The functional homology of neural regions in fish is well conserved in rodents, and their behavior exhibits sufficient complexity to enable translation to both rodents and humans (Khan & Echevarria, Reference Khan, Echevarria, Vonk, Weiss and Kuczaj2017). As such, biological traits that are similar between fish and mammals have been widely utilized in models of anxiety (Egan et al., Reference Egan, Bergner, Hart, Cachat, Canavello, Elegante and Kalueff2009) and stress neurobiology (Collier et al., Reference Collier, Kalueff, Echevarria and Kalueff2017; Song et al., Reference Song, Yang, Wang, Chen, Li, Liu and Kalueff2016, Reference Song, Liu, Zhang, Peng, Wang, Collier and Kalueff2018).
To avoid any confusion about the use of the term “stress” in this article, we provide the following brief definitions: The stress response is an organism’s adaptive reaction to restore homeostasis when encountering a threatening stimulus or event (a stressor) (Chrousos, Reference Chrousos2009), resulting in either adaptive or maladaptive consequences, known as “eustress” and “distress,” respectively (Koolhaas et al., Reference Koolhaas, Bartolomucci, Buwalda, de Boer, Flügge, Korte and Fuchs2011). This response can be modulated by cognitive appraisals, where the organism evaluates the significance of the stimulus based on stored information in memory (Cerqueira et al., Reference Cerqueira, Millot, Castanheira, Félix, Silva, Oliveira and Oliveira2017, Reference Cerqueira, Millot, Felix, Silva, Oliveira, Oliveira and Oliveira2020, Koolhas et al., Reference Koolhaas, Bartolomucci, Buwalda, de Boer, Flügge, Korte and Fuchs2011; as seen in fish). According to this perspective, the stress response involves adaptive assessments and subsequent adjustments, allowing animals to respond effectively to both predictable and unpredictable events – a process known as allostasis (McEwen & Wingfield, Reference McEwen and Wingfield2003; as reviewed in fish by Øverli & Sørensen, Reference Øverli and Sørensen2016). In fish as in other species (Faustino et al., Reference Faustino, Oliveira and Oliveira2015), such as rats (Rygula et al., Reference Rygula, Golebiowska, Kregiel, Kubik and Popik2015), dogs (Mendl et al., Reference Mendl, Brooks, Basse, Burman, Paul, Blackwell and Casey2010), lambs (Greiveldinger et al., Reference Greiveldinger, Veissier and Boissy2011), fowl (Zimmerman et al., Reference Zimmerman, Buijs, Bolhuis and Keeling2011), and bees (Bateson et al., Reference Bateson, Desire, Gartside and Wright2011), coping with stress and cognition are closely related processes. For instance, a fish’s appraisal of stimuli, rather than the intrinsic characteristic of the stimuli, can have significant effects on stress responses (Cerqueira et al., Reference Cerqueira, Millot, Felix, Silva, Oliveira, Oliveira and Oliveira2020, Reference Cerqueira, Millot, Silva, Félix, Castanheira, Rey and Oliveira2021) and related emotion-like (Cerqueira et al., Reference Cerqueira, Millot, Castanheira, Félix, Silva, Oliveira and Oliveira2017) or affective states.
This article explores “emotion-like” or “affective” states, encompassing descriptors with valence (indicating positivity or negativity, reward or aversion, pleasure or displeasure, among other attributes), intensity (low or high), and duration/persistence (Paul & Mendl, Reference Paul and Mendl2018). “Affective” is often used interchangeably with “emotion” or “mood” in animal literature (Kremer et al., Reference Kremer, Klein Holkenborg, Reimert, Bolhuis and Webb2020) across various species, including mammals (e.g., Mendl & Paul, Reference Mendl and Paul2020), birds (e.g., Košťál et al., Reference Košťál, Skalná and Pichová2020), fish (Buenhombre et al., Reference Buenhombre, Daza-Cardona, Sousa and Gouveia2021; Cerqueira et al., Reference Cerqueira, Millot, Castanheira, Félix, Silva, Oliveira and Oliveira2017), and invertebrates (Perry & Baciadonna, Reference Perry and Baciadonna2017). These states give rise to a multidimensional response that can be objectively assessed through physiological, neurological, behavioral, and cognitive indicators (Kremer et al., Reference Kremer, Klein Holkenborg, Reimert, Bolhuis and Webb2020).
Affective states can induce cognitive bias processes (CBP) (Mendl & Paul, Reference Mendl and Paul2020). (We provided a glossary of the terms related to CBP in Box 1 and their types in fish are further discussed in section two). Thus, CBP have been used to study the interplay between cognitive and emotional processes in various animal species, including fish (Buenhombre et al., Reference Buenhombre, Daza-Cardona, Sousa, Gouveia and Cajiao-Pachón2022; Espigares et al., Reference Espigares, Abad-Tortosa, Varela, Oliveira and Ferreira2021; Laubu et al., Reference Laubu, Louâpre and Dechaume-Moncharmont2019; Tan et al., Reference Tan, Handasyde, Rault and Mendl2020a). Emotion-like states also seem to affect sensitivity to reward shifts (SRS) (Burman et al., Reference Burman, Parker, Paul and Mendl2008), which is related to a CBP (Kremer et al., Reference Kremer, Klein Holkenborg, Reimert, Bolhuis and Webb2020). Two primary sources of variation in CBP have been studied: the living environment and personality traits (Kremer et al., Reference Kremer, Bus, Webb, Bokkers, Engel, van der Werf and van Reenen2021).
