Introduction
Ryegrass (Lolium spp.) is a troublesome weed in wheat (Triticum aestivum L.) production worldwide, and it is also a valuable cultivated forage, turf, and cover crop species. Nine species of Lolium are known to occur globally, and of these, perennial ryegrass (Lolium perenne L.) and Italian or annual ryegrass [Lolium perenne L. ssp. multiflorum (Lam.) Husnot] are the two most commonly occurring species globally as well as in the United States (U.S.) (Rosell Reference Rosell1967; Scholz et al. Reference Scholz, Stierstorfer and Gaisberg2000). They were introduced to the United States as a forage crop from Europe and used in Virginia as early as 1782 (Sullivan Reference Sullivan1992). They can be found growing as a natural vegetation along roadsides and in crop fields, abandoned lands, orchards, pastures, and vineyards (Panozzo et al. Reference Panozzo, Collavo and Sattin2020; Wu et al. Reference Wu, Zhang, Zhang, Lang and Sun2012).
Lolium grows in a broad range of soil textures, adapts well to poorly drained soils (Evers Reference Evers1995; Venuto et al. Reference Venuto, Redfearn, Pitman and Alison2003), and shows drought and cold tolerance (Franca et al. Reference Franca, Loi and Davies1998). It shows high adaptive potential and is a persistent species. Although these traits are favorable in permanent pasture and grazing lands (Nair Reference Nair2004), the weedy Lolium biotypes exhibit rapid growth (Bararpour et al. Reference Bararpour, Norsworthy, Burgos, Korres and Gbur2017), high fecundity (Beckie Reference Beckie2006), profuse seed shattering (Salas Reference Salas2012), and high seed dormancy (Steadman et al. Reference Steadman, Ellery, Chapman, Moore and Turner2004), which facilitate their persistence and menace in crop fields (Peeper and Wiese Reference Peeper, Wiese and Donald1990). Lolium emerges in the fall and continues to grow through summer and has currently become a major weed in summer forage production in many areas (Funderburg Reference Funderburg2018).
Among the Lolium species, L. perenne ssp. multiflorum in particular is a troublesome weed in major wheat-producing regions in the United States (Appleby et al. Reference Appleby, Olson and Colbert1976; Appleby and Brewster Reference Appleby and Brewster1992; Trusler et al. Reference Trusler, Peeper and Stone2007; Tucker et al. Reference Tucker, Morgan, Senseman, Miller and Baumann2006; Webster and Nichols Reference Webster and Nichols2012), including parts of Texas (Neely et al. Reference Neely, Bauman and McGinty2016). Reports indicate a 61% wheat yield reduction by 93 L. perenne ssp. multiflorum plants m−2 in Oregon (Appleby et al. Reference Appleby, Olson and Colbert1976; Appleby and Brewster Reference Appleby and Brewster1992) and 40% reduction by 40 plants m−2 in Texas (Stone et al. Reference Stone, Cralle, Chandler, Miller and Bovey1999). Stone et al. (Reference Stone, Cralle, Chandler, Bovey and Carson1998) found that belowground root interaction between L. perenne ssp. multiflorum and wheat reduced wheat height, leaf number, tillering, leaf area, and dry weights of leaves, stems, and roots. With heavy reliance on herbicides for controlling Lolium, L. perenne ssp. multiflorum is currently the most resistance-prone weed in U.S. wheat, with resistance to eight different herbicide mechanisms of action (Heap Reference Heap2020).
High diversity in weeds is known to favor their invasiveness and potential to adapt to various growing conditions and management regimes, including herbicide applications (reviewed in Dekker Reference Dekker2011; Akey et al. Reference Akey, Jurik and Dekker1990; Baker Reference Baker1974; Fried et al. Reference Fried, Chauvel, Munoz and Reboud2019; Jha et al. Reference Jha, Norsworthy, Bridges and Riley2008). For example, diversity for temporal and spatial seed dispersal and staggered emergence from the soil seedbank are mechanisms that help weeds adapt to local management practices and prevailing environments (Mahaut et al. Reference Mahaut, Fried and Gaba2018). Adaptive traits such as rapid vegetative growth, high levels of biomass production, fecundity, seed shattering, and dormancy, among others, have been reported to be associated with invasiveness and herbicide resistance (Beckie Reference Beckie2006; Gressel and Segel Reference Gressel, Segel, LeBaron and Gressel1982; Salas Reference Salas2012; Watkinson and White Reference Watkinson and White1985). Vila-Aiub et al. (Reference Vila-Aiub, Gundel and Preston2015) noted that accumulation of functional and adaptive traits that enhance plant fitness to a specific or a wide range of environments may favor survival of weeds to herbicides. In some cases, in addition to interpopulation diversity, intrapopulation diversity can be very high (e.g., Bangert et al. Reference Bangert, Lonsdorf, Wimp, Shuster, Fischer, Schweitzer, Allan, Bailey and Whitham2006; Shuster et al. Reference Shuster, Lonsdorf, Wimp, Bailey and Whitham2006), which allows for local adaptation (Dekker Reference Dekker2011).
A robust evaluation of morphological trait diversity (both intra- and interspecific) that contributes to weed adaptation in a particular geographic region has great ecological and evolutionary implications, as high diversity leads to invasion, succession, acclimatization, and may eventually lead to new speciation (Bennett et al. Reference Bennett, Riibak, Kook, Reier, Tamme, Bueno and Pärtel2016; Heslop-Harrison Reference Heslop-Harrison2010). In Texas, the Blackland Prairies (Figure 1) are an important winter wheat production region, with a planted area of 1.8 million ha and a production of 1.9 billion kg in 2019 (USDA-NASS 2019). A recent survey conducted by Singh et al. (Reference Singh, Maity, Abugho, Swart and Bagavathiannan2020) confirmed the widespread occurrence of L. perenne ssp. multiflorum with resistance to commonly used herbicides in the Texas Blackland Prairies. However, little is known about the diversity for adaptive traits in Lolium in the Texas Blackland Prairies. The objective of this study was to determine the extent of diversity for different morphological traits and identify the correct species based on taxonomic characteristics in Lolium populations collected in the Texas Blackland Prairies.

