Disturbed sleep is a key symptom in the pathophysiology of schizophrenia and appears as a prodromal sign prior to the first and also recurring psychotic episodes. Individuals with schizophrenia suffer from sleep-onset and sleep-maintenance insomnia, regardless of whether they are untreated or treated with antipsychotics and irrespective of the clinical course of the disorder, although atypical antipsychotics alleviate sleep disturbances better than first-generation drugs. Reference Monti and Monti1,Reference Monti and Monti2 In fact, most studies have found reduced sleep efficiency and total sleep time, as well as increased sleep latency. Reference Cohrs3 Inconsistent results have been reported for other sleep variables such as the duration or percentage of slow-wave sleep, stage 2 or rapid-eye movement (REM) sleep regulation, such as REM latency or density. Reference Benca, Obermeyer, Thisted and Gillin4,Reference Chouinard, Poulin, Stip and Godbout5 Sleep disturbances in individuals suffering from chronic schizophrenia have been discussed as a consequence of D2 dopamine receptor and 5-HT2 serotonin receptor occupancy. Reference Yamashita, Morinobu, Yamawaki, Horiguchi and Nagao6,Reference Staedt, Dewes, Danos and Stoppe7 In the circadian domain, there is relatively scarce information concerning the circadian organisation of sleep–wake cycles in people with schizophrenia, such as delayed sleep phase, free running components and fragmented sleep with enhanced daytime napping. Reference Wirz-Justice, Cajochen and Nussbaum8–Reference Wulff, Joyce, Middleton, Dijk and Foster10 These irregularities were more prominent in individuals treated with typical than with atypical antipsychotics. Reference Wirz-Justice, Cajochen and Nussbaum8,Reference Wirz-Justice, Haug and Cajochen9 Given that synchronisation of the sleep–wake cycle to the 24-h day is determined by zeitgebers such as light or social cues, individuals with schizophrenia who often withdraw from social life may be exposed to insufficient daylight, which in turn can lead to circadian rhythm disturbances. Endogenous melatonin, a neurohormone synthesised in the pineal gland and whose secretion is strictly controlled by the internal clock located in the suprachiasmatic nuclei, serves as a ‘hand of the clock’ and represents the best marker for circadian rhythms in humans. Measuring circadian rhythms of melatonin in 89 participants with schizophrenia, Rao and colleages Reference Rao, Gross, Strebel, Halaris, Huber and Bräuning11 found a significant circadian phase advance of 1.2 h compared with healthy controls. In addition to an abnormal circadian melatonin phase, one case study has documented an individual with schizophrenia with a melatonin rhythm period longer than 24 h, thus not entrained to the 24-h solar day. Reference Wulff, Joyce, Middleton, Dijk and Foster10 Another case study in a person with schizophrenia under controlled ‘constant bed rest’ laboratory conditions for more than 30 h reported advanced circadian timing of melatonin and core body temperature, but a delayed rhythm of sleep propensity. Reference Wirz-Justice, Cajochen and Nussbaum8 Furthermore, in one, Reference Monteleone, Maj, Fusco, Kemali and Reiter12 but not in another study, Reference Rao, Gross, Strebel, Halaris, Huber and Bräuning11 nocturnal melatonin levels were lower in people with schizophrenia than in healthy people. Studies on melatonin secretion in people with schizophrenia have to be interpreted with caution, since effects of antipsychotic medication per se on melatonin levels are not yet known, with the exception of a study showing that olanzapine did not significantly alter melatonin levels in individuals with schizophrenia who had predominantly negative symptoms. Reference Mann, Rossbach, Muller, Muller-Siecheneder, Pott and Linde13
Disorders of the circadian system can result in circadian misalignment, which itself causes sleep disturbances, reduced attention, impaired daytime alertness, lack of energy, memory problems and negative mood. Interestingly, all these symptoms occur in people with schizophrenia. In particular, the prefrontal cortical networks, which are involved in the cognitive control for goal-oriented tasks and inhibition of contextual and emotional distractors, Reference Banich, Mackiewicz, Depue, Whitmer, Miller and Heller14 are affected in people with schizophrenia Reference Silver, Feldman, Bilker and Gur15,Reference Maldonado-Aviles, Curley, Hashimoto, Morrow, Ramsey and O'Donnell16 and are highly susceptible to alterations in circadian rhythms and sleep. Since diminished cognitive ability is a core feature of schizophrenia, and circadian and sleep disturbances are also prevalent in people suffering from schizophrenia, it is of substantial clinical relevance to investigate the relationship between the psychophysiological systems of circadian time-keeping, sleep and cognitive functioning. The following three hypotheses were tested during a 3-week long actimetry recording. First, whether people with schizophrenia show abnormal circadian rest–activity cycles, as indexed by reduced relative amplitude between day and night-time activity, reduced interdaily stability and increased intradaily variability. Second, whether cognitive domains, such as attention, speed of processing, executive functioning and verbal fluency are impaired and correlate with the severity of the circadian rhythm disruption. Finally, whether the severity of circadian rhythm disruption is a better predictor for cognitive functioning than other conventional variables such as severity of schizophrenic symptoms, duration of illness, medication and so forth.
