (Reference Berry, Miller and Sanders22) reported that positional distribution had little or no effect on the postprandial lipaemic response. In contrast, studies by Yli-Jokipii et al. (Reference Yli-Jokipii, Kallio and Schwab88), Berry & Sanders(Reference Berry and Sanders89), Sanders et al. (Reference Sanders, Berry and Miller90) and Robinson et al. (Reference Robinson, Martin and Robinson91) observed that the presence of stearic or palmitic acid at the sn-2 position of TAG produced a reduced postprandial lipaemia compared with the sn-1 or sn-3 position.
Table 5 Postprandial cross-over-design studies on effects of TAG structure of stearic and palmitic acid-rich fats in healthy adults
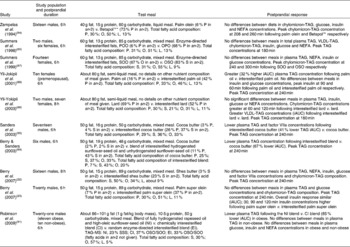
P, palmitic acid; sn, stereospecific numbering position; O, oleic acid; L, linoleic acid; S, stearic acid; iAUC, incremental area under the curve; TAG-MS, TAG molecular species.
* Lipid Nutrition B. V., Wormerveer, the Netherlands.
A plausible explanation for the differences reported between studies is the differences in the physical characteristics of the test fats used. The studies reporting a similar postprandial lipaemia following fats of different positional composition fed fats consisting of mono-saturated TAG-MS such as SOO v. OSO(Reference Summers, Fielding and Herd87) and POO v. OPO(Reference Zampelas, Williams and Morgan84, Reference Summers, Fielding and Ilic85) or fats with only a small proportion of di-saturated TAG-MS such as randomly chemically interesterified palm super olein(Reference Berry, Woodward and Yeoh23) and lard(Reference Yli-Jokipii, Schwab and Tahvonen86). The above fats would have broadly similar melting properties in their native and interesterified form and little or no solid fat content at body temperature. For example, the melting point of OPO and POO is 15 and 14·2°C respectively. In contrast the studies reporting differences in postprandial responses(Reference Yli-Jokipii, Kallio and Schwab88–Reference Robinson, Martin and Robinson91) contained a large proportion of di-saturated TAG-MS and tri-saturated TAG-MS, which would result in large differences in the physical characteristics between the native and interesterified fats and a higher proportion of solid fat content at 37°C in the interesterified v. native fats. For example, the melting points of POP, PPO, PPP, SOP, SPO and PSO are 30·5, 34·6, 55·7, 37·1, 40·2 and 41°C respectively. Furthermore, a study by our group comparing native shea butter v. randomly chemically interesterified shea butter reported no differences in postprandial lipaemia, despite differences in the proportions of stearic acid in the sn-2 position and the TAG-MS (SOS v. SSO), which is likely to be due to the large proportion of solid fat at 37°C in both test fats(Reference Berry, Miller and Sanders22). Indeed, when the native shea butter was compared with an oleic acid-rich fat, there was a significantly lower postprandial lipaemia following the native shea (by 53 %). This is in contrast to our study comparing native cocoa butter (TAG-MS: POS) with an oleic acid-rich oil(Reference Sanders, Oakley and Cooper92), which reported no differences in lipaemia. The solid fat content at 37°C in native cocoa butter and native shea butter is 0 and 22 % respectively, supporting the hypothesis that the physical characteristics of the fats determines the postprandial lipaemic response rather than the positional composition of the test fats. This is likely to be because fats that are crystalline solids at body temperature form micelles less readily, which is a critical step in determining the rate of lipolysis, and would suggest a slower absorption of crystalline fats and high-melting-point TAG-MS. To investigate this, we isolated chylomicron-TAG at hourly intervals (up to 8 h postprandially) following the consumption of native v. randomly chemically interesterified stearic and palmitic acid-rich fats, but did not observe any differences in the chylomicron total and sn-2 fatty acid composition between fats despite differences in postprandial lipaemia(Reference Berry and Sanders89, Reference Sanders, Berry and Miller90). This would suggest that the different TAG-MS (for example, PPP, PPO, POO) are metabolised at similar rates in humans and that the crystalline state of the fat mixture rather than individual TAG-MS is the important determinant of the rate of metabolism. Further studies are needed to investigate the effects of fats with different positional composition but broadly similar physical characteristics, in order to distinguish between effects of positional composition and physical properties.
