Introduction
Knotweed (Fallopia spp.) is a highly competitive, invasive, herbaceous perennial that has spread from its native range in Japan, China, and Korea to Europe and then to North America. It was first introduced to the United States in 1873 (Barney Reference Barney2006) and has since been widely distributed by humans as an ornamental plant. The knotweed complex includes Japanese knotweed [Fallopia japonica (Houtt.) Ronse Decr.; syn.: Polygonum cuspidatum Siebold & Zucc.], a dwarf-type Japanese knotweed [Fallopia japonica var. compacta (Hook.f.) J.P. Bailey], giant knotweed [Fallopia sachalinensis (F. Schmidt) Ronse Decr.; syn.: Polygonum sachalinense F. Schmidt ex Maxim.], and their hybrid, Bohemian knotweed [Fallopia × bohemica (Chrtek and Chrtková) J.P. Bailey; syn.: Polygonum× bohemica (J. Chrtek & Chrtková) Zika & Jacobson [cuspidatum× sachalinense]. The use of the term “knotweed” throughout this paper will refer only to these two species and their hybrid. Knotweed can spread prolifically via both asexual propagation (rhizomes and adventitious rooting) and sexual reproduction (seeds) (Bailey et al. Reference Bailey, Bímová and Mandák2008). Knotweed spread is also greatly facilitated by its propensity to inhabit riparian areas (Bímová et al. Reference Bímová, Mandák and Pyšek2001). Knotweed seeds are highly buoyant in water, allowing them to travel away from the mother plant to establish new knotweed populations (Lamberti-Raverot et al. Reference Lamberti-Raverot, Piola, Thiébaut, Guillard, Vallier and Puijalon2017). A study also found that even though most seeds fall close to the maternal clone, seeds can disperse via wind up to 16 m (Tiébré et al. Reference Tiébré, Vanderhoeven, Saad and Mahy2007). Additionally, rhizome fragments as small as 1 cm (0.7 g) can give rise to new plants (Bailey et al. Reference Bailey, Bímová and Mandák2008). The large number of wetlands, lakes, streams, and rivers in Minnesota makes the state particularly susceptible to the effects of a knotweed invasion. However, little research has been conducted on the spread of knotweed in Minnesota and other northern Midwest states. The primary goal of this literature review is to discuss research on the mechanisms of dispersal, genetics, and growth habit of knotweed to better understand its invasiveness with a secondary goal of proposing necessary future research specifically for the state of Minnesota.
It is important to note at the onset of this literature review that a great deal of research on the knotweed complex has been reported on European populations. Thus, much of our understanding of knotweeds is specific to Europe, which is even more reason to conduct research in Minnesota to have the geographic specificity necessary to make accurate management decisions.
Classification
The taxonomic classification of knotweed has changed numerous times since its initial classification. Fallopia japonica was originally classified as Reynoutria japonica by Houttuyn in 1777 (Bailey and Conolly Reference Bailey and Conolly2000; Beerling et al. Reference Beerling, Bailey and Conolly1994; Table 1). In 1848 it was reclassified as Polygonum sieboldii Reinw. (= Polygonum cuspidatum Sieb. & Zucc.) in reference to Phillipe von Siebold, who originally brought specimens to the Netherlands from his sojourn in Japan (Bailey and Conolly Reference Bailey and Conolly2000). These two names were combined by Makino in 1901 to create the new nomenclature Polygonum reynoutria Makino (Bailey and Conolly Reference Bailey and Conolly2000). The knotweed complex was first classified in the genus Fallopia by Decraene and Akeroyd in Reference Decraene and Akeroyd1988 (Bailey and Stace Reference Bailey and Stace1992; Decraene and Akeroyd Reference Decraene and Akeroyd1988). Throughout the literature, authors use the Polygonum, Reynoutria, and Fallopia genera. Confusion with the nomenclature of knotweed continues due to the use of multiple specific epithets. Different countries also have different preferences on the nomenclature they use (Bailey and Wisskirchen Reference Bailey and Wisskirchen2006).
Table 1. Summary of change in nomenclature of Fallopia japonica since its first classification in 1777.

a Bailey and Conolly Reference Bailey and Conolly2000.
b Beerling et al. Reference Beerling, Bailey and Conolly1994.
c Bailey and Stace Reference Bailey and Stace1992.
d Decraene and Akeroyd Reference Decraene and Akeroyd1988.
