Common ragweed is one of the most important weeds in the soybean-producing areas of the United States and Canada (Coble et al. Reference Coble, Williams and Ritter1981; Ontario Ministry of Agriculture, Food and Rural Affairs [OMAFRA] 2015; Shurtleff and Coble Reference Shurtleff and Coble1985; Weaver Reference Weaver2001). For example, Shurtleff and Coble (Reference Shurtleff and Coble1985) found that common ragweed at densities of 16 plants per 10 m row reduced soybean yield by up to 12% in North Carolina. Coble et al. (Reference Coble, Williams and Ritter1981) estimated soybean yield losses of 33 kg ha−1 when only a single common ragweed plant occurred per 10 m row in North Carolina. In Ontario, Canada, it has been reported that a single common ragweed plant per square meter reduces soybean yield by up to 10% and that five plants per square meter can result in up to 33% yield loss (Cowbrough et al. Reference Cowbrough, Brown and Tardif2003; OMAFRA 2009).
Nearly 50% of soybean hectares in the United States and Ontario, Canada, are maintained as no-till (Soil Conservation Council of Canada 2015; Wade et al. Reference Wade, Claassen and Wallander2015). The increase in no-till soybeans has increased the reliance on in-crop applications of glyphosate (Young Reference Young2006). The introduction of glyphosate-resistant (GR) soybean cultivars has provided growers with several advantages, including improved and more consistent weed control, decreased herbicide application costs, and reduced time and labor inputs (Beckie et al. Reference Beckie, Harker, Hall, Warwick, Légère, Sikkema, Clayton, Thomas, Leeson, Séguin-Swartz and Simard2006; Young Reference Young2006). In 2012 GR soybean comprised about 93 and 79% of soybean crop areas in the United States and Canada, respectively (Beckie et al. Reference Beckie, Sikkema, Soltani, Blackshaw and Johnson2014; Bøhn et al. Reference Bøhn, Cuhra, Traavik, Sanden, Fagan and Primicerio2014). However, weed management in GR cropping systems has been complicated by the development and spread of GR weed biotypes. Glyphosate resistant common ragweed has been confirmed in 15 U.S. states: Arkansas (2004), Missouri (2004), Kentucky (2006), North Carolina (2006), Ohio (2006), Indiana (2007), Kansas (2007), North Dakota (2007), South Dakota (2007), Pennsylvania (2008), Minnesota (2008), Alabama (2013), New Jersey (2013), Nebraska (2013) and Mississippi (2014) (Heap Reference Heap2015). In Canada, the first and only case of GR common ragweed to date was discovered in a soybean field in southern Ontario in 2012 (Heap Reference Heap2015; Van Wely et al. Reference Van Wely, Soltani, Robinson, Hooker, Lawton and Sikkema2015).
The high fecundity and complex seed germination behavior of common ragweed, resulting from primary and secondary dormancy, contributes to its success in establishing and maintaining a persistent seedbank in arable land (Baskin and Baskin Reference Baskin and Baskin1980; Bazzaz 1974; Dickerson and Sweet Reference Dickerson and Sweet1971). A single common ragweed plant can produce between 3,185 and 62,000 seeds, depending on plant size (Bazzaz Reference Bazzaz1974; Dickerson and Sweet Reference Dickerson and Sweet1971). In a soybean crop, ragweed plants emerging 1 mo after the crop produced up to 2,914 seeds per plant (Simard and Benoit Reference Simard and Benoit2012). Once in the seedbank, these seeds can remain viable for 39 yr (Baskin and Baskin Reference Baskin and Baskin1980). Therefore, seed production by GR common ragweed individuals that escape herbicide control can provide seedbank replenishment and could promote the subsequent spread of resistant alleles.
