INTRODUCTION
IPTi is a promising intervention for reducing malaria and anaemia in young children in malaria endemic countries which can be rolled out within the World Health Organisation's (WHO) Expanded Programme of Immunisation (EPI). A treatment dose of sulphadoxine pyrimethamine (SP) is given at the time of routine vaccination of infants and studies have shown this reduces the incidence of clinical malaria in under-ones by about 30% (Aponte et al. Reference Aponte, Schellenberg, Egan, Breckenridge, Carneiro, Critchley, Danquah, Dodoo, Kobbe, Lell, May, Premji, Sanz, Sevene, Soulaymani-Becheikh, Winstanley, Adjei, Anemana, Chandramohan, Issifou, Mockenhaupt, Owusu-Agyei, Greenwood, Grobusch, Kremsner, Macete, Mshinda, Newman, Slutsker, Tanner, Alonso and Menendez2009). The intensification of SP resistance in many parts of Africa has led to concerns that the protective efficacy will be compromised. Since SP is the only drug tested for safety, efficacy and EPI interactions, no alternative drugs are currently available for use in IPTi.
Randomised controlled trials of IPTi using sulphadoxine pyrimethamine reported protective efficacies against clinical episodes of malaria ranging from 20% to 59% and against anaemia from 10% to 50% up to 12 months of age (Schellenberg et al. Reference Schellenberg, Menendez, Kahigwa, Aponte, Vidal, Tanner, Mshinda and Alonso2001, Reference Schellenberg, Menendez, Aponte, Kahigwa, Tanner, Mshinda and Alonso2005; Chandramohan et al. Reference Chandramohan, Owusu-Agyei, Carneiro, Awine, Amponsa-Achiano, Mensah, Jaffar, Baiden, Hodgson, Binka and Greenwood2005; Macete et al. Reference Macete, Aide, Aponte, Sanz, Mandomando, Espasa, Sigauque, Dobano, Mabunda, Dgedge, Alonso and Menendez2006; Mockenhaupt et al. Reference Mockenhaupt, Reither, Zanger, Roepcke, Danquah, Saad, Ziniel, Dzisi, Frempong, Agana-Nsiire, Amoo-Sakyi, Otchwemah, Cramer, Anemana, Dietz and Bienzle2007; Grobusch et al. Reference Grobusch, Egan, Gosling and Newman2007a, Reference Grobusch, Lell, Schwarz, Gabor, Dornemann, Potschke, Oyakhirome, Kiessling, Necek, Langin, Klein Klouwenberg, Klopfer, Naumann, Altun, Agnandji, Goesch, Decker, Salazar, Supan, Kombila, Borchert, Koster, Pongratz, Adegnika, Glasenapp, Issifou and Kremsnerb; Kobbe et al. Reference Kobbe, Kreuzberg, Adjei, Thompson, Langefeld, Thompson, Abruquah, Kreuels, Ayim, Busch, Marks, Amoah, Opoku, Meyer, Adjei and May2007). The first study of IPTi with SP conducted in Ifakara Tanzania during 1999–2000 showed the highest protection against malaria (Schellenberg et al. Reference Schellenberg, Menendez, Kahigwa, Aponte, Vidal, Tanner, Mshinda and Alonso2001) but resistance has risen steeply in the following years (Malisa et al. Reference Malisa, Pearce, Abdulla, Mshinda, Kachur, Bloland and Roper2010) raising concerns about efficacy. A later trial in an area of exceptionally high SP resistance in Northern Tanzania reported no protective efficacy with SP-IPTi (Gosling et al. Reference Gosling, Gesase, Mosha, Carneiro, Hashim, Lemnge, Mosha, Greenwood and Chandramohan2009). To examine the relationship between the protective efficacy of SP-IPTi and resistance, an analysis of seven SP-IPTi trials was carried out (Griffin et al. Reference Griffin, Cairns, Ghani, Roper, Schellenberg, Carneiro, Newman, Grobusch, Greenwood, Chandramohan and Gosling2010). Two measures of SP resistance were used; contemporaneous data from six in vivo efficacy studies using SP and seven molecular studies reporting frequency of dhfr triple and dhps double mutations within 50 km of the trial sites. The results suggested that there was a reduction in the protective efficacy of SP-IPTi with increasing molecular markers of SP resistance.