Distribution and Spread
Fallopia japonica was commercially available in Europe in 1848 (Bailey and Conolly Reference Bailey and Conolly2000). In England, the first record of F. japonica dates to the late 1840s (Bailey Reference Bailey1994). The initial introduction of F. japonica to England was a single male-sterile clone that successfully spread and created a massive knotweed infestation that exists across the United Kingdom today (Bailey et al. Reference Bailey, Bímová and Mandák2008; Hollingsworth and Bailey Reference Hollingsworth and Bailey2000). The earliest herbarium record of F. japonica in the United States is from 1873 (Barney Reference Barney2006). Knotweed (F. japonica) was being sold in Minnesota as early as 1908 (Figure 1) (The Jewell Nursery Co. 1908), but residents could have purchased knotweed earlier from nurseries on the East Coast of the United States (Maule’s Seed Catalogue Reference Maule1895; James Vick’s Sons 1898).
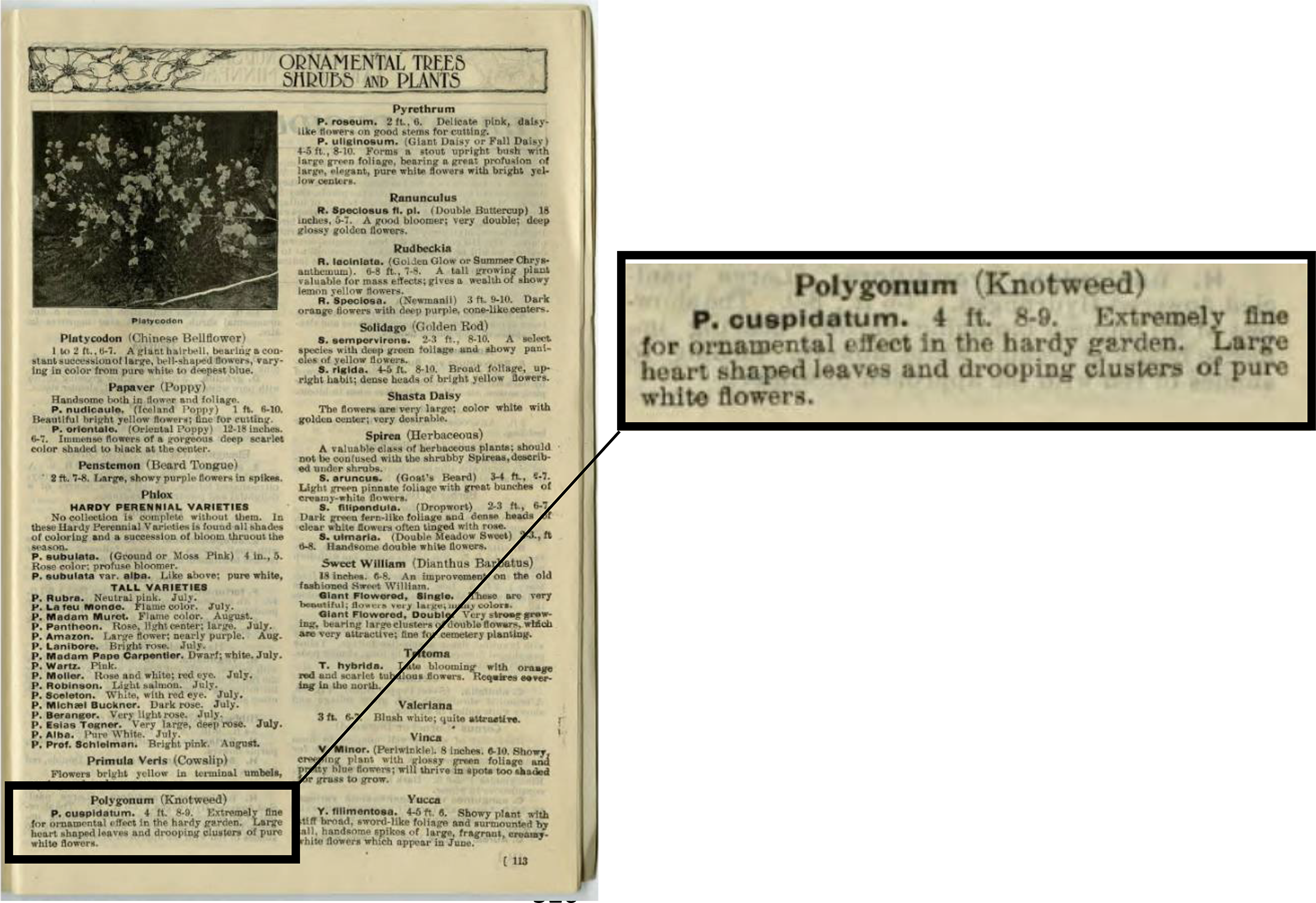
Figure 1. An advertisement from the 1908 catalogue Jewell Trees, Seeds and Plants advertising Fallopia japonica (known as Polygonum cuspidatum at the time) for sale in Minnesota. https://umedia.lib.umn.edu/item/p16022coll265:2855?q=polygonum+cuspidatum.
