INTRODUCTION
Uncertainty as to the accurate value of the reservoir effect in radiocarbon (14C) dates is a considerable challenge to prehistorians, geneticists, etc. in their attempts at reconstructing the course of events in cultural and population history. The neolithization (introduction of food production based on domesticates of Middle Eastern origin) is an instance where such uncertainty is severely hampering scientific progress. During this transformation process, which took place over major parts of the Old World, fishing-hunting-gathering was replaced with cattle husbandry and cereal production alongside dramatic changes in material culture, ritual behavior and population genetics. Denmark has a central role in the study of this epoch-making episode (Fischer and Kristiansen Reference Fischer and Kristiansen2002). It took place in this country in the decades/centuries around 3950 BC or ca. 5100 14C years BP (Persson Reference Persson1999; Fischer Reference Fischer, Fischer and Kristiansen2002; Sørensen Reference Sørensen2014). Many of the radiocarbon dates essential to the study of the Mesolithic-Neolithic transformation process in Denmark are significantly influenced by the marine reservoir effect (e.g. Tauber Reference Tauber1981a and Reference Tauber1981b; Andersen Reference Andersen1991 and Reference Andersen1993; Persson Reference Persson1999; Price et al. Reference Price2007) and a clarification of the reservoir age applying specifically to the period and geographic area in question is therefore much needed. This applies, not least, to the sorting out of the relative and absolute chronology of the many human skeletons that are currently subject to genetic study aiming e.g. at population history reconstruction. The dietary protein intake from marine sources among these neolithization period individuals varied from ca. 0% to ca. 90% (Fischer et al. Reference Fischer, Olsen, Richards, Heinemeier, Sveinbjörnsdóttir and Bennike2007).
Oceans are depleted in 14C content relative to the coeval atmosphere. The apparent 14C age difference between oceanic surface water and the contemporaneous atmosphere at a single point in time is called the marine reservoir age, R(t) (Stuiver and Polach Reference Stuiver and Polach1977; Stuiver and Braziunas Reference Stuiver and Braziunas1993). Prior to the most recent update of the marine calibration curve (Marine20) the marine reservoir age, R(t), was estimated using a 2D box model representing the modelled global ocean (Reimer et al. Reference Reimer, Bard and Bayliss2013; Heaton et al. Reference Heaton, Köhler, Butzin, Bard, Reimer, Austin, Ramsey, Grootes, Hughen and Kromer2020). Thus with Marine13 the global reservoir age amounted to about 400 14C years and was roughly constant in time (e.g. Reimer et al. Reference Reimer, Bard and Bayliss2013). However, Marine20 is calculated using a 3D ocean general circulation model taking into account the temporal changes in the atmospheric 14C content as well as carbon cycle dynamics (Heaton et al. Reference Heaton, Köhler, Butzin, Bard, Reimer, Austin, Ramsey, Grootes, Hughen and Kromer2020). As a consequence, the global reservoir age, R(t), can no longer be considered constant. For the Holocene, R(t) varies between 380 and 674 14C years with an average offset of 500 ± 60 14C years. In the early Holocene prior to ca. 6000 BC and between ca. 3000 BC and 600 AD the global average reservoir age is ca. 465 14C years, whereas the period between 6000–3000 BC and from 600 AD towards the present are characterized by reservoir ages around 550 14C years (Heaton et al. Reference Heaton, Köhler, Butzin, Bard, Reimer, Austin, Ramsey, Grootes, Hughen and Kromer2020).
Previously a reservoir age of 400 14C years was frequently applied when correcting for marine reservoir effect in archaeological and geological samples from Holocene Denmark (e.g. Heinemeier et al. Reference Heinemeier, Nielsen and Rud1993; Ascough et al. Reference Ascough, Cook and Dugmore2009; Olsen et al. Reference Olsen, Dahlström and Poulsen2019; cf. Tauber Reference Tauber1990; Rasmussen Reference Rasmussen and Andersen2013). A confirmation of this approach may be seen in data from the neolithization period, retrieved from a sediment core in marine sediments at the site of Kilen (Figure 1), which those days had an effective exchange of water with the North Sea (Petersen Reference Petersen1975; Christensen et al. Reference Christensen, Cedhagen and Hylleberg2004). Three paired samples of marine shell and terrestrial botanical material of negligible biological own age from this site reveal a reservoir age of 351–393 14C years (Philippsen et al. Reference Philippsen, Olsen, Lewis, Rasmussen, Ryves and Knudsen2013).
