INTRODUCTION
The quality of radiocarbon (14C) dating depends to a significant extent on the careful evaluation and subsequent correction of extraneous carbon contamination. Appropriate correction for extraneous carbon becomes increasingly important as technological advance (Synal et al. Reference Synal, Stocker and Suter2007; Fahrni et al. Reference Fahrni, Wacker, Synal and Szidat2013; Wacker et al. Reference Wacker, Fahrni, Hajdas, Molnar, Synal, Szidat and Zhang2013a, Reference Wacker, Fülöp, Hajdas, Molnár and Rethemeyer2013b) made the routine analysis of small scale samples (< 100 µgC) available to the broader scientific community. Carbon contamination is introduced to an analysis during various analytical steps and stems from the machine background, sample preparation and contamination inherited by the sample itself, and assuming constant contamination levels the deviation from the true 14C signal increases with ever smaller sample sizes.
The recently introduced carbonate handling system (CHS) coupled via the gas interface system (GIS) to the mini carbon dating system accelerator mass spectrometer (MICADAS AMS, as described by Wacker et al. Reference Wacker, Lippold, Molnár and Schulz2013c, Bard et al. Reference Bard, Tuna, Fagault, Bonvalot, Wacker, Fahrni and Synal2015, and Mollenhauer et al. Reference Mollenhauer, Grotheer, Gentz, Bonk and Hefter2021) proved to be particularly useful and is increasingly applied to paleoclimatic studies to obtain foraminifera based sediment stratigraphy (Küssner et al. Reference Küssner, Sarnthein, Lamy and Tiedemann2018; Ausín et al. Reference Ausín, Sarnthein and Haghipour2021), information on past carbon cycling (Winterfeld et al. Reference Winterfeld, Mollenhauer, Dummann, Köhler, Lembke-Jene, Meyer, Hefter, McIntyre, Wacker and Kokfelt2018; Wei et al. Reference Wei, Mollenhauer, Hefter, Kusch, Grotheer, Schefuß and Jia2021) or ocean circulation variations (Gottschalk et al. Reference Gottschalk, Michel, Thöle, Studer, Hasenfratz, Schmid, Butzin, Mazaud, Martínez-García and Szidat2020; Missiaen et al. Reference Missiaen, Wacker, Lougheed, Skinner, Hajdas, Nouet, Pichat and Waelbroeck2020; Ronge et al. Reference Ronge, Prange, Mollenhauer, Ellinghausen, Kuhn and Tiedemann2020). In case of the CHS-GIS-MICADAS carbon contamination levels depend on the machine background (GIS excl. zeolite trap + MICADAS), sample processing (acid digestion + CHS + GIS incl. zeolite trap), and surface contamination of the carbonate sample itself (Figure 1). The machine background can be considered roughly constant and has a larger influence on measured radiocarbon ages with decreasing sample size. The sample processing contamination scales with the number and kind of preparatory steps and volumes of chemicals used and can be considered constant for defined workflows. The total amount of surface contamination of the carbonate sample depends directly on sample size, surface structure, surface area on which contamination could be adsorbed and post-depositional alterations the sample was exposed to. Nevertheless, assuming that surface area and structure are comparable between foraminifera samples the relative contribution of surface contamination to the total amount of analyzed C would be constant, independent of sample size.
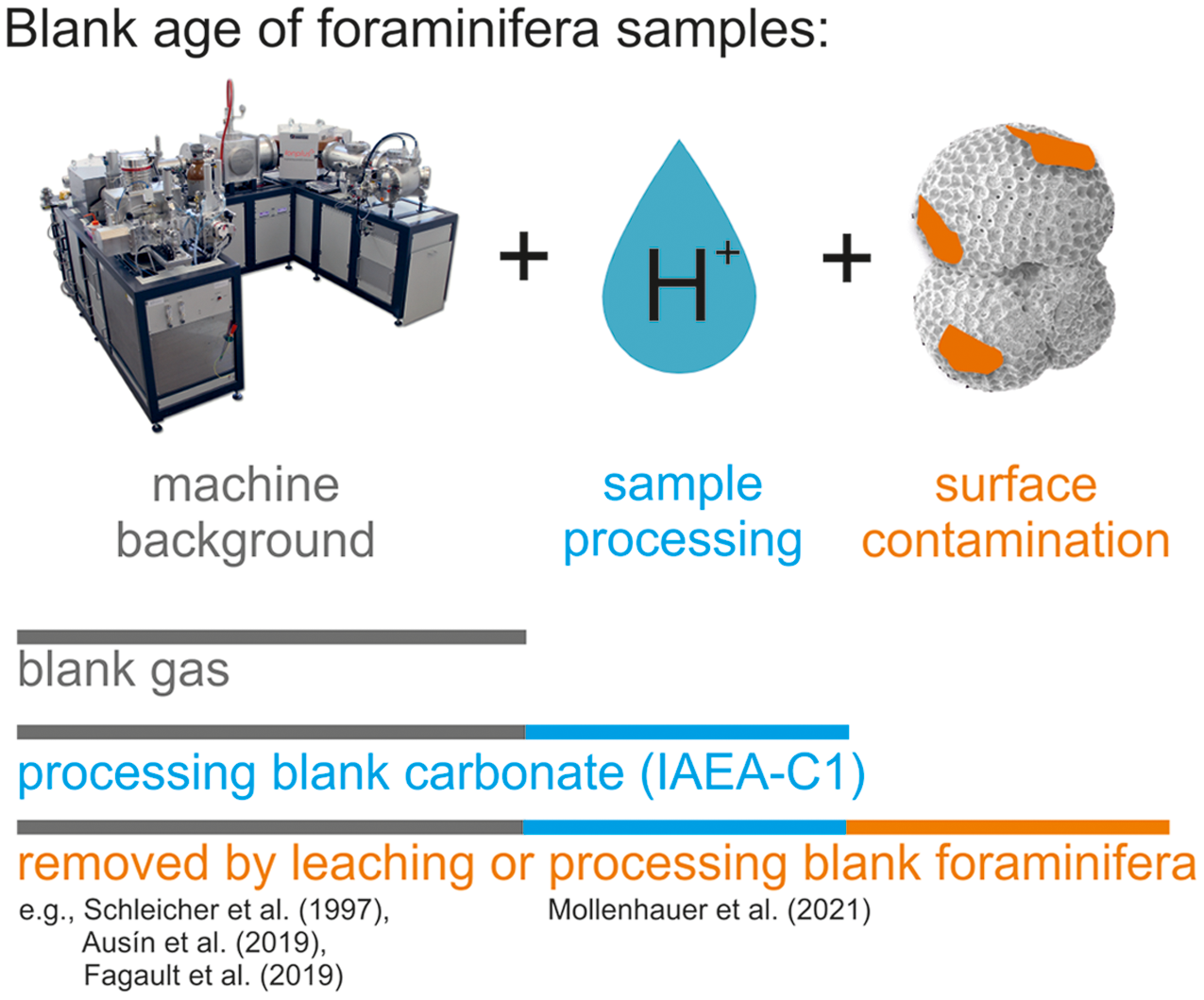
Figure 1 Theoretical concept of carbon contamination introduced during the analysis of foraminifera and how it can be assessed and corrected for.