Box 1. Glossary
Brief definition, and a reference to further reading where appropriate.
Cognitive bias processes (CBP): inclinations to process information in particular ways due to affective states. These cognitive biases include attention, memory, and judgment biases (reviewed by Kremer et al., Reference Kremer, Bus, Webb, Bokkers, Engel, van der Werf and van Reenen2021). For example, people in negative states are more likely to make negative (“pessimistic”) judgments about events or stimuli than people in more positive states (Blanchette & Richards, Reference Blanchette and Richards2010; Harding et al., Reference Harding, Paul and Mendl2004).
Attention bias (AB): refers to the selective allocation of attention to specific stimuli, studied through attention bias tasks that gauge attention allocation (reviewed by Crump et al., Reference Crump, Arnott and Bethell2018)
Memory bias (MB): involves the influence of an individual’s current emotional state on the nature of their recalled memories (Keen et al., Reference Keen, Nelson, Robbins, Evans, Shepherdson and Newberry2014). This bias remains unexplored in fish, and animal studies on this subject have only been conducted in rodents (rats: Burman & Mendl, Reference Burman and Mendl2018; mice: Takatsu-Coleman et al., Reference Takatsu-Coleman, Patti, Zanin, Zager, Carvalho, Borçoi and Frussa-Filho2013).
Judgment bias (JB): also known as the “ambiguous cue interpretation” task (ACI) (Rygula et al., Reference Rygula, Papciak and Popik2013), is the propensity to judge ambiguous cues or situations more or less optimistically (reviewed by Lagisz et al., Reference Lagisz, Zidar, Nakagawa, Neville, Sorato, Paul and Løvlie2020 for nonpharmacological studies, reviewed by Neville et al., Reference Neville, Nakagawa, Zidar, Paul, Lagisz, Bateson and Mendl2020 for pharmacological studies).
Sensitivity to reward shift (SRS): also known as “sensitivity to negative and positive feedback” (Noworyta-Sokolowska et al., Reference Noworyta-Sokolowska, Kozub, Jablonska, Rodriguez Parkitna, Drozd and Rygula2019), is an indicator of affect that more or less relies on cognition and may be viewed as a bias in evaluation and involves sensitivity to rewards and losses influenced by emotional states (Burman et al., Reference Burman, Parker, Paul and Mendl2008).
The living environment significantly influences an animal’s affective states, thereby impacting CBP driven by emotions (e.g., Mendl & Paul, Reference Mendl and Paul2020). Interventions aimed at inducing negative affective states (e.g., unpredictable housing, Harding et al., Reference Harding, Paul and Mendl2004; shaking, Bateson et al., Reference Bateson, Desire, Gartside and Wright2011); chronic stress, Rygula et al., Reference Rygula, Papciak and Popik2013) increase the likelihood of exhibiting negative cognitive bias (NCB) (Mendl & Paul, Reference Mendl and Paul2020), characterized by a tendency to interpret situations pessimistically (Enkel et al., Reference Enkel, Gholizadeh, Von Bohlen Und Halbach, Sanchis-Segura, Hurlemann, Spanagel and Vollmayr2009). For example, exposure to acute or chronic stressors in fish has been linked to anxiety-related behaviors (Buenhombre et al., Reference Buenhombre, Daza-Cardona, Sousa and Gouveia2021; Collier et al., Reference Collier, Kalueff, Echevarria and Kalueff2017; Golla et al., Reference Golla, Østby and Kermen2020). Additionally, exposure to stressors (Tan, Reference Tan2017) or non-preferred social stimuli (Laubu et al., Reference Laubu, Louâpre and Dechaume-Moncharmont2019) has been associated with NCB. Conversely, interventions aimed at inducing positive affective states, such as environmental enrichment or the use of anxiolytic drugs, often result in a more positive or balanced processing bias, referred to as an “optimistic” response (Bateson, Reference Bateson2016). Buenhombre et al. (Reference Buenhombre, Daza-Cardona, Sousa, Gouveia and Cajiao-Pachón2022) observed this effect in fish subjected to various forms of environmental enrichment. Similarly, Laubu et al. (Reference Laubu, Louâpre and Dechaume-Moncharmont2019) found that exposure to preferred social stimuli in fish results in a positive cognitive bias (PCB). These results underscore the influence of the physical and social environment on fish CBP.
In addition to environmental factors, personality traits could also contribute to variations in CBP. For example, calves (Lecorps et al., Reference Lecorps, Weary and von Keyserlingk2018) and parrots (Cussen & Mench, Reference Cussen and Mench2015), characterized as fearful or neurotic, respectively, have exhibited a more pessimistic cognitive bias while housed under the same conditions. Furthermore, housing and personality may interact to affect CBP, as seen in studies with pigs (Asher et al., Reference Asher, Friel, Griffin and Collins2016), cows (Kremer et al., Reference Kremer, Bus, Webb, Bokkers, Engel, van der Werf and van Reenen2021; Lecorps et al., Reference Lecorps, Weary and von Keyserlingk2018), and hens (Ross et al., Reference Ross, Garland, Harlander-Matauschek, Kitchenham and Mason2019).