Figure 1. Geocoordinates of Lolium collected from wheat fields in 2017 indicating (A) location within Texas, (B) population IDs, and (C) names of counties.
Materials and Methods
Field Survey and Plant Material
Field surveys were conducted during June 2017 (coinciding with wheat crop maturity window and before crop harvest) to collect Lolium seed samples from wheat production fields and field edges across the Texas Blackland Prairies (Figure 1). At each survey site, spikes from 20 to 25 plants were harvested randomly and pooled into a single sample. In total, 68 Lolium populations were collected during the survey, of which 56 were used in this study based on seed availability. The samples were dried, threshed, cleaned, and stored at room temperature (25 ± 2 C) under white fluorescent light before characterization. More specifics of the field survey methodology and sample collection can be found in Singh et al. (Reference Singh, Maity, Abugho, Swart and Bagavathiannan2020).
Characterization of Morphological Traits
Plant morphological traits were evaluated under controlled greenhouse conditions in a randomized complete block design, with two independent experimental runs. During each run, 50 seeds from each of the 56 Lolium populations were placed on top of two layers of filter paper (Whatman No. 1, Sigma-Aldrich, Inc., St. Louis, MO, USA) in a 9-cm-diameter petri dish, supplied with sufficient moisture throughout the germination period (21 d). The petri dishes were incubated at 25 C constant temperature, 60% relative humidity, and 12-h photoperiod. When the emerged seedlings were 21-d old (8- to 10-cm height), 15 randomly selected seedlings from each population were transplanted into individual pots (14-cm diameter and 12-cm height) filled with a potting soil mixture (LC1 Potting Mix, Sun Gro® Horticulture, Agawam, MA, USA). The pots were transferred to the Norman Borlaug Center for Southern Crop Improvement Greenhouse Research Facility at Texas A&M University and were maintained at 26/22 C day/night temperature regime and a 10-h photoperiod.
On each of the 15 randomly selected plants within each population, observations were carried out for the following 16 morphological traits: plant height (before flowering: stage 10 on Feekes scale; and at plant maturity: stage 11.3 to 11.4 on Feekes scale), regrowth rate, growth habit, basal node color, leaf color and width, plant texture, shoot dry biomass, tiller count per plant, spike count, spike length, seed count per spikelet, seed count per spike, total seed count per plant, and seed shattering. For measuring the regrowth, 5 plants in each population were trimmed at weekly intervals (at 15-cm height from the base) starting at about 6 wk after planting for 4 consecutive weeks (stages 4 to 7 on Feekes scale). As trimming might have a significant effect on other morphological traits, these 5 plants were excluded from analysis of the other traits.
Lolium shows significant genotypic variation for shoot elongation between jointing and heading (Ullmann et al. Reference Ullmann, Herrmann, Hasler, Cai and Taube2016) and also shows significant axillary growth during and after flowering in apical meristem (Jensen et al. Reference Jensen, Salchert and Nielsen2001). To capture potential differences for this response, plant height was measured both before flowering (before or at booting stage; stage 10 on Feekes scale) and again at plant maturity (stages 11.3 to 11.4 on Feekes scale). For measuring growth habit, the angle of the stem/main tillers with horizontal axis was measured and scored on a scale of 1 to 5. Methodology followed for the assessment of different traits is described in Table 1, which was adopted with slight modifications from the UPOV directory Guidelines for the Conduct of Tests for Distinctness, Homogeneity and Stability, Ryegrass (Lolium spp.) (UPOV 1990), and the National Plant Germplasm System of plant expression of the U.S. Department of Agriculture–Agricultural Research Service (USDA-ARS 2005), as described by Özköse and Tamkoç (Reference Özköse and Tamkoç2014).
Table 1. Methodology for assessing morphological traits across the Lolium perenne ssp. multiflorum populations collected in the Texas Blackland Prairies.

a The methodologies used to assess the morphological traits are adopted from Rosell (Reference Rosell1967), UPOV (1990), and Özköse and Tamkoç (Reference Özköse and Tamkoç2014), with slight modifications aligned with the location, growth habits of the populations, and available resources for the current study.
Comparison with Reference Samples for Species Identification
The Lolium populations used in the study exhibited high levels of diversity, and it was unclear whether the populations represented different Lolium species. To facilitate accurate species identification and differentiation, known reference samples of L. perenne ssp. multiflorum, L. perenne L., rigid ryegrass (Lolium rigidum Gaudin), and Darnel ryegrass (Lolium temulentum L.) were obtained from the Germplasm Resources Information Network (GRIN) of the USDA-ARS (USDA-ARS Plant Germplasm Introduction and Testing Research, Pullman, WA, https://npgsweb.ars-grin.gov). These reference samples were grown alongside the Texas populations in the greenhouse during the second experimental run and were taxonomically compared (Figures 2 and 3).

Figure 2. Seed morphological comparison among Lolium species obtained from the USDA-GRIN collections: (A) Lolium rigidum, (B) Lolium temulentum, (C) Lolium perenne ssp. multiflorum, and (D) Lolium perenne.