Method
Participants
Fourteen in- and out-patients diagnosed with schizophrenia on stable antipsychotic medication for at least 2 months prior to the study commencing were recruited at the Psychiatric Hospital of the University of Basel, Switzerland. The cohort comprised 13 men and 1 woman aged 28–56 years (mean age 39.9 years, s.d. = 9.6). Five were in-patients hospitalised in the general psychiatry and forensic departments, three were day clinic patients, and six were out-patients without a regular work schedule. The diagnosis of schizophrenia was 14.2 (s.d. = 10.9) years prior to the study. All participants were taking antipsychotics (Table 1). Three people were taking additional medication (SCH06: lorazepam, esomeprazole, methadone; SCH07: escitalopram, lorazepam, sodium valproate; SCH08: diazepam, sodium valproate, biperiden, methadone). The main comorbidity was substance misuse in lifetime prior to the study; mainly cannabis (n = 9), and opiates and cocaine (n = 3). Potential participants with clinically relevant alcohol and drug consumption and lack of adherence were excluded. If included in the study, individuals were reimbursed for their participation according to number of completed study parts. The study procedure was approved by the local Ethics Committee of Basel, Switzerland (EKBB), and all procedures conformed to the Declaration of Helsinki.
Table 1 Sociodemographic data listed by rank of relative amplitude

Participant | Gender | Age, years | Illness duration, years | Body mass index, kg/m2 | Living situation | Antipsychotics | Chlorpromazine equivalent | Relative amplitude |
---|---|---|---|---|---|---|---|---|
SCH13 | Male | 31 | 10 | 31 | In-patient | Quetiapine and haloperidol | 1816 | High |
SCH08 | Male | 40 | 26 | 27 | Day clinic | Aripiprazole and risperidone | 500 | High |
SCH10 | Male | 30 | 1 | 25 | Day clinic | Olanzapine | 300 | High |
SCH12 | Male | 43 | 5 | 26 | In-patient | Risperidone | 200 | High |
SCH04 | Male | 50 | 25 | 26 | Out-patient | Risperidone | 150 | High |
SCH11 | Male | 28 | 1 | 23 | Day clinic | Aripiprazole and zuclopenthixol | 367 | High |
SCH15 | Male | 43 | 24 | 32 | In-patient | Olanzapine | 400 | High (>0.85) |
SCH05 | Male | 30 | 4 | 34 | Out-patient | Risperidone | 100 | Medium (<0.85) |
SCH01 | Male | 56 | 27 | 24 | Out-patient | Risperidone | 200 | Medium |
SCH14 | Male | 37 | 17 | 38 | In-patient | Quetiapine and aripiprazole | 800 | Medium |
SCH02 | Female | 30 | 3 | 20 | Out-patient | Penfluridol | 300 | Medium (>0.7) |
SCH07 | Male | 55 | 31 | 28 | Out-patient | Risperidone | 150 | Low (<0.7) |
SCH09 | Male | 48 | 18 | 25 | In-patient | Flupentixol and levomepromazine | 1350 | Low |
SCH06 | Male | 38 | 7 | 39 | Out-patient | Risperidone depot | 600 | Low |
Clinical status: interviews and questionnaires
To assess the clinical status of the participants, the Positive and Negative Syndrome Scale (PANSS) Reference Kay, Fiszbein and Opler17 was rated by two psychiatrists at the Psychiatric Hospital by conducting the structured clinical interview for PANSS (SCI–PANSS) Reference Kay, Opler, Fiszbein, Gerhold, Huss and Luecke18 and reviewing medical records. Symptom severity was also rated on the Brief Psychiatric Rating Scale (BPRS) Reference Overall and Gorham19 and the Clinical Global Impression (CGI) scale. Reference Guy20 In addition, the Simpson–Angus Scale (SAS), an instrument for antipsychotic-induced Parkinsonism, rated disorders of extrapyramidal movement. Reference Simpson and Angus21 Sociodemographic data were collected during the interview, and subjective sleep quality was assessed using the Pittsburgh Sleep Quality Index (PSQI). Reference Buysse, Reynolds, Monk, Berman and Kupfer22