A different approach analyses CBP as stable and enduring behavioral traits. Consequently, consecutive assays measuring CBP have been employed in rats (e.g., Enkel et al., Reference Enkel, Gholizadeh, Von Bohlen Und Halbach, Sanchis-Segura, Hurlemann, Spanagel and Vollmayr2009; Rygula & Popik, Reference Rygula and Popik2016) to categorize individuals into two phenotypic traits: those with a stable PCB, referred to as “optimistic,” and those with a stable NCB, referred to as “pessimistic.” This categorization has played a pivotal role in exploring the idea that CBP could be a trait contributing to the development, persistence, and recurrence of stress-related disorders such as depression and anxiety (Noworyta et al., Reference Noworyta, Cieslik, Rygula, Dziedzicka-Wasylewska and Faron-Górecka2021; Noworyta-Sokolowska et al., Reference Noworyta-Sokolowska, Kozub, Jablonska, Rodriguez Parkitna, Drozd and Rygula2019).
The above suggests that CBP in some species may incorporate aspects of stable personality traits and more transient affective states, similar to CBP in humans (Kluemper et al., Reference Kluemper, Little and Degroot2009; Rygula et al., Reference Rygula, Papciak and Popik2013). Moreover, depending on an individual’s personality, specific subpopulations of animals may exhibit varying sensitivity to environmental influences on CBP (e.g., Asher et al., Reference Asher, Friel, Griffin and Collins2016; Ross et al., Reference Ross, Garland, Harlander-Matauschek, Kitchenham and Mason2019). This hints at a potential link between personality traits, CBP, and stress resilience – the ability to manage potential stressors without significant impacts on normal physiology and behavior (Gesto et al., Reference Gesto, Madsen, Andersen and Jokumsen2018). While research indicates that fish exhibit personality traits (e.g., Castanheira et al., Reference Castanheira, Conceição, Millot, Rey, Bégout, Damsgård and Martins2017; Toms & Echevarria, Reference Toms and Echevarria2014), as further discussed in section three, these interactions have not been thoroughly explored in fish. In this context, our review aims to critically analyze and synthesize the current knowledge regarding CBP and personality in fish. We also aim to explore the potential components of CBP and how they might interact with various personality traits, influencing stress resilience or vulnerability in animals. Also, we highlight the relevance of fish studies as models for aspects of human personality.
1.1. CBP and their types in fish
In humans, emotions and moods have been shown to lead to CBP (Mendl & Paul, Reference Mendl and Paul2020). Typically, individuals experiencing negative affective states (e.g., anxiety) tend to exhibit heightened attention toward threatening stimuli (e.g., angry facial expressions), demonstrate a greater tendency to recall negative memories, and manifest negative judgments concerning future events or ambiguous stimuli (“pessimism”) when compared to those in more positive states (Barnard et al., Reference Barnard, Wells, Milligan, Arnott and Hepper2018). Drawing an analogy, similar CBP patterns have been observed in animals, indicating that affective states can also influence attention, memory, and decision-making across various species (Baciadonna & McElligott, Reference Baciadonna and McElligott2015; Bethell, Reference Bethell2015; Raoult et al., Reference Raoult, Trompf, Williamson and Brown2017; Roelofs et al., Reference Roelofs, Boleij, Nordquist and van der Staay2016), including fish (Buenhombre et al., Reference Buenhombre, Daza-Cardona, Sousa, Gouveia and Cajiao-Pachón2022; Espigares et al., Reference Espigares, Abad-Tortosa, Varela, Oliveira and Ferreira2021; Laubu et al., Reference Laubu, Louâpre and Dechaume-Moncharmont2019; Tan, Reference Tan2017; Tan et al., Reference Tan, Handasyde, Rault and Mendl2020a).
Among cognitive measures of affective states in fish, various tasks assessing CBP, attention bias (AB), judgment bias (JB), and sensitivity to reward shift (SRS) (definitions provided in Box 1) have been employed as follows. In AB tasks, animal attention is typically assessed by tracking looking times or recording reaction times in response to specific cues. For instance, in sheep (Lee, C. et al., Reference Lee, Verbeek, Doyle and Bateson2016; Monk et al., Reference Monk, Doyle, Colditz, Belson, Cronin and Lee2018) and cattle (Lee, C. et al., Reference Lee, Cafe, Robinson, Doyle, Lea, Small and Colditz2018), anxiogenic drug administration increased looking time at a hatch (opened to reveal a threatening dog), while anxiolytics decreased it. Although eye movements can be tracked in larvae and adult fish (e.g., Dehmelt et al., Reference Dehmelt, Von Daranyi, Leyden and Arrenberg2018), there have been no formal AB studies using looking time tasks in fish. Reaction times can reveal how emotional information distracts individuals during a neutral cognitive task, potentially uncovering attentional biases, such as slower responses to negative stimuli, especially in anxious populations (Cisler & Koster, Reference Cisler and Koster2010). However, studies of this nature are scarce in animals (Crump et al., Reference Crump, Arnott and Bethell2018) and remain unexplored in fish. To our knowledge, only one study has examined AB in fish, measuring avoidance responses toward a threatening stimulus as an indicator of animal attention (Tan, Reference Tan2017). In this experiment, a rotating black strip positioned along the edge of a circular aquarium served as the threatening stimulus. Stressed fish exhibited a higher tendency to position themselves in the inner half of the tank, farther away from the threatening stimulus, in contrast to the control fish. This suggests that fish may demonstrate heightened attention toward novel visual stimuli when exposed to stress-like conditions (Tan, Reference Tan2017).