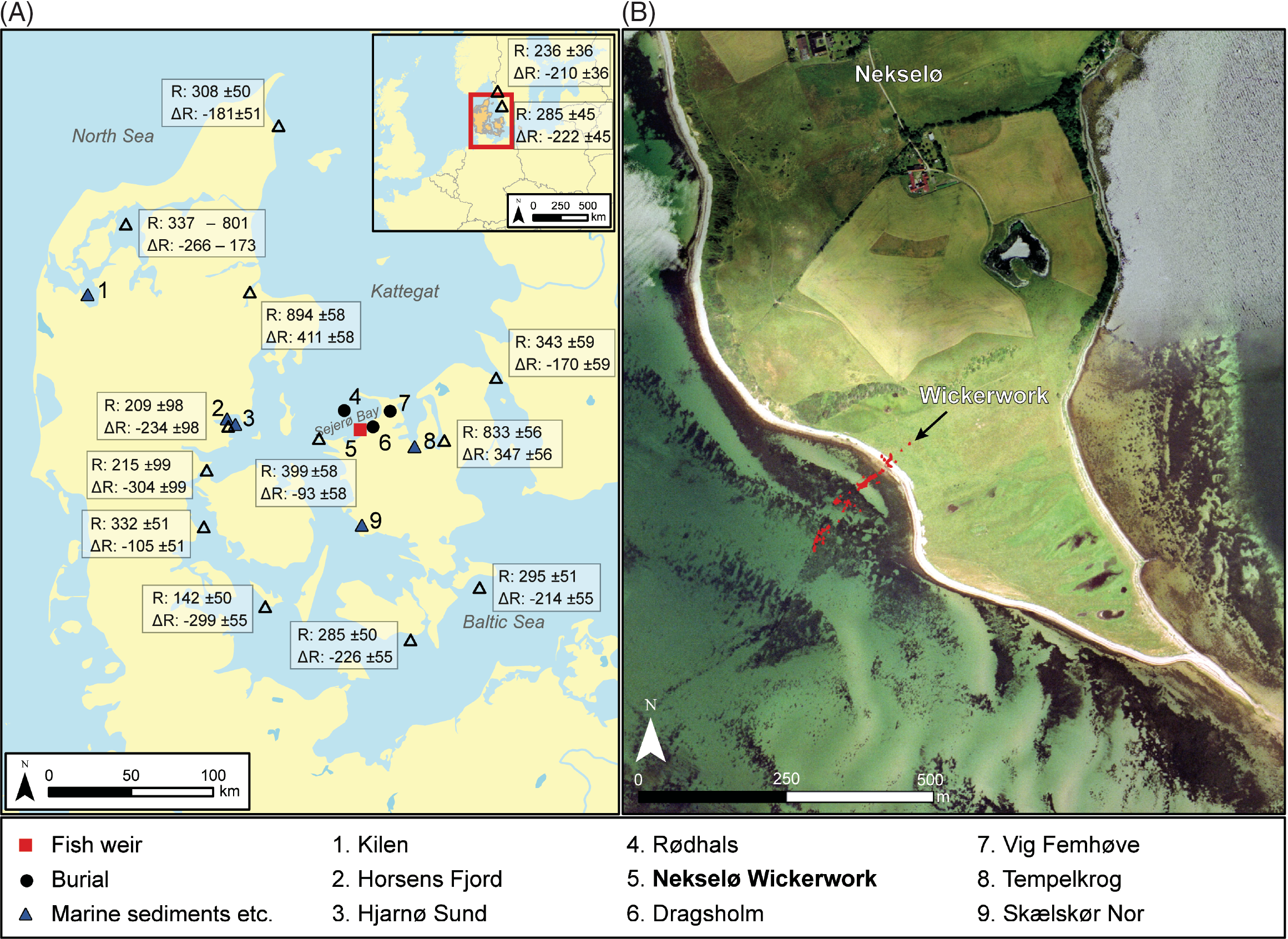
Figure 1 The location of the Nekselø Wickerwork and other sites and marine areas mentioned in the text. The reservoir ages indicated are extracted from the Marine Reservoir Database (http://calib.org/marine/) and derive from Håkansson (Reference Håkansson1969), Olsson (Reference Olsson1980), Heier-Nielsen et al. (Reference Heier-Nielsen, Heinemeier, Nielsen and Rud1995), and Lougheed et al. (Reference Lougheed, Filipsson and Snowball2013). Only suspension feeders are included, and all samples are collected between 1861 and 1952 AD. For sites/marine areas with multiple measurement the weighted average of both R and ΔR are shown. Insert to the right shows the present-day topography of the Wickerwork with a relative sea-level about 2.5 m lower than at the time of erection of the fish weirs.
For Danish marine areas, however, the modern reservoir age, R(t), is regionally highly variable, ranging between ca. 250 and 900 14C years (see Figure 1). The numerically highest values are seen in fjord environments with a considerable influx of 14C depleted groundwater. In contrast, values less than 400 years are recorded in the Danish archipelago (Kattegat region), where waters from the North Sea/North Atlantic and the Baltic Sea are mixing. The latter receives precipitation from substantial parts of northern Europe, resulting in a steady outflow of water with a reservoir age of 250–300 years into the Danish archipelago (Olsen et al. Reference Olsen, Ascough, Lougheed, Rasmussen, Weckström, Saunders, Gell and Skilbeck2017b; cf. Lougheed et al. Reference Lougheed, Filipsson and Snowball2013; Philippsen Reference Philippsen2018). In the neolithization period salinity in this archipelago was generally somewhat higher than now, possibly due to a higher exchange of water with the North Sea (Lewis et al. Reference Lewis, Ryves and Rasmussen2020). This most likely implies correspondingly higher reservoir ages in accordance with North Atlantic Ocean values. Because the changes between IntCal13 and IntCal20 overall are small, the above-mentioned reservoir ages, R(t), may still be considered accurate (Reimer et al. Reference Reimer, Bard and Bayliss2013, Reference Reimer, Austin and Bard2020).