All participants were on stable antipsychotic medication and for comparison chlorpromazine equivalents were calculated according to Rey et al Reference Rey, Schulz, Costa, Dick and Tissot23 for first-generation antipsychotics and according to Woods Reference Woods24 for second-generation antipsychotics. If the substance was not listed in the above-mentioned articles, the defined daily dosage of the World Health Organization for chlorpromazine (300 mg per day, orally) in comparison with the defined daily dosage of the antipsychotic in question were used to calculated the chlorpromazine equivalents. Reference Nose, Tansella, Thornicroft, Schene, Becker and Veronese25
Circadian rest–activity cycle measurement
Rest–activity cycles were measured by wrist actimetry using the Actiwatch system (Cambridge Neurotechnology Ltd, UK). The participants wore the activity monitor on the wrist of the non-dominant hand for 21 days continuously. Three participants (SCH01, 02, 08) were studied from May to June, whereas all other participants took part during autumn–winter (September to February). Activity gaps during the daytime because of actigraph removal (e.g. showering), were filled with activity counts averaged over the 24-h day. If the data loss was more than 3 h per day, then this particular day was excluded from further analysis. Wrist motor activity was recorded and stored in 1-min intervals along with daily sleep–wake logs that were filled in by the participants before and after sleep.
Actimetry data were analysed by the Sleep and Activity Analysis Software 7.23V (Cambridge Neurotechnology Ltd, UK). Non-parametric circadian rhythm analysis Reference Van Someren, Swaab, Colenda, Cohen, McCall and Rosenquist26 was carried out on average for 17.6 (s.d. = 3.6) continuous days with complete and edited data. This analysis yields circadian characteristics of the rest–activity cycle such as the relative amplitude, the interdaily stability index and intradaily variability. The relative amplitude is calculated from the ratio of the most active 10-h period to the least active 5-h period across the averaged 24-h profile. The interdaily stability index quantifies the invariability day by day, that is, the strength of coupling of the rhythm to supposedly stable environmental zeitgebers, and intradaily variability gives an indication of the fragmentation of the rhythm. Sleep analysis was carried out on average for 20.4 (s.d. = 2.8) nights. Sleep analysis was conducted at a low sensitivity level found to be most consistent with sleep parameters derived from polysomnography. Reference Tonetti, Pasquini, Fabbri, Belluzzi and Natale27 Nap analysis during daytime (between ‘get up time’ and ‘bedtime’) was carried out on average for 17.8 (s.d. = 3.1) days with complete and edited data-sets. A nap was defined by at least 10 min up to 3 h containing zero activity counts.
Melatonin assay
Participants were asked to collect saliva samples during two evenings on weekdays in week 1 and week 3, in order to determine melatonin secretion as a biological marker for circadian phase assessment. They collected saliva in 1-h sampling intervals starting 5 h before bedtime (6 samples, of which the first sample was excluded from analysis) and one sample at getting up time followed by two samples 1 and 2 h afterwards. Saliva sampling was carried out by the participants using salivettes (Sarstedt AG, Switzerland). In-patient sampling was monitored by the staff, out-patients and those attending the day clinic were reminded of sampling times by phone calls or short text messages on their cell phones. Complete data-sets were available for ten participants, and the time course of melatonin levels was expressed relative to bedtime and getting up time. Melatonin was determined by a direct double-antibody radioimmunoassay with an analytical sensitivity of 0.2 pg/ml and a functional least detectable dose of 0.65 pg/ml (Bühlmann Laboratories AG, Allschwil/Switzerland). Reference Weber, Schwander, Unger and Meier28 The threshold for melatonin onset was set at 30% of the highest value before lights off and the onset calculated assuming a linear increase between measurements before and after the melatonin threshold.