The machine background can be determined by analyzing 14C-free gas (blank gas, 14C free CO2) introduced to the GIS from gas bottles. The sample processing contamination of carbonates is usually assessed by digesting sample size-matched amounts of the international reference material IAEA-C1, a crystalline marble, processed identically to the samples and thus providing information on the carbon contamination introduced by the acid, the CHS, the complete GIS and the MICADAS. While processing IAEA-C1 is appropriate to assess the contamination introduced to the analysis of carbonate crystals or shells, such correction might be less ideal for foraminifera. Fine grained and porous foraminiferal tests potentially host significant amounts of surface contamination like clays and secondary carbonates (CaCO3) inherited by the foraminifera during post-depositional alteration processes and in addition potentially adsorbed gaseous CO2 (Schleicher et al. Reference Schleicher, Grootes, Nadeau and Schoon1997) introduced during sample handling. It was shown that such surface contamination could efficiently been removed by leaching the samples prior to analysis (Schleicher et al. Reference Schleicher, Grootes, Nadeau and Schoon1997; Bard et al. Reference Bard, Tuna, Fagault, Bonvalot, Wacker, Fahrni and Synal2015; Ausín et al. Reference Ausín, Haghipour, Wacker, Voelker, Hodell, Magill, Looser, Bernasconi and Eglinton2019; Fagault et al. Reference Fagault, Tuna, Rostek and Bard2019). During leaching small volumes of weak (hydrogen peroxide, H2O2) or diluted (hydrochloric acid, HCl) acid are added to the sample and following sufficient reaction time the hydrolyzed fraction (commonly 10 wt.% of the sample, hereafter referred to as leachate) is flushed out of the reaction vial. The leachate can be analyzed and may provide valuable information on the nature of contamination present in the sample. The remaining, leached sample is further completely hydrolyzed, and its radiocarbon signal is contamination corrected, most commonly, against IAEA-C1 marble.
An alternative process to assess and successfully correct for carbon contamination of a measurement (including contamination from the machine background, sample processing and surface contamination), omitting leaching, was recently reported by Mollenhauer et al. (Reference Mollenhauer, Grotheer, Gentz, Bonk and Hefter2021). Mollenhauer et al. (Reference Mollenhauer, Grotheer, Gentz, Bonk and Hefter2021) process sample size-matched 14C-free foraminifera (blank foraminifera, pre-Eamian age) for contamination correction alongside samples, assuming surface contamination on foraminifera primarily stems from adsorbed CO2 and should be comparable between sample and blank 14C-free foraminifera. In theory the Mollenhauer et al. (Reference Mollenhauer, Grotheer, Gentz, Bonk and Hefter2021) approach (untreated foraminifera corrected against 14C-free foraminifera) should provide identical radiocarbon ages as samples that were leached and corrected against IAEA-C1, as in both cases surface contamination is either mathematically or chemically removed. However, little evidence, besides agreeable results with international reference material, was provided. The assumption that surface contamination stems primarily from CO2 adsorption needs additional testing because surface contamination can also originate from early diagenetic overprinting (e.g., from carbonate coating) or core storage. It is therefore challenging to determine the full nature and origin of surface contamination on foraminiferal tests. We therefore emphasize that there is scope for optimization in the approach presented here and in Mollenhauer et al. (Reference Mollenhauer, Grotheer, Gentz, Bonk and Hefter2021), yet it provides a workaround for 14C blank consideration in sediment cores with 14C-free sections and with scarce foraminifera. In order to account for diagenetic effects, such as surface coating, it may be more appropriate to use 14C-free foraminifera of the same species and same core (location) for the blank correction. Such mono-specific blank foraminifera have more likely experienced the same post-depositional contamination and are more comparable in surface structure. However, especially in high latitudes carbonaceous fossils are extremely scares, and sediments recovered are rarely older than 50 ka, therefore site-specific correction with 14C-free foraminifera accounting for surface contamination may not be possible in most regions.
