Overview and life cycle
Neuroangiostrongyliasis (NAS), infection with the rat lungworm, Angiostrongylus cantonensis, is the leading cause of human eosinophilic meningoencephalitis globally. For various reasons, its geographical range continues extending, from a presumed origin in Southeast Asia. A wide range of other vertebrate accidental hosts can also be affected, including non-human primates, dogs, fruit bats, horses, marsupials and some species of birds. The definitive hosts include several species of rats, in whose pulmonary vasculature the adult worms reside (reviewed in Barratt et al., Reference Barratt, Chan, Sandaradura, Malik, Speilman, Lee, Marriott, Harkness, Ellis and Stark2016). Each female worm releases thousands of eggs daily, which lodge to embryonate in alveolar capillaries (Fig. 1). First-stage larvae (L1s) hatch and escape via the airways, then passing through the gut to the outside world. To infect the next definitive host, these must develop to the third stage (L3) (Fig. 2) which can occur only within the tissues of intermediate hosts, a wide range of slug and snail species (Fig. 3). These gastropod molluscs usually acquire L1s by ingesting rat feces, although some might also be invaded percutaneously. The life cycle is completed when a rat eats the infected gastropod; L3s are released in the gut, then develop through the L4 and L5 stages in the central nervous system (CNS), before returning to the right ventricle and pulmonary arteries, where they mature and copulate, potentially surviving for the life of the host.
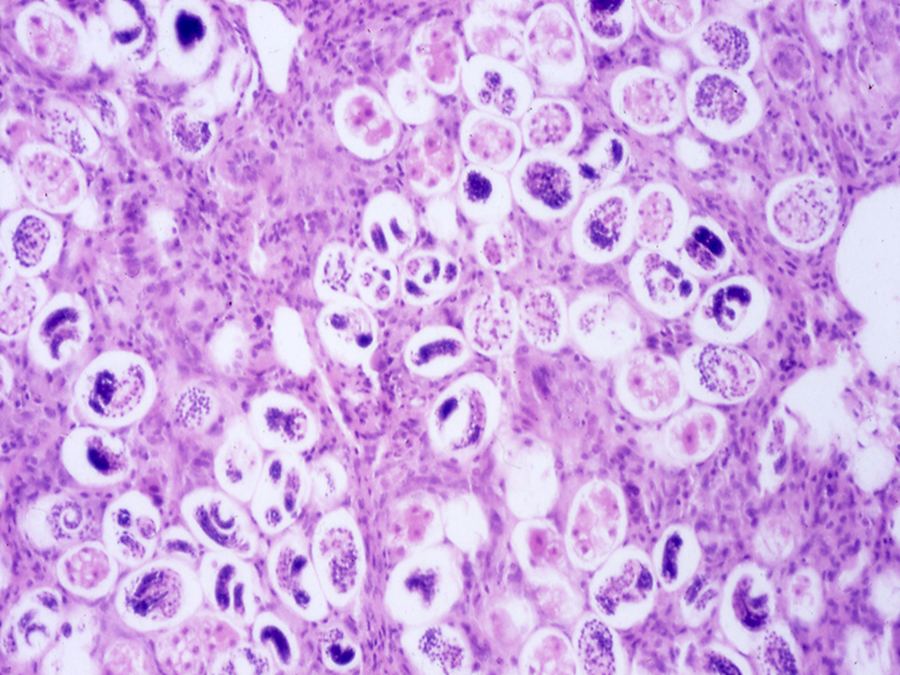
Fig. 1. High-power (40×) view of H&E stained section of heavily infected rat lung, showing numerous A. cantonensis eggs at different stages of embryonation, within capillaries of grossly-thickened inter-alveolar septa, encroaching on air spaces (worm eggs in lung section 70–80 μ m long).