JB tasks have been adapted from rodents (Harding et al., Reference Harding, Paul and Mendl2004) to various species (see Lagisz et al., Reference Lagisz, Zidar, Nakagawa, Neville, Sorato, Paul and Løvlie2020 for a review), including fish. This experimental approach assesses the expectations of favorable or unfavorable outcomes based on previously acquired cues. During training, subjects learn to respond positively (e.g., approach a location) to a positive stimulus (e.g., position and/or color A) to receive a positive outcome (e.g., food) and negatively (e.g., avoid a location) to a different stimulus (e.g., position and/or color B) to avoid relatively negative outcomes, such as receiving no food (Buenhombre et al., Reference Buenhombre, Daza-Cardona, Sousa, Gouveia and Cajiao-Pachón2022; Laubu et al., Reference Laubu, Louâpre and Dechaume-Moncharmont2019) or being chased with a net (Espigares et al., Reference Espigares, Abad-Tortosa, Varela, Oliveira and Ferreira2021) as observed in fish. Subsequently, ambiguous cues are occasionally introduced to evaluate their anticipation of positive or negative outcomes. The hypothesis is that, similar to humans, negative affective states lead animals to respond to ambiguous cues as if they predict a negative event, and vice versa (for a review and meta-analysis, see Lagisz et al., Reference Lagisz, Zidar, Nakagawa, Neville, Sorato, Paul and Løvlie2020).
So far, both physical and social conditions have been shown to affect JB in fish. Female convict cichlids assigned to non-preferred partners, which were predicted to elicit negative emotions, exhibited a pessimistic bias (Laubu et al., Reference Laubu, Louâpre and Dechaume-Moncharmont2019). In contrast, manipulations predicted to elicit positive emotions, such as constant environmental enrichment in zebrafish (Buenhombre et al., Reference Buenhombre, Daza-Cardona, Sousa, Gouveia and Cajiao-Pachón2022) and staying with a preferred partner in cichlids (Laubu et al., Reference Laubu, Louâpre and Dechaume-Moncharmont2019), generated positive JB. Additionally, there is evidence suggesting the involvement of certain key genes in JB. For instance, Espigares et al. (Reference Espigares, Abad-Tortosa, Varela, Oliveira and Ferreira2021) found that telomerase-deficient fish exhibited a more pessimistic response toward ambiguity compared to their wild-type conspecifics.
When it comes to SRS, humans, for instance, tend to exhibit greater sensitivity to potential losses than gains (e.g., Dreher, Reference Dreher2007). Moreover, individuals in a negative affective state often show enhanced sensitivity to loss or failure (e.g., Taylor Tavares et al., Reference Taylor Tavares, Clark, Furey, Williams, Sahakian and Drevets2008). The assessment of animals’ SRS tasks can be conducted through operant conditioning studies designed to investigate successive negative or positive contrast effects, as comprehensively reviewed by Rygula et al. (Reference Rygula, Noworyta-Sokolowska, Drozd and Kozub2018). For example, fish can be trained to swim down a channel to obtain either high or lower value food rewards, with reward values being unexpectedly switched, and the effect of this switch on the time taken to complete the action recorded (Tan et al., Reference Tan, Handasyde, Rault and Mendl2020b). Sensitivity to reward loss has been demonstrated in various mammals, such as rats housed in unenriched conditions, which typically exhibit indicators of a more negative affective state compared to those in enriched housing (e.g., Burman et al., Reference Burman, Parker, Paul and Mendl2008). Similarly, pharmacological manipulations in rats that boost serotonin neurotransmission have been shown to decrease sensitivity to loss and increase reward sensitivity (Bari et al., Reference Bari, Theobald, Caprioli, Mar, Aidoo-Micah, Dalley and Robbins2010). However, in the case of fish, goldfish have been observed to display a downshift in performance with reduced rewards but did not perform worse than controls, indicating no sensitivity to reward loss (e.g., Couvillon & Bitterman, Reference Couvillon and Bitterman1985). Similarly, in zebrafish, individuals conditioned to high-value rewards did not change their swimming speed when rewards were downshifted, suggesting no sensitivity to reward loss. Housing type did not affect swim time either (Tan et al., Reference Tan, Handasyde, Rault and Mendl2020a).
