Introduction
Alveolar echinococcosis (AE), caused by the cestode Echinococcus multilocularis, and cystic echinococcosis (CE), caused by Echinococcus granulosus sensu lato are parasitic zoonoses and represent two of the neglected tropical diseases recognized by the World Health Organization (Agudelo Higuita et al., Reference Agudelo Higuita, Brunetti and McCloskey2016). CE is more frequent than AE and causes more than 1 million disability-adjusted life years (DALYs) in humans, while the impact of AE accounts for more than 600 000 DALYs (Torgerson and Macpherson, Reference Torgerson and Macpherson2011). CE is distributed worldwide and is endemic in Peru, Chile, Argentina, Uruguay, Southern Brazil, the Mediterranean region, Central Asia, western China and eastern Africa (Agudelo Higuita et al., Reference Agudelo Higuita, Brunetti and McCloskey2016). AE is confined to the Northern hemisphere, notably Central and eastern Europe, Russia, China, northern Japan and the northern Region of North America, in particular Alaska (Stojkovic and Junghanss, Reference Stojkovic and Junghanss2013; Deplazes et al., Reference Deplazes, Rinaldi, Alvarez Rojas, Torgerson, Harandi, Romig, Antolova, Schurer, Lahmar, Cringoli, Magambo, Thompson and Jenkins2017). Whereas in CE the liver, lung and other organs can be affected, the most commonly affected organ in human AE is the liver (Hizem et al., Reference Hizem, M'Rad, Oudni-M'rad, Mestiri, Hammedi, Mezhoud, Zakhama, Mokni and Babba2015; Kern et al., Reference Kern, Menezes da Silva, Akhan, Mullhaupt, Vizcaychipi, Budke and Vuitton2017). In both species, metacestodes comprise fluid-filled vesicles formed by cellular and acellular compartments (Hemphill et al., Reference Hemphill, Stadelmann, Scholl, Muller, Spiliotis, Muller, Gottstein and Siles-Lucas2010). The outer, acellular surface is formed by the laminated layer (LL), a carbohydrate-rich structure synthesized by the parasite. Furthermore, E. granulosus metacestodes are surrounded by a very prominent host-derived fibrous capsule, the adventitial layer, which is composed of host connective tissue (Hemphill et al., Reference Hemphill, Stadelmann, Scholl, Muller, Spiliotis, Muller, Gottstein and Siles-Lucas2010). The cellular compartment of the parasite is formed by the germinal layer (GL), the distal part of which is the tegument that directly attaches to the inner surface of the LL (Hemphill et al., Reference Hemphill, Stadelmann, Scholl, Muller, Spiliotis, Muller, Gottstein and Siles-Lucas2010). Echinococcus granulosus metacestodes form large unilocular and well-defined cysts which increase in size. In contrast, E. multilocularis metacestodes grow infiltratively into surrounding organs and give rise to multi-vesicular cysts that form a labyrinth of chambers.
The preferred treatment option for AE is radical surgery (Kern et al., Reference Kern, Menezes da Silva, Akhan, Mullhaupt, Vizcaychipi, Budke and Vuitton2017), which is combined with chemotherapeutical treatment with benzimidazole carbamate derivatives such as albendazole (ABZ) or mebendazole (MBZ). Nevertheless, surgery is not feasible for all patients. Inoperable patients are treated solely by benzimidazole therapy, which is far from optimal. Although ABZ and MBZ have improved the survival rate and also the quality of life of many affected patients (Eckert and Deplazes, Reference Eckert and Deplazes2004; Grüner et al., Reference Grüner, Kern, Mayer, Gräter, Hillenbrand, Barth, Muche, Henne-Bruns and Kratzer2017), these drugs mostly do not exhibit parasiticidal activity against AE, leading to recurrence after treatment interruption (Hemphill et al., Reference Hemphill, Stadelmann, Rufener, Spiliotis, Boubaker, Muller, Muller, Gorgas and Gottstein2014). Thus, benzimidazole therapy has to be continued life-long, and results in high treatment costs (Horton, Reference Horton1989), and adverse side effects such as hepatotoxicity and this can lead to treatment discontinuation. In such instances, patients are left without other effective therapy options (Hemphill et al., Reference Hemphill, Stadelmann, Rufener, Spiliotis, Boubaker, Muller, Muller, Gorgas and Gottstein2014). For these reasons, alternative drugs for the treatment of AE are needed.
Over the last decades, in vitro culture models for E. multilocularis metacestodes were established and optimized, allowing large-scale in vitro drug screening (Hemphill et al., Reference Hemphill, Stettler, Walker, Siles-Lucas, Fink and Gottstein2002; Spiliotis et al., Reference Spiliotis, Tappe, Sesterhenn and Brehm2004; Hemphill et al., Reference Hemphill, Stadelmann, Rufener, Spiliotis, Boubaker, Muller, Muller, Gorgas and Gottstein2014; Stadelmann et al., Reference Stadelmann, Rufener, Aeschbacher, Spiliotis, Gottstein and Hemphill2016; Rufener et al., Reference Rufener, Dick, D'Ascoli, Ritler, Hizem, Wells, Hemphill and Lundstrom-Stadelmann2018). Medicinal plants have been in use since ever for the treatment of human diseases, and over the past decades they have attracted increasing interest in the search for treatments against infectious agents. They exhibit powerful pharmacological activities, they are accessible, are relatively low in cost, and have generally low toxicities (Graham-Brown and Healsmith, Reference Graham-Brown and Healsmith2018; Rogozea, Reference Rogozea2018). The pharmaceutical properties of aromatic plants are partially attributed to essential oils (EO) (Maggiore et al., Reference Maggiore, Albanese, Gende, Eguaras, Denegri and Elissondo2012). Several studies demonstrated the antiparasitic activities of various EO of medicinal plants, in particular also against Echinococcus spp. (Maggiore et al., Reference Maggiore, Albanese, Gende, Eguaras, Denegri and Elissondo2012; Jahanbakhsh et al., Reference Jahanbakhsh, Azadpour, Tavakoli Kareshk, Keyhani and Mahmoudvand2016; Moazeni et al., Reference Moazeni, Borji, Saboor Darbandi and Saharkhiz2017; Noal et al., Reference Noal, Monteiro, Brum, Emmanouilidis, Zanette, Morel, Stefanon, Frosi and la Rue2017; Sharifi-Rad et al., Reference Sharifi-Rad, Tayeboon, Niknam, Sharifi-Rad, Mohajeri, Salehi, Iriti and Sharifi-Rad2018).
Thymus capitatus Hoff. et Link. is a species of the genus Thymus (Lamiaceae) that is a native species in the Mediterranean region. It is a perennial herbaceous plant growing in the wild in Tunisia and is traditionally used as a spicy herb for culinary preparations, but it is also employed by cosmetic and fragrance industries. Additionally, thyme tea is consumed as a traditional remedy against gastro-intestinal disorders and in earlier times its EOs were taken to expel intestinal parasites (Figueiredo et al., Reference Figueiredo, Barroso, Pedro, Salgueiro, Miguel and Faleiro2008). Thyme species are also used as antispasmodics, tonics, antiseptics, antitussives and carminatives (Iauk et al., Reference Iauk, Acquaviva, Mastrojeni, Amodeo, Pugliese, Ragusa, Loizzo, Menichini and Tundis2015). The antiparasitic, antibacterial, antifungal, antioxidant, antinociceptive and hypoglycaemic properties of T. capitatus were demonstrated by many authors (Boubaker Elandaogylousi et al., Reference Boubaker Elandaogylousi, Akkari, B'Chir, Gharbi, Mhadhbi, Awadi and Darghouth2013; Goncalves et al., Reference Goncalves, de Meneses, de Vasconcelos, Piauilino, Almeida, Napoli, Ruberto and de Araujo2017; Saoudi et al., Reference Saoudi, Sifaoui, Chammem, Reyes-Batlle, Lopez-Arencibia, Pacheco-Fernandez, Pino, Hamdi, Jimenez, Bazzocchi, Pinero and Lorenzo-Morales2017; Grande-Tovar et al., Reference Grande-Tovar, Serio, Delgado-Ospina, Paparella, Rossi and Chaves-Lopez2018).
To the best of our knowledge, the effect of T. capitatus EO and its fractions have never been investigated in Echinococcus metacestodes. In order to fill this gap, we here demonstrate the effects of EO and defined EO fractions of T. capitatus as well as some of its major components against in vitro cultured E. multilocularis metacestodes. Metacestode-damage and parasiticidal potential were assessed in vitro. Ultrastructural analysis of treatment effects was performed by electron microscopy.