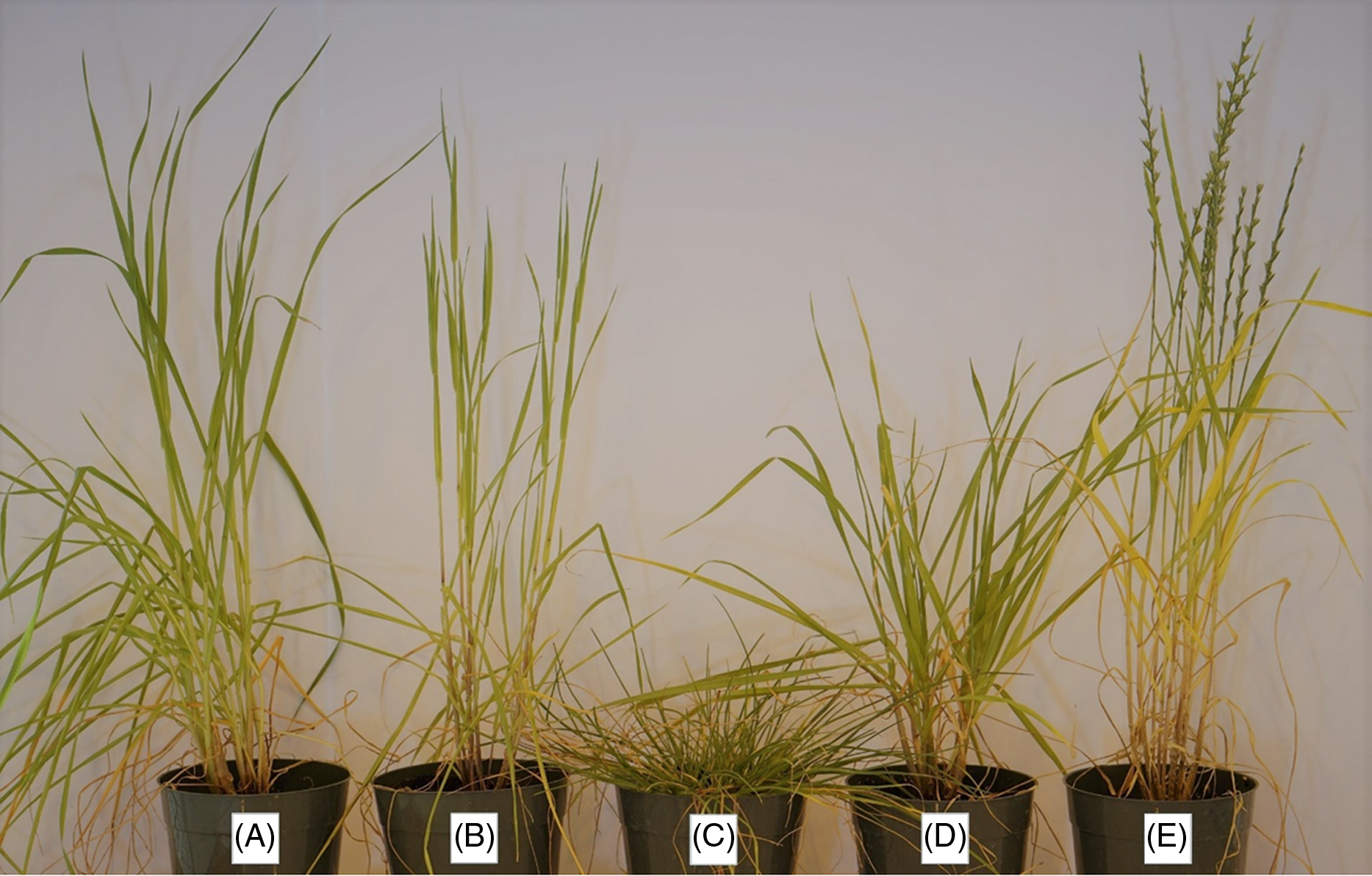
Figure 3. Positive identification of Lolium perenne ssp. multiflorum (A) in populations surveyed from the Texas Blackland Prairies, based on morphological comparison with different known Lolium species obtained from the USDA-GRIN collections (B–E): (B) Lolium rigidum, (C) Lolium perenne, (D) L. perenne ssp. multiflorum, and (E) Lolium temulentum.
Data Analysis
Plant morphological trait data were analyzed using JMP PRO v. 14 (SAS Institute, Cary, NC, USA). Interpopulation diversity for each trait is presented as range, mean, and standard error of the means of the populations. This illustrates the variability in population mean values for the different traits evaluated. For detecting intrapopulation variation for a given trait within a specific population, the difference between the highest and lowest value for the trait among the 20 individual plants (10 plants in each of the two runs) investigated within the population was calculated and presented as a frequency histogram. For determining regrowth rate, a total of 10 plants (5 plants per run) were used.
Populations were subjected to cluster analysis based on the 16 morphological traits measured. Hierarchical cluster analysis was performed based on Ward’s minimum variance method in JMP PRO v. 14, and cluster summaries are presented to show the grouping of populations. This method calculates Euclidean distance and creates cluster groupings by minimizing within-group ANOVA sum of squares. A principal component analysis was conducted using JMP to determine the association among the populations and the contribution of the different traits toward the total variability. Further, a Pearson correlation analysis was conducted to ascertain association between the morphological traits and herbicide resistance status. For this purpose, secondary data (from Singh et al. Reference Singh, Maity, Abugho, Swart and Bagavathiannan2020) on herbicide-resistance profiles of the test populations for two acetyl coenzyme-A carboxylase (ACCase)-inhibiting herbicides (diclofop-methyl, Hoelon®; Bayer Crop Science, Research Triangle Park, NC, USA and pinoxaden, Axial XL®; Syngenta Crop Protection, Greensboro, NC, USA) and one acetolactate synthase–inhibiting herbicide (mesosulfuron-methyl, Osprey®; Bayer Crop Science) were used.
Results and Discussion
Inter- and Intrapopulation Diversity
Morphological Traits
There were high levels of inter- (Table 2) as well as intrapopulation diversity (Figure 4) for all the morphological traits investigated in the study. The differences for these traits were highly significant (P < 0.001) among the Lolium populations evaluated in this study (Table 2). Mean plant height at maturity varied 2.2-fold between the shortest (51 cm) and the tallest (110.5 cm) populations (Table 2, T2), with an intrapopulation plant height difference ranging as high as 101 to 110 cm in 2% of the populations and about 51 to 60 cm in 45% of the populations (Figures 4B and 5B–E). Regrowth rate among the populations ranged from 2.2 to 7.0 on a scale of 1 to 7 (Table 2, T3), with 80% of the populations showing slow regrowth potential (<4). However, 87% of the populations showed high intrapopulation variability for this trait (Figure 4C).
Table 2. Diversity for morphological traits across the wild populations of Lolium perenne ssp. multiflorum from the Texas Blackland Prairies.