To take into account regional effects such as deep-water upwelling or freshwater inputs the regional reservoir offset, ΔR(t), is defined as the deviations in reservoir age from the global marine calibration curve (Stuiver et al. Reference Stuiver, Pearson and Braziunas1986). A study based on 27 paired samples of terrestrial plant material and shells from marine mollusks respectively, derived from sediment cores of Holocene to recent date at three sites in fjords and inlets in the Danish archipelago, also produced highly varied estimates of reservoir age. The ΔR(t) values calculated using Marine09 for the relatively ocean-oriented sites of Horsens Fjord and Tempelkrog ranged from –80 to 360 and 145 to 400 14C years respectively, while Skælskør Nor ranged from 375 to 890 14C years. The significantly larger values of the latter site with its relatively restricted exchange of water with the open sea can, again, be explained as a result of a significant influx of groundwater (Olsen et al. Reference Olsen, Rasmussen and Heinemeier2009; Reimer et al. Reference Reimer, Baillie, Bard, Bayliss, Beck, Blackwell, Ramsey, Buck, Burr, Edwards and Friedrich2009). However, due to the methodological change in calculating the marine reservoir age taken for the Marine20 calibration curve these regional reservoir offset values are no longer valid and will need recalculating before they can be used with Marine20. Nonetheless, it can be concluded that the reservoir ages, R(t) and likely also the regional reservoir offset, ΔR(t), in Danish marine waters are both site-specific and time-specific (Olsen et al. Reference Olsen, Rasmussen and Heinemeier2009; cf. Lougheed et al. Reference Lougheed, Filipsson and Snowball2013; Olsen et al. Reference Olsen, Ascough, Lougheed, Rasmussen, Weckström, Saunders, Gell and Skilbeck2017b; Philippsen Reference Philippsen2018).
More recently, a study from Hjarnø Sund, a site in the Danish archipelago characterized by an effective water exchange with the open sea, has produced two paired dates. Both are based on a marine mollusk shell (Ostrea edulis) and a charcoal, respectively. In these cases, the age difference between the marine and the terrestrial sample is 410 ± 69 and 327 ± 70 14C years (Larsen et al. Reference Larsen, Philippsen, Skriver, Astrup, Borup and Mannino2018). Since both terrestrial samples derive from hazel wood (Corylus sp.) of unknown biological age there is a risk that these values might be decades too high. In addition, a risk of re-deposition of re-worked material from older sediments cannot be fully excluded—neither in this latter case nor the above-mentioned four Holocene cases—again potentially adding to the risk that the values mentioned exaggerate the marine reservoir age.
Such risks do not pertain to the Nekselø Wickerwork assemblage of paired marine and terrestrial samples presented below. Additionally, this site represents a more open water condition and should consequently be influenced by local reservoir effect factors to a lesser extent than seen in the above-mentioned studies. Like with the marine calibration curve the calculated reservoir ages from Nekselø apply to surface-near waters. Noteworthy, this is where almost all biological activity relevant to human diet will have come from.
The Wickerwork of Nekselø
During the final centuries of the Mesolithic and especially in the initial centuries of the Neolithic, weir fishing took place at a considerable scale off the southwest coast of the island of Nekselø in the Danish archipelago (Figure 1). It was probably migrating eel that were trapped in the up to 200-m-long wood constructions (Figures 2–3). The archaeological remains consist of numerous wattle panels made from rods of hazel in combination with hundreds of vertical supporting poles (Pedersen Reference Pedersen and Fischer1995, Reference Pedersen, Daire, Dupont and Baudry2013; Bartholin Reference Bartholin1996; Fischer Reference Fischer and Pedersen2006; Pedersen et al. Reference Pedersen, Fischer and Gregory2017, Reference Pedersen, Fischer, Bartholin, Fischer and Pedersen2018). After having been hidden in marine sediments for millennia the wood constructions saw the light of day again some years ago due to sea floor erosion (Fischer Reference Fischer, Benjamin, Bonsall, Pickard and Fischer2011).

Figure 2 Wattle panel C1 of the Wickerwork. In the Neolithic it was part of a fish weir that was knocked down by the sea and rapidly covered in marine mud so that oysters could no longer live on the wood: (A) overview during clearing up of the panel after recent sea-floor erosion; (B) close-up of a part untouched by present-day erosion—to some extent, however, with scars and loss of bark arisen during excavation. When revealed by archaeologists, these hazel rods were preserved in such good condition that their bark had still kept much of its original color and texture. The unbroken deposits of silty gyttja from the stakes upward support the impression of a rapid and permanent covering in sea-bed sediments. (Photos: Anne Marie Eriksen, Danish National Museum 2016.)