SP resistance occurs via substitutions in the target enzymes dihydrofolate reductase (DHFR) and dihydropteroate synthase (DHPS) coded by point mutations in the dhfr and dhps genes (Cowman et al. Reference Cowman, Morry, Biggs, Cross and Foote1988; Peterson et al. Reference Peterson, Walliker and Wellems1988; Brooks et al. Reference Brooks, Wang, Read, Watkins, Sims and Hyde1994; Triglia and Cowman, Reference Triglia and Cowman1994). Mutant dhfr alleles are varied and code for a range of tolerance to pyrimethamine from intermediate to high, depending upon the number of mutations present. The triple mutant dhfr allele (N51I+C59R+S108N) is a significant contributor to SP treatment failure. It originated in Asia (Roper et al. Reference Roper, Pearce, Nair, Sharp, Nosten and Anderson2004) but now has a pan-African distribution dating back to the 1980s (Certain et al. Reference Certain, Briceno, Kiara, Nzila, Watkins and Sibley2008; Maiga et al. Reference Maiga, Djimde, Hubert, Renard, Aubouy, Kironde, Nsimba, Koram, Doumbo, Le Bras and Clain2007).
Sulphadoxine resistant dhps emerged during the 1990s, against this background of high level pyrimethamine resistance. In East Africa the appearance of a dhps double mutant A437G+K540E heralded the arrival of significant SP treatment failures in the region (Naidoo and Roper Reference Naidoo and Roper2010). The combination of the dhps double mutant A437G+K540E and the dhfr triple mutant were shown to be predictive of early SP treatment failure among malaria patients in Kenya, Uganda and Malawi (Omar et al. Reference Omar, Adagu and Warhurst2001; Staedke et al. Reference Staedke, Sendagire, Lamola, Kamya, Dorsey and Rosenthal2004; Kublin et al. Reference Kublin, Dzinjalamala, Kamwendo, Malkin, Cortese, Martino, Mukadam, Rogerson, Lescano, Molyneux, Winstanley, Chimpeni, Taylor and Plowe2002). In West and Central Africa the dhps K540E mutation is rare but the dhps A437G single mutant, combined with triple mutant dhfr was found to be associated with treatment failure in Gabon (Kun et al. Reference Kun, Lehman, Lell, Schmidt-Ott and Kremsner1999), Ghana (Mockenhaupt et al. Reference Mockenhaupt, Teun Bousema, Eggelte, Schreiber, Ehrhardt, Wassilew, Otchwemah, Sauerwein and Bienzle2005), the Gambia (Dunyo et al. Reference Dunyo, Ord, Hallett, Jawara, Walraven, Mesa, Coleman, Sowe, Alexander, Targett, Pinder and Sutherland2006) and Republic of Congo, Brazzaville (Ndounga et al. Reference Ndounga, Tahar, Basco, Casimiro, Malonga and Ntoumi2007). An exception is a study by Marks et al. (Reference Marks, Evans, Meyer, Browne, Flessner, Von Kalckreuth, Eggelte, Horstmann and May2005) in Ghana who reported no association of A437G with treatment outcome in vivo. Studies done in vitro indicate that the 437G single mutant confers a lesser degree of drug tolerance than the A437G and K540E combined (Brooks et al. Reference Brooks, Wang, Read, Watkins, Sims and Hyde1994; Triglia et al. Reference Triglia, Menting, Wilson and Cowman1997). Hence it is considered very likely that the consequences for IPTi efficacy are less severe.
The WHO Technical Consultation on Intermittent Preventive Treatment of Infants (WHO, 2009) took account of the differing sensitivity of single and double mutant forms of dhps, noting that 540E mutations are indicative of high level SP resistance. A prevalence of 50% of the dhps 540E mutation is the threshold recommended by WHO to determine whether or not SP-IPTi should be implemented.
In addition to the dhps 540E, there are two other mutations which are newly emerging in Africa which, when found in conjunction with the dhfr triple and dhps double mutants, indicate extremely high levels of parasite insensitivity to SP which abrogate SP efficacy for both IPTi and IPTp. These are the dhfr 164L mutation and the dhps 581G. Harrington et al. (Reference Harrington, Mutabingwa, Muehlenbachs, Sorensen, Bolla, Fried and Duffy2009) in a study in Muheza, Tanga region, Tanzania showed that the efficiency of IPTp was largely compromised when pregnant women were infected with parasites expressing the dhps- A437G+K540E+A581G haplotype. Another recent study at Hale Health Centre, situated 32 km north of Muheza, reported 55% prevalence of the same haplotype. The clinical and parasitological cure rate at day 28 was less than 20% in children aged 6–59 months treated for clinical malaria with SP (Gesase et al. Reference Gesase, Gosling, Hashim, Ord, Naidoo, Madebe, Mosha, Joho, Mandia, Mrema, Mapunda, Savael, Lemnge, Mosha, Greenwood, Roper and Chandramohan2009). The establishment of A437G+K540E+A581 G may explain the total lack of protective effect of SP for IPTi in the same area reported by Gosling et al. (Reference Gosling, Gesase, Mosha, Carneiro, Hashim, Lemnge, Mosha, Greenwood and Chandramohan2009).