Materials and methods
If not stated otherwise, all chemicals were purchased from Sigma (St. Louis, MO, USA). Dulbecco's modified Eagle medium (DMEM) and fetal bovine serum (FBS) were from Biochrom (Berlin, Germany), and all other culture media and reagents were from Gibco-BRL (Zürich, Switzerland). Plasticware was from Sarstedt (Numbrecht, Germany).
Plant material and EO extraction
The aerial parts of T. capitatus Hoff. et Link. were harvested from the delegation of Monastir, in the Central coast of Tunisia in November 2013. A specimen (T.C.008) was deposited at the Laboratory of Medical and Molecular Parasitology-Mycology (LP3M, code LR12 ES08) at the Faculty of Pharmacy of Monastir, University of Monastir, Tunisia. The plant was air dried in shady conditions. Completely dried leaves were then separated from the stem and stored at room temperature in the absence of humidity. EO was obtained from the leaves by hydrodistillation for 4 h using a Clevenger-type apparatus. The EO was then stored at 4 °C in sealed glass vials prior to further analysis and bioassays.
EO fractionation by column chromatography
The EO was passed through a silica gel column (silica gel 60, Merck 7734) using pentane-diethyl ether solvent system with increasing polarity. The eluted fractions were concentrated under reduced pressure for solvent evaporation and examined by thin layer chromatography (TLC). The fractions presenting the same migration pattern were mixed resulting in 7 main fractions (F1–F7) (Fig. 1).
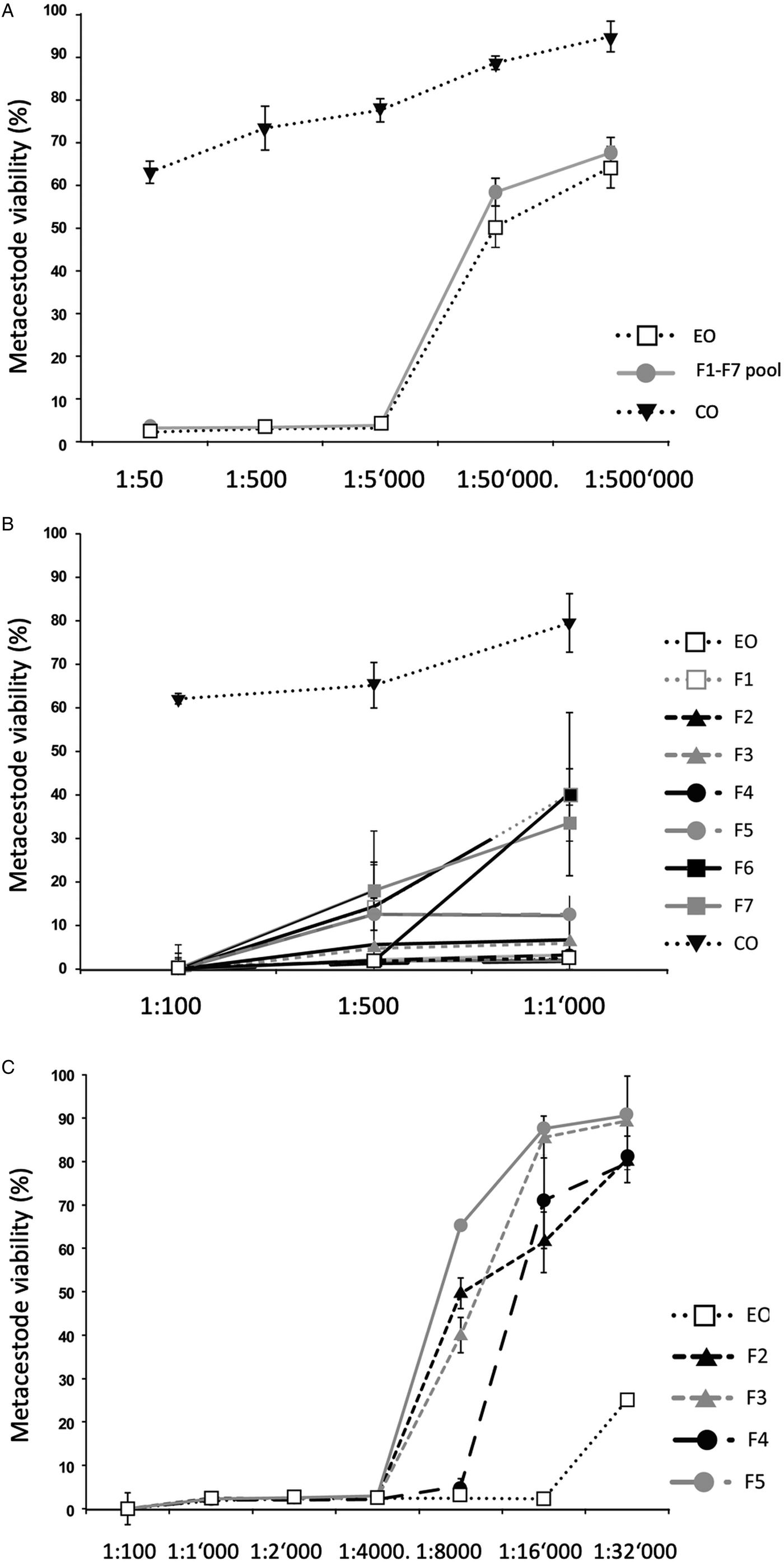
Fig. 1. Impact of T. capitatus EO and pooled fractions F1–F7 on E. multilocularis metacestode viability assessed by Alamar Blue assay. The tested dilutions are indicated on the x-axis. All measurements were performed 5 days after addition of compounds. On the y-axis, Metacestode viability (%) indicates the Alamar blue values relative to the ones obtained with controls treated with the corresponding DMSO vehicle control. Average values and respective standard deviations obtained from biological triplicates are shown. (A) Assessment of EO, the F1–F7 pool, and CO. (B) Assessment of EO, the F1–F7 individually and CO at three low dilutions (1:100, 1:500 and 1:1000). (C) Effects of EO, and of F2, F3, F4 and F5 individually at dilutions ranging from 1:100 to 1:32 000. CO, corn oil; EO, essential oil; DMSO, dimethyl sulfoxide.
Gas chromatography (GC) analysis of EO and fractions
GC analysis was carried out with an HP-5890 series II instruments equipped with a flame ionization detector (FID) and HP-5 capillary column (30 m × 0.25 mm ID, 0.25 µ m film thickness), working with the following temperature program: 50 °C for 1 min, ramp of 5 °C min−1 up to 280 °C; injector and detector temperatures 250 and 208 °C. Nitrogen was used as carrier gas at a flow rate of 1.2 mL min−1. A sample of 0.5 µL of diluted pure EO/Fs in 10% hexane was injected using split mode (split ratio 1:30). The relative proportions of the EO/Fs constituents were carried out using a built-in data-handling program provided by the manufacturer of the gas chromatograph. Component identification was carried out by comparing their retention times with those of pure authentic samples and by means of their linear retention index (LRI), relative to the series of n-hydrocarbons.
GC-mass spectrometry (MS) analysis of EO and fractions
GC-MS analysis was performed with a Varian CP-3800 (Palo Alto, CA) gas-chromatograph equipped with a HP-5 capillary column (30 m × 0.25 mm; coating thickness 0.25 mm) and a Varian Saturn 2000 ion trap mass detector. Injector and transfer line temperatures were set to 220 and 240 °C, respectively; oven temperature programmed from 60 to 240 °C at 3 °C min−1; carrier gas helium at 1 mL min−1; injection of 0.2 mL 10% hexane solution; split ratio 1:30. Identification of the EO and fractions was based on comparison of their linear retention indices relative to the series of n-hydrocarbons, and on computer matching against commercial (NIST 2014 and ADAMS) and home-made libraries, mass spectra built up from pure substances and components of known EOs as well as MS literature (Jennings and Shibamoto, Reference Jennings and Shibamoto1980; Davies, Reference Davies1990; Robert, Reference Robert1995; Stenhagen and McLafferty, Reference Stenhagen and McLafferty1974; Swigar and Silverstein, Reference Swigar and Silverstein1981).