Here we investigate the effect different blank corrections (vs 14C-free CO2, IAEA-C1 or foraminifera) have on radiocarbon data of small-sized untreated and leached foraminifera analyzed on a low energy MICADAS AMS. Based on replicate analysis of two mono-specific young (∼ 3000 14C a) and fossil (14C-free) foraminifera samples we aim (i) to answer whether the Mollenhauer et al. (Reference Mollenhauer, Grotheer, Gentz, Bonk and Hefter2021) approach is valid despite its limitations, and (ii) to determine what are overarching, not contamination related, limitations hindering increasingly precise radiocarbon dating with ever smaller sample sizes.
MATERIALS AND METHODS
Material
To test the impact of leaching and blank correction on reported radiocarbon data we selected two mono-specific planktonic foraminifera samples with expected “young” (∼3000 14C a) and fossil radiocarbon age (14C-free). Young foraminifera, shallow-dwelling species Trilobatus sacculifer, were picked from the >160 µm fraction from the surface (0–1 cm) of sediment core GeoB1403-2 (Guinea Basin, 1.19833°S, 11.711667°W, 3692 m water depth; Wefer et al. Reference Wefer, Andersen, Balzer, Bleil, Brück, Burda, Dahmke, Donner, Felis and Fischer1991) retrieved by a giant box corer in 1986 on board the RV Meteor (M16/1). For the fossil (14C-free) foraminifera sample, species Globoconella inflata, was picked from the >150 µm fraction of sediment core GeoB3316-4 interval 521–541 cm (Continental Margin off Chile, 41.93833°S, 75.2133°W, 2575 m water depth; Hebbeln et al. Reference Hebbeln, Wefer, Baltazar, Beese, Bendtsen, Butzin, Daneri, Dellarossa, Diekamp and Dittert1995) retrieved by a gravity corer in 1995 on board the RV Sonne (SO102/1).
The young and fossil (14C-free) foraminifera sample sets were each split into 20 replicates. The first 10 replicates were measured without leaching (referred to as untreated) and contained 33 specimens of T. sacculifer (young) and 22 specimens of G. inflata (fossil, 14C-free) respectively, with all replicates amounting to a total mass of ∼800 µg CaCO3 (∼100 µgC). To account for sample loss (∼10 wt.%) during leaching, the second 10 replicates (referred to as leached) contained 36 specimens of T. sacculifer and 24 specimens of G. inflata respectively, with all replicates amounting to a total mass of ∼900 µg CaCO3 (∼110 µg C). After leaching leached and untreated replicates contained comparable sample size for radiocarbon analysis (∼100 µgC).
Radiocarbon Measurement
All samples were analyzed using the carbonate handling system (CHS) coupled via the gas interface system (GIS) to the mini carbon dating system accelerator mass spectrometer (MICADAS) as described by Mollenhauer et al. (Reference Mollenhauer, Grotheer, Gentz, Bonk and Hefter2021). Briefly, samples loaded into septum sealed vials were flushed for 5 min with 70 mL/min ultra-pure Helium (He) to remove atmospheric CO2 by the CHS two-way needle. Afterwards 200 µL of 0.01 M hydrochloric acid (37% HCl, Sigma-Aldrich 1.00317.2500, diluted with MilliQ water) were added to the replicates prepared for leaching. The 200 µL of 0.01 M HCl dissolves ∼100 µg CaCO3 (∼12 µgC) corresponding to ∼10 weight % of the sample. The resulting leachate was sampled for 1 min at 70 mL/min He flow after 30 min reaction time and measured against blank gas. After flushing (or sampling the leachate), 200 µL phosphoric acid (≥85% H3PO4, Fluka 30417) were added to the untreated and previously leached replicates and the hydrolysis of carbonates took place over ∼30 min at 70°C. Following complete hydrolyzation, sample CO2 was flushed from the vials for 1 min at 70 mL/min He flow, the gas stream was passed over a phosphorus pentoxide trap to remove water vapor and the CO2 was concentrated on the GIS zeolite trap. The resulting CO2 was manometrically quantified after thermal expansion from the zeolite trap, diluted with He (∼5% CO2 in He) and fed into the MICADAS ion source under constant pressure and flow.
Radiocarbon data were normalized against Oxalic Acid II standard gas (CO2 produced from NIST Oxalic Acid II, NIST SRM4990C). To illustrate the effect of blank correction the data were further blank corrected against (a) 14C-free CO2 reference gas, (b) size-matched 14C-free foraminifera (Mollenhauer et al. Reference Mollenhauer, Grotheer, Gentz, Bonk and Hefter2021), and (c) size-matched 14C-free IAEA-C1 processed alongside the samples. Untreated replicates were corrected against untreated blanks while leached replicates were corrected against leached blanks. Normalization and blank correction was performed using the BATS software (Wacker et al. Reference Wacker, Christl and Synal2010).