1.2. Personality in fish
The concept of personality in humans encompasses enduring behavioral, emotional, and cognitive traits that persist over time and across different situations. However, the definition and measurement of personality can vary depending on the research approach employed. In the context of studying personality in nonhuman species, a trait approach is commonly adopted (Khan & Echevarria, Reference Khan, Echevarria, Vonk, Weiss and Kuczaj2017). Research indicates that fish exhibit personality traits (known as consistency in behavior and physiology across time and context and which is characteristic of a certain group of individuals) (Castanheira et al., Reference Castanheira, Herrera, Costas, Conceição and Martins2013a; Toms & Echevarria, Reference Toms and Echevarria2014). Fish researchers frequently employ a cluster of overlapping terms, including “personality traits,” “coping styles,” “behavioural syndromes,” “phenotypic expression,” “behavioural plasticity,” and “individual differences” (e.g., Buenhombre et al., Reference Buenhombre, Daza-Cardona, Sousa and Gouveia2021; Conrad et al., Reference Conrad, Weinersmith, Brodin, Saltz and Sih2011; Demin et al., Reference Demin, Lakstygal, Alekseeva, Sysoev, de Abreu, Alpyshov and Kalueff2019). Currently, studies in fishes have identified personality traits such as boldness, shyness (e.g., Thorbjørnsen et al., Reference Thorbjørnsen, Moland, Villegas-Ríos, Bleeker, Knutsen and Olsen2021), exploration, avoidance, aggressiveness, locomotor activity, and sociability (e.g., Conrad et al., Reference Conrad, Weinersmith, Brodin, Saltz and Sih2011; Khan & Echevarria, Reference Khan, Echevarria, Vonk, Weiss and Kuczaj2017; Szopa-Comley et al., Reference Szopa-Comley, Duffield, Ramnarine and Ioannou2020).
Fish personality traits, akin to those in humans, are conceptualized as latent axes of variation that underlie observed behaviors, and these traits are often quantified using mathematical models (for a detailed explanation, see Conrad et al., Reference Conrad, Weinersmith, Brodin, Saltz and Sih2011; Prentice et al., Reference Prentice, Houslay and Wilson2022; Toms et al., Reference Toms, Echevarria and Jouandot2010). Specific assays, such as the open-field test, the novel tank test, the emergence test, and the Y-maze, among others (e.g., Buenhombre et al., Reference Buenhombre, Daza-Cardona, Sousa and Gouveia2021), are employed to position fish along a continuous dimension defined by two or more axes of interest, such as boldness versus shyness. While some studies use only one of these assays to categorize fish, most employ multiple assays and assess various behaviors over time (e.g., Colchen et al., Reference Colchen, Faux, Teletchea and Pasquet2017; Conrad et al., Reference Conrad, Weinersmith, Brodin, Saltz and Sih2011; Toms et al., Reference Toms, Echevarria and Jouandot2010). This approach involves using correlational and multivariate analyses to establish behavioral clusters representing the underlying behavioral axes. For example, if a fish population exhibits variation in aggressiveness, it implies that certain individuals tend to be more aggressive, leading them to display behaviors such as attacking a mirror stimulus, engaging in increased rivalry displays, or frequently chasing tank mates (Prentice et al., Reference Prentice, Houslay and Wilson2022). However, recent findings indicate that there are distinct hormonal and genomic responses in fish when they engage in combat with real conspecific opponents compared to when they confront their own mirror images (Balzarini et al., Reference Balzarini, Taborsky, Wanner, Koch and Frommen2014; Oliveira et al., Reference Oliveira, Simes, Teles, Oliveira, Becker and Lopes2016; Teles & Oliveira, Reference Teles and Oliveira2016).
Research also suggests that fish exhibit “behavioural syndromes,” essentially personality traits that are correlated with each other (e.g., Conrad et al., Reference Conrad, Weinersmith, Brodin, Saltz and Sih2011; Torgerson-White & Sánchez-Suárez, Reference Torgerson-White and Sánchez-Suárez2022). For instance, in zebrafish (e.g., Ariyomo & Watt, Reference Ariyomo and Watt2012; Martins & Bhat, Reference Martins and Bhat2014), stickleback (e.g., Bell & Sih, Reference Bell and Sih2007), and guppies (e.g., Smith & Blumstein, Reference Smith and Blumstein2010), a recurring “bold-aggression syndrome” has been observed. This relationship may arise from shared physiological and genetic mechanisms, as well as environmental effects (e.g., Conrad et al., Reference Conrad, Weinersmith, Brodin, Saltz and Sih2011; Prentice et al., Reference Prentice, Houslay and Wilson2022). However, it is crucial to recognize that not all studies have established a direct link between boldness and aggression (Way et al., Reference Way, Kiesel, Ruhl, Snekser and McRobert2015). Additionally, the various approaches to defining and measuring boldness (Toms et al., Reference Toms, Echevarria and Jouandot2010), along with the context-dependent nature of aggressiveness (Conrad et al., Reference Conrad, Weinersmith, Brodin, Saltz and Sih2011; Dahlbom et al., Reference Dahlbom, Backström, Lundstedt-Enkel and Winberg2012; Zabegalov et al., Reference Zabegalov, Kolesnikova, Khatsko, Volgin, Yakovlev, Amstislavskaya and Kalueff2018), can introduce complexities in interpreting this association. Moreover, besides boldness, aggression is often associated with other traits such as activity and dominance, contributing to a broader “aggression” + behavioral syndrome (Zabegalov et al., Reference Zabegalov, Kolesnikova, Khatsko, Volgin, Yakovlev, Amstislavskaya and Kalueff2018).
