Introduction
Winter-dormant zoysiagrass (Zoysia japonica Steud.) is commonly characterized by brown foliage with no green tissue protruding from the canopy (Patton et al. Reference Patton, Schwartz and Kenworthy2017). However, it has been noted that close inspection of dormant zoysiagrass can reveal green tissue at the base of stems and subcanopy leaves that are at least partially green (Velsor et al. Reference Velsor, Dunn and Minner1989). Nonselective herbicides, such as glyphosate or glufosinate, are commonly used to control winter weeds in dormant turfgrass, but only five peer-reviewed articles have reported dormant-zoysiagrass response to these herbicides (Craft et al. Reference Craft, Godara and Askew2023a; Hoyle and Reeves Reference Hoyle and Reeves2017; Rimi et al. Reference Rimi, Macolino and Leinauer2012; Velsor et al. Reference Velsor, Dunn and Minner1989; Xiong et al. Reference Xiong, Diesburg and Lloyd2013). These five articles indicate that glyphosate and glufosinate did not injure zoysiagrass when treated “prior to green-up” or “during dormancy.” Aside from an ancillary mention of subcanopy green tissue observed by Velsor et al. (Reference Velsor, Dunn and Minner1989), the researchers had not elucidated canopy characteristics such as number of green leaves or stolons. In three cases, glyphosate and glufosinate injured zoysiagrass when applied later in the season to partially green zoysiagrass canopies (Rimi et al. Reference Rimi, Macolino and Leinauer2012; Velsor et al. Reference Velsor, Dunn and Minner1989; Xiong et al. Reference Xiong, Diesburg and Lloyd2013).
Despite evidence of glyphosate and glufosinate being safely applied to dormant zoysiagrass (Craft et al. Reference Craft, Godara and Askew2023a) and product labels for these herbicides indicating their use on “dormant turfgrass,” turfgrass managers are hesitant to use nonselective herbicides on dormant zoysiagrass. Extension bulletins from Arkansas and Tennessee recommend against treating dormant zoysiagrass with glyphosate (Boyd Reference Boyd2016; Brosnan and Breeden Reference Brosnan and Breeden2011). The two most logical explanations for inconsistent injury following dormant sprays are either the practitioners all mistook partially green turf as dormant or subcanopy green leaves and stems were exposed to spray droplets and were able to absorb the herbicide. Further research efforts are needed to investigate the factors that may contribute to variable spray exposure to subcanopy turf layers and the extent to which subcanopy stems may absorb glyphosate or glufosinate when compared with leaves.
Previous research documented that nozzles, which produce coarse to very coarse droplets, could increase turf canopy penetration (Shepard et al. Reference Shepard, Agnew, Fidanza, Kaminski and Dant2006). Although several studies have evaluated the influence of nozzle type and application volume on surface coverage of turf and associated weed or disease control (Benelli et al. Reference Benelli, Horvath, Womac, Ownley, Windham and Sorachan2018; Ferguson et al. Reference Ferguson, Gaussoin, Eastin, Sousek and Kruger2016; Fidanza et al. Reference Fidanza, Kaminski, Agnew and Shepard2009; Kaminski and Fidanza Reference Kaminski and Fidanza2009; McDonald et al. Reference McDonald, Dernoeden and Bigelow2006; Neal et al. Reference Neal, Bhowmik and Senesac1990; Vincelli and Dixon Reference Vincelli and Dixon2007), factors that govern spray penetration into turf canopies have scarcely been reported. Preliminary studies by Benelli et al. (Reference Benelli, Horvath, Womac, Ownley, Windham and Sorachan2018) evaluated exposure of stem, sheath, and leaves in a zoysiagrass canopy to fungicides and found subcanopy stem exposure increased with increasing application volume. Sharpe et al. (Reference Sharpe, Boyd, Dittmar, MacDonald, Darnell and Ferrell2018) showed that with increasing application volume, spray penetration also increases in the lower canopy of strawberries [Fragaria × ananassa (Weston) Duchesne ex Rozier]. Previous research examining canopy penetration across a range of spray parameters and nozzle types has been conducted primarily in cropping systems with an emphasis on weed control and found varying results with respect to droplet diameter and canopy penetration (Derksen et al. Reference Derksen, Zhu, Ozkan, Hammond, Dorrance and Spongberg2008; Hanna et al. Reference Hanna, Robertson, Carlton and Wolf2009; Knoche Reference Knoche1994; Wolf and Daggupati Reference Wolf and Daggupati2009; Zhu et al. Reference Zhu, Dorner, Rowland, Derksen and Ozkan2004). Wolf and Daggupati (Reference Wolf and Daggupati2009) observed improved penetration into a dense soybean [Glycine max (L.) Merr.] canopy from fine and medium droplets. Zhu et al. (Reference Zhu, Dorner, Rowland, Derksen and Ozkan2004) found that air-induction nozzles produced more spray deposition into the bottom of soybean canopies, while conventional flat-fan nozzles had the lowest spray penetration. Derksen et al. (Reference Derksen, Zhu, Ozkan, Hammond, Dorrance and Spongberg2008) observed better soybean canopy penetration with medium and coarse droplets compared with fine droplets. In contrast, Hanna et al. (Reference Hanna, Robertson, Carlton and Wolf2009) observed no difference between droplet sizes with respect to soybean canopy penetration.
Spray droplet size and velocity affect the structure of spray deposits and the droplet’s potential to drift (Lake Reference Lake1977). Research has shown that larger droplets correspond with higher droplet velocities (Nuyttens et al. Reference Nuyttens, Baetens, De Schampheleire and Sonck2007). Creech et al. (Reference Creech, Henry, Fritz and Kruger2015) determined that nozzle design and application pressure caused the most significant changes to spray droplet size. Increasing spray pressure reduces droplet size and may increase associated spray coverage (Liao et al. Reference Liao, Luo, Wang, Zhou, O’Donnell, Zang and Hewitt2020). As the distance between spray nozzles and the targeted pest, or “boom height,” increases, spray deposition to the target decreases due to drift (Balsari et al. Reference Balsari, Marucco and Tamagnone2007), and droplet velocities, especially of smaller droplets, also decrease (Goering et al. Reference Goering, Bode and Gebhardt1972). The most common type of nozzles used in turfgrass settings is the flat-fan nozzle (Shepard et al. Reference Shepard, Agnew, Fidanza, Kaminski and Dant2006). Air-induction nozzles were designed to produce larger droplets than standard flat-fan nozzles at a given pressure for drift control (Etheridge et al. Reference Etheridge, Womac and Mueller1999). It is conceivable that manipulating nozzle type, pressure, or boom height could alter the penetration of droplets into a turf canopy due to the aforementioned effects these parameters have on droplet size and speed.