RESULTS AND DISCUSSION
Effect of Leaching on Blanks
Blank levels (14C contamination level) for the different materials (untreated and leached) used for blank correction are summarized in Table 1. Small differences between blanks were measured for untreated materials with untreated IAEA-C1 marble showing the lowest blank (45,700 ± 780 14C a) and untreated 14C-free foraminifera the largest (41,860 ± 740 14C a) with 14C-free CO2 in between (43,800 ± 1000 14C a). These are in agreement with long term blanks reported by Mollenhauer et al. (Reference Mollenhauer, Grotheer, Gentz, Bonk and Hefter2021). Leaching of IAEA-C1 and 14C-free foraminifera did not result in a reduction of the blank levels, for IAEA-C1 the blank even increased (Table 1). The observation that chemical pretreatment on carbonate blanks has little to no effect on small-scale samples is in accordance with previously published data (Gottschalk et al. Reference Gottschalk, Szidat, Michel, Mazaud, Salazar, Battaglia, Lippold and Jaccard2018).
Table 1 Mean fraction modern carbon (F14C) and respective radiocarbon age (14C age) ± standard deviation (s.d.) values of measured blank levels for the different materials used for blank correction. Untreated and leached samples were analyzed on two different days; blank levels are shown for 14C-free CO2 for the respective day and either untreated or leached 14C-free foraminifera and IAEA-C1 marble.

Untreated and leached samples and blanks were analyzed on 2 individual days about 1 month apart. As 14C-free CO2 derived blank increased with time (not shown), we attribute the changes in blank levels to variation in the machine background between the days of analysis. This suggests that for radiocarbon dating of small-sized carbonate materials analyzed on low-energy MICADAS AMS processing and sample surface contamination contribute little to the overall blank level, which is predominantly controlled by the machine background.
Effect of Leaching on Young Foraminifera
The results for the young T. sacculifer replicates are summarized in Table 2 and illustrated in Figure 2. The mean F14C values increased slightly between untreated and leached replicates for each blank correction method. However, the leachate was enriched in 14C compared to both, untreated and leached replicates. A two-way ANOVA was performed to test the effect of blank correction (corrected against 14C-free CO2, foraminifera or IAEA-C1 marble) and sample treatment (untreated or leached). The two-way ANOVA revealed no statistically significant interaction of the mean F14C-values between blank correction approach and sample treatment (F(2,54) = 6.99×10–5, p > 0.999). Therefore, results are statistically identical irrespectively of blank correction or sample treatment employed.
Table 2 Measured fraction modern carbon (F14C) ± 2σ results of untreated and leached foraminifera blank corrected against (a) 14C-free CO2, (b) size-matched 14C-free foraminifera, and (c) size-matched IAEA-C1 marble and calculated means ± standard deviation.
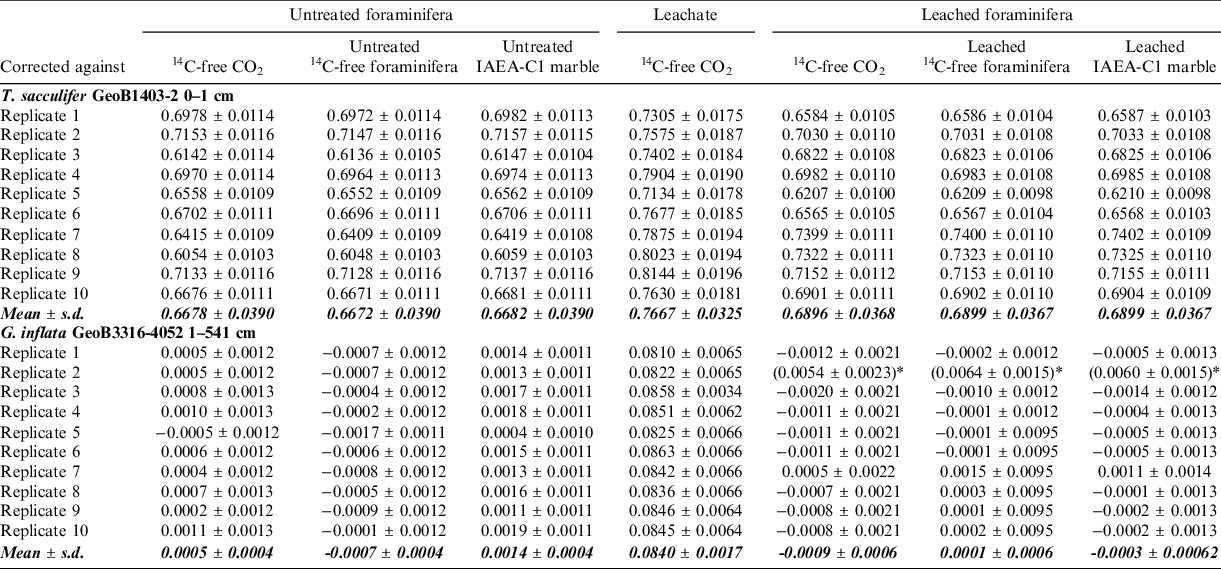
* Data considered outlier and removed from statistical evaluation.