In vitro culture and preparation of E. multilocularis metacestodes for the assessment of EO and EO components
Echinococcus multilocularis metacestodes (isolate H95) were recovered from experimentally infected BALB/c mice and were cultured in vitro as previously described (Stadelmann et al., Reference Stadelmann, Rufener, Aeschbacher, Spiliotis, Gottstein and Hemphill2016). Metacestodes were used for experiments when they reached diameters of 2–4 mm (Stadelmann et al., Reference Stadelmann, Scholl, Muller and Hemphill2010). Treatments were performed as described by Stadelmann et al. (Reference Stadelmann, Scholl, Muller and Hemphill2010). Briefly, medium without phenol red (DMEM, 100 U mL−1 penicillin G, 100 µg mL−1 streptomycin sulfate) was added to the same volume of vesicles, and parasites were distributed to 48 well plates (Greiner Bio-One, Frickenhausen, Germany) at 1 mL well−1 (12–15 vesicles well−1). Subsequently, EO, EO fractions or defined EO components were added (see below), and further incubated at 37 °C/5% CO2.
Assessment of metacestode damage induced by major EO components by phosphoglucose isomerase (PGI) assay
A measure of 100 mm stock solutions of the five pure EO components carvacrol, β-caryophyllene, limonene, thymol and eugenol were prepared in dimethyl sulfoxide (DMSO). Pre-dilutions of these compounds were prepared in culture medium and added to the metacestodes resulting in final concentrations of 100, 50, 25 and 12.5 µ m. As negative controls, non-treated metacestodes were incubated with the corresponding amount of DMSO. Triton X-100 (0.1% in PBS) was applied as a positive control [maximal release of vesicle fluid (VF)]. Each condition was tested in biological triplicate. Metacestodes were cultured in the presence of the compounds for 5 days at 37 °C and 5% CO2, humid atmosphere, after which PGI release was quantified as reported by Stadelmann et al. (Reference Stadelmann, Scholl, Muller and Hemphill2010). PGI-activity was calculated from the corresponding linear regression parameters (ΔA 340/Δt). The background was subtracted based on DMSO treatment values. Further quantification is presented as a percentage of the positive control treated with Triton X-100 (Stadelmann et al., Reference Stadelmann, Aeschbacher, Huber, Spiliotis, Muller and Hemphill2014). Linear regression and calculation of averages and standard deviations of each triplicate was performed in Microsoft Excel 2010.
In vitro assessment of metacestode viability by Alamar Blue assay
The setup of metacestode vesicles for Alamar Blue assay was the same as that described for PGI-assay. The Alamar Blue assay assesses aerobic respiration and thus the viability of cells by measuring the conversion of resazurin (weakly fluorescent) to resofurin (strongly fluorescent). The EO and a respective pool of F1–F7 were tested in a first assay using the dilutions 1:50, 1:500, 1:5000, 1:50 000 and 1:500 000. A second test was carried out with 3 lower dilutions of EO and F1-F7 (1:100, 1:500 and 1:1000). Fractions showing <20% viability at 1:1000 were further investigated at higher dilutions (1:100, 1:1000, 1:2000, 1:4000, 1:8000, 1:16 000, and 1:32 000). Negative controls were metacestodes treated with corn oil (CO) and non-treated metacestodes. As a positive control, metacestodes were supplemented with Triton X-100 (0.1%). The components carvacrol, β-caryophyllene, limonene, thymol and eugenol were prepared as 100 mm stocks in DMSO and pre-dilutions of the components were added to metacestodes at final concentrations of 100, 50, 25 and 12.5 µ m, with the corresponding amounts of DMSO as a negative control, and 0.1% Triton-X-100 as a positive control. Treatments were performed during 5 days at 37 °C, 5% CO2, humid atmosphere. Subsequently, vesicles were mechanically broken with a 1 mL pipette tip, resazurin (final concentration 20 mg mL−1) was added and suspended (Stadelmann et al., Reference Stadelmann, Rufener, Aeschbacher, Spiliotis, Gottstein and Hemphill2016). Each condition was tested in biological triplicate. Fluorescence at 595 nm was measured in an EnSpire multilabel reader (Perkin Elmer, Waltham, MA, USA) at 0 h and 5 h after the addition of resazurin. The increase in fluorescence over time was used to calculate the relative viability in relation to the respective DMSO control. IC50 calculations were made based on logit-log transformation in Microsoft Excel 2010.
In vitro viability assessment of E. multilocularis GL cell cultures using CellTiter-Glo luminescent assay
The EO, the most active fractions from the Alamar Blue assay results, carvacrol, thymol, NaCl and CO were evaluated for their effects on GL cell viability. CellTiter-Glo assay measures ATP production and thus the viability of cells. NaCl was included in the assays as it is the most commonly used solution for cyst inactivation during surgery of uncomplicated CE surgery. GL cell extraction was performed according to Spiliotis et al. (Reference Spiliotis, Mizukami, Oku, Kiss, Brehm and Gottstein2010). After overnight aggregate formation, cells (15 units well−1) were distributed to a black 384 well plate (Nunc, Thermo Scientific, Reinach, Switzerland) in 12.5 µL medium. EO and fractions were added in 12.5 µL medium to the cells to final dilutions of 1:1000, 1:16 000, 1:32 000, 1:64 000 and 1:128 000 in quadruplicates. NaCl was added to a final concentration of 20% in 12.5 µL medium. Carvacrol and thymol were added in 12.5 µL medium to the cells to concentrations of 3, 10, 30, 100 and 300 µ m in quadruplicates. Non-treated cells (medium only) and CO were used as negative controls for EO and respective fractions, while medium was used as a control for 20% NaCl. The corresponding amount of DMSO represented the negative control for both carvacrol and thymol.
After 5 days of culture at 37 °C under humid nitrogen atmosphere, the viability of GL cells was assessed by CellTiter-Glo luminescent assay. In brief, 25 µL CellTiter-Glo (Promega, Dübendorf, Switzerland) including an additional 1% Triton X-100, was added (Stadelmann et al., Reference Stadelmann, Rufener, Aeschbacher, Spiliotis, Gottstein and Hemphill2016). Plates were incubated for 15 min on a shaker at room temperature and complete disruption of all cell aggregates was confirmed by light microscopy before measurement of luminescence in an EnSpire multilabel reader (Perkin Elmer, Waltham, MA, USA) (Stadelmann et al., Reference Stadelmann, Rufener, Aeschbacher, Spiliotis, Gottstein and Hemphill2016). All values were set in relation to the corresponding controls. Averages, standard deviations and the IC50s were determined after logit-log transformation in Microsoft Excel 2010.
In vitro cytotoxicity assessments in Reuber rat hepatoma (RH) cells and human foreskin fibroblasts (HFF)
The toxicity of the EO and the most active fractions on confluent and pre-confluent mammalian cells was assessed in vitro by Alamar Blue assay (Stadelmann et al., Reference Stadelmann, Rufener, Aeschbacher, Spiliotis, Gottstein and Hemphill2016). To evaluate the growth inhibitory effects on confluent cells, 1 × 104 HFF well−1 or 5 × 104 RH cells well−1 were seeded in a 96 well plate in DMEM supplemented with FBS (10%), 100 U mL−1 penicillin G, 100 µg mL−1 streptomycin and 0.25 µg mL−1 amphotericin B. Cultures were maintained overnight at 37 °C, 5% CO2, humid atmosphere. To evaluate the growth inhibitory effects on proliferating cells, 1 × 103 HFF well−1 or 5 × 103 RH cells well−1 were seeded and allowed to attach for 5 h at 37 °C before addition of the drugs. Subsequently, the culture medium was removed and EO and fractions were added in serial 1:2 dilutions in medium starting at 1:100 in triplicates. The dilution range was further adjusted in subsequent setups. After 5 days of cultivation at 37 °C and 5% CO2, cultures were visually inspected by microscopy and viability quantified by Alamar Blue assay (Stadelmann et al., Reference Stadelmann, Rufener, Aeschbacher, Spiliotis, Gottstein and Hemphill2016). Fluorescence was measured and viability calculated as described above.
Transmission electron microscopy (TEM)
Ultrastructural alterations were investigated by TEM. Samples of in vitro cultured metacestodes were processed as described by Hemphill and Croft (Hemphill and Croft, Reference Hemphill, Croft and Rogan1997). In short, the specimens were immersed in 2% glutaraldehyde in 0.1 M sodium-cacodylate buffer, pH 7.3 for 1 h at room temperature, followed by post-fixation in 2% osmium tetroxide in 0.1 M sodium-cacodylate buffer for 2 h at room temperature. Samples were washed in distilled water and treated with 1% uranyl acetate for 30 min, washed again with distilled water and dehydrated by sequential incubations in ethanol (30, 50, 70, 90 and three times 100%). Specimens were subsequently embedded in epoxy resin (Epon 812, Fluka) and polymerization of the resin was carried out overnight at 60 °C. Sections (80–90 nm of thickness) were cut on a Reichert and Jung ultramicrotome, loaded onto 300-mesh formvar-carbon coated nickel grids (Plano GmbH, Marburg, Germany), stained with uranyl acetate and lead citrate and examined on a Phillips EM400 transmission electron microscope operating at 80 kV.