Neuropsychological tests
Neuropsychological tests for vigilance, selective attention, psychomotor speed, executive functioning and semantic verbal fluency were administered in paper-and-pencil versions by the same psychologist at the beginning and at the end of the 3-week actimetry recording episode. The Trail Making Test (TMT) assesses attention, psychomotor speed, cognitive flexibility and executive functions in two different ways (TMT A and B). Reference Reitan29 Attention, speed and reaction inhibition/distractibility (executive function) was measured by the Stroop colour–word interference task. Reference Bäumler30 Semantic verbal fluency was assessed through the Supermarket test. Reference Kalbe, Kessler, Calabrese, Smith, Passmore and Brand31
Statistical analysis
All data were normally distributed according to the Kolmogorov–Smirnov test, thus allowing parametric tests to be applied. Statistical analysis of time course in activity and neuropsychological testing was conducted using analyses of variance for repeated measures (rANOVA) with Huynh–Feldt's statistics and Bonferroni's alpha-corrected t-test for post hoc tests. Student's t-test was carried out in order to determine possible differences between groups with either high- or low-relative amplitude. To analyse the relationship between cognitive functioning and other variables such as clinical status, sleep and circadian parameters and sociodemographic variables, Pearson's correlation and then stepwise linear regression analysis was applied. Since age is known to have an influence on cognitive performance, we correlated age with the cognitive performance scores and used the resulting residuals for further analysis. Analysis was conducted using SPSS 15.0 and STATISTICA 6.1 for Windows. The alpha-criterion was set at a significance level of P = 0.05.
Results
Participants
Symptom severity comprised moderate to extreme scores in our cohort (CGI ranging from 4 to 8, mean 6.4, s.d. = 1.0). Symptom severity according to BPRS was on average 40.7 (s.d. = 11.2) and the PANSS yielded a total score of 69.3 (s.d. = 19.6), with positive symptoms of 15.2 (s.d. = 6.9) and negative symptoms of 20.9 (s.d. = 7.1). Extrapyramidal movements were negligible in all participants (SAS 0.09, s.d. = 0.1). Reference Janno, Holi, Tuisku and Wahlbeck32 Subjective sleep quality was good in 6, and moderately impaired (>5) in 8 patients (n = 14, PSQI 6.2, s.d. = 2.6). If antipsychotic use is considered as sleep-inducing medication because of its sedative action, as assessed in the PSQI subscore ‘hypnotic use’, the PSQI total mean score was 7.9 (s.d. = 2.2). These subjective sleep quality scores almost significantly correlated with actimetric-derived sleep parameters, such as sleep efficiency (R = –0.53, P = 0.052) and actual wake time after sleep onset (R = 0.53, P = 0.053).
Actimetry and melatonin measures
Visual inspection of the individual rest–activity cycles showed a broad range of sleep–wake behaviour, ranging from regular and well-entrained to interrupted 24-h rhythms with fragmented sleep and enhanced napping during the daytime (online Fig. DS1). These differences were reflected in the wide distribution of relative amplitude values between 0.54 and 0.98. In comparison, healthy participants were much better entrained, even though they also showed variability. In our independently collected older group of healthy participants (n = 23, age 64, s.d. = 5.4 years), median relative amplitude was 0.91, ranging from 0.77–0.96. Reference Pugin, Viola, Chellappa, Archer, Dijk and Cajochen33 Thus, in order to study the influence of relative amplitude per se in this group of people with schizophrenia, we decided to median split the sample into one group with high relative amplitude (>0.85, n = 7, ‘the high-amplitude group’) and one group with medium to low relative amplitude (<0.85, n = 7, ‘the low-amplitude group’). The two groups also differed significantly with respect to interdaily stability, but not intradaily variability (Table 2).
Table 2 Circadian parameters of the rest–activity cyclea

Mean (s.d.) | Group difference (unpaired t-test), P | |||
---|---|---|---|---|
Entire group (n = 14) | High-amplitude group (n = 7) | Low-amplitude group (n = 7) | ||
Relative amplitude | 0.817 (0.14) | 0.927 (0.04) | 0.706 (0.12) | 0.001 |
Interdaily stability | 0.468 (0.15) | 0.573 (0.14) | 0.363 (0.08) | 0.006 |
Intradaily variability | 0.828 (0.29) | 0.777 (0.28) | 0.880 (0.30) | 0.521 |
Other variables measuring psychopathology and the level of positive and negative symptoms (PANSS, BPRS, CGI) as well as age, education level, illness duration, body mass index, medication by chlorpromazine equivalents and subjective sleep quality (PSQI) did not significantly differ between the high- and low-amplitude groups. However, there was a tendency for more in-patient or day clinic participants in the high-amplitude group than in the low-amplitude group, which comprised more out-patients (Fisher's exact test, one-tailed, P = 0.051).