Assuming herbicide can be delivered to subcanopy layers of zoysiagrass turf, stem exposure becomes more relevant. However, glyphosate and glufosinate absorption into zoysiagrass stems has not been reported. Glyphosate and glufosinate can be absorbed by roots, stems, and leaves (Pline et al. Reference Pline, Price, Wilcut, Edmisten and Wells2001; Steckel et al. Reference Steckel, Hart and Wax1997; Thomas et al. Reference Thomas, Pline, Wilcut, Edmisten, Wells, Viator and Paulsgrove2004; Wills Reference Wills1978), so stem absorption into zoysiagrass is plausible. With the paucity of information regarding spray penetration into subcanopy layers of zoysiagrass turf and stem absorption of commonly used nonselective herbicides during zoysiagrass dormancy, studies were conducted to investigate these factors. The objectives of this research were (1) to evaluate absorption and translocation of [14C]glyphosate and [14C]glufosinate in ‘Meyer’ zoysiagrass when applied to leaves or subtending stolons; (2) determine the effect of nozzle type, nozzle height above the target, and spray pressure on droplet size and velocity and the penetration of spray droplets into a zoysiagrass turf canopy using a colorant tracer assessed via fluid extraction and spectrophotometric analysis; and (3) to assess the effect of carrier volume on spray penetration in dormant zoysiagrass turf canopies.
Materials and Methods
Herbicide Absorption and Translocation Study
Dormant Meyer zoysiagrass plant material was collected from the Virginia Tech Glade Road Research Facility in Blacksburg, VA (37.23°N, 80.44°W), in October of 2020. Zoysiagrass plugs (10.8-cm diameter and 15-cm depth) were collected using a commercial golf course cup cutter (Lever Action Hole Cutter, Pair Aide Products, Lino Lakes, MN). Zoysiagrass plugs were then placed in the greenhouse and fertilized with Miracle-Gro All-purpose Plant Food 24-18-6 (Scotts Miracle-Gro, Marysville, OH). The greenhouse was maintained at a temperature of 27 ± 6 C with approximately 420 μmol m−2 s−1 of photosynthetically active radiation (PAR) and irrigated every 24 h to maintain active growth. After green shoots started to develop, plugs were dissected, and 6-cm grass sprigs with at least three green leaves and a 1-cm-long subtending stolon were collected. Three sprigs were placed into 9-cm-diameter glass petri dishes with wet germination paper (76# Heavy Weight Seed Germination Paper Circles, AnchorSeed Solution, St Paul, MN). Before sprigs were placed into petri dishes, they were dipped into a 0.59 mg ai L−1 solution of fluxapyroxad + pyraclostrobin (Lexicon Intrinsic® fungicide, BASF, Research Triangle Park, NC) to prevent large patch (Rhizoctonia solani) (Amaradasa et al. Reference Amaradasa, Lakshman, McCall and Horvath2014). The germination paper was watered by a syringe to prevent desiccation with a one-quarter strength Hoagland’s Modified Basal Salt solution (pH 6.5) (MP Biomedicals, Solon, OH). Petri dishes with associated glass covers were placed into a growth chamber set to 30/25 C day/night temperature with 330 μmol m−2 s−1 of PAR.
The study was arranged as a completely randomized design, replicated four times with two temporal runs. Treatments were arranged in a split-plot design with a factorial arrangement of two herbicides by two application placements as main plots and three levels of harvest time as subplots. Each 3-leaf shoot with subtending stolon was considered an experimental unit, and each petri dish comprised a main plot. After 2 d in the growth chamber, three 1-µl droplets containing 4.0 kBq [14C]glyphosate or [14C]glufosinate were spotted equidistantly along the leaf or stolon. The glyphosate (phosphonomethyl-14C, specific activity = 50 µCi mmol−1, purity 99%) spotting solution was converted to the isopropylamine salt by combining 200 µl [14C]glyphosate acid with 1.6 µl isopropyl amine, then adding 0.8% v/v MON56164 surfactant (nonionic polyethoxylated tallow amine, Monsanto, St Louis, MO). Radiolabeled glufosinate (glufosinate hydrochloride, specific activity = 51.8 µCi mg−1) spotting solution contained a 0.1% v/v nonionic surfactant (Induce®, Helena Chemical, Collierville, TN).
Shoots with subtending stolons were randomly assigned within petri dishes to be harvested at 4, 24, and 72 h after treatment (HAT). At harvest, the treated leaf or stolon was removed from the plant and vortexed for 30 s in 10 ml of methanol:deionized water (1:1) plus 0.25% v/v nonionic surfactant to remove unabsorbed herbicide. A 0.5-ml aliquot of the resulting rinsate was added to 15 ml of scintillation fluid (Sciniti Verse LC Cocktail Scitanalyzed, Fisher Scientific, Pittsburgh, PA) and analyzed with a liquid scintillation spectrometer (LSS 6500 Multipurpose Scintillation Counter, Beckman Coulter, Indianapolis, IN) to determine total unabsorbed radioactivity. Following the rinse, the treated portion and remaining shoot or stolon was then immersed in liquid nitrogen, and then plant material was ground using a mortar and pestle. A 2-ml of extraction solution (4:1 methanol:deionized water) was added to the pulverized plant tissue and homogenized using the mortar and pestle. The ground plant material and extraction solution were suction filtered using a Buchner funnel fit with Whatman No.1 filter paper (Whatman International, Maidstone, UK). The mortar, pestle, funnel, and filter paper were further rinsed with an additional 8 ml of extraction solution. A 0.5-ml aliquot from the resulting extract was then added to 15 ml of scintillation fluid, and total absorbed radioactivity was determined by liquid scintillation spectrometry, as described previously.
Because the “harvest-time” subplots had a variance structure consistent with repeated measures, trends in recovered radioactivity over time were subjected to linear regression by replicate, and resulting slopes were subjected to ANOVA using PROC GLM in SAS v. 9.2 (SAS Institute, Cary, NC). Recovered radioactivity at the 3-d harvest was analyzed likewise. The effects of herbicide, placement, and herbicide by placement were tested using the mean square associated with their interaction with trial (McIntosh Reference McIntosh1983). Data were pooled over nonsignificant interactions for the purposes of mean separation. Data were pooled over trial only if trial by treatment interactions were insignificant at α = 0.05. Significant effects or interactions were subjected to Fisher’s protected LSD test at α = 0.05.
Spray Penetration Study
A field study was conducted at the Virginia Tech Glade Road Research Facility in Blacksburg, VA (37.23°N, 80.44°W), in the spring of 2019 and 2020. Meyer zoysiagrass plugs (10.8-cm diameter by 15-cm deep) were collected using a commercial golf course cup cutter (Lever Action Hole Cutter, Pair Aide Products) from a field site maintained at a regular mowing height of 5.7 cm. The soil type at the experimental site was a Duffield silt loam (fine-loamy, mixed, active, mesic Ultic Hapludalfs)–Ernest silt loam (fine-loamy, mixed, superactive, mesic Aquic Fragiudults) complex, with a pH of 6.8 and 4.8% organic matter. The intact turf plugs were placed into 14-cm-diameter pots and backfilled with native soil to ensure the turf canopy was level with the top of the pot. The experiment was implemented as a three-factor factorial, completely random design with eight replications. Factors were nozzle type, operating pressure, and nozzle height.