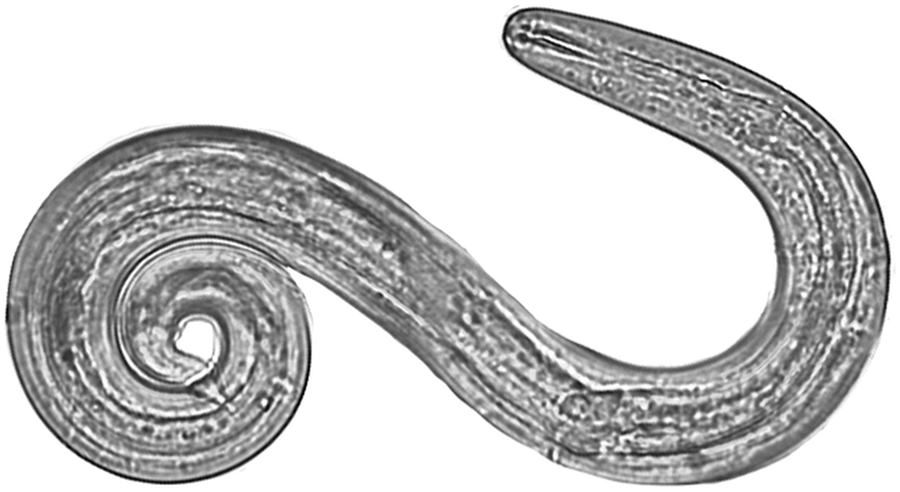
Fig. 2. Angiostrongylus cantonensis L3 isolated from a Parmarion martensi collected on East Hawai`i Island (40×, Photo by J. Jacob).

Fig. 3. Histological section showing an A. cantonensis larva very close to the outside edge of the body wall of a Parmarion martensi (10×, Photo by K. Howe).
Transmission
Most human infections are derived from gastropods; a less significant source is provided by various paratenic hosts (such as freshwater shrimps, frogs, centipedes, or monitor lizards), which can harbour L3s after ingesting gastropods and so reflect geographical/cultural idiosyncrasies. Another likely vehicle of infection is drinking water contaminated with L3s released by decomposing molluscs (Howe et al., Reference Howe, Kaluna, Lozano, Torres Fischer, Tagami, McHugh and Jarvi2019). The role of gastropod mucus deposited on vegetable greens remains inconclusive. In established endemic areas where domestic cultivation and consumption of snails are routinely practised, such as parts of Southeast Asia, NAS can assume epidemic proportions. Elsewhere, it tends to occur sporadically, albeit with a seasonal influence possibly reflecting gastropod behaviour; excepting the odd case of wilful slug/snail ingestion, the precise source of most infections is rarely identifiable. Seeing that rats and gastropods comprise integral components of most ecosystems, their elimination, in an attempt to eradicate a disease that is not generally viewed as a major public health threat, would be very difficult to accomplish, both in environmental and financial terms. However, in well-delineated locations, such as islands where the host species are not endemic, but introduced (e.g. Hawai`i), then control of rat and snail populations would be justified on ecological grounds and feasible; if effective, it would certainly diminish or eliminate the risk of human infection. Individual protection is essential for prevention, for which public education is critical. Care to avoid the ingestion of slugs and snails cannot be over-emphasized; elimination of viable, free L3s from fresh, green foodstuffs remains a challenge, although their relative importance is yet to be confirmed. Of course, thoroughly cooking all food and boiling all drinking water will kill the L3s (Alicata, Reference Alicata1967; Steel et al., Reference Steel, Jacob, Klasner, Howe, Jacquier, Pitt, Hollingsworth and Jarvi2021) assuring better protection.
Clinical manifestations
Following exposure, early symptoms will depend on the means of entry, e.g. L3s free in drinking water (or perhaps gastropod mucus on lettuce leaves?), or deeply bound within a live slug. This will affect the precise anatomical locations of invasion and initial inflammation, while the larval numbers will affect the intensity of pathological responses and ultimate tissue damage. In traditionally endemic areas, where reinfection is probably common, partial immunity might affect the clinical picture; as with nematode infections generally (Quinnell et al., Reference Quinnell, Pritchard, Raiko, Brown and Shaw2004), previous exposure does not produce sterilizing immunity, but it may retard worm migration, development, survival and establishment, and possibly the intensity of host inflammatory responses (Prociv and Turner, Reference Prociv and Turner2018).