Results
Chemical profile of T. capitatus EO and fractions
The yield of EO from T. capitatus leaves was 1.739 mL 100 g−1. The chemical composition of T. capitatus EO and fractions obtained by GC-MS is shown in Table 1. A total of 22 components representing 99.1% of the total EO, were identified. The major constituent is carvacrol (82.4%), followed by p-cymene (5.3%). Thymol (0.3%), a structural isomer of carvacrol, is also present. The data shown in Table 1 indicates that oxygenated monoterpenes, accounting for 87.3% of the total EO, are the most abundant compounds and they account for 87.3% of total EO. EO contains also 9.7% monoterpene hydrocarbons, 1.2% sesquiterpene hydrocarbons, 0.8% oxygenated sesquiterpenes and 0.1% phenylpropanoids.
Table 1. Chemical composition of Thymus capitatus essential oil (EO) and respective fractions (F1–F7)
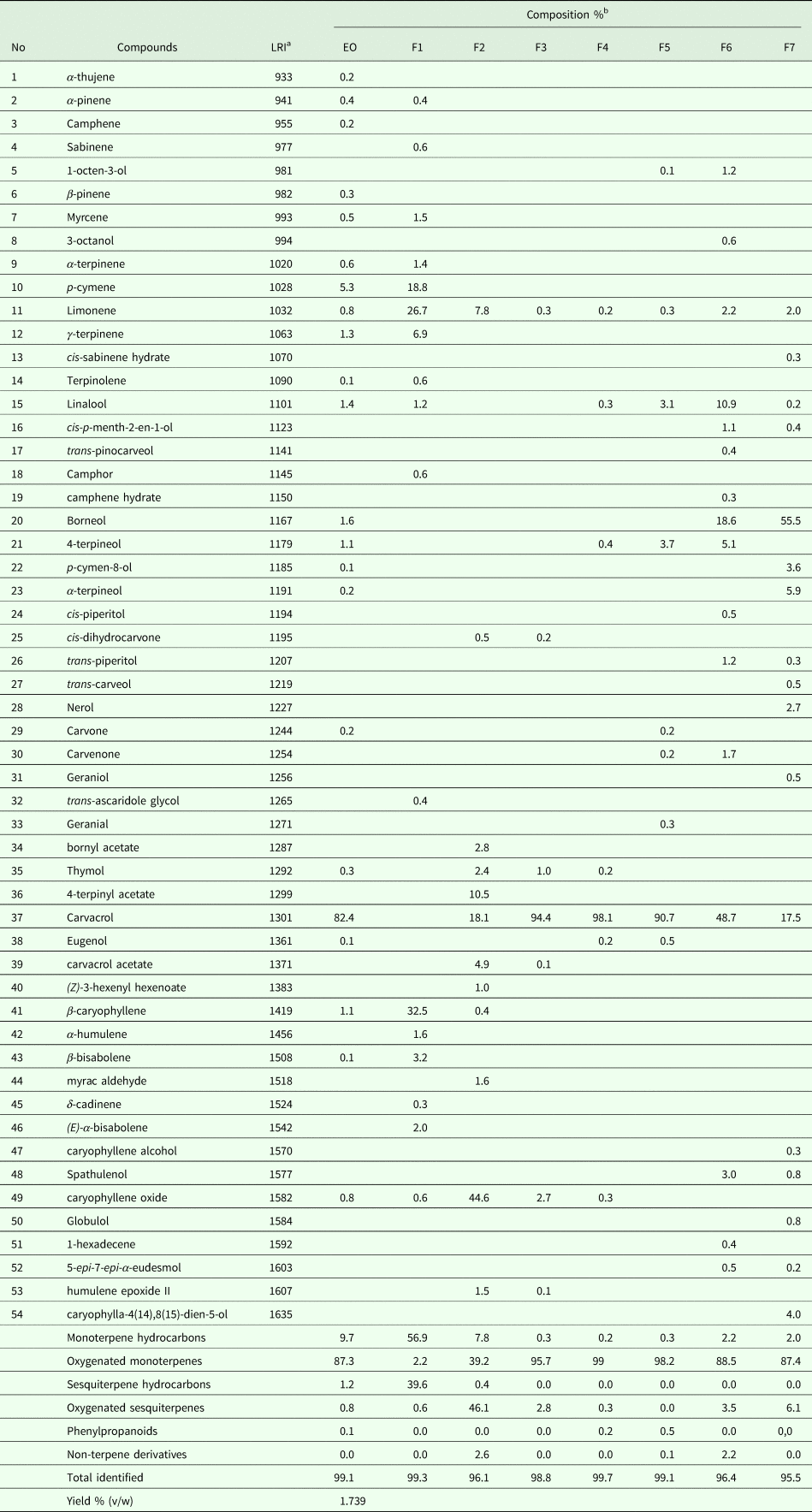
a LRI, linear retention indices (HP-5-column).
b %, percentage calculated by GC-FID on non-polar capillary column HP-5.
Separation of 5000 mg of EO by silica gel chromatography yielded 7 fractions representing 2.4% (F1, 117 mg), 0.4% (F2, 18 mg), 28.8% (F3, 1436 mg), 20.9% (F4, 1041 mg), 15% (F5, 745 mg), 2.9% (F6, 143 mg) and 0.68% (F7, 34 mg) of the total EO. F1 mainly contained monoterpene hydrocarbons (56.9%) while F2 contained 46.1% of oxygenated sesquiterpenes. All other fractions (F3–F7) mostly comprised oxygenated monoterpenes (Table 1). Fractions 3, 4 and 5 contained carvacrol at 94.4, 98.1 and 90.7%, respectively.
In vitro activities of T. capitatus EO, fractions and selected components against E. multilocularis metacestodes
Metacestode damage induced by T. capitatus EO was initially assessed by PGI-assay (Supplementary Fig. S1). Very low PGI-levels were detected with the 1:5 and 1:20 dilution of EO. In contrast, visual inspection of parasites incubated in EO revealed a clear damaging impact on the metacestode structures (to view examples see Fig. 5).
To explain these findings, PGI-activity was directly measured in E. multilocularis VF in the presence of increasing dilutions of T. capitatus EO (1:25 to 1:32 000). EO directly interfered with the read-out of the PGI-assay in a concentration-dependent manner (Supplementary Fig. S1).
As an alternative, the effects of the EO and the 7 fractions on vesicle viability were assessed by Alamar Blue assay (Fig. 1). First, the activities of EO and a pool of all fractions (F1–F7) against E. multilocularis metacestodes were assessed (Fig. 1A). Similar curves were obtained for EO and the pooled fractions: both strongly impairing vesicle viability up to a dilution of 1:5000 (Fig. 1A). In contrast, CO had a much lower impact on vesicle viability (Fig. 1A). Subsequently, the EO and the 7 fractions F1–F7 were assessed individually employing 1:100, 1:500 and 1:1000 dilutions (Fig. 1B). The EO, F2, F3, F4 and F5 exhibited the clearest negative impact on metacestode viability, which was reduced by these fractions >80% or more already at the highest dilution. Thus, further analysis was carried out using dilutions ranging from 1:100 to 1:32 000 (Fig. 1C). EO reduced metacestode viability by over 70% even at the highest dilution, while F2, F3 and F4 showed more than 50% activity at 1:8000, with F4 being the most potent fraction (Fig. 1C). The exact dilutions corresponding to EC50 values of EO, F2, F3 and F4 against E. multilocularis metacestodes, as determined by Alamar Blue assay, were calculated to be 1:49 970, 1:7309, 1:9991 and 1:11 257, respectively (Table 2).
Table 2. In vitro activities of EO, F2, F3 and F4 against cultured mammalian cells, E. multilocularis metacestodes and isolated germinal layer cells

RH, rat hepatoma cells; HFF, human foreskin fibroblasts; GL, germinal layer cells.
The indicated dilutions correspond to the concentrations that achieved halfmaximal inhibition of cell viability (IC50) or viability of metacestodes (EC50), assessed by Alamar blue assay.