The use of a late herbicide application has been advocated as a strategy for reducing weed seed production and viability (Clay and Griffin Reference Clay and Griffin2000; Crow et al. Reference Crow, Steckel, Hayes and Mueller2015; Gauvrit and Chauvel Reference Gauvrit and Chauvel2010; Hill et al. Reference Hill, Renner, VanGessel, Bellinder and Scott2016; Jha and Norsworthy Reference Jha and Norsworthy2012; Kumar and Jha Reference Kumar and Jha2015; Nurse et al. Reference Nurse, Darbyshire and Simard2015; Steadman et al. Reference Steadman, Eaton, Plummer, Ferris and Powles2006; Taylor and Oliver Reference Taylor and Oliver1997; Walker and Oliver Reference Walker and Oliver2008). Jha and Norsworthy (Reference Jha and Norsworthy2012) demonstrated that application of glufosinate, 2,4-D, or dicamba at the first appearance of an inflorescence reduced seed production and viability of GR Palmer amaranth (Amaranthus palmeri S. Wats.). However, up to now, late application options for the control of GR biotypes in crop have been limited because of crop injury risks associated with applying an efficacious herbicide at growth stages when the crop is less tolerant to these herbicides. The development and release of new Group 4–tolerant crop hybrids and varieties offers a potential mechanism to address this issue. Monsanto’s dicamba-tolerant technology (i.e., XtendTM) and Dow Agroscience’s 2,4-D–tolerant technology (i.e., EnlistTM) are anticipated to be released into the U.S. and Canadian markets shortly. While Group 4, phenoxy herbicides have shown good efficacy for controlling common ragweed at early growth stages (OMAFRA 2015; Payne et al. Reference Payne, Sleugh and Bradley2010), no information is currently available on how effective these herbicides or a tank mix of these herbicides with glyphosate might be at reducing the number and viability of GR common ragweed seeds when applied to ragweed plants at or near flowering. Therefore, the objective of the following study was to evaluate the effect of late herbicide application on growth, seed production, and seed viability of GR common ragweed.
Materials and Methods
Growth chamber and germination cabinet experiments were conducted and repeated at the Harrow Research and Development Centre, Agriculture and Agri-Food Canada, Harrow, Ontario, Canada. A randomized complete block factorial design with three replicates and two experimental runs was used to evaluate the efficacy of late herbicide application on the growth, fecundity, and seed viability of GR common ragweed. Tested factors included herbicide treatment and growth stage of common ragweed. The six herbicide treatments included: (1) a water control, (2) glyphosate (900 g ae ha−1), (3) 2,4-D (733 g ae ha−1), (4) dicamba (384 g ae ha−1), (5) 2,4-D plus glyphosate (733 g ae ha−1+900 g ae ha−1), and (6) dicamba plus glyphosate (384 g ae ha−1+900 g ae ha−1), each of which was applied to GR common ragweed at two stages based on the extended BBCH scale for dicotyledonous weed species (Hess et al. Reference Hess, Barralis, Bleiholder, Buhr, Eggers, Hack and Stauss1997). Rates of glyphosate, 2,4-D, and dicamba were selected to reflect the ratio and maximum allowed rate for their respective herbicide-tolerant cropping systems (Anonymous 2014, 2016), while maintaining the factorial structure of the experimental design.
Herbicides were applied at (1) male flower bud appearance (BBCH 51) and (2) early female flowering (BBCH 61 to 63, 10 to 30% of female flowers open; Figure 1). On average, GR common ragweed plants reached these growth stages at 28 and 43 d after planting (DAP), respectively. For context, soybean maturity groups representing the range of those grown across the United States and Canada (i.e., MG 00 to VIII) reach the R1 stage of development between 28 and 50 DAP (Boote Reference Boote1981; Van Acker et al. Reference Van Acker, Swanton and Weise1993; Zhang et al. Reference Zhang, Zhang, Watson and Kyei-Boahen2004). Based on the labels currently available for XtendTM and EnlistTM technologies, these glyphosate–phenoxy tank mixes should be applied no later than the R1 and R2 stages of soybean development, respectively (Anonymous 2015, 2016). Thus, it can be conservatively stated that the herbicide application timings evaluated in the current study approximate the latest labeled timing for these new technologies and a late, off-label application timing (i.e., BBCH 51 and 61 to 63, respectively).

Figure 1 Common ragweed stage of development at the time of herbicide application: (a) BBCH 51 (male flower buds appearance) and (b) BBCH 61 to 63 (early female flowering: 10–30% of female flowers open). The extended BBCH (Biologische Bundesanstalt, Bundessortenamt and Chemical industry) scale for dicotyledonous weed species was used (Hess et al. Reference Hess, Barralis, Bleiholder, Buhr, Eggers, Hack and Stauss1997).