Human disease is initiated by ingested L3s invading the gastrointestinal mucosae; symptoms (pain, nausea, vomiting, diarrhoea) can commence within hours. Skin rashes of uncertain aetiology can appear within days; they might result from allergy to the food and/or L3s, or disruption of cutaneous capillaries or nerves by L3s. Most reach the CNS via the circulation, although variable numbers might use alternative routes, such as along peripheral nerves and/or their associated lymphatics and blood vessels (this remains unclarified). In experimental studies, L3s are usually administered in saline, but in natural infections, an ingested gastropod might not disintegrate until well down the gut, even as far as the colon, perhaps enabling L3s to invade pelvic lymphatics and/or nerves, as a gateway into the lower spinal cord. Symptoms of CNS invasion usually commence a week or more after exposure. Unlike viruses and bacteria, the parasite does not multiply in us, but does migrate extensively and erratically, while growing hugely in size over several weeks (to about 15 mm long when leaving the CNS). This triggers essentially multifocal, often transient but progressively worsening signs and symptoms, depending very much on larval numbers and the sites they disrupt (Prociv and Turner, Reference Prociv and Turner2018).
From all this, it is not surprising that the clinical picture can be varied and unpredictable. Headache is an almost universal feature of NAS and is likely triggered by meningeal inflammation plus intracranial hypertension [elevated cerebrospinal fluid (CSF) pressure], the latter explaining the temporary relief often provided by lumbar puncture. Tissue damage, resulting from a combination of direct mechanical and chemical disruption with superimposed host ‘comet trail’ inflammation, increases with time. Many larvae do succumb to the host immuno-inflammatory response, stopping dead in their tracks, but this leads to the formation of dense, fixed inflammatory foci. The more advanced the larva, the bigger the lesion, and the longer it will take to resolve; it is very likely that the remains of an immature adult worm (L5) could persist in the CNS for months or possibly years, with surrounding chronic inflammation causing the deposition of permanent scar tissue (gliosis). However, variable numbers of L5s will survive long enough to leave the CNS via cerebral and spinal veins, and reach the lungs. Significant clinical lung disease can occur when large numbers of adult worms develop in the human pulmonary arteries (Figs 4 and 5) (Prociv and Turner, Reference Prociv and Turner2018). In several autopsies of acute cases, evidence of adult worm copulation has been reported; it would be interesting to determine if their offspring made it to the outside world, in the form of L1s in human feces. This would require careful microscopic examination of stools from large numbers of recovering patients.

Fig. 4. Formalin-fixed lobe of lung from a 1-year-old boy with a heavy infection exhibiting numerous thrombi, extensive congestion and peripheral hemorrhagic infarction (10×). Republished with permission from Prociv and Turner, (Reference Prociv and Turner2018) (Fig. 6).

Fig. 5. High power microscopic view of the pulmonary section (H&E stained) from a 1-year-old boy with a heavy infection showing worm within the thrombosed pulmonary artery, surrounded by extensive congestion and inflammation (40×). Republished with permission from Prociv and Turner (Reference Prociv and Turner2018) (Fig. 7).