GC/MS revealed that the major components of EO are carvacrol, thymol, eugenol, limonene and β-caryophyllene (Table 1). These 5 compounds were acquired commercially, and were shown not to interfere in the PGI assay (data not shown) prior to assessing them individually by PGI-assay at 4 concentrations (100–12.5 µ m, 1:2 dilution series; Fig. 2A). At 100 µ m, limonene was the most effective compound (58.4% PGI-activity in relation to Triton-X-100 treatment), while the other compounds had activities in the range of 30% or below. However, at lower concentrations, limonene was less effective. These five compounds were also tested by Alamar Blue assay employing the same concentrations, but no impairment of metacestode viability was measured for any of these components (data not shown). In the next experiment, we assessed a concentration range of 150–850 µ m for carvacrol. Carvacrol caused PGI release of more than 50% starting at 550 µ m or higher concentrations (Fig. 2B). Alamar blue assay measured a decrease in metacestode viability to 50% after treatment with 550 µ m carvacrol and 35.23% at 850 µ m (Fig. 2B).
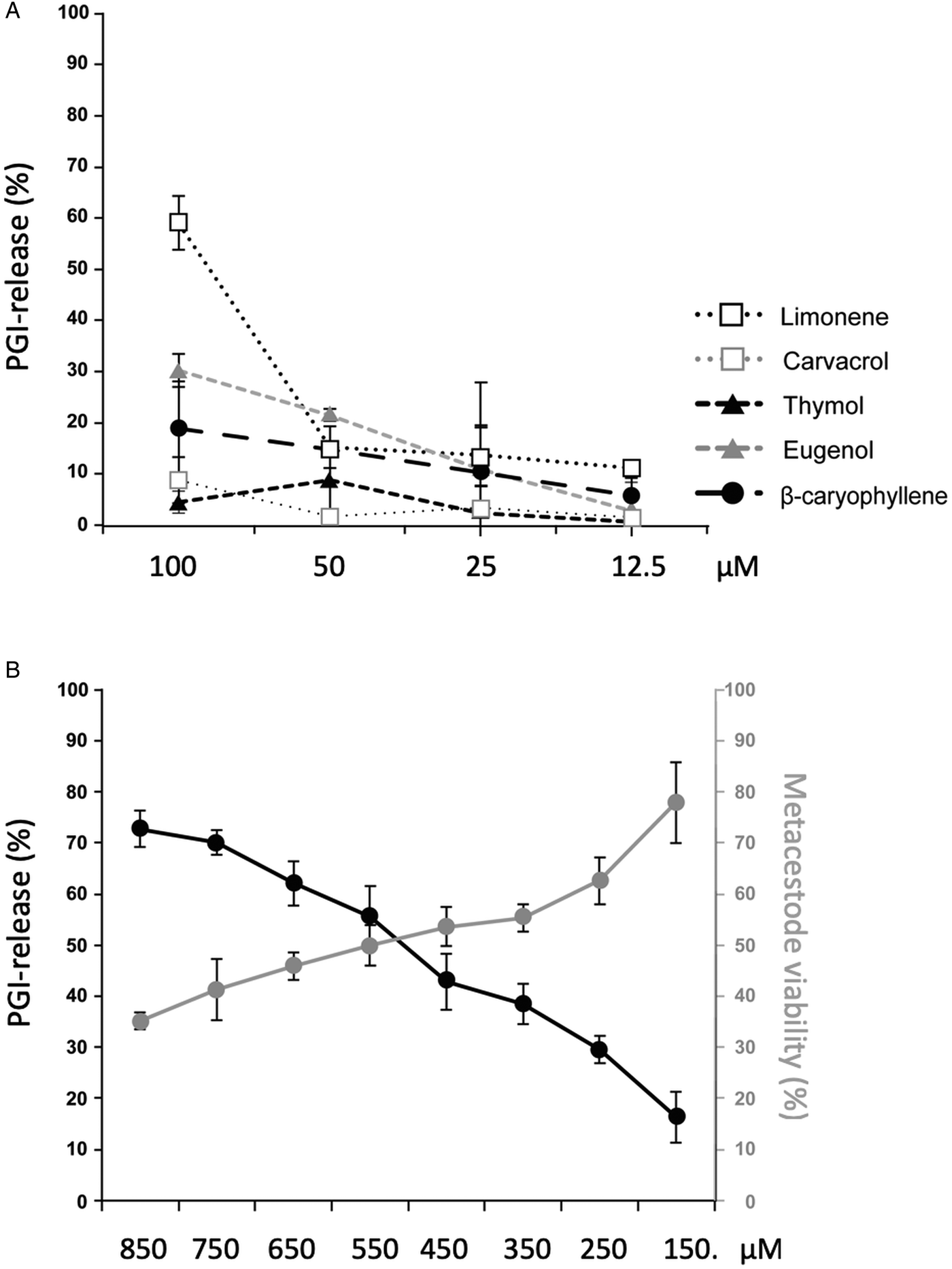
Fig. 2. Effects of the main T. capitatus EO components against E. multilocularis metacestodes. (A) PGI release after exposure of E. multilocularis metacestodes to limonene, carvacrol, thymol, eugenol and β-caryophyllene at concentrations from 100 to 12.5 µ m for 5 days. As positive control, 0.1% Triton X-100 was applied and the compound activities are expressed as percentage of this positive control. DMSO served as a negative control. Note the high PGI-activity levels in medium supernatants of metacestodes exposed to 100 µ m limonene. (B) Treatment of E. multilocularis metacestodes with high concentrations of carvacrol ranging from 850 to 150 µ m for 5 days. The black curve shows the assessment of vesicle damage by PGI-assay. The vesicle viability measured by Alamar Blue assay is depicted in grey. For all measurements, average values and standard deviations of biological triplicates are provided. EO, essential oil; PGI, phosphoglucose isomerase.
Effects of EO and selected fractions on the viability of cultured E. multilocularis GL cells
CellTiter-Glo assay was used to evaluate the impact of different dilutions of EO, F2, F3 and F4 on E. multilocularis primary cells. EO was less active against GL cells compared to metacestodes, with dilutions corresponding to IC50/EC50 values of 1:14 546 and 1:49 970, respectively (see Table 2). In contrast, F2 was twice as active, while for F3 and F4 similar dilutions in the 1:10 000 range were measured for both metacestodes and GL cells corresponding to EC50 and IC50 values, respectively.
Cytotoxicity of EO and selected fractions in RH cells and HFF
Cytotoxicity was assessed by Alamar Blue assay for EO, F2, F3 and F4 using confluent and pre-confluent cultures of RH cells and HFF. The dilutions corresponding to IC50 values are shown in Table 2. F2, F3 and F4 exhibited largely similar cytotoxicity values with the exception of F2, which showed reduced toxicity towards confluent and preconfluent HFF. EO was highly toxic for RH cells and HFF at a range of dilutions higher than dilutions corresponding to E. multilocularis GL cells. F2 and F4 were generally less toxic to host cells than to E. multilocularis cells. They were therefore regarded as the most promising EO fractions.
Morphological and ultrastructural investigations of treated E. multilocularis metacestodes by light and electron microscopy
Light microscopy (Fig. 3) showed that parasites exposed to CO or 0.2% DMSO did not show any alterations compared to non-treated metacestodes. Vesicles remained with intact turgor and the inner surface of the vesicle wall appeared to be densely covered by parasite tissue (Fig. 3A–C). When metacestodes were treated with 100 µ m carvacrol (Fig. 3D) or thymol (Fig. 3E), no alterations could be detected and vesicles remained largely intact, similar to the controls. In contrast, treatments with EO, F2, F3 and F4 at dilutions ranging from 1:500 to 1:32 000 resulted in dramatic morphological changes. The GL detached from the interior lining of the LL and formed a dense aggregate in the vesicle interior (Fig. 3F–P). Due to the detachment of the GL, the LL became translucent. These changes occurred most rapidly with EO treatments, already within 1 h at 1:500 (Fig. 3F) and also after 5 days of treatment at 1:32 000 (Fig. 3K).