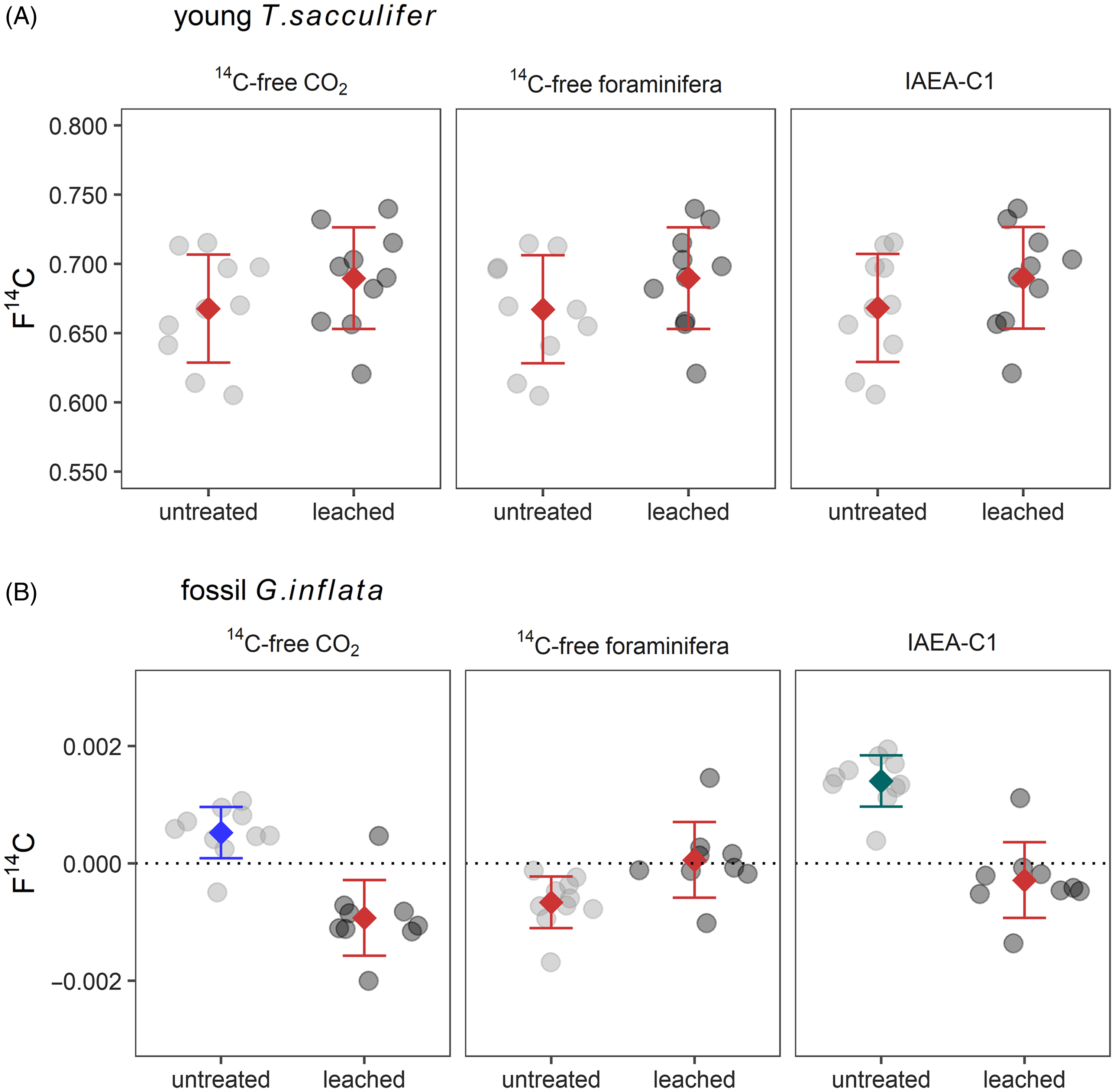
Figure 2 Sina plot (Sidiropoulos et al. Reference Sidiropoulos, Sohi, Pedersen, Porse, Winther, Rapin and Bagger2018) illustrating jitter of measured F14C values for (A) young T. sacculifer from core GeoB1403-2 interval 0–1 cm, and (B) fossil G. inflata from core GeoB3316-4 interval 521–541 cm, untreated (light gray circles) and leached (dark gray circles), separated for the respective blank corrections (corrected against 14C-free CO2, 14C-free foraminifera and IAEA-C1) employed, resulting leachate is not shown. Respective means ± standard deviations are shown with diamonds and error bars. Means sharing the same color are statistically similar based on the PostHoc tests at the 95% level of significance.
The observed increase between untreated and leached mean F14C is statistically insignificant, they do overlap within their standard deviation as replicate analysis showed significant scatter. The standard deviation of the individual means exceeds the analytical precision (±1σ, derived from counting statistics) of the MICADAS by a factor of >6. In contrast Mollenhauer et al. (Reference Mollenhauer, Grotheer, Gentz, Bonk and Hefter2021) reported that for a modern and homogenized coral CaCO3 standard the standard deviation of repeat analysis was lower compared to the analytical precision (±1σ). This indicates that for modern small-sized foraminifera samples other factors such as sample heterogeneity or natural variability play a large effect. This is as expected because the low blank values of all three materials only affect high F14C samples marginally. Nonetheless, data shown here confirm that the Mollenhauer et al. (Reference Mollenhauer, Grotheer, Gentz, Bonk and Hefter2021) approach is valid irrespectively of sample treatment. Corrected radiocarbon data obtained for young foraminifera are identical.