Seed Collection and Preparation
Common ragweed involucral achenes (hereafter referred to as “seeds”) of GR biotype were collected from a farm in Essex County, Ontario, Canada (42.28°N, 82.84°W; Van Wely et al. Reference Van Wely, Soltani, Robinson, Hooker, Lawton and Sikkema2015) in September 2013. The seeds were stored dry in a controlled-environment room (5 C and 50% relative humidity [RH]) until the commencement of the experiment.
Common ragweed seed possess Type 2 non–deep physiological dormancy (i.e., dormancy due to exogenous characteristics caused by a physiological mechanism of the embryo that inhibits the emergence of the radicle; Baskin and Baskin Reference Baskin and Baskin2004, Reference Baskin and Baskin2014; Finch-Savage and Leubner-Metzger Reference Finch-Savage and Leubner-Metzger2006). Therefore, seeds were initially cold stratified to relieve dormancy and facilitate germination. In preparation for stratification, 200 seeds were placed in a petri dish lined with blue blotter paper (i.e., steel-blue germination blotters, Anchor Paper, St. Paul, MN) and moistened with 10 ml of reverse-osmosis (RO) water. Petri dishes were then placed within a light-tight aluminum canister and refrigerated at 4 C for 6 wk. Following stratification, seeds were transferred to new petri dishes (25 seeds dish−1) containing blue blotter paper moistened with 10 ml of RO water. These dishes were then placed into a seed germination cabinet (model G1000, Conviron, Controlled Environment, Canada, Winnipeg, Manitoba, Canada) with a photoperiod of 14 h, 60% RH, and an alternating temperature of 25/15 C (day/night).
Plant Propagation
Germinated seeds of GR common ragweed were planted one to a cell in 24-cell plug flats (6.4 cm cell top diameter and 6 cm cell depth) filled with high-porosity potting media (BM6, Berger, Saint Modest, Québec, Canada). Trays were placed into a growth chamber (model PGC20, Conviron, Controlled Environment, Winnipeg, Manitoba, Canada) with a photoperiod of 14 h, 80% RH, and an alternating thermoperiod of 25/20C (day/night). The seedlings were watered daily and fertilized weekly with a 1 g L−1 nutrient solution (Plant-Prod ® 20-20-20 N-P-K Classic, Master Plant-Prod, Brampton, Ontario, Canada).
Once seedlings reached the 3- to 4-leaf stage (BBCH 13), two plants were transplanted into each of 108 plastic pots (16.5 cm diameter, 1.4 L) filled with BM6 potting media. The pots were randomly assigned among three growth chambers (i.e., 36 pots in each replicate chamber) and maintained under the same conditions used for seedling growth. Although only 24 pots per replicate were required to conduct the experiment (see “Herbicide Application” section), 36 pots were initially created to ensure uniformity among the 24 pots selected. Two plants in each pot were thinned to one plant per pot at the 6- to 8-leaf stage. The pots were randomly rearranged within the chamber weekly to minimize position effects. The plants were watered daily except for the day of fertilization (once a week) with 2 g L−1 Plant-Prod® 20-20-20 N-P-K Classic.
Herbicide Application
Twenty-four plants of similar size within each growth chamber were selected and then randomly divided into two subgroups (i.e., BBCH 51 and BBCH 61 to 63) containing 12 plants each. The two plants in each cabinet receiving the same treatment combination (i.e., herbicide by growth stage) were considered subsamples. Once they reached the corresponding stage of development (Figure 1), the plants were randomly assigned to one of six herbicide treatments. Herbicides were applied to the plants using an automated spray chamber equipped with 8002E even-spray nozzles set to apply at a rate of 333.3 L ha−1 pressurized by CO2. Following herbicide application, plants were placed on separate benches in a greenhouse compartment to minimize the opportunity for herbicide transfer between treatments. The environmental conditions in the greenhouse were similar to those established in the growth chambers (described earlier). After 24 h, the plants were returned to their assigned growth chambers. It is important to note that plants from all treatment combinations (i.e., herbicide by growth stage) were present in all three replicate growth chambers, and we did not attempt to control for pollen transfer among treatments. As a result, pollen from the untreated control could have, in theory, pollinated plants receiving herbicide applications, thus overriding any negative effects of pollen limitation on the fecundity of these treatments. Because common ragweed is a highly outcrossing species (Friedman and Barrett Reference Friedman and Barrett2008), we chose to avoid biasing our measure of fecundity by restricting pollination to selfing. Moreover, previous research into the effects of herbicide application on pollination biology has demonstrated that glyphosate, 2,4-D, and dicamba have limited or a transitory influence on pollen production and viability but notable, detrimental impacts on the development of female flower organs (Amer and Ali Reference Amer and Ali1974; Pepper et al. Reference Pepper, Weibel and Santelmann1970; Pline et al. Reference Pline, Edmisten, Wilcut, Wells and Thomas2003). During the course of our experiment, we observed that common ragweed plants had a long duration of pollen shed (approximately 3 to 4 wk), and they continued producing male flowers on secondary branches even after herbicide application. We therefore conclude that the supply of pollen was not likely a confounding effect on ragweed fecundity; rather, the effect of herbicides on female flower development and receptivity was a more probable cause for the observed reductions in seed production.