Spray nozzles evaluated were XR TeeJet® 11006 (XR) and Turbo TeeJet® Induction 11006 (TTI) flat-spray tips (TeeJet® Technologies, Springfield, IL). Nozzles were chosen based on their ability to produce different droplet sizes and velocities and to represent common nozzles used in the turf industry (Shepard et al. Reference Shepard, Agnew, Fidanza, Kaminski and Dant2006). These two nozzles were operated at all possible combinations of 103- and 414-kPa pressures at 25 and 61 cm above the turf canopy. A blue colorant (Blazon® Spray Pattern Indicator, Milliken Chemical, Spartanburg, SC) was mixed 50% v/v with water, and the application volume was held constant at 374 L ha−1 for all treatments by manipulating boom sprayer speed (Figure 1A). Spray was allowed to dry for 24 h at room temperature, and the turf canopy of each treated plug was then dissected with scissors using reference jigs to guide the cutting operation (Figure 1B). The 5.7-cm canopy was separated into three 1.9-cm sections. Canopy leaf and stem material was suspended in 50 ml of water and shaken for 10 s. The suspension was filtered with Whatman No. 42 filter paper (Whatman International) using a Buchner funnel under negative pressure (Figure 1C). Another 10 ml of water was used to rinse the extraction container, Buchner funnel, and filter paper. Preliminary studies indicated a 97 ± 2% extraction efficiency using this procedure. The resulting extract was further diluted 1:4 with deionized water, a 2-ml aliquot was added to a 5-ml cuvette (Figure 1D), and absorbance was measured via spectrophotometer (Genesys 5, Thermo Spectronics, Rochester, NY) at 650 nm. Colorant concentration was calculated based on a standard curve comprising 30 known dye concentrations between 0 and 5,000 ppm measured for absorbance at 650 nm in triplicate.
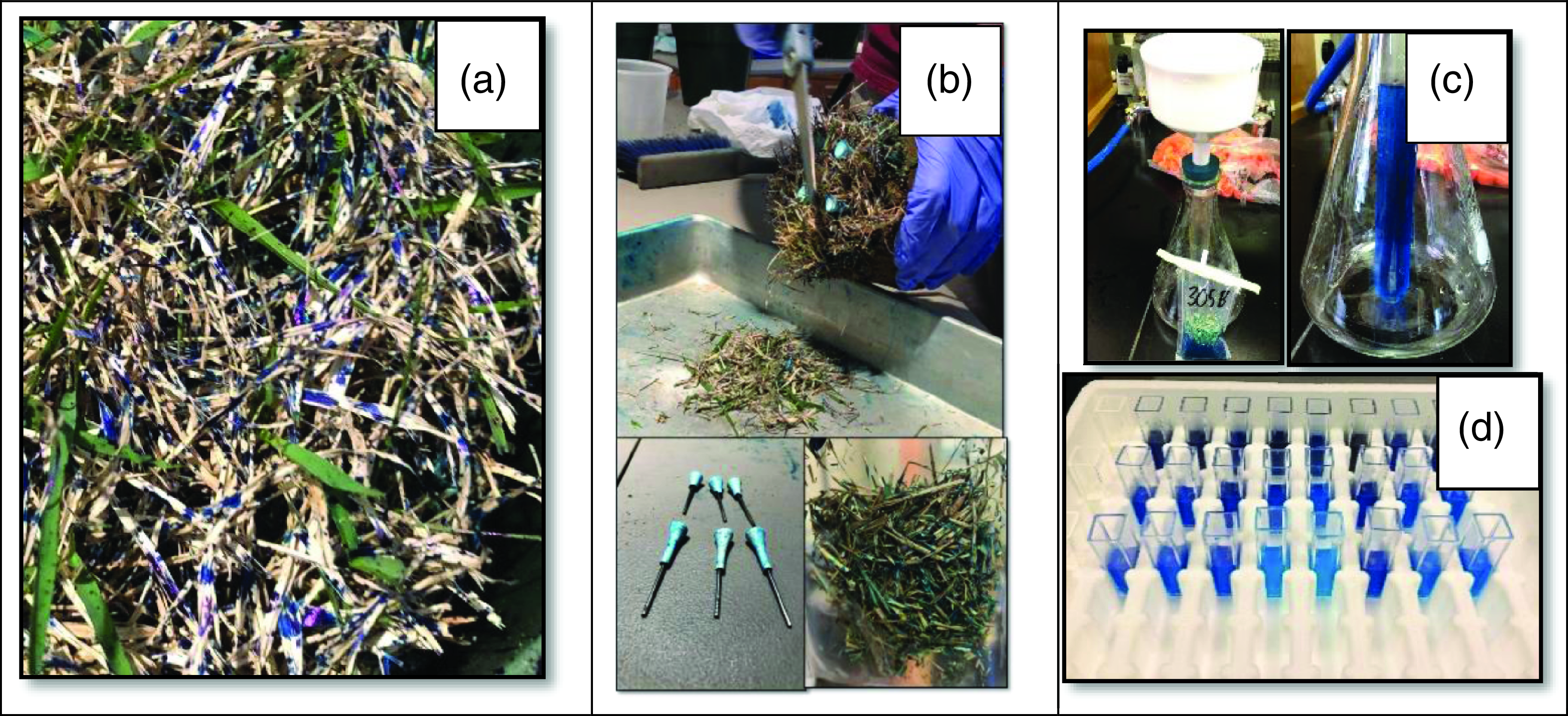
Figure 1. To assess boom height, spray nozzle, and pressure effects on spray penetration into zoysiagrass canopies, zoysiagrass plugs were sprayed with colorant solution (A), and canopies were divided into three partitions using spacers (B), extracted by shaking for 1 min in Whirl-pak® bags, vacuum filtered (C), and subjected to spectrophotometric analysis (D).
After colorant extraction, the remaining plant material was dried for 48 h at 60 C and weighed on a 10,000th g scale. The material was then combusted at 500 C for 5 h, and the resulting ash weight was subtracted from the dried plant material weight to exclude any possible soil or other contaminants. A nontreated comparison was subjected to the same process and served as background for the spectrophotometric analysis. In addition, all plant material incised from each of the three canopy layers of nontreated plugs was subjected to surface area measurement (LI-3100 Area Meter, Li-Cor, Lincoln, NE) to determine the relationship between biomass and surface area (Figure 2). The resulting slope and intercept were used to relate biomass to the estimated surface area so that extracted colorant could be expressed as milliliters of colorant per square meter of tissue surface area.