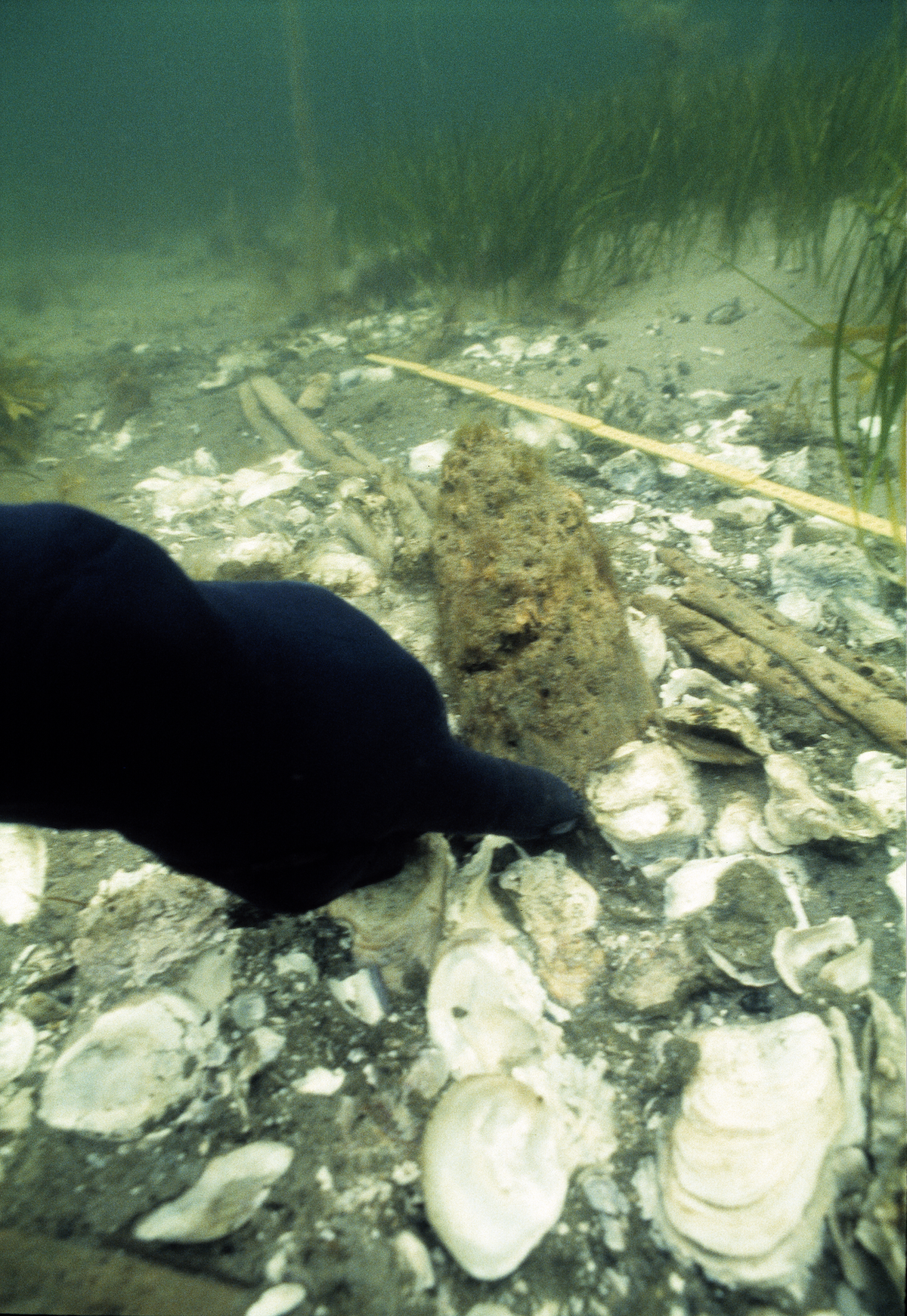
Figure 3 Wattle rods and a still vertical supporting pole of weir panel E, surrounded by shells of oyster that thrived on the fish weir during its use period in the Neolithic. The diver’s finger points towards a paired set of shells from an individual still attached to a vertical pole (No. 904 in Table 1). Prior to taking the photo, a growth of algae was picked off the pole for the sake of visibility. Microscopic rootlets from this vegetation may have penetrated the outer part of the wood and subsequently become part of the samples dated. (Photo: Anders Fischer 1998.)
The landscape characteristics in the vicinity of the Nekselø Wickerwork imply that the local supply of freshwater and ground water to the site was minimal. Moreover, the weirs were built into open sea at the most wind and wave exposed side of the island, thus granting an effective mixing of waters (Figure 1).
The 18 previously published 14C dates of constructional wood from the fish weirs at the site span the interval of time ca. 5600–4300 14C BP (Fischer Reference Fischer and Milner2007; Fischer and Jensen Reference Fischer, Jensen, Fischer and Pedersen2018). In South Scandinavian archaeological terminology this period is equivalent with the final part of the Late Mesolithic Ertebølle Culture and most of the chronological range of the subsequent Neolithic Funnel Beaker Culture (Nielsen Reference Nielsen, Hvass and Storgaard1993; Fischer Reference Fischer, Fischer and Kristiansen2002).
Oyster (Ostrea edulis) thrived at the Nekselø Wickerwork. This species prefers saline conditions and demands a firm substrate with little silting on which to settle (Milner Reference Milner2002: 10; Lewis et al. Reference Lewis, Ryves, Rasmussen, Olsen, Knudsen, Andersen, Weckström, Clarke, Andrén and Juggins2016), and the fish weirs served this need very well (Figure 3). On the surface of oyster valves from the site a round-bottom furrow is often seen. A couple of especially well-defined examples are shown in Figure 4. They represent the contact region between mollusk and constructional wood.

Figure 4 Stray-found oyster shells (lower/proximal valves) found near panels C1 and C2. Their surfaces show a well-defined furrow, which is the negative of the constructional wood on which these mollusks originally grew. (Photo: Anders Fischer.)
Central to the present study are two wattle panels (Figure 2), that were joined together by the tongue-and-groove principle (cf. Pedersen Reference Pedersen, Pedersen, Fischer and Aaby1997) and consequently are absolutely contemporaneous. Originally, they were part of a ca. 4-m-high fence on the seabed. This construction is recorded in places of erosion at a straight line over a distance of some 40 m. Additional data for this study derives from a nearly upright-standing wickerwork (section E, Figure 3), located some further 40 m out in the sea.