Fallopia sachalinensis arrived in Europe in 1864 at the botanic gardens of St. Petersburg, Russia (Bailey and Wisskirchen Reference Bailey and Wisskirchen2006). Fallopia × bohemica was not recorded in Europe until the 1980s, when it was first described by Chrtek and Chrtková, although it is now known to have occurred earlier, as early as 1872, and spread undetected (Bailey and Wisskirchen Reference Bailey and Wisskirchen2006). The undetected spread is primarily due to the difficulty in visually identifying the hybrid (Bailey and Wisskirchen Reference Bailey and Wisskirchen2006). Morphological traits of F. × bohemica are variable and can exhibit traits of F. japonica or F. sachalinensis. Fallopia × bohemica was confirmed in the United States in 2001 (Bailey and Wisskirchen Reference Bailey and Wisskirchen2006). Interestingly, F. × bohemica was not described in its native range in Japan until 1997 because the parental species were not sympatric in Japan until that time (Bailey Reference Bailey2003).
A total of 47% of 92 knotweed populations sampled in the first transcontinental genetic study of knotweed in the United States were identical to the British male-sterile clone of F. japonica (Grimsby and Kesseli Reference Grimsby and Kesseli2009); this included one population sampled from Duluth, MN (Grimsby and Kesseli Reference Grimsby and Kesseli2009; see supplemental information here: http://www.genetics.umb.edu). As many as 54% of samples in this study were found to be F. japonica (50 samples), 3% F. sachalinensis (3 samples), and 42% F. × bohemica (39 samples). This contrasts with a study of knotweed in the western United States that found F. × bohemica to be more common than F. japonica with a ratio of 5:1 (Gaskin et al. Reference Gaskin, Schwarzländer, Grevstad, Haverhals, Bourchier and Miller2014). The difference between these two studies could potentially be attributed to the fact that the Grimsby and Kesseli study specifically requested collaborators to collect “Japanese knotweed,” which may have dissuaded collectors from sending in samples of other taxa. There is potential that the composition of knotweed taxa in Minnesota is similar to that seen in other areas across the United States, but without a thorough sampling and genetic testing, it is impossible to know which species is most prevalent.
Invasiveness
It is necessary to first understand what unique characteristics make knotweed such a strong invader in order to ultimately control it. Invasive nonnative plant species have many effects on environments in their adventive ranges. Invasive alien plants reduce the overall fitness and growth of local plant species, decrease plant species abundance and diversity, and decrease animal species’ fitness and abundance (Vilà et al. Reference Vilà, Espinar, Hejda, Hulme, Jarošík, Maron, Pergl, Schaffner, Sun and Pyšek2011). Knotweeds have been shown to reduce the overall biomass of macroinvertebrates in their stands by up to 60% and also negatively impact the biomass, cover, and species richness of native plants (Lavoie Reference Lavoie2017). It has been found that 1.6 to 10 times as many species grow outside knotweed stands as compared to within (Aguilera et al. Reference Aguilera, Alpert, Dukes and Harrington2010). Knotweeds also negatively impact riparian areas by changing leaf litter nitrogen composition (Urgenson Reference Urgenson2006) and reducing ecosystem services such as access to riverbanks (Kidd Reference Kidd2000). Knotweeds are also often considered aesthetically displeasing (Kidd Reference Kidd2000).
Managing and eradicating invasive plants can also be extremely costly. An estimated US$500 million is spent yearly on the management of nonnative plant species just on residential properties alone in the United States (Pimentel et al. Reference Pimentel, Zuniga and Morrison2005). It would cost an estimated €32.3 million (US$38 million) annually to control all the knotweed populations in Germany (Reinhardt et al. Reference Reinhardt, Herle, Bastiansen and Streit2003).
Reproduction
Knotweed can spread asexually by both rhizomes and adventitious rooting of stem fragments (Bailey et al. Reference Bailey, Bímová and Mandák2008). Knotweed primarily spreads via rhizome dispersal occurring from floods or human activity in the adventive range (Bailey et al. Reference Bailey, Bímová and Mandák2008). Reproduction via adventitious rooting of stem fragments results in lower levels of regeneration for F. japonica and F. × bohemica as compared with regeneration from rhizomes. However, F. sachalinensis has higher levels of regeneration from adventitious rooting of stem fragments and only low levels of regeneration when grown from rhizomes (Bímová et al. Reference Bímová, Mandák and Pyšek2003). Overall, vegetative regeneration is highest in F. × bohemica (Bímová et al. Reference Bímová, Mandák and Pyšek2003). Knotweeds form shoot clumps or crowns composed of dead shoots from previous growth years and underground wintering buds that give rise to new aerial shoots in the spring (Bailey et al. Reference Bailey, Bímová and Mandák2008; Dauer and Jongejans Reference Dauer and Jongejans2013). Size of crowns varies between species, with F. japonica having larger crowns than F. sachalinensis, while the hybrid, F. × bohemica, has an intermediate crown size (Bailey et al. Reference Bailey, Bímová and Mandák2008).