Growth and Reproductive Parameter Measurement
When seeds on test plants began to dry and ripen, causing a shift in color to brown, a fine-mesh (0.1 mm) polyester bag was wrapped around each plant and tied at the base to ensure all seeds produced were collected. Each plant was cut at the soil surface approximately 90 DAP and placed into an individual paper bag. The samples were dried at 40 C for 1 wk. Aboveground dry biomass was determined by subtracting seed weight from total aboveground weight. Seeds were separated manually from the rest of the plant, and chaff and debris were removed using a seed blower (Model 757 South Dakota Seed Blower, Seedburo Equipment, Des Plaines, IL). Seeds were then counted by an electronic seed counter (Model ESC-1, Agriculex, Guelph, Ontario, Canada) and a 100-seed weight was estimated. Seeds were placed into paper envelopes and stored in the dark at 5 C and 50% RH until seed viability tests were initiated.
Seeds collected from the two plants in each cabinet receiving the same treatment combination (i.e., herbicide type by growth stage) were pooled. Then, 120 seeds per replicate of each treatment combination were surface sterilized with 5.5% sodium hypochlorite solution for 5 min and subsequently rinsed with running RO water for 10 min. Seeds were cold stratified for 6 wk (as described earlier in “Seed Collection and Preparation”). Following the stratification period, 120 seeds were assigned to one of four petri dishes lined with blue blotter paper moistened with 10 ml of RO water. Petri dishes were randomly assigned, by replicate, to a separate shelf of the germination cabinet under the same germination conditions as used previously (see “Seed Collection and Preparation”). The number of germinated seeds in each dish was recorded daily for a 21 d period, with all germinated seeds removed daily. Germination was defined by the presence of a radicle that was at least 2 mm in length. Seeds that had not germinated after the 21 d period were subjected to an initial pressure test with forceps, and those that did not resist the slight pressure were considered nonviable. The viability of all remaining seeds was determined using the tetrazolium chloride test (Peters Reference Peters2000). The germination and viability tests were repeated twice.
Statistical Analysis
All analyses were performed using R software (v. 3.1.2; R Core Team 2015). To analyze the effect of herbicide treatment and growth stage on biomass, seed number, 100-seed weight, and seed viability, linear mixed-effects models were fit using the lmer function of the lme4 package (Bates et al. Reference Bates, Maechler, Bolker and Walker2015). The fixed effects were herbicide treatment and growth stage. Intercepts for block and trial run were considered random effects. The two plants in each cabinet receiving the same treatment combination (i.e., herbicide by growth stage) were considered subsamples and, as such, were averaged for statistical analyses of biomass, seed number, and 100-seed weight. Percentages of control in biomass, seed number, and 100-seed weight were calculated and were analyzed. These data were transformed prior to the analysis as follows: aboveground biomass and seed weight (log10 transformation); seed number (square-root transformation); and percentage of control and seed viability (arcsine square-root transformation). Back-transformed means are presented in tables and figures based on the interpretation from the transformed data. P-values were obtained by likelihood ratio tests of the full model with the effect in question against the model without the effect in question. Mean separations were conducted with the glht function of the multcomp package (Hothorn et al. Reference Hothorn, Bretz and Westfall2008).