Furthermore, in fish, the shy-bold dimension is associated with individual variations in both behavioral and physiological responses to stressful stimuli, often referred to as “stress coping styles” (e.g., Castanheira et al., Reference Castanheira, Conceição, Millot, Rey, Bégout, Damsgård and Martins2017; Thörnqvist et al., Reference Thörnqvist, McCarrick, Ericsson, Roman and Winberg2019; Torgerson-White & Sánchez-Suárez, Reference Torgerson-White and Sánchez-Suárez2022). These trait variations frequently cluster into two contrasting styles, representing the extremes of a continuous axis. Fish can be characterized as either proactive (engaging in active coping or bold behaviors like “fight-flight”) or reactive (exhibiting passive coping or shy behaviors, often labeled as “non-aggressive”) (e.g., Castanheira et al., Reference Castanheira, Herrera, Costas, Conceição and Martins2013b; Saraiva et al., Reference Saraiva, Castanheira, Arechavala-López, Volstorf, Studer, Saraiva and Studer2018). For example, lines of rainbow trout selected for stress-induced plasma cortisol levels exhibited correlated changes in social, feeding, and locomotor behavior (as reviewed by (Øverli et al., Reference Øverli, Winberg and Pottinger2005). Similarly, wild-type guppies demonstrated evidence of genetic correlation structures between stress-related behavioral traits (such as thigmotaxis and freezing) expressed in open-field trials (OFTs) and the levels of free circulating cortisol produced in response to an isolation and confinement stressor (Houslay et al., Reference Houslay, Earley, White, Lammers, Grimmer, Travers and Wilson2022).
However, the stress coping style model presents certain challenges. Traits vary along two independent axes: a qualitative coping style axis and a quantitative stress reactivity axis (Koolhaas et al., Reference Koolhaas, de Boer, Coppens and Buwalda2010), which can make it challenging and subjective at times to determine how observed data align with these axes (Houslay et al., Reference Houslay, Earley, White, Lammers, Grimmer, Travers and Wilson2022). Furthermore, only a limited number of studies in fish have incorporated repeated observations of both endocrine and behavioral stress response traits (Boulton et al., Reference Boulton, Couto, Grimmer, Earley, Canario, Wilson and Walling2015; Thörnqvist et al., Reference Thörnqvist, McCarrick, Ericsson, Roman and Winberg2019), and some of these studies present inconsistent (Boulton et al., Reference Boulton, Couto, Grimmer, Earley, Canario, Wilson and Walling2015) and context-dependent findings (Alfonso et al., Reference Alfonso, Sadoul, Gesto, Joassard, Chatain, Geffroy and Bégout2019; Thomson et al., Reference Thomson, Watts, Pottinger and Sneddon2012), complicating the interpretation somewhat (Prentice et al., Reference Prentice, Houslay and Wilson2022). Additionally, a recent study suggests that a single divergent stress coping style may not fully capture the diverse range of behavioral clusters beyond the original bimodal reactive–proactive characterization (Rajput et al., Reference Rajput, Parikh and Kenney2022). Furthermore, behavioral clusters can be influenced by factors such as social context (Magnhagen & Bunnefeld, Reference Magnhagen and Bunnefeld2009), strain, and sex (Rajput et al., Reference Rajput, Parikh and Kenney2022; Wong et al., Reference Wong, French and Russ2019).
1.3. Trait sensitivity to stress and cognitive bias processes
From fish through humans, some individuals are likely better at coping with adverse conditions than others (Sørensen et al., Reference Sørensen, Johansen and Øverli2013). Experience (e.g., habitat complexity and rearing conditions) (Lee, C. J. et al., Reference Lee, Cafe, Robinson, Doyle, Lea, Small and Colditz2018) and genetic factors (e.g., fibroblast growth factor receptor 1a simultaneously increase aggression, boldness, and exploration in adult zebrafish) (Norton et al., Reference Norton, Stumpenhorst, Faus-Kessler, Folchert, Rohner, Harris and Bally-Cuif2011) may control this among individual differences in fish’s stress responses (Sørensen et al., Reference Sørensen, Johansen and Øverli2013). These consistent differences among fishes modulate the way they perceive and react to their environment, which in turn affects their robustness (Vindas et al., Reference Vindas, Magnhagen, Brännäs, Øverli, Winberg, Nilsson and Backström2017) and resilience (Buenhombre et al., Reference Buenhombre, Daza-Cardona, Sousa and Gouveia2021) to challenges. For instance, proactive individuals create routines, are explorative and risk-taking (Sih et al., Reference Sih, Bell, Johnson and Ziemba2004), and seem to have a high level of active avoidance, locomotor activity, and low flexibility in behavioral responses when faced with challenges, while reactive individuals behave with the opposite patterns (Ruiz-Gomez et al., Reference Ruiz-Gomez, Huntingford, Øverli, Thörnqvist and Höglund2011; Sih et al., Reference Sih, Bell, Johnson and Ziemba2004). In addition, proactive individuals exhibit typical physiological and neuroendocrine characteristics such as higher expression of dopamine and opioid receptors (Thörnqvist et al., Reference Thörnqvist, McCarrick, Ericsson, Roman and Winberg2019), lower levels of 5-hydroxyindoleacetic acid (5-HIAA) and baseline ratio of 5-HIAA/serotonin (5-HT) (Øverli et al., Reference Øverli, Pottinger, Carrick, Øverli and Winberg2001; Winberg & Thörnqvist, Reference Winberg and Thörnqvist2016), lower hypothalamus–pituitary–adrenal/interrenal activity (Øverli et al., Reference Øverli, Sørensen, Pulman, Pottinger, Korzan, Summers and Nilsson2007; Silva et al., Reference Silva, Martins, Engrola, Marino, Øverli and Conceição2010) as compared to reactive individuals.