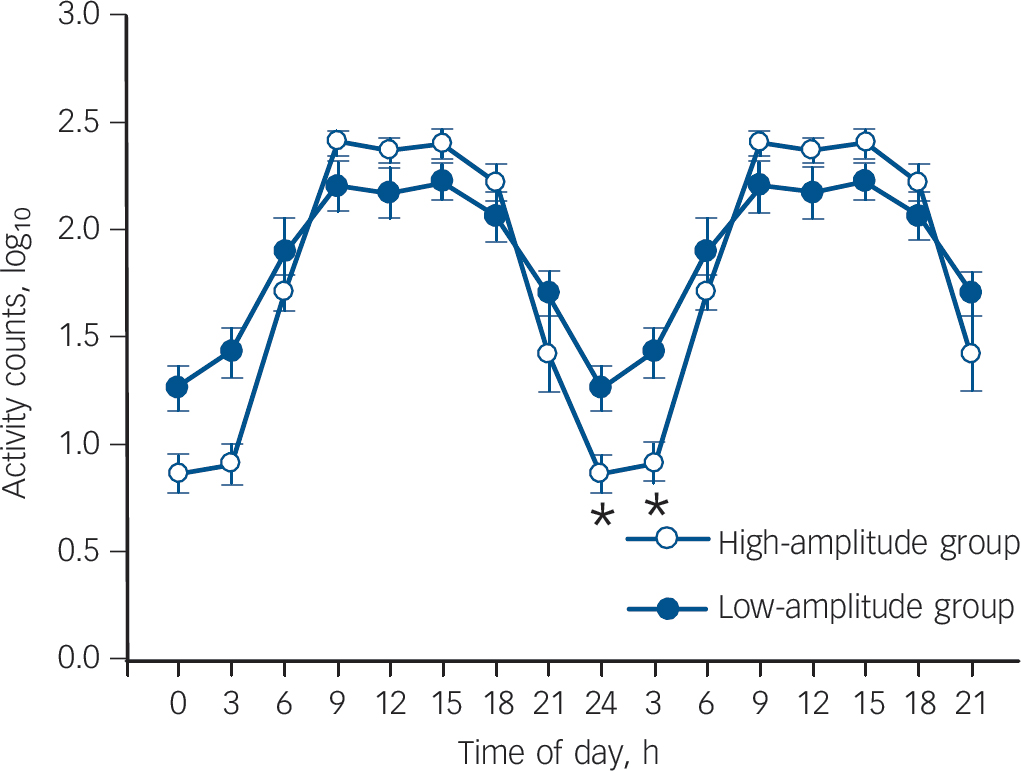
Fig. 1 Average activity double-plot for relative amplitude for median-split groups (low-amplitude group: n = 7; high-amplitude group: n = 7).
Activity data were log-plotted to emphasise the activity differences (mean with standard errors of mean).
The average activity counts of the two groups, log-transformed and collapsed into 3-h bins (Fig. 1), did not reveal a significant difference at group level (two-way rANOVA, factor group: F (1,12) = 0.55, P = 0.472), but yielded a significant factor time (F (7,84) = 89.91, P<0.001) and significant group×time interaction (F (7,84) = 7.65, P<0.001). Post hoc comparisons revealed that the low-amplitude group had significantly higher night-time activity in the time bins 00.00–03.00 h (P = 0.045) and 03.00–06.00 h (P<0.001). This result was corroborated by the actimetry-derived sleep parameters that showed significantly shorter actual sleep time, increased movement time during sleep, and an increased fragmentation index (restlessness) in the low-amplitude group compared with the high-amplitude group (Table 3). Both groups went to bed rather early and spent prolonged time in bed. Furthermore, the participants in the low-amplitude group tended to nap more and to feel sleepier during the daytime than those in the high-amplitude group.