Effect of Leaching on Fossil Foraminifera
The mean F14C values decreased between untreated and leached replicates for 14C-free CO2 and IAEA-C1, but not for 14C-free foraminifera based blank correction. However, the respective means are highest for IAEA-C1 marble and decrease for 14C-free CO2 to 14C-free foraminifera corrected replicates. The general decrease in mean F14C of the leached replicates suggests that the leachate was enriched in 14C relative to the untreated sample. Except for the IAEA-C1 and 14C-free CO2 corrected untreated replicates, the means are below the expected F14C = 0, suggesting marginal overcorrection, and insufficient correction with IAEA-C1 respectively. However, differences are marginal.
The two-way ANOVA revealed statistically significant interaction between blank correction and sample treatment (F(2,51) = 28.19, p < 0.001) of the mean F14C values. The observation is confirmed by a Tukey’s honest significance pairwise test (TukeyHSD) of all combinations of mean F14C values. Means of untreated 14C-free CO2 and IAEA-C1 blank corrected replicates are statistically unique, all other means are statistically comparable to each other (indicated by shared colors in Figure 2). Further, the main effects of blank correction (F(2, 51) = 15.65, p < 0.001) and sample treatment of foraminifera were significant (F(1, 51) = 31.15, p < 0.001).
The statistical evaluation of the data confirms that the Mollenhauer et al. (Reference Mollenhauer, Grotheer, Gentz, Bonk and Hefter2021) approach is valid. Untreated samples corrected against 14C-free foraminifera processed alongside the samples result in statistically comparable mean radiocarbon ages compared to leached foraminifera samples corrected against 14C-free CO2 gas or IAEA-C1 marble.
Under which conditions does leaching improve the accuracy of foraminiferal radiocarbon ages?
Leaching is intended to clean the sample and thus improve the reliability of reported radiocarbon data of leached over untreated foraminifera. While cleaning in general is beneficial, whether or not data improvement can be achieved by this method most strongly depends on two factors: (a) analytical precision, and (b) natural variability. The analytical precision of the measurement determines at which age level leaching generates statistically improved results compared to untreated foraminifera. Only if the F14C difference between untreated and leached foraminifera (ΔF14Cuntreated-true) exceeds the analytical precision (±2σ) a statistical improvement of the data can be claimed. ΔF14Cuntreated-true depends on the true F14C of the foraminifera (F 14 C true , obtained after leaching) and the F14C and relative contribution of contaminant C (F 14 C contamination , f contamination ) mixed with the true F14C signal when an untreated sample is analyzed. To decide if leaching potentially leads to significant improvement of radiocarbon age accuracy, it is therefore important to estimate the contribution and isotopic composition of the contamination.
The F14C values obtained for leachates depend on the F14C signatures and the relative contribution of surface contamination and the fraction of foraminiferal CaCO3 that is dissolved during the leaching process. Therefore, these values typically cannot be used for the interpretation of foraminiferal F14C values for the purposes of age model development. Nevertheless, the F14C results of the paired leachate and leached replicates, corrected both against 14C-free CO2, can be used to estimate the relative contribution and F14C of the contamination removed during leaching assuming constant contamination. This can be achieved by combining the mass balance equations (eqs. 1 and 2) and the linear regression between F14C values of leached samples and the corresponding leachate (eq. 3). In these equations, term F 14 C leachate represents the F14C value of the leachate, F 14 C true the true F14C value of the foraminifera (measured after leaching), F 14 C contamination is the radiocarbon level of the contamination removed during leaching, f true and f contamination the relative contribution of the leached foraminifera and contamination to the leachate. Finally, m and b are the slope and intercept of the linear regression.