Currently there is a need for standardized information about the dhps 540E, 581G and dhfr 164L mutations in African sites where implementation of SP-IPTi is being used or considered for use. The purpose of this review is to summarise current knowledge about the prevalence of the dhps 540E and 581G and the dhfr 164L markers in a form which is freely accessible and easily understood. We have created maps which are available in interactive form on the worldwide web. They have utility not only in guiding IPTi – SP policy but also in highlighting the gaps in surveillance coverage which need to be addressed.
MATERIALS AND METHODS
Data collection
We conducted online literature searches periodically during October 2005 to February 2011 using the Pubmed, African Journal Online and Bioline databases using the search terms ‘malaria’, ‘dhps’ and ‘dhfr’ (Naidoo and Roper, Reference Naidoo and Roper2010). We included published studies which had been conducted in any malarious African country excluding studies of malaria imported from Africa to non-African countries, animal studies, vaccine trials, prophylaxis studies, methodology studies and in vitro studies of resistance. There were no restrictions applied on the basis of malaria case detection, age, pregnancy status, transmission intensity or molecular method used to detect resistant mutations.
We reviewed the full text of suitable studies and extracted the prevalence of dhfr and dhps mutations. Data were first written on a proforma containing predesigned data fields, including geo-referenced study site and study year, and subsequently double entered into a data entry system to ensure quality control. We recorded the proportion of infections containing individual dhfr or dhps point mutations and where possible, the haplotypes of associated point mutations in each gene. The details of studies that were identified and included in our analyses are provided on our website (www.drugresistancemaps.org).
Data analysis
We analysed the prevalence of dhps K540E, A581G and dhfr I164L. Graphs showing 540E prevalence through time were generated in Intercooled Stata ver 9.2 with calculated 95% exact binomial confidence intervals for each study sample. Studies where the year of sampling was not reported were not included in the plots of prevalence through time. We generated graphs to illustrate the prevalence of dhps 540E over a 20 year period, from 1988 to 2009 in six geographical categories. Pearce et al. (Reference Pearce, Pota, Evehe, Ba El, Mombo-Ngoma, Malisa, Ord, Inojosa, Matondo, Diallo, Mbacham, Van Den Broek, Swarthout, Getachew, Dejene, Grobusch, Njie, Dunyo, Kweku, Owusu-Agyei, Chandramohan, Bonnet, Guthmann, Clarke, Barnes, Streat, Katokele, Uusiku, Agboghoroma, Elegba, Cisse, Ie, Giha, Kachur, Lynch, Rwakimari, Chanda, Hawela, Sharp, Naidoo and Roper2009) identified five geographical regions of mainland Africa consisting of neighbouring countries which share the same dhps resistance allele lineages. In this study, we added a sixth cluster consisting of island populations and these are listed in Table 1.
Table 1. Regional sub-division of African countries based on shared dhps resistance lineages (Pearce et al. Reference Pearce, Pota, Evehe, Ba El, Mombo-Ngoma, Malisa, Ord, Inojosa, Matondo, Diallo, Mbacham, Van Den Broek, Swarthout, Getachew, Dejene, Grobusch, Njie, Dunyo, Kweku, Owusu-Agyei, Chandramohan, Bonnet, Guthmann, Clarke, Barnes, Streat, Katokele, Uusiku, Agboghoroma, Elegba, Cisse, Ie, Giha, Kachur, Lynch, Rwakimari, Chanda, Hawela, Sharp, Naidoo and Roper2009) and including islands as a separate category
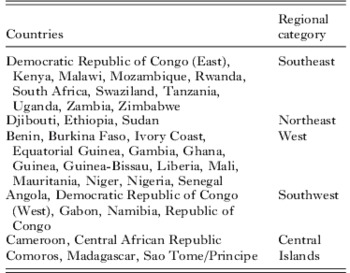
Vector maps were created in Mapinfo ver 9.0 to illustrate the spatial distribution of dhfr 164L and dhps 581G prevalence reports. All studies, including those whose study year was not stated were included in these maps. To illustrate the recent prevalence of 540E we selected only those studies where the samples were collected in 2004 or later and created a composite map showing sites where 540E was examined and highlighting those where prevalence exceeded the recommended threshold of 50%.