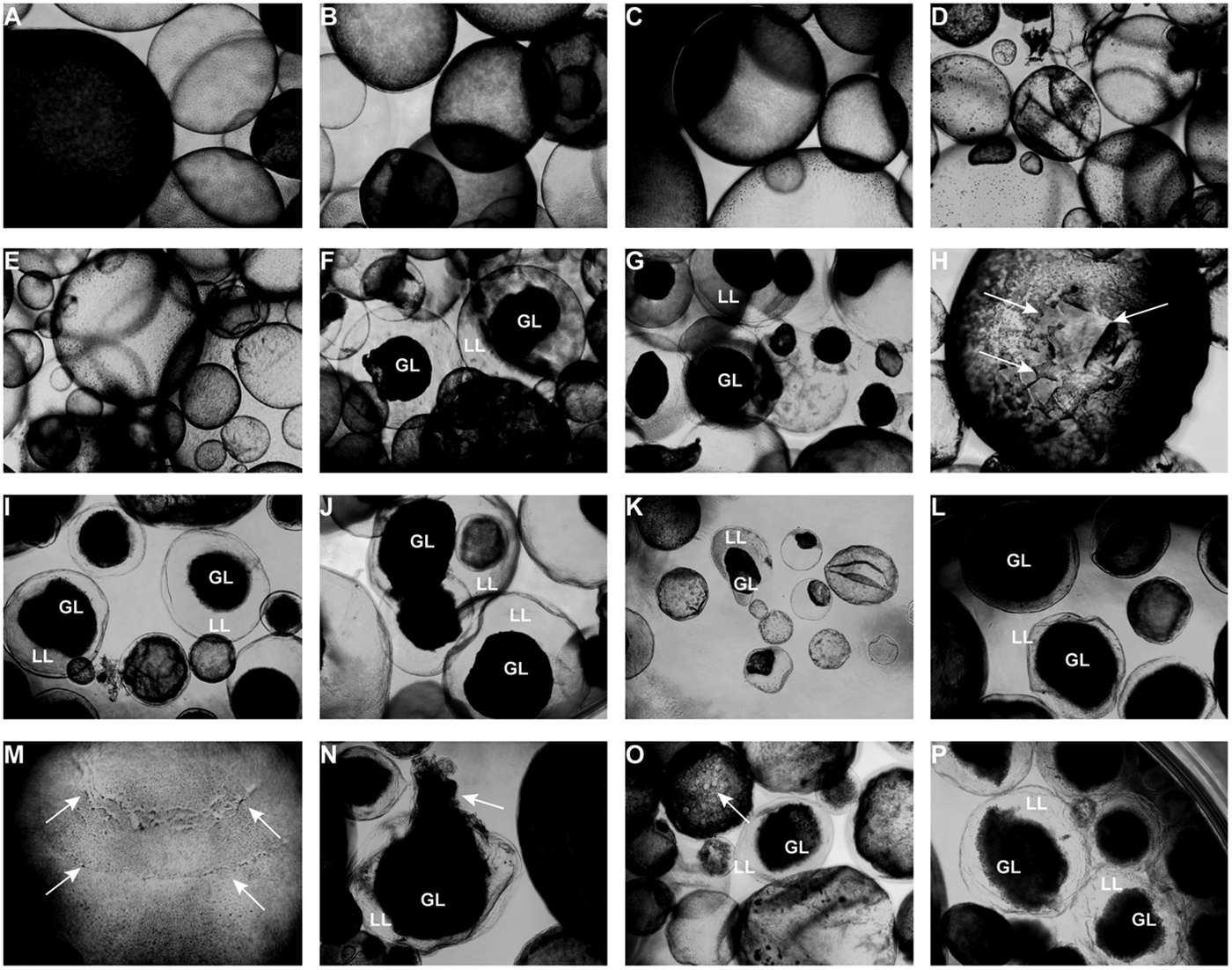
Fig. 3. Light microcscopy of E. multilocularis metacestodes treated in vitro with T. capitatus EO and EO components. All images (except for (F) were obtained at 20x magnification after 5 days of compound exposure. (A) Incubation with the corn oil; (B) Non-treated control; (C) 0.2% DMSO control; (D) Carvacrol at 100 µ m; (E) Thymol at 100 µ m; (F) EO diluted 1:500; (G-H) EO diluted 1:1000. Detachment of the germinal layer from the laminated layer is indicated by white arrows in (H). (I) EO diluted 1:4000. (J) EO diluted 1:16 000; (K) EO diluted 1:32 000; (L) F2 diluted 1:2000. (M) F2 diluted 1:8000. White arrows point towards alterations in the GL; (N) F3 diluted 1:2'000; (O) F4 diluted 1:1000, pore formation in the parasite tissue is indicated by white arrows; (P) F4 diluted 1: 8000. EO, essential oil; DMSO, dimethyl sulfoxide; GL, germinal layer.
TEM was employed to investigate the ultrastructural damage upon treatment of metacestodes. Untreated E. multilocularis metacestodes vesicles (Fig. 4A, B) exhibited the typical morphological features. An acellular LL surrounds the entire parasite, representing the outer surface of the metacestode. The proximal surface of the LL contained the parasite tissue, which is composed of the tegument, a syncytial layer that mediates the contact to the LL, with numerous microtriches protruding from the tegument into the LL. Small vesiculated structures are seen often in the vicinity, or still associated with microtriches indicating that they are released and then incorporated into the LL (Fig. 4F). The GL is a relatively densely packed tissue containing undifferentiated cells with a large nucleus and nucleolus (named also stem cells), muscle cells, nerve cells, connective tissue and fully-loaded glycogen storage cells (Fig. 4A, B). TEM micrographs taken after exposure to F1 did not show any structural alterations compared to the controls, validating the results obtained by Alamar Blue assay (Fig. 4C). After 1 h exposure to EO (1:400) (Fig. 4D) and after 5 days exposure to F4 (1:2 000) (Fig. 4E) TEM demonstrated complete lysis of parasite tissues. Compared to the LL of non-treated parasites (Fig. 4F), the LL of EO treated metacestodes appeared more electron dense, and contained, besides empty vesiculated structures, also many electron dense particles (Fig. 4G). Microtriches, if present at all, were profoundly shortened, or not present at all.
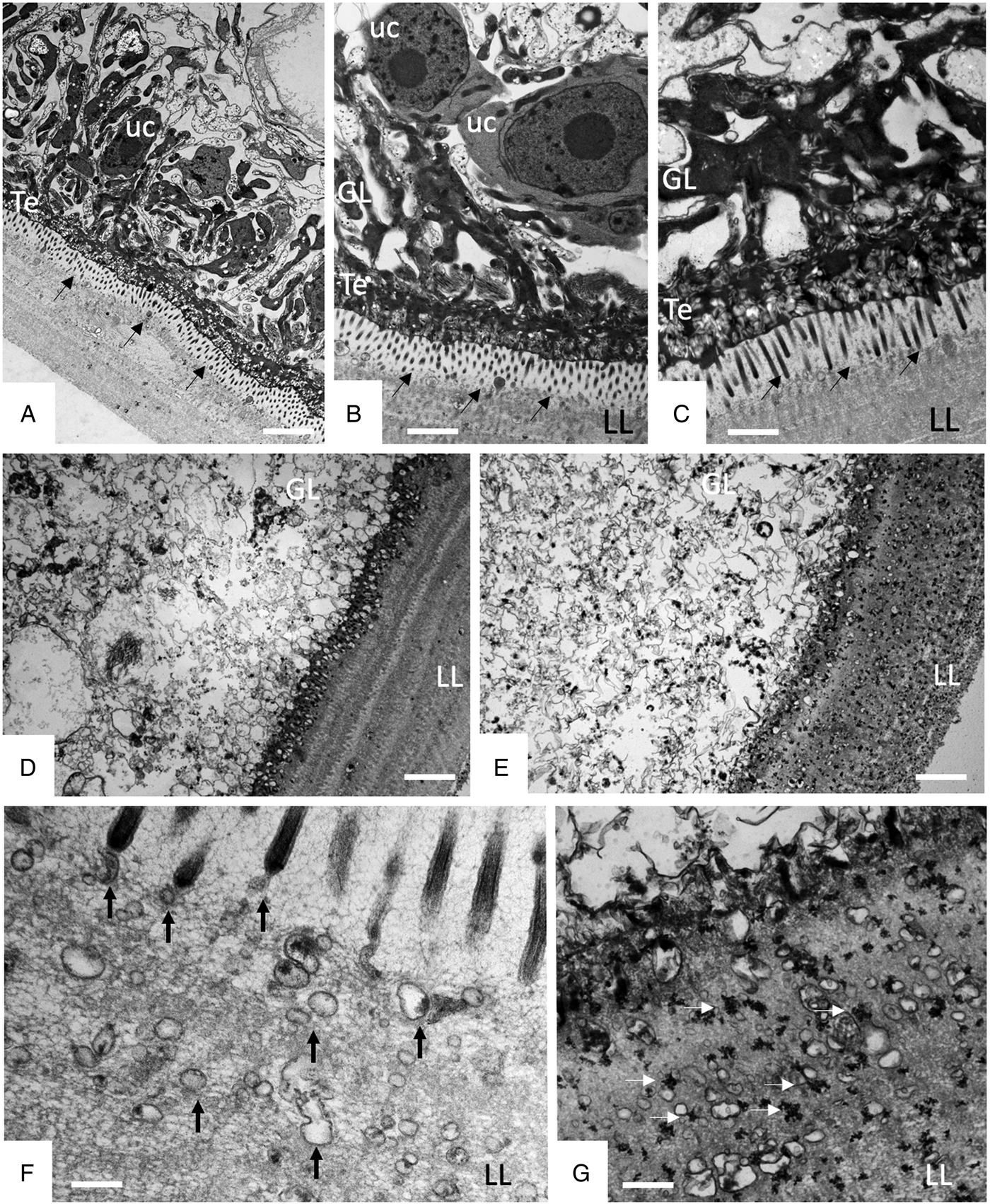
Fig. 4. Effects of EO (1 h, 1:400), F1(5 days, 1:2000) and F4 (5 days, 1:2000) on the ultrastructure of E. multilocularis metacestodes. (A) and (B) show sections through the metacestode wall of non-treated and corn oil-treated metacestodes, (C) metacestode treated with F1. Small arrows in (A–C) point towards microtriches. (D) was treated with EO (1:400) for 1 h, (E) was exposed to F4 (1:2000) during 5 days. (F) and (G) show higher magnification views of the interface between laminated layer and tegument in non-treated metacestodes (F) and parasites treated with F4 (G). Vertical black arrows in (F) point towards small vesiculated structures that are released and then incorporated into the LL; white horizontal arrows in (G) indicate the presence of electron-dense precipitates that are deposited into the laminated layer during treatment with F4. LL, laminated layer; GL, germinal layer; uc, undifferentiated cell; Te, tegument; Bars in A = 3.2 µ m; B = 1.8 µ m; C = 1.2; D and E = 2.0 µ m; F, G = 0.5 µ m.