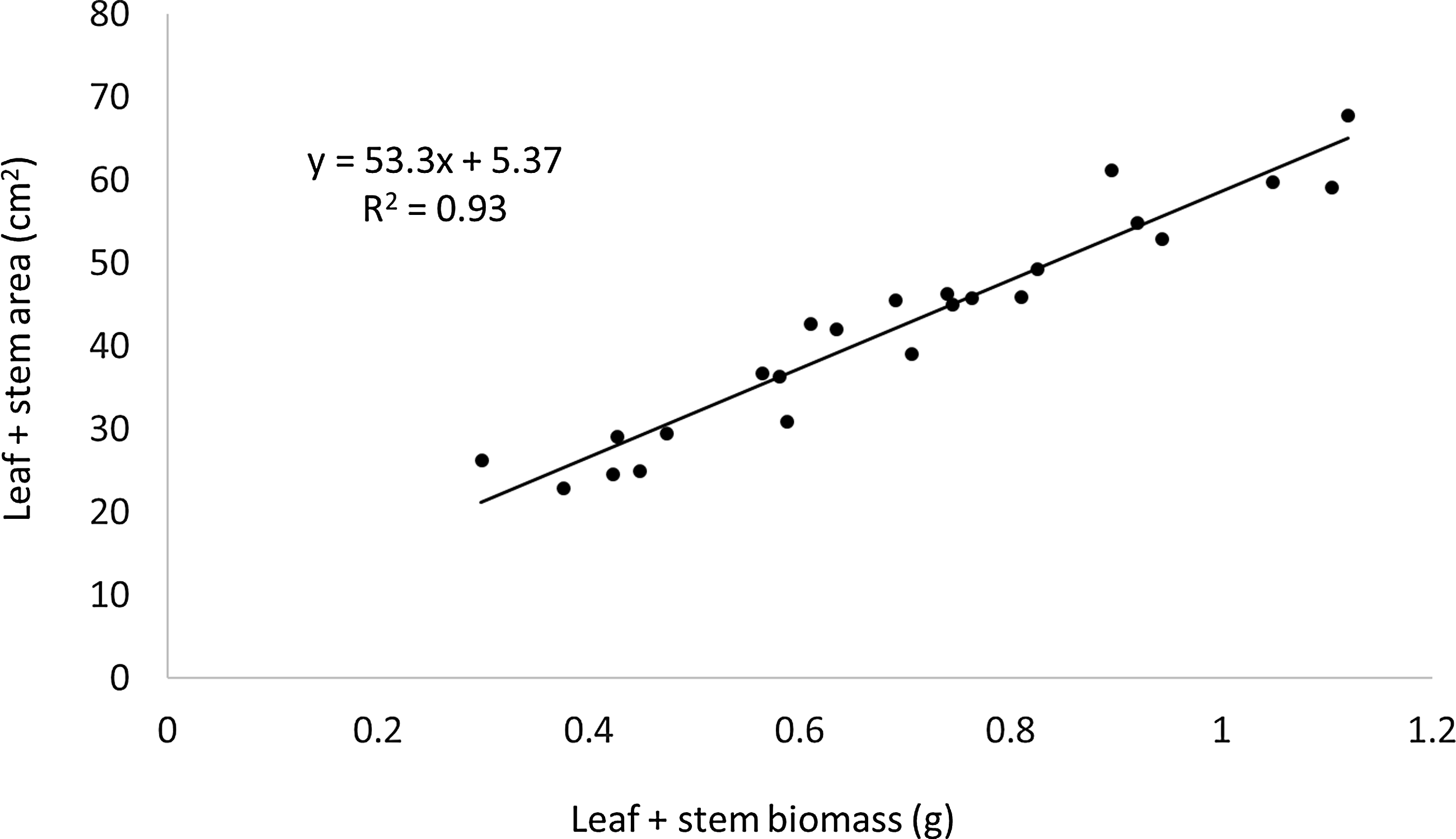
Figure 2. Relationship between surface area and biomass of excised leaf and stem material from nontreated turf.
Data were subjected to a combined ANOVA using PROC GLM in SAS v. 9.2 (SAS Institute), with sums of squares partitioned to reflect the effects of year, replication, nozzle type, operating pressure, and nozzle height above the turf canopy. Year was considered a random variable, and the mean square of all main effects or interactions of nozzle type, operating pressure, and height were tested with the mean square associated with their interaction with year (McIntosh Reference McIntosh1983). Appropriate means were separated, as described previously.
Droplet Diameter and Velocity Study
The same nozzles, pressures, and heights evaluated in the previous study were duplicated in the laboratory using the same spray equipment mounted to a custom-built droplet analyzer. Droplet characteristics for each of the eight combinations of nozzle type, operating pressure, and nozzle height were measured via high-speed video analysis at 15,000 frames s−1. A high-speed video camera (Edgertronic™, Sanstreak, Campbell, CA) was positioned with a field of view spanning 23 mm in height, with the upper edge of the viewable area set to 25 and 61 cm below the operating nozzles. Twelve 100-watt halogen light bulbs illuminated the viewing area. Imaged droplets were restricted to a 2-cm field of view via a 2 cm by 40 cm opening in a plastic shield positioned between the spray nozzle and the camera viewing area. Scale objects were used to determine on-screen size relationships, and the video background consisted of repeating black and white bars of known width for additional reference. Diameter and speed of droplets were measured manually from the output display and converted to actual size via scale relationships. Droplet velocities were determined by counting the number of video frames required for a given droplet to traverse the 23-mm vertical viewing area and subjecting the data to the following equation.

where Y is the droplet speed in millimeters per second (m s−1), ƭ is the observed number of frames required for vertical traversal, and Ɣ is the vertical viewing area of the camera in millimeters. Droplets that appeared unusually slow compared with the majority were assumed to have impinged the depth-of-field restriction opening and were excluded from the analysis. Horizontal diameter and velocity were determined for 100 randomly chosen droplets in each of 10 replicate video clips for each level of nozzle, operating pressure, and height. Data were subjected to ANOVA using PROC GLM in SAS v. 9.2 (SAS Institute) with sums of squares partitioned to reflect the effects of replication, nozzle type, pressure, and height above the droplet assessment area. Significant effects or interactions were then subjected to linear or second-order polynomial regression analysis to relate droplet diameter to droplet velocity.
Carrier Volume Study
A field study was conducted to evaluate the effect of carrier volume on herbicide penetration in dormant zoysiagrass canopies in the spring of 2021 and 2022. The experiment was implemented as a single-factor (i.e., carrier volume), randomized complete block design with four replications and three temporal runs (one in 2021 and two in 2022). The spray solution consisted of glyphosate (Roundup Pro® Concentrate, Bayer Environmental Sciences, Research Triangle Park, NC) at 560 g ae ha−1 and Blazon® blue spray pattern indicator (Milliken Chemical, Spartanburg, SC) at 25% v/v delivered at 60, 138, 271, 415, and 584 L ha−1. Flat-fan spray tips (TeeJet® Technologies) used to deliver 60, 138, 271, 415, and 584 L ha−1 of spray solution were 1100067, 110015, 11004, 11005, and 11006, respectively. Two 2.3-cm circular filter papers were held with wire clips and positioned vertically such that the edge of one filter paper was flush with the top of the 5.6-cm-tall turf canopy, and the edge of the other filter paper was flush with the soil surface (Figure 3A). These filter papers were oriented to minimize turf canopy disturbance. One additional pair of filter papers was positioned in a 10-cm-diameter area that had been completely cleared of aboveground vegetation in each plot as a comparison. The colorant was extracted separately from the top and bottom papers by placing each paper with 2 ml deionized water in small glass vials and shaking on a rotary shaker at 200 rpm for 5 min (Figure 3B and 3C). The resulting extract was further diluted 1:4 with deionized water, a 2-ml aliquot was added to a 5-ml cuvette, and absorbance was measured using a spectrophotometer (Genesys 5, Thermo Spectronics) at 650 nm. The absorbance values were further converted to colorant concentration (ppm) and were calculated based on a standard curve comprising 30 known dye concentrations between 0 and 5,000 ppm measured for absorbance at 650 nm in triplicate. All subsamples within each replicate were averaged, and the percent colorant recovered from papers in the lower canopy was calculated as the colorant concentration in lower canopy papers divided by total colorant concentration observed in both papers from lower and upper canopy levels.