Fallopia japonica frequently reproduces via seed in its native range (Bailey Reference Bailey2003; Bram and Mcnair Reference Bram and Mcnair2004) and has been reported to reproduce via seed in the adventive range (Bram and Mcnair Reference Bram and Mcnair2004; Forman and Kesseli Reference Forman and Kesseli2003), although this is thought to be less common than asexual reproduction (Bailey et al. Reference Bailey, Bímová and Mandák2008). Knotweed produces prolific seed, with a study of F. japonica and F. sachalinensis in Pennsylvania reporting 50,000 to 150,000 seeds annually per stem (Niewinski Reference Niewinski1998). Knotweeds have high germination rates of up to 92% (field germinations) (Bram and Mcnair Reference Bram and Mcnair2004), 93% (dried seed) (Groeneveld et al. Reference Groeneveld, Belzile and Lavoie2014), and even up to 100% (overwintered seed) (Forman and Kesseli Reference Forman and Kesseli2003). Germination has been shown to rely on seed maturity levels (Bram and Mcnair Reference Bram and Mcnair2004). Knotweeds are dioecious, but there are known cases of gynodioecious plants in each taxa (Bailey et al. Reference Bailey, Bímová and Mandák2008; Beerling et al. Reference Beerling, Bailey and Conolly1994; Holm et al. Reference Holm, Elameen, Oliver, Brandsæter, Fløistad and Brurberg2018; Karaer et al. Reference Karaer, Terzioğlu and Kutbay2020; Niewinski Reference Niewinski1998). There are also reports of androdioecious knotweed from the Amami Islands of Japan (Mitsuru Hotta, personal communication, in Bailey Reference Bailey2003). There is currently no research on knotweed pollen viability reported in the literature. Sexual reproduction may not be an important management concern if there is no pollen donor present. On the other hand, if many viable pollen donors are present, then sexual reproduction is a major concern and a priority for management decisions. That is why it is critical that research be conducted on male and female fertility of knotweed.
Structure of Growth
A study in the Czech Republic found that, on average, invasive species as a whole were 1.2 m taller than native species across all habitat types (Divíšek et al. Reference Divíšek, Chytrý, Beckage, Gotelli, Lososová, Pyšek and Molofsky2018). Knotweeds are incredibly tall and range in height from 2 to 4 m thus shading out other plants (Bailey et al. Reference Bailey, Bímová and Mandák2008; Bímová et al. Reference Bímová, Mandák and Pyšek2001). Fallopia japonica has a smaller overall stature ranging from 2 to 3 m in height; F. sachalinensis is the tallest of the species and reaches 4 m in height; while the hybrid F. × bohemica has the greatest range in height of 2.5 to 4 m (Bailey et al. Reference Bailey, Bímová and Mandák2008). Knotweeds create a monoculture (Figure 2) with large leaves that form an extremely dense canopy, shading other plants throughout the majority of the growing season, making it difficult for smaller plants to grow in the same area (Bailey et al. Reference Bailey, Bímová and Mandák2008; Siemens and Blossey Reference Siemens and Blossey2007). This is especially true of F. sachalinensis, whose leaves can reach 40 cm in length (Bailey and Stace Reference Bailey and Stace1992). However, it is worth noting that Moravcová et al. (Reference Moravcová, Pyšek, Jarošik and Zákravský2011) concluded that shading is unlikely the primary invasive mechanism of knotweeds, as light treatment studies yielded inconclusive results.