The aforementioned pair of panels, labeled C1 and C2, were located more than 100 m from the coeval seashore. Rough weather made them tip over and lay down horizontally (Figure 2). The remarkable preservation of their hazel rods implies that during their time of use they were only directly exposed to sea water for a short while. Otherwise, the disintegrating actions of bacteria, fungi, crustacea, etc. (cf. Gregory and Matthiesen Reference Gregory, Matthiesen, Fischer and Pedersen2018) would have altered their surfaces significantly (Figure 2B). The 4 years of growth—inferred by direct visual inspection of the hinges and surfaces of the shells of two oyster that had lived on these panels—could very well represent the whole length of time between the erection and capsizing of the construction, the latter event causing the mollusks to suffocate.
The wooden building materials and the oysters sitting on them are not absolutely contemporaneous. When judging the scale of this time gap the following aspects should be considered: wood anatomical inspections of a large assemblage of rods and poles from the Wickerwork have revealed that felling took place in late winter/early spring (Pedersen et al. Reference Pedersen, Fischer and Gregory2017). Experience from practical experiments (cf. Pedersen and Jørgensen Reference Pedersen and Jørgensen2006) suggests that the weaving of stakes into panels began soon after. Their installation in the water could wait until the close of summer, when eels from the Baltic Sea and the Danish archipelago began to migrate towards their spawning ground in the Atlantic (Pedersen Reference Pedersen, Daire, Dupont and Baudry2013). Present-day experiments indicate that if stored on land for a year hazel panels will lose resilience to a degree that would make them unfit as constructional elements in weirs for eel fishing in the open sea. Additionally, written sources on weir fishing in northern Europe in historical time indicate that when used in sea water hazel panels usually had to be replaced within a maximum of 4 years (Schmelling Reference Schmelling1971, 112; cf. Brinkhuizen Reference Brinkhuizen1983). In sum, we can expect a time gap of a half to four years between the harvest of the rods in the Nekselø Wickerwork and the beginning of the growth of the oysters found on the wattle.
Sampling and Dating Wood and Oyster Shells
Three paired sets of oyster shells were observed still firmly attached to the constructional wood that once served as their living place. Two of these relate to wattle panels C1 and C2 (Figure 5A). The third pair of solidly attached shells was found on a supporting pole by panel E (Figures 3 and 5B). The potential of using these combinations of shell and wood for high precision estimates of marine reservoir age was realized instantaneously. Field and laboratory procedures could therefore be arranged to make the most of the opportunity. The strategy included a concern with what uncertainties were at play relating to biological own age, recent contamination, etc.

Figure 5 Examples of dated materials: (A) a paired set of oyster shells attached to the wickerwork of panel C2; (B) the lower (cupped) and upper (flat) valve of the oyster shown in Figure 3 and the outer ca. four year-rings sliced off from the pole on which the mollusk once grew. The negative of the pole is seen on the uppermost right part of the cupped valve. (Photos: Anne Marie Eriksen [A] and Anders Fischer [B].)
When removed from their in situ position each shell and each portion of constructional wood was placed in a separate plastic bag. A bit of sea water was added to the wood samples to avoid collapse of the wood structure. Subsequently standard procedures of storage under dark and cold conditions with no access for atmospheric oxygen were followed for the purpose of minimizing the growth of microorganisms. No preservatives were involved. Wood species determination was performed on micro slices of the samples observed via a standard microscope. Likewise, the season of harvest was determined on meticulously cut sections of the outermost year rings. During the subsequent slicing out the youngest year rings for radiocarbon dating an estimate was made on the average biological own age of each sample. Care was taken that the material selected did not include sediment or macroscopic biological material from present-day sea weeds, etc. Using the outermost year rings may, however, have introduced a risk that wood samples were contaminated by recent microscopic substances such as fungi, bacteria and rootlets. This risk clearly applies to a sample from panel E, taken from a vertical pole that had been eroded out of the marine gyttja and exposed directly to light and oxygen-rich sea water, most likely for several years prior to sampling (Figure 3). As to the shell samples such a risk of contamination from recent organisms was eliminated through physical and chemical purification procedures, see below.
The collection of samples for estimating the marine reservoir age at the Nekselø Wickerwork took place in two rounds. During a swift inspection of the site in 1998 a vertical pole with an intact pair of oyster shells attached to it was lifted (Figures 3 and 5B). Parts of the mollusk and the pole was shipped to the dating laboratory in Trondheim, where 14C measurements of relatively small samples could be performed via the radiometric decay counting method. Panel C1 was also observed during this diving inspection. As a consequence of its special research potential it was covered with a protective top of geotextile and sand bags (Pedersen et al. Reference Pedersen, Fischer and Gregory2017; Skriver et al. Reference Skriver, Galili, Fischer, Fischer and Pedersen2018). These were removed for a couple of days in 2016, during which parts of C1 and the previously unknown panel C2 were exposed and samples for wood anatomical analysis and AMS dating were collected (Figure 2). The resulting new samples were handed over to the AMS Centre in Aarhus.