Regarding individual differences in cognition, these have rarely been addressed in fish compared with humans and rodents (Lucon-Xiccato & Bisazza, Reference Lucon-Xiccato and Bisazza2017). Some studies suggest that fish’s personality traits can exert different influences on cognitive performance, depending on the specific task. Faster learning rates to avoid experiencing an unpleasant stimulus (an aversion learning paradigm that requires avoidance or reduced levels of activity) have been observed in risk-averse reactive individuals (Baker & Wong, Reference Baker and Wong2019; Budaev & Zhuikov, Reference Budaev and Zhuikov1998), and it has been hypothesized that reactive individuals may perceive stressors as more threatening, which could facilitate faster encoding of aversive experiences (Baker & Wong, Reference Baker and Wong2019). The expression of two neural plasticity and neurotransmission-related genes (npas4a and gabbr1a) may be involved in fear learning differences among stress coping styles (Baker & Wong, Reference Baker and Wong2021). On the contrary, the more risk-prone proactive individuals tend to show faster acquisition of memories that require higher levels of activity or paradigms with positive and rewarding valence (Baker & Wong, Reference Baker and Wong2019; Lucon-Xiccato & Bisazza, Reference Lucon-Xiccato and Bisazza2017). Likewise, Ferrari et al. (Reference Ferrari, Wisenden and Chivers2010) found that shy rainbow trout had better memory for a predator odor 8 days after conditioning it with alarm cues from conspecific skin. The latency of the fish to emerge from an opaque chamber placed in a novel tank after a 20-min habituation was used to categorize the trout. The longer the latency to emerge, the shier the individual was. However, it is worth noting that not all studies have established these associations (Kareklas et al., Reference Kareklas, Elwood and Holland2018; Vital & Martins, Reference Vital and Martins2013).
Despite several studies exploring numeracy, spatial cognition, social cognition (as reviewed by Lucon-Xiccato & Bisazza, Reference Lucon-Xiccato and Bisazza2017; Salena et al., Reference Salena, Turko, Singh, Pathak, Hughes, Brown and Balshine2021), and more recently, some studies exploring CBP in fish, the influence of fish’s personality traits on cognitive processes other than cognitive achievement has not been explored yet. Nonetheless, fish personality traits could likely be intertwined with CBP, as observed in pigs (Asher et al., Reference Asher, Friel, Griffin and Collins2016), cows (Kremer et al., Reference Kremer, Bus, Webb, Bokkers, Engel, van der Werf and van Reenen2021), hens (Ross et al., Reference Ross, Garland, Harlander-Matauschek, Kitchenham and Mason2019), and dogs (Barnard et al., Reference Barnard, Wells, Milligan, Arnott and Hepper2018).
CBP in animals is often considered a transient state influenced by the animal’s mood (Mendl et al., Reference Mendl, Burman, Parker and Paul2009). However, an alternative perspective has emerged, suggesting that CBP could also be seen as enduring traits (Faustino et al., Reference Faustino, Oliveira and Oliveira2015). Evidence from studies in rodents (e.g., Noworyta et al., Reference Noworyta, Cieslik, Rygula, Dziedzicka-Wasylewska and Faron-Górecka2021; Noworyta-Sokolowska et al., Reference Noworyta-Sokolowska, Kozub, Jablonska, Rodriguez Parkitna, Drozd and Rygula2019; Rygula et al., Reference Rygula, Papciak and Popik2013) and calves (Lecorps et al., Reference Lecorps, Weary and von Keyserlingk2018) supports this idea, demonstrating that these animals exhibit stable individual differences in their levels of pessimism or optimism within CBP. Furthermore, Rygula et al. (Reference Rygula, Papciak and Popik2013) found that rodents categorized as optimistic or pessimistic after chronic stress exposure consistently made pessimistic judgments about ambiguous stimuli. This suggests that CBP in animals, similar to humans, may encompass characteristics of both a trait and a transient state (Kluemper et al., Reference Kluemper, Little and Degroot2009; Rygula et al., Reference Rygula, Papciak and Popik2013).
CBP has also been considered a vulnerability factor for the etiology, maintenance, and recurrence of stress-related disorders (e.g., Clark & Beck, Reference Clark and Beck2010). In humans, patients with these disorders often exhibit NCB (e.g., Disner et al., Reference Disner, Beevers, Haigh and Beck2011; Robinson, Reference Robinson, Baune and Harmer2019). Similarly, in rodents, environmental, pharmacological, and genetic manipulations that induce stress-like states (reviewed by Nguyen et al., Reference Nguyen, Guo and Homberg2020) have been found to cause NCB. Furthermore, rats classified as pessimistic tend to show higher vulnerability to stress-induced anhedonia (Rygula et al., Reference Rygula, Papciak and Popik2013), increased sensitivity to reward losses (Rygula & Popik, Reference Rygula and Popik2016), and an inflammatory immune profile compared to optimistic animals (Curzytek et al., Reference Curzytek, Kubera, Trojan, Wójcik, Basta-Kaim, Detka and Rygula2018).