Comparison of eq. 1 and the linear regression (eq. 3) reveals that the slope (m) is defined by the relative contribution of leached foraminifera (f true, eq. 4) and the intercept (b) is defined by the relative contribution of the contamination (f contamination = 1–f true ) multiplied by its F14C value (eq. 5).


Applying these calculations to the leachate and leached F14C pairs of the reported young foraminifera replicates (Figure 3) one calculates that the leachate contains 27 % contamination with a relatively modern radiocarbon composition (F 14 C contamination = 0.9742), confirming that the contamination mainly stems from adsorbed atmospheric CO2. As we leached approximately 10% of the sample, we can estimate that an untreated sample would contain 2.7% contamination and 97.3% original foraminifera. Using this information one can modify the mass balance equation (eq. 1) to calculate the radiocarbon value of an untreated foraminifera sample (F 14 C untreated ) for every given F 14 C true (eq. 6), and calculate the expected isotopic difference between them (ΔF 14 C untreated-true , eq.7):


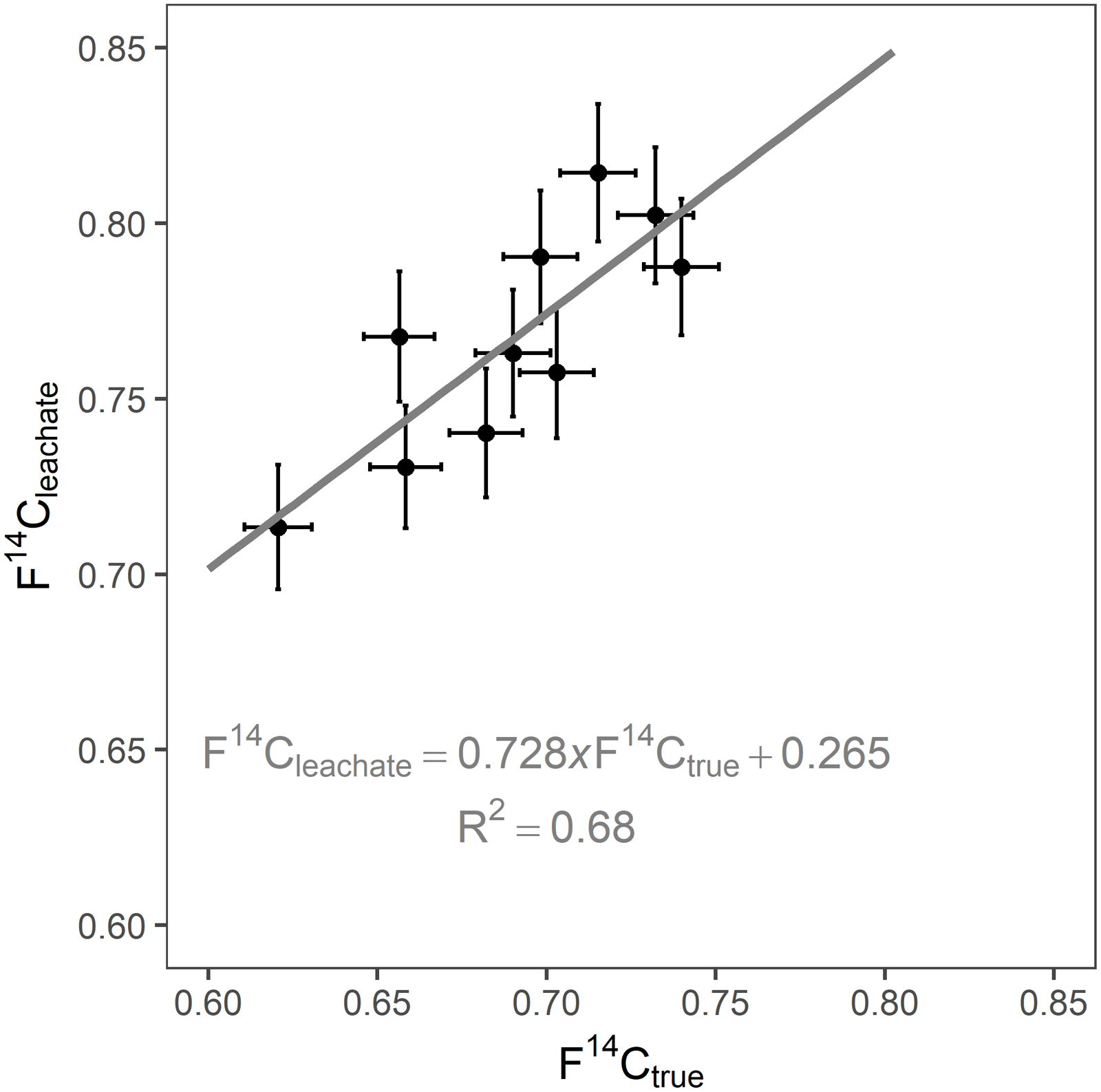
Figure 3 Linear regression between leached (F 14Ctrue) and corresponding leachate (F 14 C leachate ) of the young foraminifera T. sacculifer corrected against 14C-free CO2. Error bars show analytical precision (±2σ).
Figure 4 shows the expected isotopic difference between untreated and leached foraminifera (ΔF 14 C untreated-true ) depending on the true F14C value for three scenarios. In scenario A the untreated sample contains the relative amount (f contamination = 0.027) and isotopic composition of contamination adsorbed onto the surface (F 14 C contamination = 0.9742) estimated based on the young T. sacculifer in this study. The isotopic difference exceeds the analytical precision for samples older than ∼4500 14C a (F 14 C true = 0.5693). Therefore, leaching results in a statistically robust improvement compared to analyzing the same sample without leaching only if the true age is older than 4500 14C a. If the samples are younger F14C values obtained would be statistically indistinguishable.

Figure 4 Estimated limits of leaching. Lines show the theoretical difference (ΔF 14 C untreated-true ) between the expected (F 14 C true , obtained after leaching) and the measured value, if sample was not pretreated (F 14 C untreated ) for 3 scenarios. Solid line (A) the untreated foraminifera analysis contains contaminations determined in this study (f contamination = 0.027; F 14 C contamination = 0.9742); dashed line (B) relative contribution of contamination as A but of older isotopic composition (f contamination = 0.027; F 14 C contamination = 0.6000); and dotted line (C) twice the relative contribution of contamination with isotopic composition as A (f contamination = 0.054; F 14 C contamination = 0.9742). The gray shaded area depicts the ±2σ analytical uncertainty. If ΔF 14 C untreated-true is smaller than the 2σ analytical precision leaching would not result in a statistically significant different F14C value as analyzing the same sample untreated. Outside the 2σ area leaching would result in significantly different results.
On the one hand, the age beyond which leaching could potentially result in significantly improved radiocarbon ages, depends directly on the contamination contained in untreated samples. In scenario B the relative amount of contamination was kept constant, but its isotopic composition was changed (F 14 C contamination = 0.6) to simulate that the contamination was not derived from atmospheric CO2, but rather from aged surface contamination (e.g., clays or secondary carbonates). The results suggest that, if the contamination is isotopically older, leaching provides significant improvement only for samples older than ∼9500 14C a (F 14 C true = 0.3095). If the relative amount of contamination was double (scenario C) the limit would only be ∼2300 14C a (F 14 C true = 0.7469).