At the dating laboratories, shell samples were mechanically cleaned and subsequently purified using demineralized water in an ultrasonic bath. Next, the outer 20–25% of the shells were removed using 1M HCl at room temperature. Finally, the samples were dissolved in 80% H3PO4 in evacuated seal-caped glass tubes. Wood samples were pretreated using an acid-base-acid (ABA) pretreatment method (1M HCl, 1M NaOH at 80°C and lastly 1M HCl at room temperature), and the ones dealt with at the Aarhus AMS Centre (AARAMS) were combustion in sealed evacuated quartz tubes containing 200 mg pre-cleaned CuO. Subsequently, they were graphitized using the H2 reduction method and an iron catalyst (e.g. Vogel et al. Reference Vogel, Southon, Nelson and Brown1984) and 14C analyzed using a 1MV Tandetron accelerator (cf. Olsen et al. Reference Olsen, Tikhomirov, Grosen, Heinemeier and Klein2017a).
The radiocarbon results (Table 1) are reported as conventional 14C dates BP and are corrected for fractionation by normalizing to –25‰ using online 13C/12C ratios (Stuiver and Polach Reference Stuiver and Polach1977), while radiocarbon ages were converted to calendar years using OxCal 4 and IntCal20 (Ramsey et al. Reference Ramsey, Dee, Lee, Nakagawa and Staff2010; Reimer et al. Reference Reimer, Austin and Bard2020). It should be noted that the 14C ages obtained for oyster shells are remarkably alike. In contrast, the dates for wood samples display larger variation—a situation that is explained by the fact that the two statistical outliers both derive from wood that had been exposed by present-day erosion and could therefore have been contaminated by microscopic material of recent date (cf. caption to Figure 3).
Table 1 Dates of oyster shells and the wood on which they once grew. The paired samples of shell and wood from panels C1 and C2 were observed in the field to be physically directly connected. They are, therefore, in principle contemporaneous. The same applies to the samples from panel E. When counting in details, the reservation has to be taken that the oysters could not begin to live before their wooden substrate had been harvested and installed. Samples marked with * are outliers and are not included in the summary statistics, written in bold font.

Reservoir ages (R) are calculated using paired samples of atmospheric and marine origin by subtracting the 14C age of wood (atmospheric) samples from the 14C age of shell (marine) samples. The error on these values are found using propagation of errors. Combined 14C ages are calculated as weighted mean values and the validity of the weighted mean is considered using reduced χ2 statistics. The χ2 is passed if and only if X≤Y where X is the χ2 of the sample and Y the 95% χ2 limit (Bevington and Robinson Reference Bevington and Robinson2003).
Regional reservoir age offsets (ΔR) are calculated by resampling of the calendar year (θ) probability distribution provided by the wood samples using 5000 random points. Each of the resampled calendar years, θi, is then translated into a marine 14C age, Mi, representing the global marine 14C content at calendar year θi. Thus Mi is estimated from Marine20 as Mi=N(μMarine20(θi),σMarine20(θi)) where μ and σ are the average and uncertainty of Marine20 at calendar year θi and N is the normal distribution. The marine sample represented here as the shell samples are drawn from a normal distribution S = N(μSample,σSample) where μ and σ are the 14C value and associated error of the shell sample. ΔR is then calculated as: ΔRi = Mi – Si. The skewness and kurtosis of the resulting ΔR histogram is used to estimate if the ΔR probability distribution resembles a normal distribution (skewness = 0, kurtosis = 3) and if so then the ΔR value is estimated as the mean and standard deviation of the individual 5000 ΔRi values. Calculations of the regional reservoir age offsets (ΔR) are performed using MatLab 2020 (version 9.8).
Calculating Reservoir Age
From panels C1+C2 three oyster and five wood samples have been 14C dated (Table 1). The age determination of AAR-11619 statistically disagrees with the other four wood 14C ages. For this reason, it is considered an outlier, probably a result of the above-mentioned risk of contamination by recent microscopic substances. The combined 14C age of the remaining four wood samples is 4531 ± 12 14C BP (χ2 2.4≤2.6). Correspondingly, the combined 14C age of the three shell samples is 4790 ± 17 (χ2 0.0≤3.0). Thus, for the physically connected panels C1 and C2 the age difference between marine and terrestrial samples, i.e., the reservoir age, R, is 259 ± 27 14C years.
From panel E the combined value for oyster (T-18054 and AAR-29246) yields 4792 ± 47 14C BP (χ2 0.0≤3.8). The wood sample T-18053 is for statistical reasons considered an outlier, cf. above mentioned risk of recent biological contamination. Thus, the age difference between marine and terrestrial samples for this panel is calculated 307 ± 32 14C years.
In case panels C1+C2 and E represent independent—although chronologically close—building episodes, the combined reservoir age of these events is 273 ± 18 14C years. If alternatively, panels C and E are from one and the same construction event, as would seem possible from the archaeological evidence, we reach the slightly different result of 289 ± 24 14C years by making a weighted average of all wood samples (4502 ± 12 14C years, χ2 9.0≤9.5) and all shell samples (4791 ± 21 14C years, χ2 0.0≤9.5). Because, the inference of contemporaneity is open to discussion, we favor the value of 273 ± 18 14C years.
The regional reservoir age offset, ΔR, is shown in Figure 6. The resulting skewness of the ΔR histograms range between –0.28 and –0.07 indicating a tailing towards lower ΔR values in all histograms. The calculated kurtosis is close to 3, which together with the low skewness values suggests that each ΔR probability distribution can be assumed normal distributed. Hence the ΔR for Panel C1+C2, Panel E and all panels combined are estimated from the mean and standard deviation of the ΔR histograms and amounts to –243 ± 90, –228 ± 84 and –226 ± 80 14C years, respectively (Figure 6). The combined ΔR value of panels C1+C2 and E, assuming independent episodes, is calculated as the weighted mean of the ΔR value of Panel C1+C2 and Panel E (Table 1).