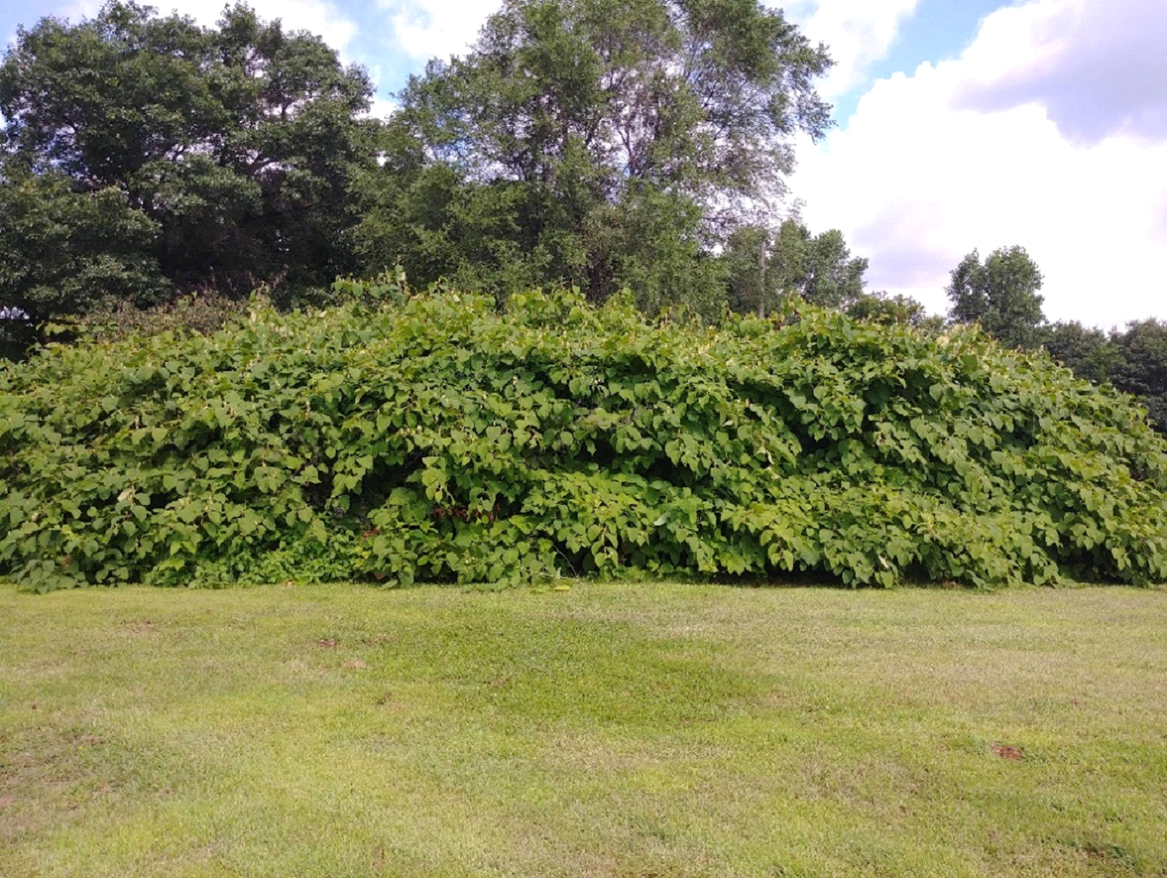
Figure 2. Example of a knotweed monoculture growing in Minnesota. Photograph shows a Fallopia × bohemica population from Brooklyn Center, MN, on August 16, 2019.
It is generally accepted that knotweed rhizomes can grow up to 7 m from the crown of origin, but recent research has shown that F. japonica rhizomes typically extend no more than 4 m (Fennell et al. Reference Fennell, Wade and Bacon2018). Still, knotweed rhizomes are a formidable opponent, as they can grow up through asphalt (Wade et al. Reference Wade, Child and Adachi1996). They can also cause bank destabilization when growing alongside water bodies, as the rhizomes are less able to bind soil together compared with some native riparian plants (Reinhardt et al. Reference Reinhardt, Herle, Bastiansen and Streit2003).
Soil Conditions
Knotweed is an early successional species growing on volcanic ash and recent lava flows in its native habitat in Japan (Bailey et al. Reference Bailey, Bímová and Mandák2008; Barney et al. Reference Barney, Tharayil, Ditommaso and Bhowmik2006). The range of habitats and soil types it is known to grow in is extremely diverse. It can be found across 35 latitudinal degrees and grows from sea level to 3,500 m above sea level (Bailey Reference Bailey2003). It often grows in riparian and ruderal areas, areas experiencing human disturbances, forest margins, urban landscapes, and gardens (Bailey et al. Reference Bailey, Bímová and Mandák2008; Clements et al. Reference Clements, Larsen and Grenz2016; Mandák et al. Reference Mandák, Pyšek and Bímová2004). It grows on a variety of terrains, including sandy soils, swamps, rocky banks, and alluvial floodplains (Barney et al. Reference Barney, Tharayil, Ditommaso and Bhowmik2006). Knotweeds have highly plastic salt-tolerance traits and are now known to grow in salt marsh habitats in the eastern United States (Richards et al. Reference Richards, Walls, Bailey, Parameswaran, George and Pigliucci2008). Furthermore, knotweeds are known to grow in soils with high concentrations of metal pollutants (Michalet et al. Reference Michalet, Rouifed, Pellassa-Simon, Fusade-Boyer, Meiffren, Nazaret and Piola2017). Indeed, knotweed growth rates are greater in soils with average concentrations of metallic pollutants (2 mg kg−1 Cd, 150 mg kg−1 Cr, 100 mg kg−1 Pb, and 300 mg kg−1 Zn) compared with unpolluted soil (Michalet et al. Reference Michalet, Rouifed, Pellassa-Simon, Fusade-Boyer, Meiffren, Nazaret and Piola2017). Fallopia × bohemica accumulated the greatest concentration of metals relative to either F. japonica or F. sachalinensis (Michalet et al. Reference Michalet, Rouifed, Pellassa-Simon, Fusade-Boyer, Meiffren, Nazaret and Piola2017).