Table 3 Actimetry-derived sleep parametersa

Mean (s.d.) | Group difference (unpaired t-test), P | |||
---|---|---|---|---|
Entire group (n = 14) | High-amplitude group (n = 7) | Low-amplitude group (n = 7) | ||
Bedtime, h : min | 21 : 22 (1 : 19) | 21 : 28 (0 : 57) | 21 : 16 (1 : 41) | 0.785 |
Get up time, h : min | 7 : 56 (1 : 00) | 8 : 01 (0 : 31) | 7 : 51 (1 : 22) | 0.783 |
Total sleep duration, h : min | 10 : 15 (1 : 41) | 10 : 16 (0 : 52) | 10 : 14 (2 : 19) | 0.983 |
Actual sleep time as % of total sleep time | 91.6 (5.8) | 94.8 (2.1) | 88.37 (6.8) | 0.035 |
Sleep efficiency, % | 89.0 (6.2) | 92.4 (3.0) | 85.6 (6.9) | 0.035 |
Sleep latency, min : s | 14 : 05 (7 : 05) | 12 : 53 (6 : 42) | 15 : 17 (8 : 15) | 0.561 |
Movement time, % | 14.9 (7.8) | 10.0 (3.1) | 19.7 (8.2) | 0.02 |
Fragmentation index | 32.8 (14.4) | 23.8 (8.0) | 41.8 (14.1) | 0.012 |
Daytime napping, h : min | 1 : 04 (0 : 40) | 0 : 44 (0 : 36) | 1 : 24 (0 : 36) | 0.065 |
Daytime sleepiness, PSQI | 1.2 (0.8) | 0.86 (0.7) | 1.57 (0.79) | 0.096 |
The time course of mean salivary melatonin secretion showed differences in the two groups and the calculation of the melatonin onset in the low- and the high-amplitude groups differed significantly (Fisher's exact test, P<0.048, Fig. 2). In five out of six participants in the high-amplitude group, melatonin onset could be calculated and averaged 20: 45 h (s.d. = 0.28 min). The phase angle (i.e. the time between melatonin onset and bedtime) between melatonin onset and bedtime ranged from 14 min to 2 h 4 min (mean 57 min, s.d. = 47 min), that between melatonin offset in the morning and getting up from 13 min to 1 h 35 min (mean 55 min, s.d. = 42 min). In contrast, no clear melatonin onset could be detected in any of the four participants in the low-amplitude group. Three of these participants also showed later melatonin offsets compared with participants in the high-amplitude group, with a phase angle of 1 h 37 min, 1 h 46 min and 2 h 18 min to getting up.
We found significant correlations between circadian parameters (relative amplitude, interdaily stability index, intradaily variability) and variables concerning wakefulness during sleep epochs and daytime sleepiness (Table 4). All variables related to psychopathology and/or age did not yield significant correlation to circadian parameters.
Table 4 Correlations of circadian parameters (relative amplitude, interdaily stability index, intradaily variability)a

Pearson's correlation coefficients, R | |||
---|---|---|---|
Relative amplitude | Interdaily stability index | Intradaily variability | |
Actual wake time as % of total sleep time | –0.64* | – 0.12 | 0.13 |
Sleep efficiency | 0.61* | 0.11 | – 0.09 |
Fragmentation index | –0.61* | – 0.24 | 0.15 |
Daytime sleepiness, Pittsburgh Sleep Quality Index | –0.77** | –0.62* | 0.64* |
Mean nap time, % | –0.67** | – 0.49 | 0.86** |
Age | 0.31 | – 0.08 | – 0.03 |
Illness duration | – 0.10 | – 0.10 | – 0.03 |
Positive and Negative Syndrome Scale | |||
General | 0.24 | 0.08 | – 0.07 |
Negative symptoms | – 0.16 | – 0.27 | 0.26 |
Positive symptoms | 0.48 | 0.12 | – 0.34 |
Brief Psychiatric Rating Scale | 0.16 | 0.02 | – 0.26 |
Cognitive performance
Participants in the high- and low-amplitude groups differed significantly at both testing times in the Stroop interference task and semantic fluency task, with a significant tendency for cognitive flexibility (two-way rANOVA factor group: Stroop interference task: F (1,12) = 9.69, P = 0.009, Supermarket test: F (1,12) = 7.09, P = 0.021, TMT A: F (1,12) = 2.48, P = 0.144, TMT B: F (1,12) = 3.77, P = 0.078). Independent of the high and low relative amplitude, cognitive performance improved in participants from test to retest in the Stroop interference task (rANOVA factor test–retest: F (1,12) = 5.44, P = 0.038), but not in the other neuropsychological tasks (P>0.138). Thus, for further analyses cognitive performance was averaged across the test–retest sessions.
Participants with regular rest–activity cycles (high-amplitude group n = 7) performed within the average range of the norm values predicted for the general population, in attention (TMT A 26.1 s (s.d. = 7.4); average value of age group 25–54 years: 24.4–31.7 s) Reference Tombaugh, Kozak and Rees34 and in executive tasks (TMT B 66.3 s (s.d. = 32.8); average value of age group 25–54 years: 50.7–63.8 s); Reference Tombaugh, Kozak and Rees34 in the Stroop interference task: 78.6 (s.d. = 15; normative values of age group 30–50 years: 80–95 s) Reference Bäumler30 and in the semantic fluency task (number of items: 25.3 (s.d. = 5.5); general population ≥ 20). Reference Kalbe, Kessler, Calabrese, Smith, Passmore and Brand31 Participants in the high-amplitude group had significantly better cognitive performance scores compared with participants with medium to low circadian amplitude in the Stroop interference task measuring reaction inhibition (unpaired t-test: P = 0.03), as well as in the semantic fluency task (unpaired t-test: P = 0.02), and they tended to show increased attention assessed with the TMT A (unpaired t-test: P = 0.097). Cognitive flexibility assessed by the TMT B did not yield a significant difference between the two groups (P = 0.189,Fig. 3).