Figure 6 (Left) Calibrated probability distributions of the wood samples, using OxCal 4.4 (Ramsey et al. Reference Ramsey, Dee, Lee, Nakagawa and Staff2010) and IntCal20 (Reimer et al. Reference Reimer, Austin and Bard2020). (Right) Regional reservoir age offsets, ΔR, calculated using Marine20 (Heaton et al. Reference Heaton, Köhler, Butzin, Bard, Reimer, Austin, Ramsey, Grootes, Hughen and Kromer2020).
For the Mesolithic Dragsholm double inhumation (see below) the regional reservoir offset is calculated using the terrestrial red deer bone (AAR-7417) as proxy for the calendar age probability distribution (Table 2). The combined 14C age of individual A and B of 6254 ± 28 14C years BP is considered the best estimate for calculating the reservoir age, R, of 297 ± 43 14C years (Table 2). As a proxy for the marine 14C content we add the reservoir age, R, of 297 ± 43 14C years to the red deer bone 14C age (AAR-7417) yielding 6280 ± 57 14C years BP from which we derive a ΔR value of –263 ± 99 14C years (skewness = 0.0, kurtosis = 3.0).
Table 2 Cross dates from the Late Mesolithic Dragsholm double inhumation, based on the one hand on a burial gift of terrestrial material and on the other hand human skeletal material from two females, both representing a high intake of marine food. AMS dates and stable isotope measurements from Price et al. (Reference Price2007). Calculations of marine contribution to protein diet and of marine reservoir age based on the C+N model presented in Fischer et al. (Reference Fischer, Olsen, Richards, Heinemeier, Sveinbjörnsdóttir and Bennike2007).

DISCUSSION
14C age determinations of humans and animals, the diet of which derived partly or fully from aquatic environments, often have such a large reservoir age offset that a correction is mandatory to make use of them (e.g., Fischer Reference Fischer, Fischer and Kristiansen2002; Olsen et al. Reference Olsen, Heinemeier, Lübke, Lüth and Terberger2010, Reference Olsen, Dahlström and Poulsen2019; Wood et al. Reference Wood, Higam, Buzilhova, Suvorov, Heinemeier and Olsen2013; Martindale et al. Reference Martindale, Cook, McKechnie, Edinborough, Hutchinson, Eldridge, Supernant and Ames2018). When reservoir correcting and calibrating 14C dates of such individuals it is necessary to reconstruct their dietary habits using stable isotope analysis (e.g. Fischer et al. Reference Fischer, Olsen, Richards, Heinemeier, Sveinbjörnsdóttir and Bennike2007; Olsen et al. Reference Olsen, Heinemeier, Lübke, Lüth and Terberger2010; Fernandes et al. Reference Fernandes, Grootes, Nadeau and Nehlich2015; King et al. Reference King, Snoddy, Millard, Grocke, Standen, Arriaza and Halcrow2018). A further requirement is to know the reservoir age of the specific place and time accurately. We consider the reservoir age, R(t), of 273 ± 18 14C years and the corresponding reservoir age offset, ΔR(t), of –234 ± 61 14C years for The Wickerwork the currently best estimate available for the open waters of the southern Kattegat region during the neolithization period.
In this connection we return to the data from the above mentioned study conducted on samples from three fjords/inlets of the Danish archipelago (Olsen et al. Reference Olsen, Rasmussen and Heinemeier2009). If we focus not on the extremes but the medians of the reservoir age, R(t), it is interesting to note that the values for the two sites with an effective exchange of water with the open sea is 250 ± 119 14C years (Horsens Fjord) and 231 ± 46 14C years (Tempelkrog), while the value for the site with a more restricted water exchange is 565 ± 57 14C years (Skælskør Nor) (excluding samples based on shells from the species of Scrobicularia plana and Hydrobia, which are deposit-feeders, and who will therefore most likely include carbon older than their actual time of life). Consequently, a reservoir age, R(t), in the order of what is obtained from the Nekselø Wickerwork may be typical for the more ocean-exposed parts of the Danish archipelago.
The reservoir offset, ΔR(t), of –234 ± 61 14C years is comparable with the modern (1861–1952 AD) estimates of the ΔR values around Kattegat and the Baltic Sea ranging between –170 and –299 14C years (Figure 1). The recently updated Marine20 calibration curve includes carbon cycle dynamics and therefore the global reservoir age, R(t), is time variable (Heaton et al. Reference Heaton, Köhler, Butzin, Bard, Reimer, Austin, Ramsey, Grootes, Hughen and Kromer2020). Thus, because the carbon cycle is included in the marine calibration curve, it is tempting to conclude that the ΔR(t) values in the Kattegat region are time invariable. However, both the modern and Neolithic ΔR values are calculated for periods where the global reservoir age, R(t), is ca. 550 14C years, the period in-between is characterized by lower R(t) values around 465 14C years (Heaton et al. Reference Heaton, Köhler, Butzin, Bard, Reimer, Austin, Ramsey, Grootes, Hughen and Kromer2020). Additionally, the marine calibration curve represents the global ocean and in contrast the Baltic Sea and Kattegat are influenced by regional hydrological and geological processes which are not taken into account and which cannot be assumed synchronous with the changes included in Marine20 (e.g. Zillén et al. Reference Zillén, Conley, Andrén, Andrén and Björck2008). Thus, further research is needed into the ΔR(t) variability of the Kattegat and Baltic Sea region to investigate the time dependency of the regional reservoir offset.