a The ratio of higher and lower extremes of the range. Values have been rounded to the nearest decimal point.
b Indicates significant difference among the populations for a particular trait.
c Regrowth rate was scored as follows: (1) <2 cm d−1, (3) 2–3.5 cm d−1, (5) 3.5–5 cm d−1, (7) >5 cm d−1.
d Growth habit was scored as follows: (1) >60° from the horizontal axis, (3) 30°–60°, (5) <30°; a greater angle indicates erectness and a smaller angle indicates prostrate growth habit.
e Leaf color was scored as follows: (1) light green, (3) green, (5) dark green.
f Basal node color was scored as follows: (1) light green, (3) green, (5) reddish.
g Plant texture was scored as follows: (1) very smooth, (3) smooth, (5) rough, (7) very rough.

Figure 4. Frequency distribution of the difference between maximum and minimum values for a given trait, illustrating intrapopulation variation. (A) Plant height before flowering (cm); (B) plant height at maturity (cm); (C) regrowth rate measured on a scale of 1 to 7, with 1 being very slow and 7 representing very fast growth; (D) growth habit measured on a scale of 1 to 5, with 1 being erect and 5 being prostrate; (E) leaf color measured on a scale of 1 to 5, with 1 being light green and 5 being dark green; (F) basal node color measured on a scale of 1 to 5, with 1 being light green and 5 being reddish; (G) leaf blade width (mm); (H) plant texture measured on a scale of 1 to 7, with 1 being very smooth and 7 being very rough; (I) tiller count per plant; (J) shoot dry biomass per plant (g); (K) spike count per plant; (L) spike length (cm); (M) seed count per spikelet; (N) seed count per spike; (O) seed count per plant; and (P) seed shattering (%). For example, in A, 4% of the populations had the greatest intrapopulation plant height difference of 61 to 70 cm between the shortest and tallest plants, whereas 5% of the populations had the lowest difference of 0 to 10 cm.

Figure 5. Image showing intrapopulation diversity for plant height (B vs. C; D vs. E), maturity (A–C), and growth habit (prostrate vs. erect) (B vs. C; D vs. E) in Lolium perenne ssp. multiflorum populations collected in the Texas Blacklands. Plants in A–C belong to a single population, as plants in D and E.
Table 3. Clustering of the Lolium perenne ssp. multiflorum populations from the Texas Blackland Prairies based on their morphological traits.a

a Abbreviations: PF, plant height before flowering (cm); PM, plant height at maturity (cm); RG, regrowth rate scored as follows: (1) <2 cm d−1, (3) 2–3.5 cm d−1, (5) 3.5–5 cm d−1, (7) >5 cm d−1; PG, plant growth habit scored as follows: (1) >60° from the horizontal axis, (3) 30°–60°, and 5 for <30°; LC, leaf color scored as follows: (1) light green, (3) green, (5) dark green; NC, basal node color scored as follows: (1) light green, (3) green, (5) reddish; LW, leaf blade width (mm); PT, plant texture scored as follows: (1) very smooth, (3) smooth, (5) rough, (7) very rough; TC, tiller count per plant; SD, shoot dry biomass per plant (g); SP, spike count per plant; SL, spike length (cm); SS, seed count per spikelet; SC, seed count per spike; TS, total seed count per plant; SH, seed shattering (%).
b Indicates the number of populations falling within a given cluster. Among the 56 populations used in the experiment, 51 had sufficient data for conducting cluster analysis.
Average plant growth habit was erect but varied widely from prostrate to erect (2.7-fold variation) (Table 2, T4; Figure 5, B vs. C and D vs. E), with 39% of the populations showing an intrapopulation difference of 3.1 to 4.0 on a scale of 1 to 5 (Figure 4D). Lolium is a highly tillering species and, on average, produced about 10 tillers per plant (Table 2, T9); mean tiller count varied from 5 to 14 across populations, with 27% of the populations showing an intrapopulation difference of 9.1 to 18 tillers per plant (Figures 4I and 6D and E). In general, most populations had green leaf and basal node color but varied greatly at the intrapopulation level (Figure 4E and F). In particular, leaf color varied from light green to dark green (Figure 6A–C). Mean leaf blade width, which is considered a key taxonomic trait in Lolium (Bararpour et al. Reference Bararpour, Norsworthy, Burgos, Korres and Gbur2017), varied between 2 and 12 mm at an individual leaf level across all plants (Figure 7), with 30% of the populations having the highest intrapopulation difference of 4.1 to 6 mm (Figure 4G). Though the average plant texture was smooth, there was a high intrapopulation variability for this trait (Figure 4H). Average plant size varied tremendously among the populations, and there was an 11.3-fold interpopulation difference for shoot biomass (Table 2, T10); about 9% of the populations exhibited the highest intrapopulation shoot biomass difference of 12.1 to 16.5 g (Figure 4J).
Table 4. Principal components analysis (PCA) of wild populations of Lolium perenne ssp. multiflorum from the Texas Blackland Prairies based on 16 morphological traits.


Figure 6. An example of diversity among the Lolium perenne ssp. multiflorum populations evaluated in this experiment for leaf color (A–C) and tillering habit (D and E).

Figure 7. Diversity among the Lolium perenne ssp. multiflorum populations evaluated in this experiment for leaf blade width.
Yield Traits
Plant yield traits were also highly variable among the Lolium populations (P < 0.001; Table 2). Spike count per plant, which determines fecundity, had a mean interpopulation variability of 2 to 11, but some populations had an intrapopulation difference as high as 40 spikes per plant (Figure 4K). Spike length, however, was relatively consistent across populations and also less variable at the intrapopulation level (Figure 4L). Mean seed count per spikelet varied from 1.4 to 5.7 at the interpopulation level (Table 2, T13), with 18% of the populations exhibiting an intrapopulation difference of 6.1 to 8 seeds per spikelet (Figure 4M). Across all plants, seed production per spikelet varied from 1 to 20 (data not shown). The mean seed number was 432 seeds per plant, and there was 11.3-fold variation for this trait among the different populations (Table 2, T15). It is important to note that the spikes showed a greater taxonomic variation, ranging from branched rachis (i.e., panicle type) to spike with multiple spikelets originating at the same point (Figure 8). The seeds also highly varied for the presence and length of awns (Figure 3). Seed shattering, an important weedy trait, varied from 5% to 54% among the populations (Table 2, T16). With respect to intrapopulation variability, seed shattering showed a continuous frequency distribution across all the populations investigated (Figure 4P).