RESULTS
Prevalence of the dhps 540E mutation
We identified 119 publications describing 260 unique surveys of 540E prevalence which fulfilled the inclusion criteria and these are listed in full in the supplementary bibliography S1 (see http://journals.cambridge.org/PAR). The cumulative total number of isolates tested for 540E was 30 224, of which 10 339 (36%) tested positive with 540E. The regional distribution of surveys is summarized in Table 2. The most intensively studied area was the Southeast region where 129 surveys were carried out in 83 unique study sites in the 11 Southeast region countries listed in Table 1. The prevalence of 540E for successive surveys in each region is plotted with 95% exact binomial confidence intervals in Fig. 1. The 540E was common in surveys conducted throughout Southeast and Northeast Africa and its prevalence, which tended to increase over time, was seen to approach 100% in some areas. In Northeast Africa 27 surveys were conducted in 13 unique study sites in three countries and a prevalence of 100% 540E was reported at one site in 2004.

Fig. 1. Surveys of 540E prevalence (with 95% confidence intervals) conducted during 1988–2008 and displayed according to their geographic region.
Table 2. Prevalence of dhps K540E, dhps A581G and dhfr I164L

In the West, Central, Southwest and Island regions, the prevalence of 540E never exceeded 50%. In the West we recorded 45 surveys in 35 unique study sites within 14 countries. Among these the majority of surveys reported the 540E mutation was rare or absent, An exception was a reported prevalence of 23% in Ibadan, Nigeria. In the five countries of the Southwest region, there were 21 surveys in 16 study sites and the prevalence of 540E was universally less than 50%. Within the Central region 21 surveys from 11 different study sites all reported 540E prevalences of under 6%. Similarly the 17 surveys at 12 sites among island populations reported low prevalences of 540E and the maximum value recorded was 20% in 2004 in Sao Tome and Principe.
Prevalence of the dhfr 164L mutation
All publications in which surveys of dhfr 164L were carried out are listed in the supplementary bibliography S2 (see http://journals.cambridge.org/PAR). Of 19 597 isolates tested for 164L in 183 surveys and 114 unique sites, 164L was detected in just 130 isolates (0·7%). The regional distribution of the surveys and mutant positive isolates are shown in Table 2. In Fig. 2 a map illustrating the prevalence of 164L shows all the surveys which examined codon 164 in African parasites. Detection rates for the 164L mutation ranged from 0·6% to 13·7% of isolates tested. The 164L mutation was found in the Central African Republic, Comoros, Kenya, Madagascar, Malawi, Rwanda and Uganda but was absent in 21 other countries where surveys were conducted.

Fig 2. Map of dhfr 164L survey sites. Countries where surveys were conducted are shaded and the survey sites are indicated by open circles. For positive surveys the prevalence of 164L is indicated.
Prevalence of the dhps 581G mutation
All publications in which surveys of dhps 581G were carried out are listed in the supplementary bibliography S3 (see http://journals.cambridge.org/PAR). Of 15 331 isolates tested for 581G in Africa, the mutation occurred in 782 (5%) isolates in 13 countries namely Cameroon, Democratic Republic of Congo, Ethiopia, Ghana, Kenya, Madagascar, Malawi, Mali, Niger, Rwanda, Sudan, Tanzania and Uganda. The 581G was reported in all the regions except for the Southwest (Table 2). The survey sites and prevalence measurements are recorded in the map in Fig. 3 which shows 14 other countries where surveys were negative. The majority of the surveys recorded 581G<50% although in one study carried out prior to 1997 in Sotuba, Mali the 581G mutation was reported to be 100%.

Fig 3. Map of dhps 581G survey sites. Countries where surveys were conducted are shaded and the survey sites are indicated by open circles. For positive surveys the prevalence of 581G is indicated.
Recent surveys of the dhps 540E resistance marker and their application for SP-IPTi
To focus on the current situation, a subset of recent data was selected using only the surveys of dhps 540 carried out between 2004 and 2009. In all, 90 recent surveys were identified in which 4243 (35%) of 12 167 samples were positive for the 540E mutation. The regional distribution of these surveys and the proportion which reported prevalences higher than the 50% cut-off are summarised in Table 2, while their geographical locations are shown in the map in Fig. 4. The Southeast and Northeast regions contained all the survey sites with prevalence of the 540E mutation >50%. Prevalence exceeded 50% in eight countries namely Ethiopia, Kenya, Uganda, Rwanda, Tanzania, Malawi, Zambia and Mozambique. Within this subgroup 40 recent surveys were conducted of which 34 surveys recorded a prevalence of 50% or more.Exceptions being four studies in Maputo, Mozambique (2004 6% and 11%, 2005 16%. 2006 49%) and 2 studies in Zambia (Macha 2006 11%, Mpongwe 2004 46%). Within individual countries surveys recorded broadly consistent prevalences of the 540E mutation.