Results and Discussion
The total aboveground biomass of GR common ragweed at physiological maturity was influenced by the main effects of herbicide treatment and growth stage but not their interaction (Table 1). When herbicide treatments were applied at BBCH 51, biomass accumulation at maturity was 22% lower than when herbicides were applied at BBCH 61 to 63 (Table 2A). The application of 2,4-D alone or in a tank mix with glyphosate resulted in a statistically significant reduction in biomass relative to the control (Table 2A). As expected, the application of glyphosate to the GR biotype did not result in a reduction in total aboveground biomass at maturity. Although the exact mechanism conferring resistance to glyphosate in the tested biotype is not known, the resistance mechanism likely protects biomass production at flowering stages.
Table 1 Effect of the herbicide treatment, growth stage, or their interaction on (A) original data and (B) data expressed as a percent of control for dry biomass, 100-seed weight, seed production, and seed viability of glyphosate-resistant common ragweed.

a Significance of χ2 values was tested using P-values obtained by likelihood ratio tests of the full model with the effect in question against the model without the effect in question.
*p<0.05.
**p<0.01.
***p<0.001.
Table 2 Means and percentage control of glyphosate-resistant common ragweed (A) dry biomass and (B) 100-seed weight in response to the herbicide treatments applied at the appearance of male flower buds (BBCH 51) or at the early female flowering (BBCH 61 to 63).Footnote a
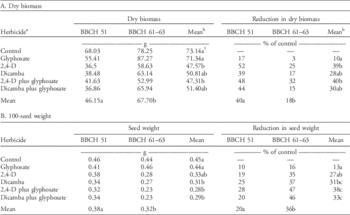
a The herbicide treatments include: reverse-osmosis water control; glyphosate (900 g ae ha−1); 2,4-D (733 g ae ha−1); dicamba (384 g ae ha−1); 2,4-D plus glyphosate (733 g ae ha−1 plus 900 g ae ha−1); and dicamba plus glyphosate (384 g ae ha−1 plus 900 g ae ha−1).
b Means for main effects of herbicide and application timing within a data set sharing the same letters are not significantly different (Tukey’s honest significant difference, α=0.05).
The main effects of herbicide treatment and growth stage influenced the 100-seed weight of GR common ragweed, but the interaction of these factors was not significant (Table 1). When herbicides were applied at BBCH 51, the 100-seed weight of seeds produced at maturity was 16% greater than when herbicides were applied at BBCH 61 to 63 (Table 2B). When glyphosate or 2,4-D was applied alone, there was no significant reduction in the 100-seed weight relative to the control (Table 2B). Although dicamba applied alone did result in a significant reduction in the 100-seed weight, the biological magnitude of this reduction was similar to that observed for 2,4-D (Table 2B). The largest reduction in 100-seed weight was observed when 2,4-D or dicamba were applied in a tank mix with glyphosate, with an average decline of 35% relative to the control.
Seed production in GR common ragweed was influenced by herbicide treatment, growth stage, and their interaction (Table 1). Compared with the control, applications of 2,4-D, dicamba, or dicamba plus glyphosate at BBCH 51 provided greater than 80% reduction in seed production (Figure 2). At BBCH 61 to 63, all herbicide treatments, including glyphosate alone, reduced seed production relative to the control; however, this reduction was notably lower on average than that observed at BBCH 51 (i.e., −70% at BBCH 51 vs. −45% at BBCH 61 to 63). Overall, seed production following the application of glyphosate–phenoxy tank mixes was similar to that observed following the application of phenoxy herbicides alone, regardless of growth stage (Figure 2).

Figure 2 Glyphosate-resistant common ragweed seed production relative to the control in response to herbicide application at BBCH 51 (male flower bud appearance) or at BBCH 61 to 63 (early female flowering). The extended BBCH (Biologische Bundesanstalt, Bundessortenamt and Chemical industry) scale for dicotyledonous weed species was used (Hess et al. Reference Hess, Barralis, Bleiholder, Buhr, Eggers, Hack and Stauss1997). The control plants produced 4719±656 and 4005±1010 seeds when treated with water at BBCH 51 and BBCH 61 to 63, respectively. Bars sharing the same letter indicate no significant difference (Tukey’s honest significant difference, α=0.05).
The viability of the seeds produced by GR common ragweed was influenced by herbicide treatment, growth stage, and their interaction (Table 1). At BBCH 51, seed viability was similar among the herbicide treatments, with the exception of dicamba applied alone (Figure 3). In contrast, at BBCH 61 to 63, all herbicide treatments reduced seed viability relative to the control; however, treatments containing a Group 4 herbicide had significantly lower viability than when glyphosate was applied alone (Figure 3). As was the case with seed production, reductions in seed viability were similar when Group 4 herbicides were applied alone or in a tank mix with glyphosate, irrespective of growth stage at application.