Studies on early changes (within the first 48 h) induced by F4 showed that no notable changes occurred after 6 h of treatment (Fig. 5A), but first alterations such as the formation of large cytoplasmic vesicles with granular and membranous material of unknown nature could be seen after 12 h of treatment in some cells (Fig. 5B). Further changes progressively occurring with time led to a much thinner GL, gradual decay of microtriches and separation of the LL from the GL after 24–48 h (Fig. 5C–E).
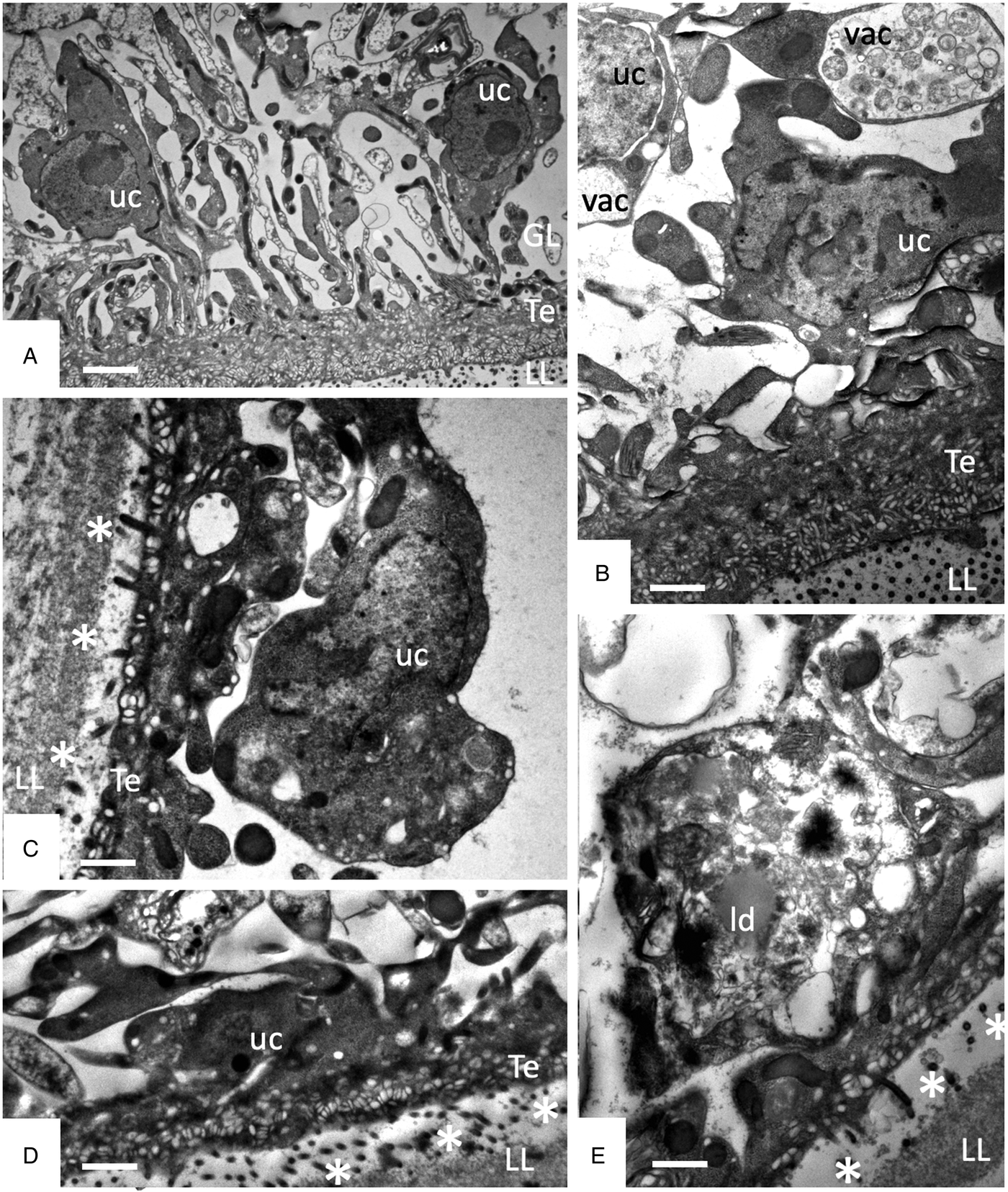
Fig. 5. Early changes within the first 48 h of treatments of E. multilocularis metacestodes with F4 (1:2000). (A) shows metacestodes after 6 h, (B) after 12 h, (D) and after 24 h, and (E) after 48 h of F4 treatment. LL, laminated layer; GL, germinal layer; Te, tegument; uc, undifferentiated cell; vac, vacuole; ld, lipid droplet; * indicates separation of LL and GL. Bars in A = 2.4 µ m; B = 1.2 µ m; C = 1 µ m; D and E = 1 µ m
Discussion
In a search for novel active drugs as potential treatment options against echinococcosis, the in vitro activities of T. capitatus EO, of its major components, and of the 7 EO fractions F1–F7 obtained after silica gel chromatography, were studied employing in vitro cultured E. multilocularis metacestodes and GL cell cultures. EO and fractions F2 and F4 emerged as the most promising agents, but neither of the respective main components contained in EO, F2 or F4 exhibited damaging impact on E. multilocularis metacestodes, indicating the anti-parasitic activities may be based on synergistic effects between different compounds rather than a single component.
Chemical analysis of T. capitatus EO revealed that carvacrol is the major component of EO, which is well in accordance with the literature on the same species collected from other parts of the Mediterranean region (Dzamic et al., Reference Dzamic, Nikolic, Giweli, Mitic-Culafic, Sokovic, Ristic, Knezevic-Vukcevic and Marin2015; Saija et al., Reference Saija, Speciale, Trombetta, Leto, Tuttolomondo, La Bella, Licata, Virga, Bonsangue, Gennaro, Napoli, Siracusa and Ruberto2016). Carvacrol is also the dominant component in F3, F4 and F5. Thymol represents 0.3% of the total EO. Since two chemotypes, namely carvacrol and thymol types, have been recognized in T. capitatus (Boubaker Elandaogylousi et al., Reference Boubaker Elandaogylousi, Akkari, B'Chir, Gharbi, Mhadhbi, Awadi and Darghouth2013; Maissa and Walid, Reference Maissa and Walid2015; Saoudi et al., Reference Saoudi, Sifaoui, Chammem, Reyes-Batlle, Lopez-Arencibia, Pacheco-Fernandez, Pino, Hamdi, Jimenez, Bazzocchi, Pinero and Lorenzo-Morales2017), we can confirm here that the investigated T. capitatus EO is a carvacrol chemotype. This is in accordance with previous studies on T. capitatus from Tunisia (Bounatirou et al., Reference Bounatirou, Smiti, Miguel, Faleiro, Rejeb, Neffati, Costa, Figueiredo, Barroso and Pedro2007; Maissa and Walid, Reference Maissa and Walid2015). Karousou et al. (Reference Karousou, Koureas and Kokkini2005) reported that the high carvacrol content in T. capitatus was associated with dry dwarf-shrub formations of the lowland. Also, this is in line with the characteristics of our harvest location. We have not investigated whether the drying process of T. capitatus leaves would alter the chemical profile.