Allelopathy
Fallopia japonica contains chemicals with the potential to cause allelopathic effects; these chemicals include resveratrol, resveratroloside, piceid, piceatannol glucoside, polydatin, emodin, and catechins (Serniak Reference Serniak2016; Vastano et al. Reference Vastano, Chen, Zhu, Ho, Zhou and Rosen2000). Fallopia × bohemica has also been found to have allelopathic effects on nearby plants, particularly affecting seed germination and seedling growth (Siemens and Blossey Reference Siemens and Blossey2007). One study found that mechanical control of F. × bohemica via stem cutting causes an overall reduction in production of allelochemicals (Murrell et al. Reference Murrell, Gerber, Krebs, Parepa, Schaffner and Bossdorf2011). Fallopia sachalinensis also has allelopathic capabilities and has been shown to have the greatest phytotoxic effects on other plants (Moravcová et al. Reference Moravcová, Pyšek, Jarošik and Zákravský2011).
The highest level of phenolic compounds is found in the rhizomes (Vaher and Koel Reference Vaher and Koel2003). However, the decomposition of knotweed litter from each of the taxa also has phytotoxic effects on other plants (Moravcová et al. Reference Moravcová, Pyšek, Jarošik and Zákravský2011). These allelopathic chemicals are significant, because they greatly increase knotweed’s invasive and competitive ability.
Genetic Diversity
Knotweed has higher levels of genetic diversity in its native range than its invasive range (Bailey Reference Bailey2003). There are also many more subspecies and varieties of knotweed in the native range compared with the adventive range (Bailey Reference Bailey2003; Inamura et al. Reference Inamura, Ohashi, Sato, Yoda, Masuzawa, Ito and Yoshinaga2000). Hybridization of knotweed in Japan is limited, which differs from the knotweed found in Europe and North America, where hybridization is common (Bailey Reference Bailey2003; Grimsby et al. Reference Grimsby, Tsirelson, Gammon and Kesseli2007). Clonal invasive species that reproduce asexually typically have lower genetic variation (Bailey Reference Bailey2003), so it would follow that invasive knotweed would have low genetic variability; however, the ability of an invasive to hybridize can increase its invasive success (Ellstrand and Schierenbeck Reference Ellstrand and Schierenbeck2006). Indeed, F. × bohemica shows heterosis in that it is more invasive than its parents (Parepa et al. Reference Parepa, Fischer, Krebs and Bossdorf2014), spreads faster than both parents (Mandák et al. Reference Mandák, Pyšek and Bímová2004), and has a higher regenerative ability than its parents (Bímová et al. Reference Bímová, Mandák and Pyšek2003).
Knotweed in the United Kingdom shows interspecific diversity, with F. japonica, F. × bohemica, and F. sachalinensis all clustering separately in diversity analyses and F. × bohemica clustering two-thirds closer to F. japonica than F. sachalinensis (Hollingsworth et al. Reference Hollingsworth, Hollingsworth, Jenkins, Bailey and Ferris1998). This could potentially be due to multiple backcrossing events. It was found that F. japonica and F. × bohemica are most genetically similar, whereas F. japonica and F. sachalinensis are the least similar (Holm et al. Reference Holm, Elameen, Oliver, Brandsæter, Fløistad and Brurberg2018). It has also been found that F. × bohemica shows higher diversity compared with either parent, which could be explained by spread via sexual reproduction (Hollingsworth et al. Reference Hollingsworth, Hollingsworth, Jenkins, Bailey and Ferris1998).
Low genetic diversity was found for all three of the knotweed taxa across Norway (Holm et al. Reference Holm, Elameen, Oliver, Brandsæter, Fløistad and Brurberg2018). Due to these low levels of genetic variation, it was concluded that knotweed likely has not reproduced sexually in Norway (Holm et al. Reference Holm, Elameen, Oliver, Brandsæter, Fløistad and Brurberg2018).
Within-species genetic diversity was found to be low across all taxa in a study of knotweeds in Poland and Japan (Bzdega et al. Reference Bzdega, Janiak, Książczyk, Lewandowska, Gancarek, Sliwinska and Tokarska-Guzik2016), with F. japonica and F. sachalinensis showing the lowest levels of polymorphism. Fallopia japonica populations in this study were not found to be a single clone.