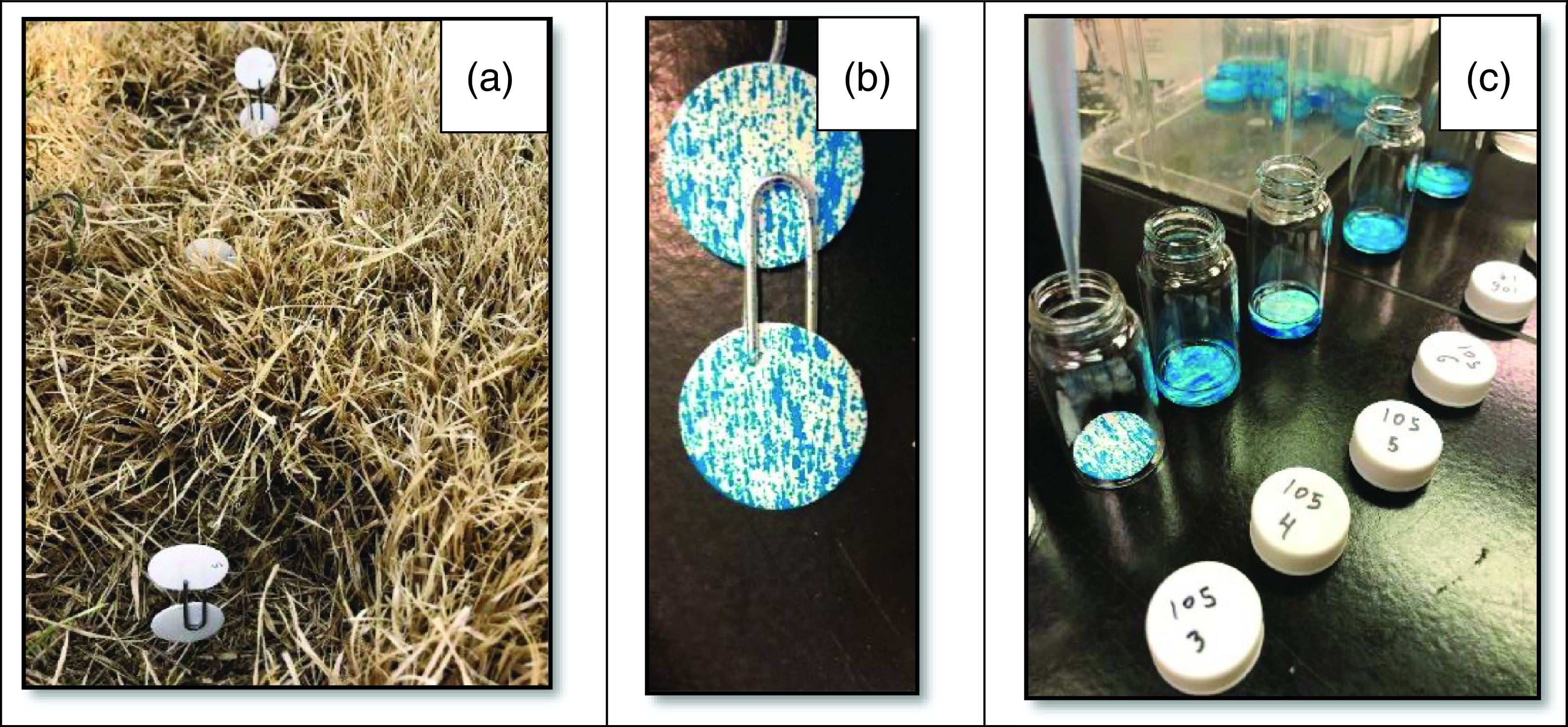
Figure 3. To assess the influence of application volume on spray penetration, filter papers were affixed to wire and distributed in dormant zoysiagrass turf and comparison voided areas (A) such that one paper was aligned to the top of the turf canopy and the other was aligned with the ground. Papers were removed after treatment with herbicide and colorant solution (B), and colorant was water extracted (C) before spectrophotometric analysis.
Percent colorant extracted from filter papers positioned in the lower canopy level was subjected to ANOVA using PROC GLM in SAS v. 9.2 (SAS Institute) with sums of squares partitioned to reflect the effects of the temporal run, replication, and carrier volume. Temporal run was considered a random variable, and the mean square of all main effects or interactions of carrier volume was tested with the mean square associated with their interaction with run (McIntosh Reference McIntosh1983). If temporal run interactions were nonsignificant at α = 0.05, percent colorant in turfgrass lower canopy data were pooled over temporal runs. Significant effects or interactions were then subjected to linear regression analysis to relate the percent colorant delivered to lower zoysiagrass canopy levels to carrier volume using SigmaPlot v. 13.0 (Systat Software, San Jose, CA).
Results and Discussion
Herbicide Absorption and Translocation Study
The interaction of trial with herbicide, placement, or herbicide by placement was not significant for any response variable. Data were pooled over trial to examine the significant herbicide by placement interaction for both the slope of responses over harvest times (P = 0.0005) and observed values of recovered radioactivity at 3 d after treatment (DAT; P = 0.0044) from rinsing the treated area, extracting from shoot tissue, and extracting from stolon tissue (Table 1). Based on regression slopes, recovered radioactivity from the rinse of treated leaves declined 6.6% d−1 for glyphosate, which was about half that of glufosinate (Table 1). Radioactivity recovered from the rinse of treated stolons did not vary between herbicides with respect to time (Table 1). When applied to leaves, glufosinate resulted in nearly double the daily increase of recovered radioactivity from shoots compared with glyphosate (Table 1), indicating more rapid foliar absorption. Neither herbicide translocated from leaves to stolons appreciably, as daily accumulation in stolons was not more than 0.3% of recovered leaf-applied herbicide. Recovered radioactivity accumulation per day in stolons was dependent on where the herbicides were initially placed but not on the herbicide (Table 1).