Initially, we employed the PGI-screening assay, which allows the quantitative testing of compounds that impact the physical integrity of E. multilocularis metacestodes (Stadelmann et al., Reference Stadelmann, Scholl, Muller and Hemphill2010). PGI is a prominent enzyme in the metacestode VF, which is released into the culture supernatant upon distortion of the physical integrity of the metacestode.
Due to the interference of EO components in the enzymatic reaction of the PGI-screening assays, we employed the Alamar Blue test to analyze the parasiticidal potential E. multilocularis metacestodes (Stadelmann et al., Reference Stadelmann, Rufener, Aeschbacher, Spiliotis, Gottstein and Hemphill2016). Parasiticidal activity is crucial for a candidate compound to be developed for the treatment of AE, because surviving stem cells could otherwise be able to initiate the re-growth of metacestodes upon discontinuation of treatment – as it is the case for ABZ, the standard drug used for treatment (Brehm and Koziol, Reference Brehm and Koziol2014; Stadelmann et al., Reference Stadelmann, Rufener, Aeschbacher, Spiliotis, Gottstein and Hemphill2016). The Alamar Blue assay demonstrated that EO and a pool of F1–F7 impaired the viability of E. multilocularis metacestodes to the same extent, also confirming the suitability and quality of silica gel chromatography for the fractionation of EO. Further dilution series revealed that EO exhibited a very strong parasiticidal activity up to a dilution of 1:5000. By the inclusion of CO in all tests, we could also confirm that the observed activity was the result of the composition of T. capitatus EO itself and not of a simple physical contact between parasite and oily components. Some of the fractions obtained by silica gel chromatography exhibited lower anti-parasitic activity. At a dilution of 1:1000, F1, F6 and F7 led to <20% reduction of parasite viability, and therefore these fractions were not further considered in subsequent assays. EO, F2, F3, F4 and F5 were further assessed at higher dilutions. At a dilution of 1:8000, EO, F2, F3 and F4 still retained their anti-parasitic activities, and this correlated well with morphological alterations observed by microscopy.
Cytotoxicity measurements in RH and HFF were carried out to determine a potential therapeutic window and showed that EO and F3 were as toxic for mammalian cells as they were for the parasite. However, F2 and F4 exhibited more specific toxicity for metacestodes compared to RH and HFF. The cytotoxic effects of EO fractions could be attributed to lipophilic properties, which could cause their accumulation in the cell membranes, leading to increased permeability, leakage of enzymes and metabolites, and finally to cell death (Sertel et al., Reference Sertel, Eichhorn, Plinkert and Efferth2011). No previous studies investigating the anti-infective effects of T. capitatus extracts have assessed the toxicity to mammalian cells.
The major components of EO and EO fractions such as limonene, eugenol, β-caryophyllene, carvacrol and thymol identified therein were also studied with respect to anti-metacestode activities. In the PGI-assay, limonene and eugenol exhibited some activities only at very high concentrations of 100 µ m, while carvacrol and thymol, when added at 100 µ m, had only marginal effects. The low activities of these compounds were confirmed by Alamar Blue vesicle viability assay. EO and F3 were active in isolated GL cell cultures at a 1:16 000 dilution, by reducing cell viability to 19.55 and 49.52%. This dilution corresponded to a carvacrol concentration of 301.69 µ m and 349.23 µ m. However, pure carvacrol, added to GL cells at 300 µ m, did not reduce cell viability. This indicates that the observed activity of EO and respective fractions against metacestodes and GL cells of E. multilocularis resulted from synergistic effects caused by multiple compounds contained in these EO fractions, or alternatively the effects could be mediated by a minor, but nonetheless very powerful compound not yet identified in these EO samples. It is important to keep in mind that differences in the activity of fractions and compounds on primary cells vs whole vesicles could mainly be due to effects on the tegument, which is one of the most important structures for vesicle integrity. In contrast to our findings. Fabbri et al. reported profound impact of carvacrol used at 10 µg mL−1 (which corresponds to 66.57 µ m) on the GL of E. granulosus hydatid cysts, and reported that in vivo treatment with carvacrol resulted in a reduction of parasite weight in E. granulosus infected mice (Fabbri et al., Reference Fabbri, Maggiore, Pensel, Denegri, Gende and Elissondo2016).
The plant species T. capitatus was previously described to contain components that are active against other parasites such as Haemonchus contortus (Boubaker Elandaogylousi et al., Reference Boubaker Elandaogylousi, Akkari, B'Chir, Gharbi, Mhadhbi, Awadi and Darghouth2013), Giardia lamblia (Machado et al., Reference Machado, Dinis, Salgueiro, Cavaleiro, Custodio and Sousa Mdo2010a, Reference Machado, Sousa Mdo, Salgueiro and Cavaleiro2010b) and promastigotes of Leishmania infantum, Leishmania tropica and Leishmania major (Machado et al., Reference Machado, Dinis, Santos-Rosa, Alves, Salgueiro, Cavaleiro and Sousa2014). All these studies had attributed the anti-parasitic activity of T. capitatus to the entire extracted preparation, i.e. EO (Machado et al., Reference Machado, Dinis, Salgueiro, Cavaleiro, Custodio and Sousa Mdo2010a, Reference Machado, Sousa Mdo, Salgueiro and Cavaleiro2010b, Reference Machado, Dinis, Santos-Rosa, Alves, Salgueiro, Cavaleiro and Sousa2014) or crude extracts (Boubaker Elandaogylousi et al., Reference Boubaker Elandaogylousi, Akkari, B'Chir, Gharbi, Mhadhbi, Awadi and Darghouth2013). To our knowledge, this is the first report on studying simultaneously the effects of EO of T. capitatus as well as defined EO fractions on E. multilocularis in vitro. Moreover, previous preparations were based on aqueous or alcoholic extracts, and they were thus exclusively composed of polar molecules (Boubaker Elandaogylousi et al., Reference Boubaker Elandaogylousi, Akkari, B'Chir, Gharbi, Mhadhbi, Awadi and Darghouth2013). The extraction method described here, based on hydro-distillation for preparation of EO, includes small hydrophobic molecules (<300 Da), most of which satisfy the theoretical criteria for prediction of drug-likeness (Lipinski et al., Reference Lipinski, Lombardo, Dominy and Feeney2001). These small molecules easily diffuse across cell membranes and are able to interact with intracellular targets (Edris, Reference Edris2007).
Taken together, our findings suggest F2 and F4 as the most promising fractions of the studied T. capitatus EO. These fractions were active against E. multilocularis metacestodes and GL cells in vitro, and were less toxic for RH and HFF cell cultures in vitro. As the main components of these fractions did not display in vitro activity, it is conceivable to conclude that the anti-metacestode activity is due to synergistic effects of various major and minor components of these EO fractions. Our results justify future investigations to validate and improve the use of the more active F4 in particular as a potential therapeutic alternative for AE treatment. Therefore, further molecular work and drug association studies are needed to identify its precise content, as well as to identify potential drug targets.
Supplementary material
The supplementary material for this article can be found at https://doi.org/10.1017/S0031182019000295.
Author ORCIDs
Britta Lundström-Stadelmann, 0000-0003-2672-5766; Selim M'rad, 0000-0003-1298-3289; Andrew Hemphill 0000-0002-0622-2128.
Acknowledgements
Many thanks to Reto Rufener and Dominic Ritler for many helpful pieces of advice and technical support.
Financial support
This work was supported by the Tunisian Ministry of Higher Education and Scientific Research, the European Cooperation in Science and Technology (COST, CM1307), the Foundation Sana, Switzerland, and the Swiss National Science Foundation (SNSF, grant numbers 160108 and 179439). Amani Hizem was supported by a Swiss Government Excellence Fellowship and the University of Bern.
Conflicts of interest
None.
Ethical standards
For initiation of in vitro cultures of E. multilocularis metacestodes, all manipulations with animals followed the guidelines of the Swiss legislation on experimental animal procedures and the experiments were approved by the Bernese cantonal authorities under the license number BE112/14.