Fig. 2 Individual mean values (sampling week 1 and 3) of salivary melatonin plotted relative to bedtime and get up time in the high-amplitude group (a) and low-amplitude group (b).
The analysis of cognitive daytime functioning as a dependent variable with the relative amplitude of sleep–wake cycles as an independent variable yielded significant results for all of the assessed neuropsychological variables (Pearson's rank correlation, n = 14, TMT A, relative amplitude: R = –0.66, P = 0.011; TMT B, relative amplitude: R = –0.70, P = 0.005; verbal fluency, relative amplitude: R = 0.76, P = 0.002; Stroop interference task, relative amplitude: R = –0.83, P<0.001; verbal fluency, relative amplitude: R = 0.76, P = 0.002). Stepwise linear regression analysis was carried out with five possible predictors: relative amplitude as circadian parameter, sleep efficiency as a sleep parameter, PANSS positive symptoms and PANSS negative symptoms as variables concerning psychopathology, and age. Relative amplitude had the most impact on performance in the Stroop interference task (t = – 5.11, P<0.001), followed by age (t = 2.45, P = 0.032). A total of 79.7% of the variance in the Stroop interference task can be explained by these two variables (R 2 = 0.797). Attention and psychomotor speed (TMT A) were mostly affected by age (t = 4.22, P = 0.001) and relative amplitude (t = –3.23, P = 0.008) with R 2 = 0.78. Cognitive flexibility together with psychomotor speed (TMT B) were best predicted by relative amplitude (t =–3.42, R 2 = 0.49, P = 0.005). Relative amplitude also had most impact on the semantic fluency task (Supermarket test, t = 4.09, R 2 = 0.58, P = 0.002). Sleep efficiency and positive and negative symptoms scores were excluded by the stepwise regression analysis as predictors for cognitive performance.

Fig. 3 Cognitive performance scores in the high- (n = 7) and low-amplitude group (n = 7).
Reaction times for the Trail Making Test A (TMT A) and B (TMT B), Stroop interference task (P = 0.03) and number of items in the semantic fluency task (P = 0.02) (mean with standard errors of mean).

Fig. 4 Correlation of relative amplitude with the age-adjusted (a) Stroop interference task (R = –0.80, P = 0.0006) and (b) Trail Making Test A (TMT A) scores (R = –0.67, P = 0.009).
Correlations with age-corrected cognitive scores and relative amplitude yielded a considerable impact of relative amplitude on Stroop interference performance (t =–4.597, P = 0.0006, R 2 = 0.64, Fig. 4a), on TMT A performance (t =–3.12, P = 0.009, R 2 = 0.45, Fig. 4b), on TMT B performance (t =–2.85, P = 0.015, R 2 = 0.40) and on the verbal fluency performance (t = 3.86, P = 0.002, R 2 = 0.55).
Discussion
Circadian rhythms in participants with schizophrenia
Participants with schizophrenia showed a wide range of circadian sleep–wake cycle patterns, from normal to highly interrupted, as indexed by a relative amplitude of day/night activity. In accordance with other studies in people treated with atypical antipsychotic drugs, Reference Wirz-Justice, Cajochen and Nussbaum8,Reference Wirz-Justice, Haug and Cajochen9 all our participants seemed to be entrained to the 24-h solar day with most of the sleep epochs occurring during the night and wakefulness during the day. However, participants with a low relative amplitude showed significantly more activity and wakefulness during sleep and a lower day-by-day stability of their activity (interdaily stability index) than those participants with a normal relative amplitude. This suggests that participants in the low-amplitude group suffered from a lack of effective environmental zeitgebers. Moreover, their melatonin onset occurred after their usual bedtime, which suggests an abnormal phase relationship between their sleep epochs and their internal clock.
Participants in the high-amplitude group were mainly in-patients or day clinic patients, whereas in the low-amplitude group there were more out-patients. Thus, we cannot role out the possibility that the daily clinical routines in the clinic had beneficial zeitgeber effects such that they helped to consolidate the sleep–wake cycles of the participants.