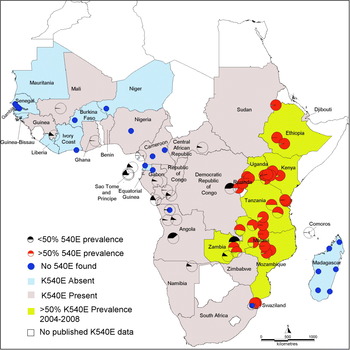
Fig 4. Map showing recent measures of the prevalence of 540E. All surveys conducted since 2004 are indicted by pie charts. The red pie charts indicate survey sites where prevalence exceeded 50% and these countries are shaded brown. The black pie charts indicate surveys with less than 50%. Blue circles indicate zero prevalence 540E. The countries shaded pink indicate that 540E has been detected at <50% at some stage whereas the blue ones are where it has never been detected. White indicates countries where no data was available.
In Ethiopia, three surveys were conducted in Jimma, Dilla and Humera all in 2004 and these reported consistently high 540E prevalences of 97%, 97% and 100% respectively (Gebru-Woldearegai et al. Reference Gebru-Woldearegai, Hailu, Grobusch and Kun2005; Schunk et al. Reference Schunk, Kumma, Miranda, Osman, Roewer, Alano, Loscher, Bienzle and Mockenhaupt2006; Pearce et al. Reference Pearce, Pota, Evehe, Ba El, Mombo-Ngoma, Malisa, Ord, Inojosa, Matondo, Diallo, Mbacham, Van Den Broek, Swarthout, Getachew, Dejene, Grobusch, Njie, Dunyo, Kweku, Owusu-Agyei, Chandramohan, Bonnet, Guthmann, Clarke, Barnes, Streat, Katokele, Uusiku, Agboghoroma, Elegba, Cisse, Ie, Giha, Kachur, Lynch, Rwakimari, Chanda, Hawela, Sharp, Naidoo and Roper2009). The sites cover a wide area – Jimma and Dilla are approximately 220 km apart and Humera is 900 km from Dilla. Although there have been no surveys published subsequently,and no surveys detected dhfr 164L the extreme prevalence of the 540E in 2004 and early reports of low level 581G (<2%) indicate that SP-IPTi is likely to be compromised by drug resistance in Ethiopia.
In Kenya, nine surveys were carried out in nine unique sites between 2004 and 2006. The dhps K540E prevalence was always greater than 50% and ranged from 74% in Iguhu, Kakamega District to 99% in Kombewa, (these sites are about 12 km apart) (Bonizzoni et al. Reference Bonizzoni, Afrane, Baliraine, Amenya, Githeko and Yan2009; Zhong et al. Reference Zhong, Afrane, Githeko, Cui, Menge and Yan2008). There are reports of dhfr 164L at prevalences around 3% in western Kenya suggesting that a very highly SP resistant form might be emerging here (McCollum et al. Reference McCollum, Poe, Hamel, Huber, Zhou, Shi, Ouma, Vulule, Bloland, Slutsker, Barnwell, Udhayakumar and Escalante2006).
In Uganda, 540E prevalence has exceeded 50% since 1999 (Staedke et al. Reference Staedke, Sendagire, Lamola, Kamya, Dorsey and Rosenthal2004). Surveys conducted during 2002–2004 in six national surveillance sites recorded prevalences of between 80% (in Mubende) and 98% (in Tororo) (Francis et al. Reference Francis, Nsobya, Talisuna, Yeka, Kamya, Machekano, Dokomajilar, Rosenthal and Dorsey2006), and most recently dhps 540E prevalences of 98% and 100% were recorded in Rukungiri and Kabale in 2005 (Lynch et al. Reference Lynch, Pearce, Pota, Cox, Abeku, Rwakimari, Naidoo, Tibenderana and Roper2008). Overlaid upon dhps 540E there are emerging foci of dhps 581G (up to 45% prevalence) and dhfr 164L (up to 14% prevalence) in southwest Uganda recorded in 2005 (Francis et al. Reference Francis, Nsobya, Talisuna, Yeka, Kamya, Machekano, Dokomajilar, Rosenthal and Dorsey2006; Lynch et al. Reference Lynch, Pearce, Pota, Cox, Abeku, Rwakimari, Naidoo, Tibenderana and Roper2008) indicating that SP-IPTi will be severely compromised by resistance in this area.