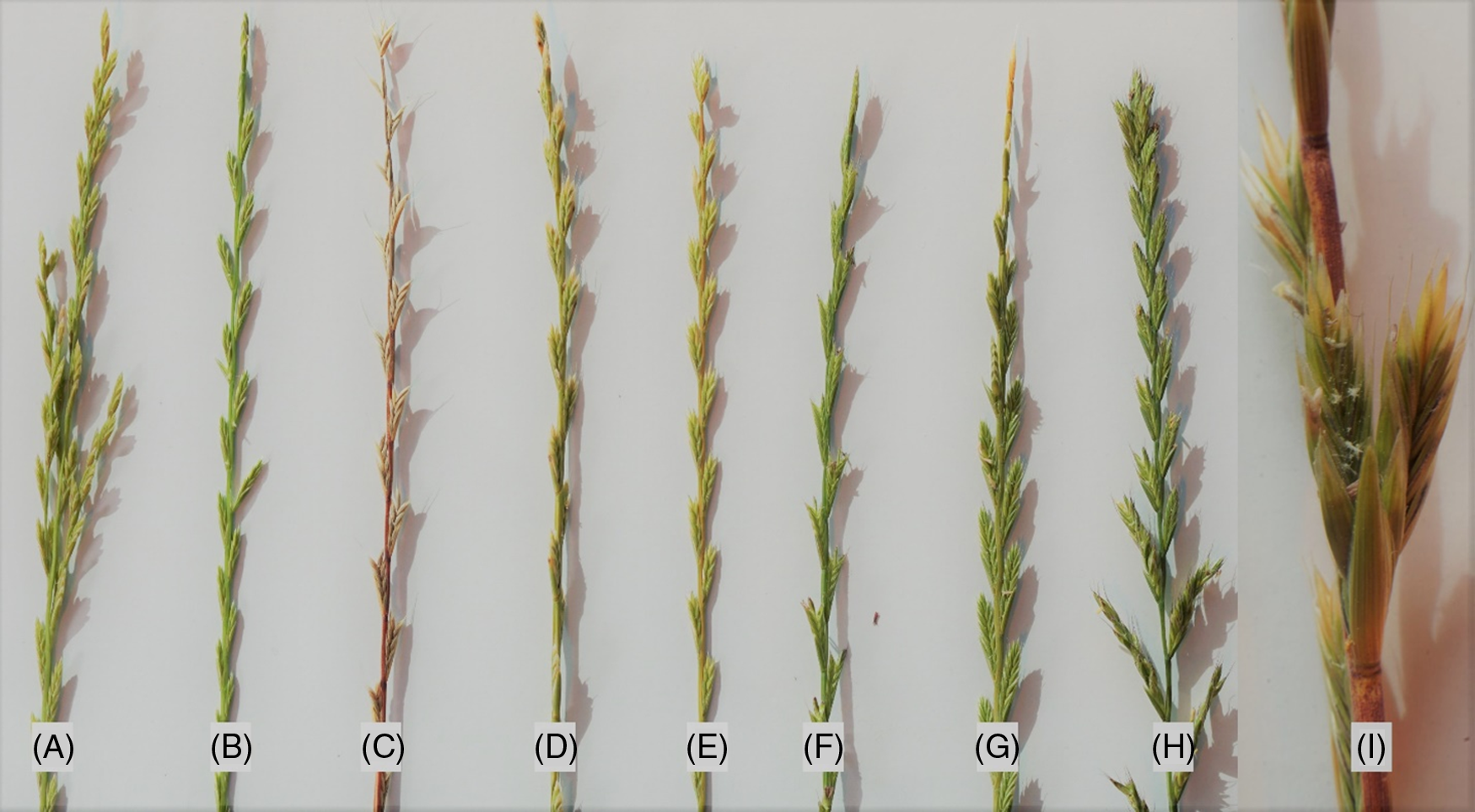
Figure 8. Diversity among Lolium perenne ssp. multiflorum populations collected in the Texas Blackland Prairies for spike morphology and maturity differences: (A) short or no awn, rare panicle type with 12–15 seeds per spikelet on main rachis but 5–7 seeds per spikelet on branches; (B) short or no awn with 5–7 seeds per spikelet; (C) long outer glume and awn, but early maturity with 5–7 seeds per spikelet; (D) long awn, spikelet arrangement on rachis is alternate spiral with 5–7 seeds per spikelet; (E) long awn, spikelet arrangement on rachis is alternate distichous with 8–10 seeds per spikelet; (F) long outer glume and awn, but late maturity with 5–7 seeds per spikelet; (G) short awn with 14–16 seeds per spikelet; (H) very long awn with 14–16 seeds per spikelet; and (I) spike with multiple spikelets at the same point.
Overall, a high degree of intra- as well as interpopulation diversity was observed for the different morphological and yield traits investigated here. High intrapopulation diversity favors field survival of a weed population under varying environmental and management conditions, which ultimately enhances the persistence of the population (Dekker Reference Dekker2011). The specific adaptive trait(s) favorable to a population can be governed by various factors, including the nature of the selection agent and the environment, but the existence of diversity for such traits allows for selection to act on them. Our findings show that Lolium is well equipped for adaptive evolution due to the presence of high levels of diversity.
Bararpour et al. (Reference Bararpour, Norsworthy, Burgos, Korres and Gbur2017) also found high diversity for different traits in a large collection of L. perenne ssp. multiflorum in Arkansas. Similar findings of high diversity in L. perenne ssp. multiflorum were also reported by Rosell (Reference Rosell1967) and Terrell (Reference Terrell1968). Lolium perenne ssp. multiflorum is a highly outcrossing species (Lopes et al. Reference Lopes, Reis, Barata and Nunes2009; Tobina et al. Reference Tobina, Yamashita, Koizumi, Fujimori, Takamizo, Hirata, Yamada and Sawada2008), and continuous gene flow and allele exchange could be attributed to the high degrees of intra- and interspecific diversity observed in this species. Cross-pollination between diverse biotypes of L. perenne ssp. multiflorum and other compatible species can lead to numerous intermediate types with wide variation for morphological and yield traits (Cresswell et al. Reference Cresswell, Sackville, Roy and Viegas2001; Schoth and Weihing Reference Schoth, Weihing, Hughes, Heath and Metcalfe1962; Wang et al. Reference Wang, Cogan, Pembleton and Forster2015). Further, the lack of intensive domestication in this species may also have contributed to the wide diversity for adaptive traits (Lopes et al. Reference Lopes, Reis, Barata and Nunes2009). From an adaptive evolution standpoint, the occurrence of high intra- and interspecific diversity can greatly favor invasion and persistence of Lolium across changing management regimes and climatic conditions, as has been reported with a large number of other weeds (Dukes and Mooney Reference Dukes and Mooney1999; Nicotra et al. Reference Nicotra, Atkin, Bonser, Davidson, Finnegan, Mathesius, Poot, Purugganan, Richards, Valladares and van Kleunen2010). This also allows for rapid invasion and colonization of new habitats by the species without the need for local selection (Williams et al. Reference Williams, Mack and Black1995).