Diagnosis and treatment
What, then, are the implications for diagnosis and treatment? To minimize CNS damage from ongoing infection, while the L3s are still small (ca. 0.3 mm long at invasion), treatment should be started as soon as the diagnosis is considered (Prociv and Turner, Reference Prociv and Turner2018). While the efficacy of some anthelminthics is unknown, albendazole appears very effective in rapidly killing these migratory larvae in the CNS and should be given immediately. Indeed, should a child or adult be known to have ingested a potentially infected snail or slug, then pre-emptive therapy is mandatory to prevent L3s from even reaching the CNS. In such an emergency, were albendazole not immediately available, mebendazole or pyrantel pamoate (over-the-counter pinworm medicine) (Jacob et al., Reference Jacob, Tan, Lange, Saeed, Date and Jarvi2021) could be given immediately, although in vivo pyrantel pamoate trials for A. cantonensis have not yet been conducted. Given their anthelminthic actions only within the gut lumen, these drugs will have no effect on L3s that have already invaded deeper tissues, so should be followed up by albendazole treatment as soon as practicable. Corticosteroids, by non-specifically dampening CNS inflammation, should be administered concomitantly with albendazole, but judiciously, to alleviate CNS symptoms, signs and severity of potential damage. Approximately after 3 weeks post-exposure, most of the worms are expected to have died or grown larger and are preparing to leave the CNS. At this point, killing remaining viable L5s using anthelmintics, may potentially contribute to the formation of additional CNS lesions (Prociv and Turner, Reference Prociv and Turner2018). This has been confirmed by acute magnetic resonance imaging changes in cases observed by one author (Prociv P unpublished data). The serious obstacle to this approach is precisely timing the stage of infection, which is only rarely possible. Few patients can identify their point of exposure, even with careful interrogation, providing only vague clues to when the larvae might have been taken on board (Jarvi et al., Reference Jarvi, Eamsobhana, Quarta, Howe, Jacquier, Hanlon, Snook, McHugh, Tman, Miyamura, Kramer and Meyers2020). Larval numbers can be guessed only in retrospect, from clinical consequences.
The other major challenge for clinicians is that a reliable diagnosis, either in the form of seroconversion or detection of parasite DNA [by polymerase chain reaction (PCR)] in CSF or blood, can take several weeks. A recently developed ultra-sensitive PCR might prove helpful (Sears et al., Reference Sears, Qvarnstrom, Dahlstrom, Snook, Kaluna, Balaz, Feckova, Slapeta, Modry, Jarvi and Nutmanin press). Even the onset of eosinophilia in blood or CSF can be delayed, although it must be stressed that normal CSF should be absolutely free of eosinophils and must be stained accordingly for examination; thus, there is no ‘normal cut-off number’, so detection of just one in a blood-free sample indicates pathology (Graeff-Teixeira et al., Reference Graeff-Teixeira, Aramburu da Silva and Yoshimura2009). As for neuro-imaging studies, the latest-generation MR scanners (or perhaps positron emission tomography scanners) might provide adequate resolution to identify larvae migrating in the CNS, but this would be a painstaking (and expensive) procedure. Of course, an identifiable larva in the CSF (or an eye) offers conclusive evidence, but is rarely encountered outside those highly endemic areas where repeated, low-grade exposure perhaps affects population immunity and hence worm behaviour. Measuring the size of any recovered larva is essential, for it allows a reasonable estimation of the stage of infection.
So, given the risk of extending potentially irreversible CNS damage while awaiting diagnostic confirmation, anthelminthic treatment should be given purely on clinical suspicion: if the presentation is consistent with early NAS, in the appropriate epidemiological setting, it should be started immediately. It must be noted that early, severe NAS, especially in young children, but sometimes adults as well, can resemble the more common Guillain-Barré syndrome, which has no specific treatment, but a better prognosis (Prociv and Turner, Reference Prociv and Turner2018).
Respiratory symptoms are a surprisingly common feature in late NAS and very likely to indicate lung involvement. These should not be disregarded but monitored clinically and radiologically. Anthelminthic therapy, however, is unlikely to prove beneficial, as dead worms will simply plug up the pulmonary arterial branch they occupy, without any resolution of the damage already caused.
Long-term sequelae
Despite even timely diagnosis and treatment, the nature of this disease will cause many patients to suffer prolonged, sometimes permanent, clinical sequelae. While some become convinced the parasite has survived, even multiplied, in their brain, the simple explanation is that their CNS lesions have been too extensive for complete resolution. They may be affected by irritating and debilitating symptoms indefinitely, with the only treatment available being non-specific, as offered by physiotherapy and pain clinics. It is perfectly understandable that some resort to ‘alternative’ therapies that remain scientifically unconfirmed, purely out of desperation, although rarely with satisfaction. These long-term sequelae often lead to great frustration, with the superimposed burdens of financial hardship, lack of insurance and strain on caregiving family and friends (or total lack of support). Given the paucity of detailed clinical follow-up in such chronic cases, let alone post-mortem studies, very little is known about the underlying pathology of late stage NAS (Meyer, Reference Meyer2021).