In Rwanda, surveys at Rukara and Mashesha in 2005 and reported prevalences of 97% and 84% 540E respectively (Karema et al. Reference Karema, Imwong, Fanello, Stepniewska, Uwimana, Nakeesathit, Dondorp, Day and White2010). The same surveys revealed emergence of the dhfr 164L (12%) and dhps 581G (61%) at Rukara which is approximately 120 km from the highly resistant populations in southwest Uganda. At the more distant survey site at Mashesha (approximately 170 km from southwest Uganda) dhfr 164L was still absent but dhps 581 was 30%. The intensification of resistance in both sites predicts that SP efficacy will be exceptionally poor.
The collation of 540E data from seven recent surveys in five different sites in Tanzania indicated a range in prevalence from 50% in 2005 in Chamwino to 97% in 2007 in Korogwe District (Enevold et al. Reference Enevold, Nkya, Theisen, Vestergaard, Jensen, Staalsoe, Theander, Bygbjerg and Alifrangis2007; Alifrangis et al. Reference Alifrangis, Lusingu, Mmbando, Dalgaard, Vestergaard, Ishengoma, Khalil, Theander, Lemnge and Bygbjerg2009). In Korogwe, Alifrangis et al. (Reference Alifrangis, Lusingu, Mmbando, Dalgaard, Vestergaard, Ishengoma, Khalil, Theander, Lemnge and Bygbjerg2009) also reported dhps 581G at 12% but no dhfr 164L in surveys conducted between 2003 and 2007.
In Malawi, five surveys were conducted in 2005 and 2007 in four unique sites spanning the length of country. The prevalence of 540E reached extremes of 96%–100% (Bridges et al. Reference Bridges, Molyneux and Nkhoma2009; Nkhoma et al. Reference Nkhoma, Molyneux and Ward2007). Additionally in 2003, the prevalence of 581G was 3% and 164L was 4% in Blantyre (Alker et al. Reference Alker, Mwapasa, Purfield, Rogerson, Molyneux, Kamwendo, Tadesse, Chaluluka and Meshnick2005) although both these mutations remained absent in the other Malawian sites surveyed (Plowe et al. Reference Plowe, Cortese, Djimde, Nwanyanwu, Watkins, Winstanley, Estrada-Franco, Mollinedo, Avila, Cespedes, Carter and Doumbo1997; Bwijo et al. Reference Bwijo, Kaneko, Takechi, Zungu, Moriyama, Lum, Tsukahara, Mita, Takahashi, Bergqvist, Bjorkman and Kobayakawa2003; Bell et al. Reference Bell, Nyirongo, Mukaka, Zijlstra, Plowe, Molyneux, Ward and Winstanley2008; Bridges et al. Reference Bridges, Molyneux and Nkhoma2009; Ochong et al. Reference Ochong, Bell, Johnson, D'alessandro, Mulenga, Muangnoicharoen, Van Geertruyden, Winstanley, Bray, Ward and Owen2008).
The picture in Zambia is mixed. Surveys in six Zambian sites during 2004 recorded 540E prevalences of between 46% – 75% (Pearce et al. Reference Pearce, Pota, Evehe, Ba El, Mombo-Ngoma, Malisa, Ord, Inojosa, Matondo, Diallo, Mbacham, Van Den Broek, Swarthout, Getachew, Dejene, Grobusch, Njie, Dunyo, Kweku, Owusu-Agyei, Chandramohan, Bonnet, Guthmann, Clarke, Barnes, Streat, Katokele, Uusiku, Agboghoroma, Elegba, Cisse, Ie, Giha, Kachur, Lynch, Rwakimari, Chanda, Hawela, Sharp, Naidoo and Roper2009) with just one survey at Mpongwe falling below the 50% prevalence cut-off. However a survey in Macha in 2006 reported prevalence of 10% 540E (Mkulama et al. Reference Mkulama, Chishimba, Sikalima, Rouse, Thuma and Mharakurwa2008). No 164L was detected in Macha (Mkulama et al.2008) and there is no recent data on 581G. These surveys indicate limited efficacy of SP in Zambia although additional monitoring of SP resistance genes including 581G are required.