Table 1. Influence of herbicide and application placement on slope of recovered 14C radioactivity × time in days and extracted radioactivity at 3 d after application from a rinse of the treated portion (rinse), extraction from shoot tissue (shoot), and extraction from stolon tissue (stolon) for zoysiagrass sprigs with a 1-cm stolon subtending a 3-cm leaf shoot in herbicide absorption and translocation study. a, b
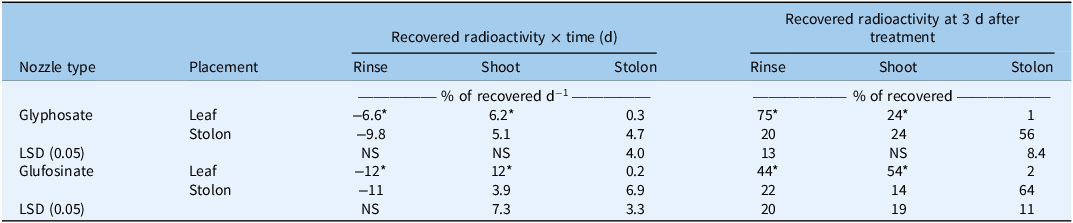
a Shoots were treated on the adaxial surface of the newest, fully expanded leaf, and stolons were treated on the adaxial surface of the internode preceding the shoot.
b Means followed by an asterisk (*) are significantly different between herbicides within a given placement location (leaf vs. stolon) at α = 0.05, and LSDs compare between application placement within a given herbicide
At 3 DAT, only 25% of recovered radioactivity associated with leaf-applied glyphosate was absorbed into plants, while 56% of recovered radioactivity from leaf-applied glufosinate had been absorbed (Table 1). When applied to stolons, recovered radioactivity from both herbicides was 78% to 80% absorbed (Table 1). Glufosinate had more radioactivity in shoots following leaf treatment than following stolon treatment. When applied to leaves, neither herbicide appreciably translocated to stolons after 3 d, but 14% to 24% of recovered radioactivity translocated from treated stolons to shoots (Table 1).
Although absorption of glyphosate or glufosinate into zoysiagrass has not been previously reported, Harker and Dekker (Reference Harker and Dekker1988) showed that 10% of foliar-applied glyphosate translocated to quackgrass [Elymus repens (L.) Gould] rhizomes when maintained at the same day/night temperatures of the current study. This result contrasts with our study on zoysiagrass, in which only 1% of recovered radioactivity had translocated to stolons 3 d after leaf treatment. Hoss et al. (Reference Hoss, Al-Khatib, Peterson and Loughin2003) found that prairie cupgrass (Eriochloa contracta Hitchc.) has higher translocation out of the treated leaf area following [14C]glyphosate treatment compared with [14C]glufosinate, which is similar to our results following treatment to zoysiagrass.
Research investigating herbicide absorption into grass stolons is still lacking in the literature. Results from our research suggest that herbicides applied to dormant zoysiagrass have potential to absorb into subcanopy stolons more readily than into leaves. In addition, absorption of radioactivity following treatment of either herbicide to stolons was rapid, as evidenced by scanning the 4-h harvest data (data not shown) or by multiplying slopes in Table 1 by three (i.e., number of days to 3-d harvest) and then adding the value to the observed values for 3 DAT in Table 1. For example, glyphosate applied to stolons had a slope of rinse radioactivity of −9.8, which yields −29.4% and added to the observed value at the 3-d harvest of 20% equals 49.4%. The actual mean for radioactivity in the rinse at 4 h after glyphosate treatment was 50.4% (data not shown). Results indicate that about half of the radioactivity had been absorbed into stolons within 4 HAT and suggest that irrigation would not be an effective means of reducing injury from direct spray deposition. The best way to safen zoysiagrass from direct spray deposition is to limit the percentage of green tissue present at application, but the absorption and translocation of glyphosate and glufosinate, especially in subcanopy stolons, suggest that reducing the amount of spray that reaches lower canopy levels could also reduce injury potential.
Spray Penetration Study
The interaction of year or operating pressure with nozzle type, height, or their interaction was not significant, so data were pooled to present the significant nozzle type by height interaction for the percent of colorant recovered at each canopy level (Table 2). It should be noted that the average recovery based on the targeted colorant application rate was 69 ± 14% (data not shown). Visual inspection of the thatch layer at the soil surface suggested that a large percentage of colorant was delivered to the soil surface and variable delivery to the soil may have contributed to the aforementioned recovery rate. When expressed as a percentage of recovered colorant, the majority of colorant was recovered from the upper canopy, and recovery from the middle and lower canopy levels exhibited an apparent stepwise reduction in all cases, although these comparisons were not statistically analyzed due to spatial variance structure. When the XR nozzle was operated at 61-cm height, 73% of the colorant was recovered from the top canopy layer, which was higher than all other evaluated treatments (Table 2). A decrease in recovered colorant was noted from the XR nozzle and 61-cm height combination in the middle (17%) and lower (11%) canopies as compared with either nozzle at the 25-cm height and to the TTI nozzle at the 61-cm height (Table 2). TTI nozzles at either height and XR nozzles at the 25-cm height deposited 56% to 59% of droplets in the upper canopy, 24% to 27% of droplets in the middle canopy, and 17% to 19% of droplets in the lower canopy (Table 2). Research findings were similar to those of Zhu et al. (Reference Zhu, Dorner, Rowland, Derksen and Ozkan2004), as they also documented that induction-type nozzles penetrate crop canopies more than XR nozzles. Researchers have demonstrated that droplets of size less than 150 µm are prone to drift and reduce subcanopy deposition to crops when applied at greater distances between nozzle and target (Yates et al. Reference Yates, Cowden and Akesson1985). Previous studies were often conducted under sustained or generated wind, while our methods avoided the influence of wind by choosing a wind-free day and actively avoiding spray during any detectable gusts.
Table 2. Influence of nozzle type and height above zoysiagrass turf on total colorant and colorant per tissue surface area extracted from three 1.9-cm canopy positions within 5.7-cm zoysiagrass canopy in spray penetration study. a, b, c
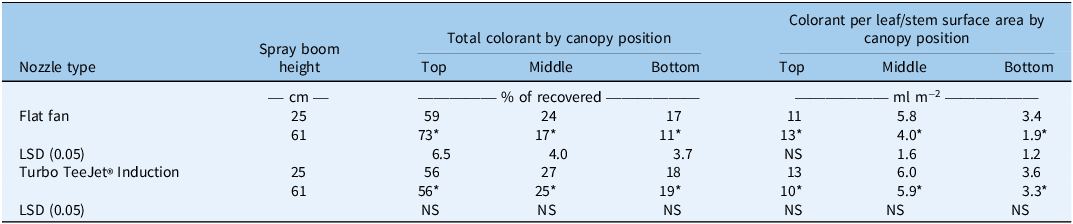
a Tissue surface area was estimated via an area meter for all excised portions of nontreated turf canopies and subjected to linear regression to determine its relationship to biomass (Figure 2). The resulting slope and intercept were used to estimate surface area based on biomass of all tissue that had been subjected to colorant extraction.
b Data were averaged over 2 yr (2019 and 2020) and two spray pressures (103 and 414 kPa)
c Means followed by an asterisk (*) are significantly different between nozzle types within a given spray boom height, and LSDs compare between spray boom height within a nozzle type.