Further, the age limit depends on the analytical precision. The reported precision depends on sample specific 14C counting statistics (function of radiocarbon age and analysis time, limited by sample size) and additional variables like scatter of blanks and external uncertainty (see Wacker et al. (Reference Wacker, Christl and Synal2010) for full description of reported uncertainty). While one can assume that blank scatter and external uncertainty are roughly constant between analytical campaigns the counting statistics derived uncertainty will vary depending on the experimental setup chosen. The ±2σ uncertainty shown in Figure 4 was derived from numerous (n > 100) foraminifera analyses of constant size (∼100 µgC) and spanning nearly the entire range of F14C values reported by this laboratory. If the sample size was significantly smaller (< 20 µgC), the uncertainty is expected to increase as counting statistics derived precision is reduced. Reduced precision would shift the age limit, beyond which leaching results in significantly improved radiocarbon age, to older samples. On the contrary, analytical precision would significantly increase for larger, graphitized samples (∼1000 µgC) and would shift the limit to younger ages.
The provided age limits are not intended as strict guidelines. The calculations are based on a few measurements (n=10) spanning only a limited analytical window and will vary between laboratories, experimental setups and sample sizes. Additional paired leachate and leached analysis, especially for older samples, would greatly improve the estimated age limits, but are outside the scope of this work. Rather than defining strict limits, the intention is to highlight the limitations of leaching and to suggest a workflow of how these limits can be estimated.
Besides analytical precision, the beneficial effect of leaching is further limited by natural variability and sample heterogeneity. The standard deviation of the mean F14C values reported for young T. sacculifer replicate analyses greatly exceeded the analytical precision, highlighting that sample heterogeneity has a very large effect on the reliability of reported radiocarbon ages in line with findings by Dolman et al. (Reference Dolman, Groeneveld, Mollenhauer, Ho and Laepple2021) and Zuhr et al. (Reference Zuhr, Dolman, Ho, Groeneveld, Löwemark, Grotheer, Su and Laepple2022). Dolman et al. (Reference Dolman, Groeneveld, Mollenhauer, Ho and Laepple2021) reported on replicate small-scale (3–30 tests of foraminifera) radiocarbon analyses and could show that due to bioturbation variance of the measured F14C means is a function of sample size (number of tests per sample) and sediment accumulation rate. Thus, sample heterogeneity influences the true uncertainty of a radiocarbon analysis (variance between replicates), which likely exceeds the improvements possibly obtained by leaching of young foraminifera samples or the correction against 14C-free foraminifera. This is of particular importance as technically the MICADAS setup would allow to measure the radiocarbon age of individual foraminifera tests (Wacker et al. Reference Wacker, Lippold, Molnár and Schulz2013c). The data here show that, for young samples, the choice of material used for blank correction is trivial and the need for an additional leaching step is obsolete, if sample heterogeneity is not accounted for.
CONCLUSIONS
Radiocarbon dates of small-sized monospecific foraminifera analyzed on a MICADAS AMS were compared between different blank corrections and sample treatments. While for young foraminifera neither sample blank correction nor sample pretreatment showed significant differences between the means of replicate analyses, both had a significant effect on fossil samples. The data show that chemical pretreatment (leaching) does remove surface contamination (mainly stemming from adsorbed atmospheric CO2) and thus improves the reliability of reported radiocarbon ages. The same effect can be achieved, if untreated samples are corrected against a blank value determined from sample size-matched 14C-free foraminifera prepared and measured together with the samples, because contamination from atmospheric CO2 would affect samples and blanks alike. The data presented thus confirm that the Mollenhauer et al. (Reference Mollenhauer, Grotheer, Gentz, Bonk and Hefter2021) approach, omitting leaching by correcting against 14C-free foraminifera, is valid and provides equally reliable radiocarbon dates, despite the limitation that the 14C-free foraminifera used here are not mono-specific and from the same core (location) and might therefore not have experienced comparable diagenetic alterations as the samples.
Theoretical considerations further show that leaching only has a beneficial effect on radiocarbon data reliability for older samples, where the isotopic difference between untreated and chemically pretreated samples exceeds analytical precision. The age above which an improvement in radiocarbon age accuracy is to be expected crucially depends on the F14C of surface contamination, its relative contribution to total carbon, and the analytical precision; all these parameters may vary between laboratories and sample sets. The beneficial effect could further be masked, if natural variability and sample heterogeneity are not accounted for. While chemical pretreatment is certainly a suitable method to improve radiocarbon data accuracy within its limits, the process includes the risk of introducing an additional source of contamination and requires more extensive sample pretreatment. Blank correction against blank foraminifera is a suitable alternative to chemical pretreatment, as it provides equally reliable radiocarbon dates and at the same time reduces the risk of sample contamination and eases the workflow of processing foraminifera samples.
ACKNOWLEDGMENTS
We are grateful for the technical support and sample preparation efforts from Elizabeth Bonk, Heike Röben, and Torben Gentz. Funding for the MICADAS radiocarbon laboratory was provided through institutional core funding and HG was funded by the German Science Foundation within the Cluster of Excellence EXC 2077 “The Oceans Floor – Earth’s Uncharted Interface” (project number 390741603). We want to thank Dr. Julia Gottschalk and one anonymous reviewer for their helpful and constructive comments that helped improve the manuscript.