Figure 3 Seed viability of glyphosate-resistant common ragweed in response to herbicide application at BBCH 51 (male flower bud appearance) or at BBCH 61 to 63 (early female flowering). The extended BBCH (Biologische Bundesanstalt, Bundessortenamt and Chemical industry) scale for dicotyledonous weed species was used (Hess et al. Reference Hess, Barralis, Bleiholder, Buhr, Eggers, Hack and Stauss1997). Bars sharing the same letter indicate no significant difference (Tukey’s honest significant difference, α=0.05).
Reducing the seed production of weed escapes is a central tenet of any proactive seedbank management strategy (Buhler et al. Reference Buhler, Hartzler and Forcella1997; Davis Reference Davis2006; Walsh et al. Reference Walsh, Newman and Powles2013). Targeting weed seed production becomes even more important when the weed escapes are known to occur as a result of herbicide resistance. In the case of GR common ragweed, seed production by escapes has the potential to create a large, long-lived seedbank that may contribute to the persistence and spread of this resistant biotype. Results of the present study demonstrate that the application of Group 4 herbicides has the potential to significantly reduce the seed production of GR common ragweed when applied at the appearance of male flower buds (Fig 1a). This result is in agreement with that of Jha and Norsworthy (Reference Jha and Norsworthy2012), who observed that 2,4-D (1,060 gha−1) or dicamba (280 g ha−1), each applied at the early inflorescence emergence stage, reduced seed production of GR Palmer amaranth by 75 to 95% and 84 to 95%, respectively. Similarly, Fawcett and Slife (Reference Fawcett and Slife1978) and Taylor and Oliver (Reference Taylor and Oliver1997) reported that the application of 2,4-D or dicamba near flowering or bud formation effectively reduced seed production in common lambsquarters (Chenopodiumn album L.) and sicklepod [Senna obtusifolia (L.) H. S. Irwin & Barneby], respectively. It is important to note, however, that the effectiveness of 2,4-D and dicamba at reducing seed production of GR common ragweed was significantly reduced when these herbicides were applied 15 d later, when female flowers start to be receptive (Fig 1b). In fact, at this stage of development, there was no significant difference among 2,4-D, dicamba, and glyphosate in terms of their ability to influence the seed production of our GR common ragweed biotype.
In general, seed viability and seed weight were reduced following the application of 2,4-D or dicamba during the reproductive stages of common ragweed development. Unsurprisingly, reductions in these traits were largest when treatments containing 2,4-D or dicamba were applied during the later stages of flowering (i.e., BBCH 61 to 63), when fertilized embryos may have been less likely to abort. Results from previous studies offer variable accounts of success in reducing weed seed viability following the application of 2,4-D or dicamba at developmental stages bracketing flowering (Fawcett and Slife Reference Fawcett and Slife1978; Jha and Norsworthy Reference Jha and Norsworthy2012; Kumar and Jha Reference Kumar and Jha2015; McCarty and Hatting Reference McCarty and Hatting1975; Taylor and Oliver Reference Taylor and Oliver1997). For example, Taylor and Oliver (Reference Taylor and Oliver1997) reported that dicamba (1,100 and 2,200 g ha−1) applied at the early bud stage or the early flowering stage did not affect seed viability of sicklepod, whereas Jha and Norsworthy (Reference Jha and Norsworthy2012) observed that dicamba (280 g ha−1) applied at the first sign of inflorescence decreased seed viability of GR Palmer amaranth by 45%. Much of this variation among studies can likely be attributed to small differences in the developmental stage at which the herbicide application was made, as the progression from vegetative to reproductive stages can sometime be quite rapid. In our experiment, for instance, the progression from BBCH 51 to BBCH 61 to 63 required on average 15 d, however, individual common ragweed plants displayed considerable plant-to-plant variation in the time required to make this transition.