Similar trends were noted in percentage recovery data when extracted colorant was expressed as quantity per square meter of plant tissue (Table 2), estimated based on the relationship of biomass to tissue surface area (Figure 2). Nozzle type by height interaction was significant (P < 0.05), and interactions composed of year or pressure were nonsignificant (P > 0.05). At 61-cm boom height, 13 ml of colorant m−2 was deposited to the upper canopy when XR nozzles were used compared with 10 ml m−2 deposited when sprayed using TTI nozzles (Table 2). However, reductions in colorant were observed in middle and lower canopy levels when the XR nozzles were used compared with TTI nozzles operated at 61 cm from the turf (Table 2). The TTI nozzles deposited equivalent amounts of colorant regardless of height above the turf, providing results similar to percentage recovery data (Table 2). Results suggest that substantial exposure of subcanopy tissue will occur irrespective of nozzle type, operating pressure, or boom height when the application volume is held constant at 374 L ha−1. Previous work has shown that application volume can increase the efficacy of fungicides when targeting subcanopy pathogens (Benelli et al. Reference Benelli, Horvath, Womac, Ownley, Windham and Sorachan2018), but future research is required to elucidate the effect of application volume along with other parameters to mitigate the risk of nonselective herbicide injury to dormant turfgrass. Practitioners could avoid induction nozzles and raise boom heights to impart better safety to zoysiagrass when using nonselective herbicides during dormancy.
Droplet Diameter and Velocity Study
A three-way interaction of nozzle type by operating pressure by spray boom height was significant (P = 0.0373) for droplet velocity, but further analysis by nozzle type showed that TTI nozzles were not significantly influenced by height with respect to droplet velocity. The relationship of droplet diameter to droplet velocity is shown as second-order polynomial regressions comprising all observations averaged over both heights for TTI nozzles and the interaction of pressure by height for XR nozzles (Figure 4). As the data comprised hundreds of randomly selected droplets, they may partially suggest the distribution of droplet diameters. However, the main purpose was to determine droplet velocity and to calculate volume median diameter by nozzle type and pressure (Figure 4).
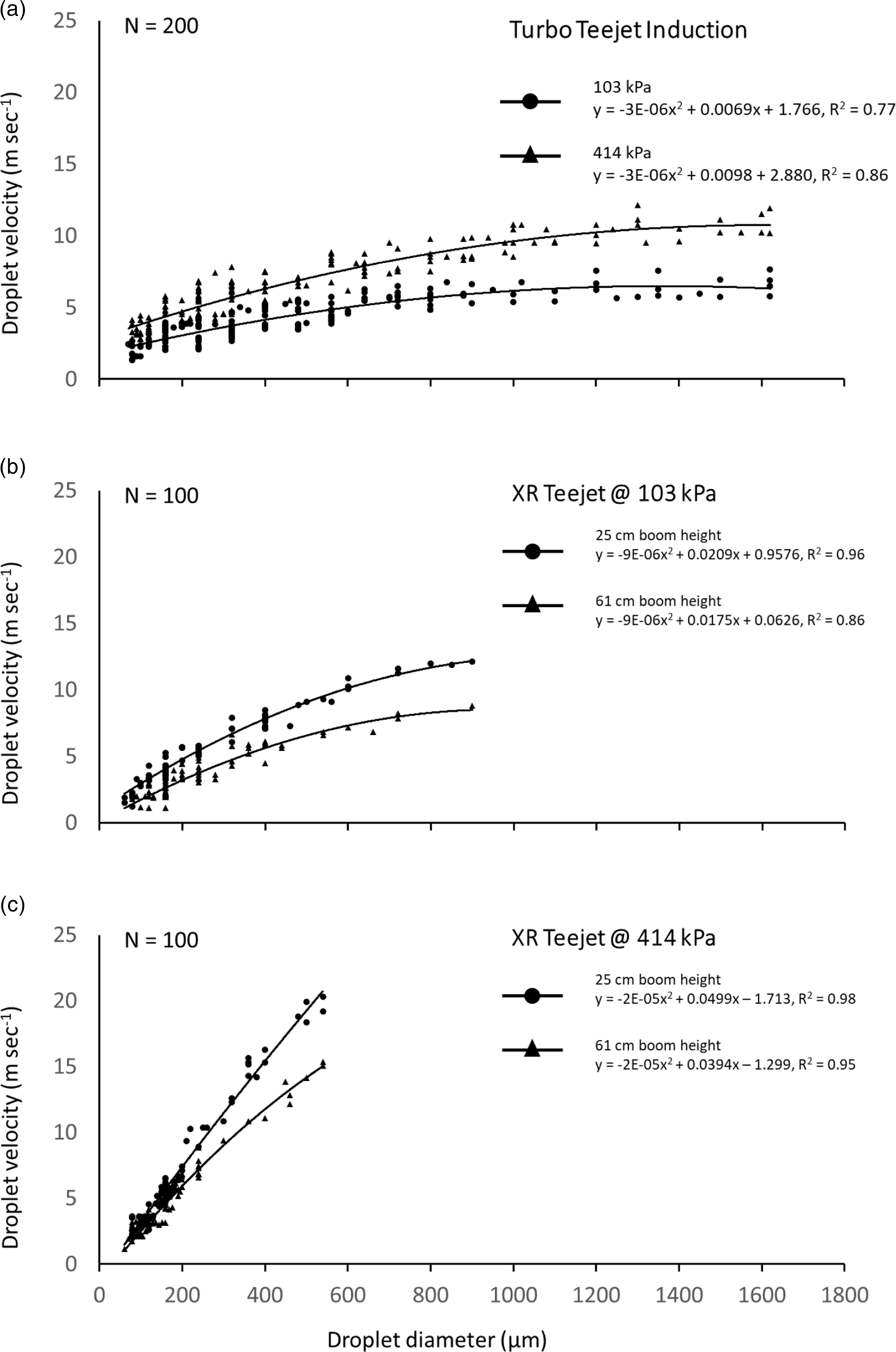
Figure 4. Relationship between horizontal droplet diameter and droplet velocity from Turbo TeeJet® Induction spray tips at 103 and 414 kPa, averaged over 25- and 61-cm boom heights (A), XR TeeJet® flat-fan spray tips at (B) 103 and (C) 414 kPa at separated by boom height.
In all cases, droplet velocity increased with increasing droplet diameter, but these trends were dependent on the nozzle type, pressure, and in the case of XR nozzles, also on the height above the target (Figure 4). TTI nozzles exhibited a range of measurable droplet sizes between 90 and 1,620 µm regardless of pressure (Figure 4). Increasing pressure did, however, increase droplet velocity from TTI nozzles by about 2.5 to 5.0 m s−1 for droplets ranging from 400 to 1,620 µm (Figure 4). In contrast, the range of droplet diameters for XR nozzles was strongly influenced by pressure, being 60 to 900 µm at 103 kPa and 60 to 540 µm at 414 kPa (Figure 4). Increasing pressure from 103 kPa to 414 kPa nearly doubled droplet velocity from XR nozzles at droplet diameters greater than 200 µm (Figure 4). Unlike TTI nozzles, increasing height above the target had a substantial effect on droplet velocity from XR nozzles at either pressure with droplets >200 µm increasing by 2 to 4 m s−1 (Figure 4). These data agree with previously measured droplet velocities, showing that change in droplet speed with the change in distance from the target has also been calculated and measured (Goering et al. Reference Goering, Bode and Gebhardt1972).