Sleep–wake cycles and cognition
Superior cognitive functioning was observed in participants with normal circadian properties, whereas participants in the low-amplitude group performed worse in the Stroop colour–word interference task and the semantic verbal task (Supermarket test). Given that disturbances in circadian rhythms are associated with impaired cognitive functioning, it is very likely that the observed misalignment of the rest–activity cycle with respect to the internal clock impacted on cognition (i.e. learning). Reference Wright, Hull, Hughes, Ronda and Czeisler35 Our data from participants with schizophrenia confirm findings by Oosterman and colleages Reference Oosterman, van Someren, Vogels, Van Harten and Scherder36 who also found that actimetric rest–activity rhythm variables, such as the amplitude of day/night activity and intradaily variability, predicted cognitive functioning (mental speed and executive functions) in home-dwelling elderly people. Age was found to have an influence on cognitive performance. This effect was expected since ageing of the human brain predominantly affects the prefrontal cortex, and executive function is known to decline with age in healthy as well as in mentally ill people, such as individuals with schizophrenia. Reference Tombaugh, Kozak and Rees34,Reference Bhatia, Garg, Pogue-Geile, Nimgaonkar and Deshpande37
Our findings suggest that the circadian variable relative amplitude was the most powerful variable next to age to predict cognitive outcomes, even after these findings were corrected for the age influence on cognitive performance scores. The neuropsychological tests applied are related to prefrontal cortex functioning, which undergoes continuous activity during wakefulness. This cortical region was found to be highly sensitive to sleep loss in a sleep deprivation protocol, Reference Harrison, Horne and Rothwell38 and these functions appear also to be involved in schizophrenia. Reference Mesholam-Gately, Giuliano, Goff, Faraone and Seidman39 In our cohort, cognitive outcome may also be affected by lack of sleep, since participants with low relative amplitude showed fragmented sleep with more activity and wakefulness during sleep episodes and enhanced daytime sleepiness than participants with normal rest–activity cycles. However, not all tests have previously been found to be affected by sleep quality. Performance of the interference task did not decrease during a total sleep deprivation protocol. Reference Sagaspe, Sanchez-Ortuno, Charles, Taillard, Valtat and Bioulac40
Clinical status did not show any relation to cognitive performance, nor were relative amplitude or interdaily stability index related to the severity of either positive or negative symptoms. This was against our initial predictions, since negative symptoms (characterised as lack of interest and affect, as well as social withdrawal) can imply weak zeitgebers, such as social cues or daylight exposure, and therefore desynchronised circadian activity rhythms in these individuals.
Limitations of the study
Our cohort comprised a highly heterogeneous group of individuals who were investigated over 3 weeks in real-life settings. They had different medication intake, clinical status and living situations (in- and out-patients) with and without a daily routine. Despite this high heterogeneity we found circadian rhythms, sleep disturbances and age to be main factors to explain cognitive deficits.
In particular, flattened day/night activity rhythms (low relative amplitude) was correlated with sleep disturbances. However, patients with a low relative amplitude also showed delayed melatonin onset, which indicates disturbed timing of the circadian system. This delayed circadian phase might have contributed to their sleep disturbance, since stable sleep regulation requires adequate synchronisation of the circadian and homeostatic processes.
It remains to be investigated whether there is a similar decline of cognitive functioning because of flattened circadian rest–activity cycles in healthy controls, or if they can possibly cope better and longer. Furthermore, it will be interesting to investigate this relationship in other disorders affecting cognition.
Implications
Consolidated circadian sleep–wake cycles correlate with cognitive functioning in people with schizophrenia. This suggests that chronotherapeutic treatments, such as enhancing healthy sleep and daily activity as well as light therapy, may provide a useful adjunct to aid rehabilitative processes in people with schizophrenia. However, it remains uncertain whether both cognition and disturbed circadian rest–activity cycles may be symptoms of other underlying processes. Further studies focusing on individuals in the early stages or at risk of developing schizophrenia are needed to unearth the nature of this association.
Funding
This study was supported by the Velux Foundation (#425), Switzerland, and Bristol-Myers Squibb, Switzerland.
Acknowledgements
We thank the staff of the general psychiatry and the forensic departments of the Psychiatric Hospital of the University of Basel for monitoring saliva sampling of the participants. Claudia Renz and Bühlmann Laboratories, Allschwil, Switzerland, for the melatonin assays, Sarah L. Chellappa, MD, for corrections to the manuscript and Dr Katharina Wulff for explanations on the method and analysis of actimetry data.
eLetters
No eLetters have been published for this article.