Diversity coupled with selection by crop management practices favors the accumulation of beneficial traits and promotes weed species persistence (Dekker Reference Dekker2011; Owen et al. Reference Owen, Goggin and Powles2015). For example, populations with reduced growth rate and higher seed dormancy in L. rigidum (Vila-Aiub et al. Reference Vila-Aiub, Neve and Powles2005), reduced growth rate in goosegrass [Eleusine indica (L.) Gaertn.] (Han et al. Reference Han, Vila-Aiub, Jalaludin, Yu and Powles2017) and kochia [Bassia scoparia (L.) A.J. Scott] (Murphy and Tranel Reference Murphy and Tranel2019), and reduced plant height and fecundity in L. perenne (Yanniccari et al. Reference Yanniccari, Vila-Aiub, Istilart, Acciaresi and Castro2016) were reported to be associated with herbicide resistance. It has been reported that tall plant height and high shoot biomass generally enhance species competitiveness (Blair Reference Blair2001; Fraga et al. Reference Fraga, Agostinetto, Vargas, Nohatto, Thurmer and Holz2013), and the presence of diversity for these traits may facilitate species dominance in a competitive environment. Seed shattering is another important weedy trait, and diversity for this trait offers a weed population an extended window for seed dispersal (Burton et al. Reference Burton, Beckie, Willenborg, Shirtliffe, Schoenau and Johnson2017; Delouche et al. Reference Delouche, Burgos, Gealy, Zorrilla de San Martin, Labrada, Larinde and Rosell2007) and facilitates adaptation to management (Ashworth et al. Reference Ashworth, Walsh, Flower, Vila-Aiub and Powles2016). For instance, the success of harvest weed seed control (HWSC) strategies relies on the ability of weeds to retain seed at the time of harvest (Walsh et al. Reference Walsh, Newman and Powles2013). Selection imposed by HWSC can lead to adaptation by favoring early-shattering phenotypes if there is sufficient diversity for seed shattering in the weed population (Ashworth et al. Reference Ashworth, Walsh, Flower, Vila-Aiub and Powles2016).
Species Identification
Among the total populations (68) collected from the Texas Blackland Prairies, four were found to be L. perenne based on the morphological and taxonomic comparison with GRIN reference samples (Maity et al. Reference Maity, Abugho, Singh, Subramanian, Smith and Bagavathiannan2019). However, all 56 Lolium populations evaluated in the current study were variants of L. perenne ssp. multiflorum with a few offtypes of L. perenne or probable hybrids between the two species (Figures 2 and 3).
The high inter- as well as intrapopulation diversity observed here for various traits can easily lead to misinterpretation that they belong to different species (Balfourier et al. Reference Balfourier, Imbert and Charmet2000). Lolium species identification has always been challenging for growers and seed certification officers (Rosell Reference Rosell1967). Plant height, growth habit, leaf blade width, spikelet count per plant, and presence/absence of awn are considered as key taxonomically important traits for Lolium identification (Rosell Reference Rosell1967). However, the variation observed for these traits in our populations indicate that these traits are not solely reliable for species identification. Other studies have proposed that presence/absence of awn, leaf blade width (Bararpour et al. Reference Bararpour, Norsworthy, Burgos, Korres and Gbur2017), and spikelet count per plant (Terrell Reference Terrell1968) can be used for this purpose, yet our results do not support these suggestions. Moreover, as argued by Jenkin (Reference Jenkin1933), Lolium is still relatively new in origin and is still accumulating morphological and physiological changes that may eventually lead to divergence of new ecotypes and even speciation.
Population Clustering
In hierarchical cluster analysis, six distinct clusters were formed based on the 16 different morphological traits at a 47% similarity level (Figure 9). These six clusters (1 to 6) consisted of 16, 8, 5, 14, 5, and 3 populations, respectively. In general, the clusters did not consistently follow any specific geographic association for population grouping (Figures 1 and 9), but there were several notable commonalities for functional traits. The populations in the cluster 5 represented erect and tall plants, which in turn produced the maximum shoot dry biomass per plant (7.3 g), spikes per plant (9), and spike length (23 cm), and the second highest tiller count per plant (11), representing the most vigorous group. Plants in this cluster also had the narrowest leaves (1.2-mm wide) and the greatest seed shattering (29%) (Table 3).

Figure 9. Hierarchical clustering showing grouping of the Lolium perenne ssp. multiflorum populations based on 16 morphological traits, computed based on correlations. In the dendrogram, the relative distances between clusters are given by the horizontal distances between vertical lines that join the clusters. The blue line at the bottom represents the similarity index among the clusters which indicates 0%–100% from left to right. The change in slope of the blue line indicates that the differences in clusters that are joined up to the point are comparatively small. The vertical red line marks the similarity level at which the number of clusters was chosen. WC with a specific number indicates the population number used in the study.
Cluster 6 had only three populations, representing short plants (24.9 cm before flowering and 61.3 cm at maturity), with a rapid regrowth rate (Table 3). However, the regrowth rate did not correspond to shoot dry biomass production or seed production, probably due to the adverse effect of repeated trimming during the peak growth period, which may have partitioned more photosynthates toward foliar growth (Table 3). Populations in cluster 4 had the greatest tiller count per plant, which resulted in the maximum seed count per plant (Table 3). Cluster 1 generally produced low seeds per spikelet (3) or per plant (453), with a fair amount of seed shattering (16%) (Table 3). It was observed in cluster 1 that plants with spreading growth habit also had dark green leaves. Populations in cluster 3 generally had prostrate to semi-erect plants with wide leaves and rough plant texture.