Fallopia japonica spreads exclusively by vegetative reproduction, and the clones are monotypic in the western United States (Gaskin et al. Reference Gaskin, Schwarzländer, Grevstad, Haverhals, Bourchier and Miller2014). The Gaskin et al. (Reference Gaskin, Schwarzländer, Grevstad, Haverhals, Bourchier and Miller2014) study used amplified fragment length polymorphisms (AFLPs) to compare F. japonica with multiple samples of the clone that invaded the United Kingdom and found them to be genetically identical. Fallopia sachalinensis was also found to spread primarily by vegetative means and was mostly monotypic in the western United States (Gaskin et al. Reference Gaskin, Schwarzländer, Grevstad, Haverhals, Bourchier and Miller2014). However, F. × bohemica differed from its parents, in that it was found to spread by both asexual and sexual mechanisms, had the lowest number of monotypic populations, the highest proportion of loci that are polymorphic, and the highest genetic diversity (Gaskin et al. Reference Gaskin, Schwarzländer, Grevstad, Haverhals, Bourchier and Miller2014).
A study in Massachusetts that used simple sequence repeat (SSR) markers also found the UK clone of F. japonica in all three of the populations surveyed (Grimsby et al. Reference Grimsby, Tsirelson, Gammon and Kesseli2007). This study found 26 genotypes from 66 samples across three distinct F. japonica populations. They also found evidence for sexual spread of knotweed in Massachusetts, as most knotweed patches were composed of unique genets that were not found in other patches.
A transcontinental study of knotweed analyzed 92 locations across the United States and, using SSR markers, identified 36 genotypes (Grimsby and Kesseli Reference Grimsby and Kesseli2009). Fallopia × bohemica had the most diversity, as it was composed of 26 genotypes, while F. japonica samples were made up of 8 genotypes, and F. sachalinensis had only 2 genotypes. The UK clone of F. japonica was also detected in this study.
Another study used random amplified polymorphic DNA analysis to study the genetic diversity of F. japonica along two creeks in Kentucky (Wymer et al. Reference Wymer, Gardner, Steinberger and Peyton2007). The authors found no evidence of asexual spread and concluded that the genetic diversity that did exist resulted from multiple introductions.
Populations of F. japonica and F. × bohemica have been shown to have a large amount of epigenetic diversity. Epigenetic diversity occurs through processes such as DNA methylation or histone modification instead of DNA base pair changes. One study used AFLP genetic diversity markers to measure genetic diversity, which were then compared with epigenetic diversity levels found using methylation-sensitive AFLP epigenetic diversity markers that could identify methylated cytosine (Richards et al. Reference Richards, Schrey and Pigliucci2012). The authors found that a single clone of F. japonica contained 129 epigenotypes, even though it was only composed of one genotype and had no genetic variation (Richards et al. Reference Richards, Schrey and Pigliucci2012). This study also analyzed F. × bohemica and found 85 epigenotypes, but only 7 genotypes, across 155 individuals. Both F. japonica and F. × bohemica showed higher levels of epigenetic variation than genetic variation, with the epigenetic variation for F. × bohemica being 10 times higher than its genetic variation. This is important, because epigenetic variation is one explanation for the phenotypic diversity and successful establishment of clonally spread F. japonica in diverse environments with invasive populations that have low genetic variation. (Banerjee et al. Reference Banerjee, Guo and Huang2019).
In a study of central European F. japonica, a single genotype of F. japonica contained 27 different epigenotypes (Zhang et al. Reference Zhang, Parepa, Fischer and Bossdorf2016). The authors also found that the epigenetic variation was a full order of magnitude higher than the genetic variation. Their study was able to correlate epigenetic diversity with both phenotypic diversity and the climate from which the F. japonica population originated. Notably, F. japonica varied in some key phenotypic traits associated with invasiveness, such as specific leaf area. They concluded this correlation could potentially lead to habitat adaptation, which could explain how a single clone of F. japonica was able to become such a strong invader across much of Europe.
It is important that a genetic diversity study be conducted in Minnesota, because it has been shown that each congener can react differently to different control methods (Bímová et al. Reference Bímová, Mandák and Pyšek2001). It has also been shown that F. japonica, F. sachalinensis, and F. × bohemica all react differently to biological control with the psyllid Aphalara itadori Shinji (Grevstad et al. Reference Grevstad, Shaw, Bourchier, Sanguankeo, Cortat and Reardon2013). Thus, it is imperative that land managers and homeowners know exactly which taxa is invading an area so that they can choose the most effective control method.
Ploidy and Cytogenetics
Knotweed has a base chromosome number of x = 11 (Bailey and Stace Reference Bailey and Stace1992). In Japan, high-altitude dwarf F. japonica has been found as a tetraploid (2n = 4x = 44), and tall lowland F. japonica has been found as tetraploid and octoploid (2n = 8x = 88; Bailey Reference Bailey2003). Limited sampling found F. japonica from China to be octoploid and decaploid (Bailey Reference Bailey2003). Fallopia sachalinensis is tetraploid in its native range (Bailey Reference Bailey2003) with the exception of Korean F. sachalinensis being dodecaploid (Kim and Park Reference Kim and Park2000).