This Special Issue of Parasitology is based on cutting-edge research presented at the 6th International Workshop on Angiostrongylus and Angiostrongyliasis held in Hilo, Hawai`i, in January 2020. It provides research updates on gastropod and rat infection levels under natural conditions in multiple geographic areas (Niebuhr et al., Reference Niebuhr, Siers, Leinbach, Kaluna and Jarvi2021; Estano et al., Reference Estano, Bordado and Paller2021; Walden et al., Reference Walden, Slapinski, Rosenburg and Wellehan2021), under laboratory conditions (Hamilton et al., Reference Hamilton, Tagami, Kaluna, Jacob, Jarvi and Follett2021; Wun et al., Reference Wun, Davies, Speilman, Rogan and Malik2021a, Reference Wun, Malik, Yu, Chow, Lau, Podadera, Webster, Rogan, Slapeta and Daviesb), as well as alternative parasite transmission pathways (Modry et al., Reference Modry, Feckova, Putnova, Manalo and Otranto2021). The latest research on infection in non-human species is described (Feckova et al., Reference Feckova, Djoehana, Putnova, Petrikova, Knotek and Modry2021; Malik et al., Reference Malik, Rogan, Pai, Churcher, Davies, Braddock, Linton, Yu, Bell, Wimpole, Dengate, Collins, Brown, Rappas, Jaensch, Wun, Martin and Slapeta2021; Tsai and Chen, Reference Tsai and Chen2021; Wun et al., Reference Wun, Davies, Speilman, Rogan and Malik2021a, Reference Wun, Malik, Yu, Chow, Lau, Podadera, Webster, Rogan, Slapeta and Daviesb). Methods for preventing infection or reducing risks of human exposure (Howe et al., Reference Howe, Bernal, Brewer, Millikan and Jarvi2021; Steel et al., Reference Steel, Jacob, Klasner, Howe, Jacquier, Pitt, Hollingsworth and Jarvi2021), documentation of chronic sequelae (Meyer, Reference Meyer2021) and updates on research in disease diagnosis and treatment (Ansdell et al., Reference Ansdell, Kramer, Godnell, Murphy, Bernard, Blalock, Yates, Lteif, Smith and Melish2021; Eamsobhana et al., Reference Eamsobhana, Yong, Prasartvit, Wanachiwanawin and Gan2021; Jacob et al., Reference Jacob, Tan, Lange, Saeed, Date and Jarvi2021; Jarvi et al., Reference Jarvi, Atkinson, Kaluna, Snook and Steel2021) are provided. We hope these research papers will provide clinicians, researchers, educators and the general public with information that might help to reduce the risk of infection in humans and other animals, develop more efficient and targeted diagnostic methods, rationalise therapeutic approaches in acute and chronic cases, and direct future research on this fascinating but potentially destructive disease.
Acknowledgements
We would like to thank all those who helped organize and make this meeting happen including Professor Gordon Ching, Rene Siracusa (posthumously), Dr Vernon Ansdell, Dr Karen Pellegrin, Eileen O'hara, Patricia Macomber, UHH undergraduate and pre-med students (especially Victoria Rapoza and Nicole ‘Pua’ Garza) and members of the Jarvi lab who helped in all aspects (Kay Howe, Dr John Jacob, Lisa Kaluna, Kirsten Snook, Dr Argon Steel, and Yaeko Tagami). We would like to thank Tanya Ibarra for assistance with graphics, and Sunny Walker for assistance with the website. We thank Hawai`i Island legislators Senator Russell Ruderman, Representative Dr. Richard Creagan and Representative Chris Todd, as well as Lt. Governor Dr Josh Green, and Senator Kai Kahele for their long-term support of our work through research and education to make the state of Hawai`i a safer place to visit and live. Mahalo!
Financial support
Hawai`i State Legislature, National Institutes of Health, National Institute of General Medical Sciences, IDeA Networks of Biomedical Research Excellence (P20GM103466), Gordon Ching and community members, Hawai`i County Research and Development, Hawai`i County Council, Hilo Medical Center, Hilo, HI, Malama O Puna, Pahoa, HI and Hawai`i Department of Health.