Several studies have clustered wild biotypes and/or landraces of Lolium based on morphological and genetic diversity (Charmet et al. Reference Charmet, Balfourier and Monestiez1994; Jafari et al. Reference Jafari, Hessamzadeh, Abdi and Saeedi2003; Loos Reference Loos1994; Lopes et al. Reference Lopes, Reis, Barata and Nunes2009; Monestiez et al. Reference Monestiez, Goulard and Charmet1994; Oliveira et al. Reference Oliveira, Lindner, Bregu and González1997). In all these studies, Lolium showed a wide range of diversity and was grouped predominantly based on functional trait similarity. Blackmore et al. (Reference Blackmore, Thomas, McMahon, Powell and Hegarty2015, Reference Blackmore, Thorogood, Skøt, McMahon, Powell and Hegarty2016) grouped European ecotypes of Lolium in relation to their geographic origin and distribution. However, Panozzo et al. (Reference Panozzo, Collavo and Sattin2020) did not find any grouping based on geographic origin among different Lolium ecotypes studied in Italy. In the current study, the clusters did not show any association with the geographic origin of the populations; rather, they were grouped by common functional traits. The occurrence of high levels of gene flow in Lolium (Balfourier et al. Reference Balfourier, Charmet and Ravel1998) may have masked differences among geographically close populations (Balfourier et al. Reference Balfourier, Imbert and Charmet2000). Further, movement of equipment such as combine harvesters among nearby farms could also be contributing to propagule dispersal and reduced population differentiation (Anderson and Hartzler Reference Anderson and Hartzler2018). Likewise, Lopes et al. (Reference Lopes, Reis, Barata and Nunes2009) reported high similarity for adaptive traits among closely occurring Lolium populations in Portugal, suggesting the contribution of gene flow. However, local selection as influenced by management practices implemented within a production field, which is known to vary greatly among fields within a geography, might be contributing to random population differences for certain adaptive traits that are eventually clustered together. In addition to local selection, random propagule dispersal in Lolium through contaminated planting seed (Benvenuti Reference Benvenuti2007), dispersal by cattle manure and soil amendments (Fischer et al. Reference Fischer, Poschlod and Beinlich1996; Yamada et al. Reference Yamada, Matsuo and Tamura1972), irrigation water (Tosso et al. Reference Tosso, Ferreyra and Muñoz1986), random movement of custom farm equipment (Burke et al. Reference Burke, Kahl and Tautges2017; Hodkinson and Thompson Reference Hodkinson and Thompson1997), and other anthropogenic means may be contributing to the clustering pattern observed here.
Principal Component Analysis
The principal components analysis (PCA) revealed that the first four components explain 59% of the total variation among the populations. The first four components and traits with the greatest loadings are given in Table 4. The first principal component (PC1) is attributed mainly to the yield traits, whereas PC2 and PC3 pertain primarily to the vegetative traits. A biplot and a loading plot for the first two principal components are presented in Figure 10. Total seed count per plant (TS) contributes to the most variability in the PC1, followed by the seed count per spike (SC), spike length (SL), and tiller count per plant (TC), whereas the basal node color (NC) contributes the highest in PC2, followed by the leaf color (LC), regrowth rate (RG), and seed count per spike (SC) (Figure 10). PC1 and PC2 together accounted only for 39% of the variability, so the distinct grouping of Lolium populations was not clear from the biplot. However, some populations show distinct separation from the main group. For example, WC22 was placed in PC2 away from the other populations; close examination of the data reveals that this population had reddish basal nodes with dark green leaves, fast regrowth rate, and moderately high seed count per spike (SC), whereas the other populations in PC2 had green basal nodes and leaves.

Figure 10. Principal component analysis of Lolium perenne ssp. multiflorum populations with 16 morphological traits evaluated in this study. (A) Loading plot: WC with a specific number indicates the population number used in the study. (B) Biplot or scoring plot: PF, plant height before flowering (cm); PM, plant height at maturity; RG, regrowth rate scored as follows: (1) <2 cm d−1, (3) 2–3.5 cm d−1, (5) 3.5–5 cm d−1, (7) >5 cm d−1; PG, plant growth habit scored as follows: (1) >60° from the horizontal axis, (3) 30°–60°, (5) <30°; LC, leaf color scored as follows: (1) light green, (3) green, (5) dark green; NC, basal node color scored as follows: (1) light green, (3) green, and (5) reddish; LW, leaf blade width (mm); PT, plant texture scored as follows: (1) very smooth, (3) smooth, (5) rough, (7) very rough; TC, tiller count per plant; SD, shoot dry biomass; SP, spike count per plant; SL, spike length (cm); SS, seed count per spikelet; SC, seed count per spike; TS, total seed count per plant; SH, seed shattering (%).
PCA has been successfully utilized for understanding the multidimensional variability through linear combinations in Lolium (Bennett Reference Bennett1997; Lopes et al. Reference Lopes, Reis, Barata and Nunes2009). Diversity for morphological and yield traits among Lolium populations have been shaped by the environments they were exposed to, which directly influence grouping and contribution of specific traits to the total variability (Lopes et al. Reference Lopes, Reis, Barata and Nunes2009). In a study involving various Lolium species, Bennett (Reference Bennett1997) showed that PC1 pertained to vegetative traits, whereas PC2 and PC3 were influenced by yield traits. In the current study, most of the populations were grouped together, suggesting natural hybridization and propagule movement leading to a lack of population differentiation (Arojju et al. Reference Arojju, Barth, Milbourne, Conaghan, Velmurugan, Hodkinson and Byrne2016; Blackmore et al. Reference Blackmore, Thorogood, Skøt, McMahon, Powell and Hegarty2016; Jonaviciene et al. Reference Jonaviciene, Statkeviciute, Kemesyte and Brazauskas2014; Thorogood et al. Reference Thorogood, Yates, Manzanares, Skot, Hegarty, Blackmore, Barth and Studer2017). However, some populations such as WC22, WC4, and WC31(2), tend to be positioned away from the others in the PCA, which likely reflects their distinctive traits evolved through continuous adaptation in a particular habitat as a result of local selection (Rosell Reference Rosell1967).