The postprandial phase involves the complex interaction of metabolic systems and is also associated with changes in novel cardiovascular risk factors such as insulin sensitivity, haemostatic factors and endothelial function. Fats of different positional composition may induce differences in insulin and glucose responses due to effects of differing rates of metabolism on gut hormone responses and/or exposure of pancreatic β cells to SFA. When chylomicron-TAG are hydrolysed by lipoprotein lipase, some of the NEFA released may escape uptake into the tissues and circulate as NEFA in the plasma. Postprandial changes in the NEFA pool have been shown to reflect the fatty acid composition of the test meal(Reference Fielding, Callow and Owen93), which with the combined action of insulin suppressing hormone-sensitive lipase, will result in circulating NEFA primarily from dietary origin. Different fatty acids increase insulin differently depending on their chain length and saturation(Reference Stein, Stevenson and Chester94). Therefore, if different fatty acids are preferentially hydrolysed by lipoprotein lipase, depending on their positional composition and released as NEFA(Reference Nilsson-Ehle, Egelrud and Belfrage16) it is plausible that differences in the positional composition of test fats may result in differences in postprandial insulin and glucose concentrations. Indeed, Berry et al. (Reference Berry, Woodward and Yeoh23) reported greater increases in insulin at 30, 90 and 120 min following native palm oil v. randomly chemically interesterified palm oil(Reference Berry, Woodward and Yeoh23) and Sundram et al. (Reference Sundram, Karupaiah and Hayes76) reported an impaired insulin secretion following an interesterified stearic acid-rich fat compared with native palm oil(Reference Sundram, Karupaiah and Hayes76), suggesting that SFA in the sn-2 position may have an inhibitory effect on β cell function. However, this observation requires confirmation, as these studies were not designed to test the effect of TAG structure on postprandial glucose and insulin concentrations.
It has also been proposed that TAG structure may influence acute changes in haemostatic factors, in particular factor VII activated (FVIIa) concentration. Indeed, our group reported a lower FVIIa response following randomly chemically interesterified fats rich in stearic acid(Reference Sanders, Berry and Miller90) and palmitic acid(Reference Berry, Woodward and Yeoh23) compared with the corresponding native fat, which may be a consequence of the attenuated postprandial lipaemic response. However, it was previously shown that the extent of the postprandial increase in plasma TAG was not proportional to the increase in FVIIa(Reference Sanders, Oakley and Cooper92); therefore it is possible that the physical properties of the interesterified fats may influence the postprandial FVIIa response by their effects on the fluidity of the chylomicron surface and the subsequent incorporation of phospholipids which may have downstream effects on FVIIa concentrations(Reference Mariani, Bernardi and Bertina95, Reference Kjalke, Silveira and Hamsten96).
Summary and conclusions
The nutritional-related effects of interesterified fats have largely been overlooked as a potential health issue. However, the food industry now relies heavily on interesterified fats to produce fats with required physical properties. Their incorporation into food products is likely to increase with time, due to the removal of partially hydrogenated fatty acids and the relative expense and sustainability of naturally occurring fats with desirable functionality (such as cocoa butter). The health effects of interesterified fat blends should therefore be considered as an important nutritional issue to address. Although animal and infant studies provide good evidence to suggest that interesterified plants fats, with a greater proportion of long-chain SFA in the sn-2 position, are better absorbed, this is not supported by human adults studies. The chronic effects of interesterified fats and fats with different TAG structure on digestibility and fasting lipids in adult humans are not well studied. Furthermore, interpretation of results is complicated by differences in the amount of fat fed, duration of feeding period, subject population and choice of metabolic study or free-living study. To date, the evidence would suggest that TAG structure has no effect in human adults on either digestibility or fasting lipids and that interesterified fats do not elicit a different metabolic response when compared with their native counterparts. Additional research is required to clarify the long-term health effects of interesterified fats used commercially. The acute effects of TAG structure and interesterification are better characterised, but studies are confounded by concomitant effects on the physical characteristics of the fats. The evidence summarised in the present review suggests that when TAG structure and interesterification influence melting properties to produce fats that are crystalline at body temperature, there is an attenuation of the postprandial lipaemic response. However, further research should aim to distinguish between effects of physical characteristics and the proportion of palmitic or stearic acid in the sn-2 position. Recent studies also highlight the need to address the acute and chronic effects of differences in TAG structure on insulin sensitivity. In summary, there is little evidence to support a deleterious effect of interesterified fats or fats rich in sn-2 SFA on chronic or acute health outcomes.
Acknowledgements
I thank Dr Martijn Zieverink, Unimills B.V., the Netherlands, for technical advice.
I have no conflicts of interest.