In the adventive range, F. japonica var. japonica has been found to be octaploid, and F. japonica ‘Compacta’ has been found as a tetraploid (Mandák et al. Reference Mandák, Pyšek, Lysák, Suda, Krahulcová and Bímová2003). There have been no reports of F. japonica as a diploid. Fallopia sachalinensis has been found as a mixture of tetraploid, hexaploid, and octoploid, and F. × bohemica is primarily hexaploid with evidence for tetraploids and octoploids as well (Mandák et al. Reference Mandák, Pyšek, Lysák, Suda, Krahulcová and Bímová2003). The hybrid created between F. sachalinensis and Compacta has also been found as a tetraploid (Bailey and Stace Reference Bailey and Stace1992). Even though F. × bohemica can be crossed with itself or either parent, resulting in a range of euploid and aneuploid progeny, it is primarily found in nature in a euploid state as a tetraploid, hexaploid, octoploid, or infrequently a decaploid (Bailey and Wisskirchen Reference Bailey and Wisskirchen2006).
Tetraploid knotweed shows normal bivalent pairing in meiosis and a low level of chiasma (Bailey and Stace Reference Bailey and Stace1992). Tetraploid and octoploid F. × bohemica have a more normal meiosis than the hexaploid F. × bohemica (Bailey and Stace Reference Bailey and Stace1992). The hexaploid F. × bohemica shows irregular meiosis that consists of numerous univalents and multivalents not surpassing quadrivalents (Bailey and Stace Reference Bailey and Stace1992). The DNA 2C-values per 2x genome of the taxa ranged from 1.23 to 1.62 pg, with F. sachalinensis at 1.33 pg, Compacta at 1.29 pg, F. japonica ranging from 1.30 to 1.62 pg, and F. × bohemica ranging from 1.23 to 1.59 pg (Bailey and Stace Reference Bailey and Stace1992). This paper also posits that the tetraploids are much older than the octoploids, because the tetra-haploid genome of F. japonica var. japonica can form bivalents, yet the di-haploid genome of F. sachalinensis cannot.
Ploidy levels of knotweed are important, because they can impart reproductive barriers or reduce fertility, which determine the taxa that can successfully reproduce sexually together. For example, F. japonica can produce seed after pollination by the related species Bukhara fleeceflower (Fallopia baldschuanica Regel; syn.: Polygonum baldschuanicum Regel), but this hybrid seed is infertile and rarely becomes established (Bailey et al. Reference Bailey, Bímová and Mandák2008).
Conclusion
The depth of research on knotweeds is impressive, but it would be poor practice to assume knotweeds will operate similarly in a different environment such as Minnesota or that we have sufficient knowledge to efficiently control it. Research on the genetic diversity of knotweed has been conducted elsewhere in the United States (Gaskin et al. Reference Gaskin, Schwarzländer, Grevstad, Haverhals, Bourchier and Miller2014; Grimsby and Kesseli Reference Grimsby and Kesseli2009; Grimsby et al. Reference Grimsby, Tsirelson, Gammon and Kesseli2007; Richards et al. Reference Richards, Schrey and Pigliucci2012; Wymer et al. Reference Wymer, Gardner, Steinberger and Peyton2007), but to date no such research has taken place in the Midwest or Minnesota. This is especially worrisome, given that knotweed has been reported in every midwestern state (EDDMapS 2021; https://www.eddmaps.org/) and all three taxa are listed as noxious in Minnesota (Midwest Invasive Plant Network 2018). The density of knotweed varies across the state, with the highest number of knotweed populations being reported in Duluth and the Twin Cities (EDDMapS 2021). For example, there are currently 264 confirmed reports of knotweed just in Duluth alone (EDDMapS 2021), meaning there is a knotweed population every 0.78 km2. With varied mechanisms of dispersal, including intentional human spread, it is extremely important to know which species are present in Minnesota and the primary means by which each taxa is spreading, either by sexual or asexual means, so that their spread can be better combated to inform and improve management outcomes. Each taxa responds differently to mechanical, chemical, and biological control (Bímová et al. Reference Bímová, Mandák and Pyšek2001; Grevstad et al. Reference Grevstad, Shaw, Bourchier, Sanguankeo, Cortat and Reardon2013). Thus, it is essential to know exactly which type of knotweed is going to be treated before a control measure is selected. Because it can be difficult to visually distinguish the taxa from one another, genetic testing is essential to provide accurate identification information. Measuring fertility and sexual reproduction of invasive knotweeds will foretell increasing genetic diversity and the potential for evolution of resistance to management.
Acknowledgments
This work was supported by the Minnesota Invasive Terrestrial Plants and Pests Center and the Environment and Natural Resources Trust Fund and facilitated by the Minnesota Department of Agriculture. No conflicts of interest have been declared.