It is also worth returning to results from the study of a Late Mesolithic inhumation burial at Dragsholm (Petersen Reference Petersen1974, Reference Petersen, Sulgostrowska and Tomaszewski2008) (not to be confused with the Early Neolithic male burial from same place, mentioned below). The site is located within the Danish archipelago and at the time of its construction it lay directly above the beach. It contained the well-preserved skeletons of two females and a burial gift made of terrestrial material—a spatula produced from bone of red deer. Samples of the skeletons and the spatula were subject to several rounds of 14C dating and measuring of stable isotopes (Price et al. Reference Price2007). The δ13C values showed that both humans had mainly subsisted on marine food. Model-based calculations of the marine reservoir effect pertaining to them range between 212 ± 60 and 362 ± 63 14C years (Table 2). The average reservoir age, R(t), calculated on data from the samples most intensely cleaned for preservatives is 297 ± 43 14C years—i.e., in agreement with reservoir age values of the Wickerwork of Nekselø.
The combination of results from Dragsholm and Nekselø indicates that the marine reservoir age for these two archaeological find spots did not differ essentially during a period of several centuries around the Mesolithic-Neolithic divide. This may not be surprising, since the two sites are located within a distance of only 6 km, and originally, they were both directly connected with/part of the Sejerø Bay. This result leads us to conclude that a relatively certain reservoir corrected radiocarbon based chronological sorting of neolithization period human skeletons from the surroundings of this bay should be possible. Among these skeletons are several that are fundamental to the current debate on the neolithization of Denmark, such as:
-
Rødhals Man, who is considered a late survivor of the indigenous Mesolithic (Ertebølle Culture) population (Fischer Reference Fischer, Fischer and Kristiansen2002);
-
Dragsholm Man, who according to his burial gifts as well as the presently available radiocarbon dates and dietary stable isotope measurements is one of the earliest known members of the local Early Neolithic farming society (Funnel Beaker Culture) (Fischer Reference Fischer, Fischer and Kristiansen2002);
-
Early Neolithic individuals, possibly representing the elite among the local Funnel Beaker Culture population, who were interred in the Vig Femhøve dolmen (Sjögren and Fischer Reference Sjögren and Fischerin prep.).
CONCLUSION
The radiocarbon assemblage from the Nekselø Wickerwork has the special quality of extensive replication of dates representing a chronologically sharply restricted event. We have six marine and eight terrestrial 14C dates directly relating to one and the same incident. Consequently, it is possible to conduct in-depth source critical evaluation of age determinations to an unusual extent. In this way a solid estimate on marine reservoir age in prehistoric time has been reached: 273 ± 18 14C years.
Our re-evaluation of previously produced geological and archaeological data from marine environments in the Danish archipelago north of the Baltic Sea generally indicate similar reservoir ages. We acknowledge that each point in time and space may have its own aquatic reservoir age. Based on the data presently available, we on the other hand, judge the ca. 273 years to be a more realistic estimate than the previously favored ca. 400 14C years when correcting for reservoir age in radiocarbon dates of neolithization period marine samples from open sea exposed sites in the Danish archipelago. The reservoir age estimate from the Wickerwork is directly applicable in culture-historical and genetic studies of human skeletons and burials essential to the ongoing debate of the neolithization of Southern Scandinavia.
ACKNOWLEDGMENTS AND CREDITS
The Nekselø Wickerwork is recorded in the Danish Agency for Palaces and Culture’s national register of sites and monuments under number 401263-3. Field observations mentioned are primarily thanks to a registration and condition assessment program for underwater cultural heritage, conducted under the auspices of the National Forest and Nature Agency, Denmark. Some of the samples presented in the present paper were salvaged during these activities of the 1990s. Others were brought to the surface thanks to a subsequent condition assessment project, organized by David Gregory of the Danish National Museum in cooperation with the first author of the present paper. The latter activity was funded by the Danish Agency for Palaces and Culture. We thank Torben Malm of the mentioned agency for his leading role in the practical work of revealing wattle sections C1 and C2. Additionally, Anne Marie Eriksen of the National Museum of Denmark is thanked for photo documentation and participation in the partial excavation of these two wattle sections in 2016. Likewise, we thank Thomas Bartholin for wood anatomical determinations of samples from the Nekselø Wickerwork, Steinar Gulliksen for the analytical activities conducted at the Trondheim Radiocarbon dating laboratory, and Nicky Milner for advice on estimating biological age of oyster shells. Peter Moe Astrup of Moesgaard Museum kindly informed us on finds conditions etc. of two charcoal samples from Hjarnø Sund, discussed in the text. Moreover, we thank Rich Potter of University of Gothenburg’s Department of Historical Studies, for producing Figure 1, including the integration of its part B, which was originally produced by Thomas Eriksen of the Danish Agency for Culture on the basis of the Danish Cadastra Office’s ortophoto from 1999. Additionally, Karl-Göran Sjögren, Douglas Price and an anonymous peer reviewer are thanked for critical reading and constructional commenting on previous versions of the manuscript.
The paper is a spin-off from a genetical cum archaeological project directed by Eske Willerslev and Kristian Kristiansen, funded by Svenska Riksbankens Jubileumsfond, within the project “Towards a new European Prehistory. Integrating aDNA, isotopic investigations, language and archaeology to reinterpret key processes of change in the prehistory of Europe”.