The developmental stages bracketing flowering are sensitive to biotic and abiotic stressors (Lauxmann et al. Reference Lauxmann, Annunziata, Brunoud, Wahl, Koczut, Burgos, Olas, Maximova, Abel, Schlereth, Soja, Bläsing, Lunn, Vernoux and Stitt2015; Morrison and Stewart Reference Morrison and Stewart2002; Stephenson Reference Stephenson1981; Tollenaar and Daynard Reference Tollenaar and Daynard1978). Stressors encountered during these stages often result in flower or fruit abortion and in some cases reductions in fruit mass, depending on the severity or duration of the stress. While the application of herbicides during the stages bracketing flowering could elicit such responses simply via their activity as stressors of plant growth, the herbicides from Groups 4 and 9 used in the present study also translocate through the plant and accumulate in metabolic sinks, such as reproductive tissues (Duke et al. Reference Duke, Rimando, Pace, Reddy and Smeda2003; Senseman 2007; Sprankle et al. Reference Sprankle, Meggitt and Penner1975; Wyrill and Burnside Reference Wyrill and Burnside1976). In particular, previous research has demonstrated that the application of glyphosate to susceptible species or biotypes during the stages bracketing flowering increases flower and fruit abortion and reduces the quality and viability of seed that may form (Clay and Griffin Reference Clay and Griffin2000; Gougler and Geiger Reference Gougler and Geiger1981; Nurse et al. Reference Nurse, Darbyshire and Simard2015; Steadman et al. Reference Steadman, Eaton, Plummer, Ferris and Powles2006; Taylor and Oliver Reference Taylor and Oliver1997; Walker and Oliver Reference Walker and Oliver2008). In a susceptible common ragweed biotype, for example, glyphosate (540 g ae ha−1) applied at BBCH 53 to 55 (i.e., the bud appearance stage) and BBCH 61 (flowering) completely inhibited seed production (Gauvrit and Chauvel Reference Gauvrit and Chauvel2010). In our study, glyphosate applied when male flower buds were present reduced neither seed number nor viability, whereas at the onset of female flowering, seed production was reduced by 45% and viability declined by 14%, resulting in a net 48% reduction in viable seed production relative to the control. As was noted previously, all of the herbicides applied at BBCH 61 to 63, alone or in a tank mix, resulted in statistically similar reductions in seed production, indicating that this effect can most likely be attributed to the sensitivity of this growth stage to stressors rather than to the herbicidal activity of a specific chemical compound. However, while seed viability did decline following glyphosate application at BBCH 61 to 63, the reduction in viability was significantly less than when 2,4-D or dicamba was applied at the same growth stage (i.e., 14 vs. 47 and 60%, respectively). The efficacy of 2,4-D or dicamba at reducing common ragweed seed production and viability when applied at the growth stages examined in this study suggests that the herbicides were translocated to meristematic tissue, as would be expected in a Group 4–susceptible biotype. Conversely, the lack of reduction in seed production at BBCH 51 and the slight decline in seed viability at BBCH 61 to 63 following glyphosate application suggest that altered absorption, translocation, or metabolism of glyphosate might restrict the movement of the herbicide into reproductive organs in this GR biotype. Previous studies have suggested both target-site and non–target site mechanisms for glyphosate resistance in common ragweed; however, a definitive consensus on the mechanism of resistance has yet to be reached (Brewer and Oliver Reference Brewer and Oliver2009; Gaines et al. 2016; Parrish Reference Parrish2015).
Results from the current study add to our knowledge of the efficacy of 2,4-D or dicamba for the control of GR weed escapes. For GR common ragweed, 2,4-D or dicamba applied alone or in a tank mix with glyphosate could be effectively used to reduce seed production when applied at the onset of the flowering period. Providing that such late applications can be made with an acceptable level of crop safety, they may be an effective option for limiting seedbank replenishment, reducing population establishment, and preventing further spread of the resistant alleles. The recent development of crop hybrids and varieties with tolerance to Group 4 herbicides could facilitate grower adoption of targeted weed seed control strategies for GR biotypes. It should be emphasized, however, that in spite of the potential of a Group 4–tolerant cropping system for targeted control of GR escapes, the timing of the herbicide application relative to the developmental stage of the weed is of critical importance in determining the efficacy of the strategy with respect to seed production or viability.
Acknowledgments
The authors are grateful for the funding provided in support of this project by Agriculture and Agri-Food Canada (project #J-00919). The authors would also like to acknowledge Dana Gagnier and Janet Blackburn for technical assistance and Peter Sikkema for reporting the original GR population.