The operating pressure influenced droplet velocity for both XR and TTI nozzles (Figure 4), but only XR nozzles exhibited a difference in deposition at various zoysiagrass canopy layers (Table 2). Results suggest that droplet diameter and associated mass may have more influence on canopy penetration than velocity. The lack of difference in canopy penetration by TTI nozzles at different observed droplet velocities may also be influenced by the air-entrained design of droplets generated by induction nozzles. These droplets are designed to splatter upon impact (Grayson et al. Reference Grayson, Webb, Pack and Edwards1991) and could result in a more uniform distribution in lower turfgrass canopies regardless of droplet speed. Despite slight differences in lower canopy penetration that we have attributed partially to a greater distance between XR nozzles and the turf, the fact remains that all canopy layers are exposed to a large percentage of droplets regardless of nozzle type, operating pressure, or boom height. Our findings imply that underlying stems can be exposed at any level of zoysiagrass dormancy.
Carrier Volume Study
The interaction of carrier volume by temporal run was nonsignificant, so data composed of percent colorant extracted from papers at the lower canopy position were pooled over temporal runs. The regression analysis revealed a positive correlation, and carrier volume variability explained almost 92% of the variation in recovered colorant from the lower canopy position (Figure 5). At a carrier volume of 60 L ha−1, 23% of the total colorant applied was recovered from the lower canopy position, while 37% of the total colorant was recovered when applied at 584 L ha−1 of carrier volume (Figure 5). With a nearly 10-fold reduction in carrier volume from 584 L ha−1 to 60 L ha−1, the colorant penetration in the lower canopy position was reduced by 38% (Figure 5). Linear regression analysis indicated that the intercept for percent colorant recovered from lower canopy position was 23%, and with each 100 L ha−1 of addition in carrier volume, the percent colorant recovered from lower canopy increased by 2.3% (Figure 5). Research findings were similar to those of Sharpe et al. (Reference Sharpe, Boyd, Dittmar, MacDonald, Darnell and Ferrell2018), who demonstrated that spray droplet penetration increased in the strawberry lower canopy with increasing application volume. Our data were based on the recovery of colorant, which was used as a proxy for herbicide. It is important to note that increased carrier volume not only increases the delivery of our measured colorant but would also vastly increase the quantity of fluid delivered to lower canopies and, thus, the potential delivery of herbicides to subcanopy stolons via improved coverage.
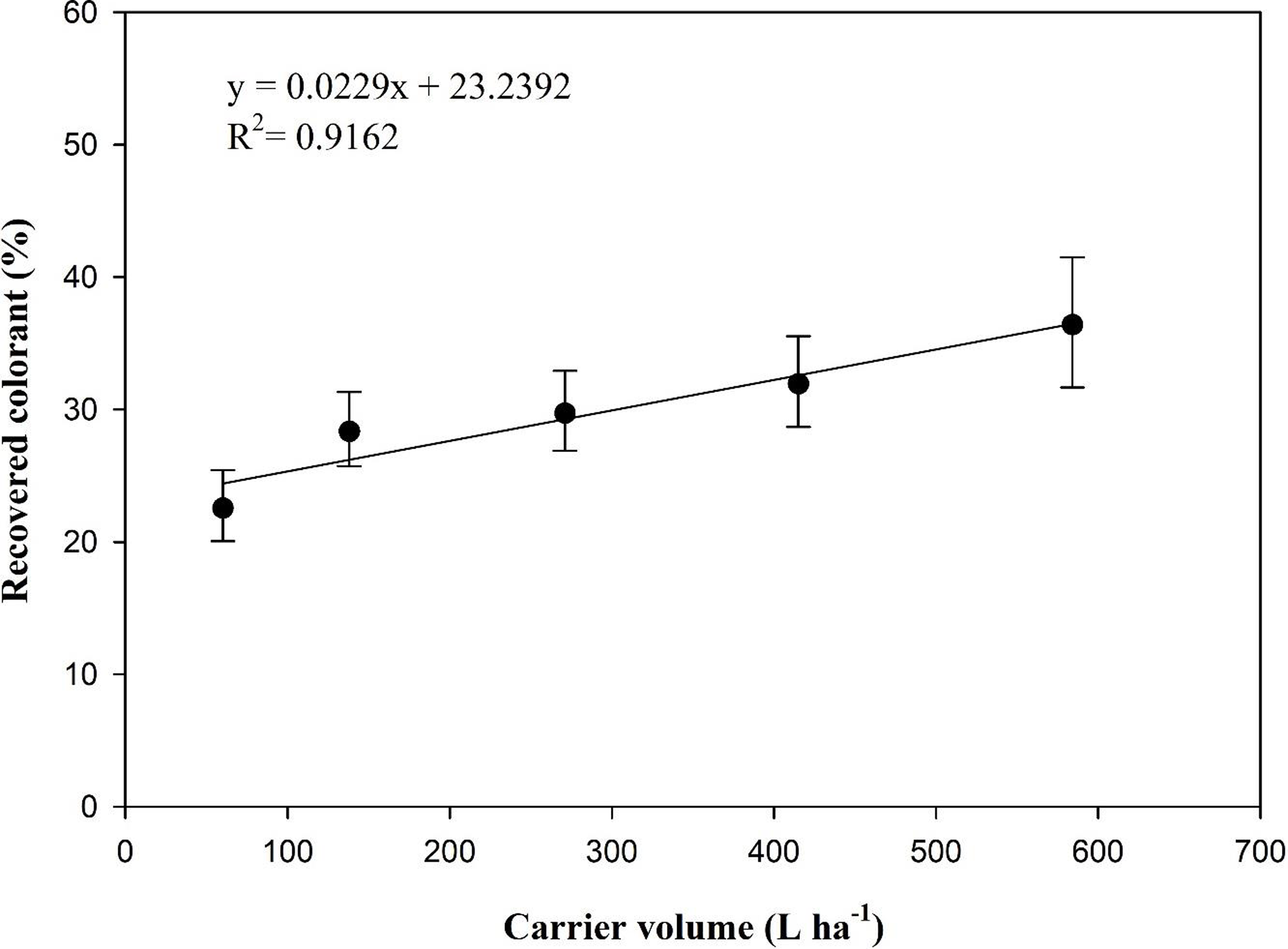
Figure 5. Percent of recovered colorant from lower canopy position in dormant zoysiagrass from the study evaluated the effect of carrier volume on spray penetration in dormant zoysiagrass canopy.
Most nozzle, pressure, and height configurations tested in the canopy penetration study indicated that as much as 19% of applied droplets will be deposited to the lower canopy (Table 2). Our experiments did not account for dead sheath material that would shield a large percentage of subcanopy zoysiagrass stems, as these were removed to reduce error associated with the absorption study. With the increase in number of green leaves in the zoysiagrass canopy, the risk associated with herbicide injury also increases exponentially, as observed by Craft et al. (Reference Craft, Godara, Brewer and Askew2023b) when glyphosate or glufosinate was applied to partially green zoysiagrass canopies. Our data suggest that subcanopy stolons can also readily absorb both glyphosate and glufosinate. By increasing spray height, avoiding induction-type nozzles, and adopting low-volume application strategies, practitioners can limit the spray penetration into lower levels of zoysiagrass turf canopies and potentially mitigate injury from nonselective herbicides during post-dormancy transition.
Acknowledgments
The authors received no specific funding for conducting this research. The authors declare no conflict of interest.