In Mozambique, annual surveys between 2004 and 2008 at sentinel sites across Maputo district recorded the rise in prevalence of 540E across that region (Raman et al. Reference Raman, Little, Roper, Kleinschmidt, Cassam, Maharaj and Barnes2010). Surveys in 2004 reported prevalences of 6% and 11%. In 2005 this increased to 16% and in 2006 to 49%. By 2008, prevalence exceeded 50% in all sentinel sites and the average prevalence across the region was 65%. No dhfr 164L or dhps 581G was found in Maputo during 2004–2008 (Raman et al. Reference Raman, Little, Roper, Kleinschmidt, Cassam, Maharaj and Barnes2010) and other studies found no 581G in other sites in Mozambique (Fernandes et al. Reference Fernandes, Figueiredo, Do Rosario and Cravo2007; Raman et al. Reference Raman, Sharp, Kleinschmidt, Roper, Streat, Kelly and Barnes2008; Enosse et al. Reference Enosse, Magnussen, Abacassamo, Gomez-Olive, Ronn, Thompson and Alifrangis2008). The resistant genotype is well established and surveillance at multiple sites confirms this to be highly consistent across a large geographical area.
In Madagascar, the dhps 540E was absent in 2006 (Andriantsoanirina et al. Reference Andriantsoanirina, Ratsimbasoa, Bouchier, Jahevitra, Rabearimanana, Radrianjafy, Andrianaranjaka, Randriantsoa, Rason, Tichit, Rabarijaona, Mercereau-Puijalon, Durand and Menard2009) but an emerging focus of 164L mutant alleles was reported in the south of the country in annual surveys conducted in 2006, 2007 and 2008. Uniquely in Africa, the Malagasy 164L mutation is found as a single mutant and not in combination with mutations at codons 51, 59 or 108. In vitro assays predict that the 164L on its own will not confer significant resistance to pyrimethamine (Lozovsky et al. Reference Lozovsky, Chookajorn, Brown, Imwong, Shaw, Kamchonwongpaisan, Neafsey, Weinreich and Hartl2009). Based on the criteria of the 50% 540E prevalence threshold there should be no impediment to implimentation of SP-IPTi in Madagascar. However, given the unique combination of resistance mutations and the indications of their evolution in isolation from the mainland, it is recommended that local assessments of SP efficacy be conducted.
In Mali, there were reports of dhps 581G, one from a survey in Bandiagara during 2000 (Thera et al. Reference Thera, Sehdev, Coulibaly, Traore, Garba, Cissoko, Kone, Guindo, Dicko, Beavogui, Djimde, Lyke, Diallo, Doumbo and Plowe2005) and another carried out prior to 1997 (Wang et al. Reference Wang, Lee, Bayoumi, Djimde, Doumbo, Swedberg, Dao, Mshinda, Tanner, Watkins, Sims and Hyde1997). The implications of this for efficacy of SP-IPTi are unknown and unfortunately recent surveys have not tested for 581G so the current situation with respect to this mutation in Mali is unknown. However the rarity of 540E in Mali was reconfirmed in recent large scale surveys (Tekete et al. Reference Tekete, Djimde, Beavogui, Maiga, Sagara, Fofana, Ouologuem, Dama, Kone, Dembele, Wele, Dicko and Doumbo2009, Djimde et al. Reference Djimde, Fofana, Sagara, Sidibe, Toure, Dembele, Dama, Ouologuem, Dicko and Doumbo2008, Dicko et al. Reference Dicko, Sagara, Djimde, Toure, Traore, Dama, Diallo, Barry, Dicko, Coulibaly, Rogier, De Sousa and Doumbo2010).
CONCLUSION
High level SP resistance, as indicated by the prevalence of the 540E mutation, is advanced in sites all across East Africa. In Ethiopia, Kenya, Uganda, Rwanda, Tanzania, Malawi, Zambia and Mozambique measures of the prevalence of 540E were consistently 50% or higher since 2004. In addition, there has been significant intensification of resistance in parts of Uganda and Rwanda through acquisition of dhps 581G and dhfr 164L mutations. In northern Tanzania there is a well established focus of dhps 581G although 164L is absent. IPTi with SP is not recommended in these areas.
Elsewhere in Africa the 540E is encountered less frequently. Of the 36 countries for which data exists 27 have been confirmed to have 540E at low prevalence, leaving 10 countries: Burkina Faso, Gambia, Guinea-Bissau, Ivory Coast, Liberia, Madagascar, Mauritania, Niger, Senegal and Equatorial Guinea where recent surveys have been carried out but the 540E has still not been recorded. Based on this, SP-IPTi , is still practicable throughout much of Africa.