In fish, Espigares et al. (Reference Espigares, Abad-Tortosa, Varela, Oliveira and Ferreira2021) observed NCB in a mutant strain of zebrafish with shorter telomeres and in aging fish with age-related telomere shortening. These mutant zebrafish exhibit early phenotypic alterations, including increased inflammation, which are common in aged organisms and may contribute to the NCB observed in the telomerase-deficient mutants. Additionally, Espigares et al. (Reference Espigares, Alvarado, Faísca, Abad-Tortosa and Oliveira2022) found that fish categorized as pessimistic increase their reproductive investment after chronic stress, leading to increased vitellogenesis. These findings suggest that in animals, including fish, optimistic and pessimistic traits and states may confer different levels of resilience to individuals in stressful situations (Faustino et al., Reference Faustino, Oliveira and Oliveira2015), indicating that pessimistic traits/states may be less resilient to stress, and vice versa.
2. Conclusions
Clearly, zebrafish models of CBP are still in the early stages of development, and numerous unanswered questions persist (Table 1). For instance, although zebrafish do exhibit CBP, these responses may be influenced by individual differences, such as age, sex, personality, and strain, owing to environmental and genetic variations, along with their interplay (Volgin et al., Reference Volgin, Yakovlev, Demin, de Abreu, Alekseeva, Friend and Kalueff2019). Consequently, CBP research in fish must meticulously consider, distinguish, and control for these factors and their interactions, with particular attention to personality traits. The intricate nature of these variables can potentially complicate the interpretation of CBP results, as we have discussed in this review across various species. Moreover, CBP may encompass both transient affective states and more enduring personality traits (e.g., Faustino et al., Reference Faustino, Oliveira and Oliveira2015; Kluemper et al., Reference Kluemper, Little and Degroot2009; Rygula & Popik, Reference Rygula and Popik2016). Consequently, CBP in fish could serve as a valuable model for disentangling which of these components is being assessed and how negative or positive states/traits influence an animal’s evaluation of ambiguous stimuli. This understanding can aid in identifying individuals who may exhibit higher stress resilience. For example, rodents in negative states have been shown to be less resilient to aversive events, as demonstrated by Rygula et al. (Reference Rygula, Papciak and Popik2013), and pessimistic fish display different reproductive outcomes after experiencing stress, as observed by Espigares et al. (Reference Espigares, Alvarado, Faísca, Abad-Tortosa and Oliveira2022). Additional research is warranted to establish the validity and reproducibility of CBP as suitable measures of affective states or traits in fish. Finally, insights gained from fish research on CBP may contribute to cognitive models suggesting that stress-related disorders in humans are linked to biases in cognitive processing (Beck, Reference Beck2008).
Table 1. Selected outstanding questions in fish CBP research
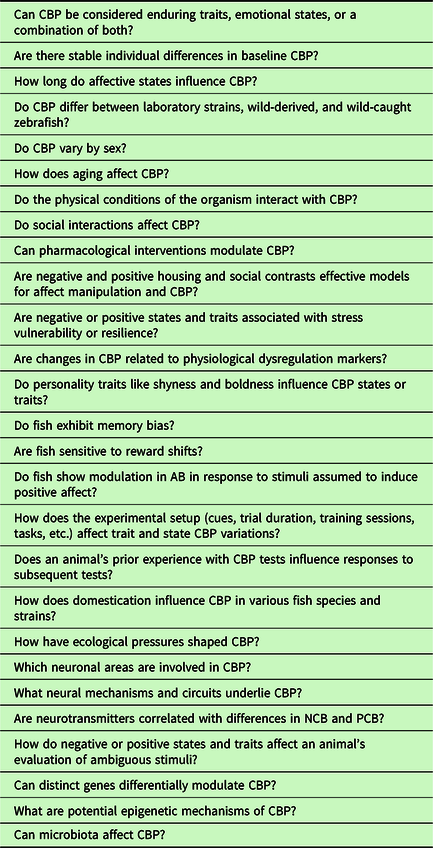
Author contributions
Conceptualization: J.B, EADC, DMR, ADO, AR, CMG, PT, MNCP, FV, APT, and PS; Validation: J.B, EADC, DMR, ADO, and AR; Searching, screening, and articles extraction: J.B, EADC, DMR, and ADO; Writing – original draft: J.B, EADC, DMR, ADO, AR, CMG, PT, and APT; Visualization: J.B, EADC, DMR, ADO, and AR; Writing – review and editing: J.B, EADC, DMR, ADO, AR, and APT; Approbation: J.B, EADC, DMR, ADO, AR, CMG, PT, MNCP, FV, APT, and PS.
Funding
This research did not receive any specific grant from funding agencies in the public, commercial, or not-for-profit sectors.
Competing interests
The authors report no declarations of interest.