It will be important to maintain surveillance for markers of SP resistance to monitor changes over the next few years. SP has been withdrawn as a treatment for clinical malaria and is now recommended solely for use in intermittent preventive treatment in pregnant women and infants With this policy change there is expected to be a significant reduction in drug pressure (Malisa et al. Reference Malisa, Pearce, Abdulla, Mshinda, Kachur, Bloland and Roper2010). The cumulative reports of 540E so far have shown rising prevalence in East Africa and a gradual spread westward (Naidoo and Roper, Reference Naidoo and Roper2010), it is likely these trends will slow or even discontinue now that SP drug pressure is reduced.
Surveillance in support of use of SP for IPTi or IPTp should be concentrated in areas where there is no data and those where resistance is currently borderline or heterogeneous. In the absence of local information the closest approximation is to look at resistance levels in sites in neighbouring states. For example, in Burundi it may be expected that high levels of SP resistance would occur because of measures made in neighbouring sites in Rwanda and Eastern Democratic Republic of Congo. In situations where resistance levels are borderline, such as the Democratic Republic of Congo and Sudan, it may be necessary to employ district level surveillance to inform IPTi policy at local administrative levels.
Supporting evidence of the region-wide circulation of drug resistance genotypes comes from molecular analysis of flanking microsatellite markers around dhps (Pearce et al. Reference Pearce, Pota, Evehe, Ba El, Mombo-Ngoma, Malisa, Ord, Inojosa, Matondo, Diallo, Mbacham, Van Den Broek, Swarthout, Getachew, Dejene, Grobusch, Njie, Dunyo, Kweku, Owusu-Agyei, Chandramohan, Bonnet, Guthmann, Clarke, Barnes, Streat, Katokele, Uusiku, Agboghoroma, Elegba, Cisse, Ie, Giha, Kachur, Lynch, Rwakimari, Chanda, Hawela, Sharp, Naidoo and Roper2009) This study of resistant dhps alleles sampled in 20 African mainland countries showed that parasite populations are regional and probably are linked through networks of human circulation. Once a resistance mutation is established at a site it can rapidly be disseminated to other populations in the same regional genepool. Circulation of infected people between the major regions and between mainland and island populations is less frequent compared with their movement within regions and consequently exchange of resistance genes is more stochastic. Rogier (Reference Rogier, Pradines, Bogreau, Koeck, Kamil and Mercereau-Puijalon2005) reported that the Djibouti-Ethiopian railway was suspected to be an effective route for propagating malaria parasites. Both these countries lie in the Northeast region and it is expected (but as yet untested) that the SP resistance alleles will be common to both countries.
The importance of parasite circulation to shared resistance alleles among regional populations is an implicit assumption underlying the WHO recommendations. The presence of the dhps 540E is indicative of shared phenotype. In Madagascar, a picture of evolution of SP resistance determinants which was independent of the mainland emerged and a 164L mutation was found in a novel haplotype not recorded in mainland Africa. This illustrates an important caution in the application of a simple threshold prevalence of the 540E mutation. On the mainland, it serves as a valuable approximation of resistance levels among populations which share common resistant alleles and in which efficacy in relation to those alleles is well understood. They are not informative in areas where background genetics of dhfr and dhps are significantly different from areas where efficacy has been evaluated. In such cases, local evaluations of SP-IPTi efficacy are required.
Alternative treatments such as artemisinin-based combination therapies have not yet been sufficiently tested for safety, efficacy and interactions with the EPI to be advocated as alternatives to SP for IPTi. So use of IPTi rests upon the continuing efficacy of SP. Our recommendations for ongoing molecular surveillance of SP resistance markers in support of SP-IPTi policy in Africa are three fold. First, it is necessary that surveillance is introduced to the neglected geographical areas and coverage increased in countries like the Democratic Republic of Congo which have borderline levels of 540E. Secondly, ongoing molecular surveillance of dhps 540E should be maintained and expanded in sites throughout West and Central Africa in support of the continuing use of SP for intermittent preventive treatment there. Thirdly, monitoring of molecular markers in East Africa should be used to record the effect of reduction in drug pressure on the prevalence of SP resistance mutations.
ACKNOWLEDGEMENTS
We thank the database section of the Malaria Research Programme, Medical Research Council for support rendered.
Financial Support
The development of the drug resistance database was supported by funding from the Gates Malaria Partnership. The development of the website http://www.drugresistancemaps.org/ipti/ was funded by the IPTi consortium. Travel costs for CR to attend the special symposium on paediatric parasitology were paid by the BSP.