Correlation Analysis
Correlation among the Different Morphological and Yield Traits
A correlation matrix heat map of the different traits being studied provided vital information on the nature of association among the traits (Figure 11). Plant height (before flowering) showed positive correlation with tiller count (P = 0.02), spike count (P = 0.0003), shoot dry biomass (P = 0.006), and seed count (P = 0.05), but not with spike length, seed count per spike, or total seed count; however, plant height at maturity was significantly correlated with spike length and total seed count, but not with tiller count (Figure 11). Regrowth rate was positively correlated with leaf and basal node color; the darker the basal node color, the greater the regrowth rate. Plants with dark basal node color also had a prostrate growth habit. Tiller count was highly correlated with shoot dry biomass (P = 0.002), spike length (P = 0.01), spike count (P = 0.0001), and seed count per spike (P = 0.04), which further contributed to its positive association with total seed count per plant (P = 0.0007). Moreover, the different yield traits were highly correlated with one another (P < 0.0001) (Figure 11). Seed shattering was not significantly associated with any of the morphological and yield traits studied.

Figure 11. Correlation matrix showing the strength of association between different morphological traits in the Lolium perenne ssp. multiflorum populations collected in the Texas Blackland Prairies. The marker band with numbers on the right side indicates significance level, where red (0) represents the highest significance level, and blue (1) represents the lowest significance level.
Correlation between taxonomically as well as agronomically important traits offers valuable insights into how traits evolve and interact under diverse cropping intensities and production practices (Korres et al. Reference Korres, Norsworthy, Tehranchian, Gitsopoulos, Loka, Oosterhuis, Gealy, Moss, Burgos, Miller and Palhano2016). Further, knowledge of correlation between different traits allows for combining desirable traits for breeding improved Lolium cultivars for use as forage or turf species (Jian et al. Reference Jian, ShengRong and AiXing2000). Many studies have examined correlation among different morphological and yield traits in relation to herbicide sensitivity and have found variable degrees of association (Owen et al. Reference Owen, Goggin and Powles2015; Vila-Aiub et al. Reference Vila-Aiub, Yu and Powles2019; Watkinson and White Reference Watkinson and White1985). Parallel evolution of strongly associated traits leads to better adaptation and/or elimination of a less fit biotype in a cropping environment, which has tremendous ecological and agronomic importance (Oliveira et al. Reference Oliveira, Lindner, Bregu and González1997). Evidence suggests that cropping systems and management practices influence trait association in different weed species, improving their fitness in their environments (Fried et al. Reference Fried, Chauvel, Munoz and Reboud2019; Vila-Aiub et al. Reference Vila-Aiub, Yu and Powles2019).
Correlation between Morphological and Yield Traits and Herbicide Resistance
The leaf blade width showed positive correlation with survival to pinoxaden (r = 0.3, P < 0.05) and multiple herbicides (r = 0.3, P < 0.05), whereas spike count was negatively correlated with survival to mesosulfuron-methyl (r = −0.3, P < 0.05) (Figure 11). Survival to diclofop was negatively correlated with plant height at maturity (r = −0.3, P < 0.07) and positively with plant texture (r = 0.2, P < 0.07).
It has been reported that intensive herbicide use patterns have selected for increased or decreased expression of certain traits parallel to resistance evolution in weeds (Darmency et al. Reference Darmency, Colbach and Corre2017; Jasieniuk et al. Reference Jasieniuk, Brule-Babel and Morrison1996; Martinez-Ghersa et al. Reference Martinez-Ghersa, Ghersa, Benech-Arnold, Mac Donough and S´anchez2000; Mortimer Reference Mortimer1997). Different plant adaptive traits such as competitiveness, biomass, height, leaf area, regrowth rate, photosynthetic rate, or seed production potential have been investigated concurrent with herbicide-resistance evolution (reviewed in Mortimer Reference Mortimer1997; Watkinson and White Reference Watkinson and White1985). Ghanizadeh and Harrington (Reference Ghanizadeh and Harrington2019) reported that triazine-resistant common lambsquarters (Chenopodium album L.) phenotypes were shorter and produced less biomass than susceptible phenotypes. Delayed germination was recorded in diclofop-resistant L. perenne ssp. multiflorum (Ghersa et al. Reference Ghersa, Martínez-Ghersa, Brewer and Roush1994; Gundel et al. Reference Gundel, Martinez-Ghersa and Ghersa2008) and L. rigidum (Vila-Aiub et al. Reference Vila-Aiub, Neve and Powles2005). Henckes et al. (Reference Henckes, Cechin, Schmitz, Piasecki, Vargas and Agostinetto2019) reported greater plant height, shoot dry matter, and absolute growth rate and reduced number of tillers and leaf area ratio in L. perenne ssp. multiflorum biotypes resistant to glyphosate, iodosulfuron-methyl, and pyroxsulam compared with susceptible biotypes. In our study, plant height, leaf width, plant texture, and spike count were the only traits that were correlated with herbicide resistance. Contrary to the report of Henckes et al. (Reference Henckes, Cechin, Schmitz, Piasecki, Vargas and Agostinetto2019), our finding of positive correlation between leaf width and herbicide resistance indicates that resistant plants may have a greater photosynthetic potential, leading to greater fitness, though this phenomenon is yet to be confirmed in our populations. Nevertheless, it appears that the specific correlations observed vary greatly across weed species, traits, and herbicides and may be influenced by various genetic, biological, and management factors.
All Lolium populations investigated in this study were L. perenne ssp. multiflorum, which confirms the predominant occurrence of this species in the Texas Blackland Prairies. Lolium perenne ssp. multiflorum boasts a high level of diversity, both intra- as well as interpopulation, which allows it to constantly adapt to changing habitat conditions and invade new geographic regions. The range of diversity found in the Texas Blacklands Lolium populations is consistent with the observation of multiple herbicide resistance in them. The high outcrossing nature of the species and long-distance propagule movement may further worsen the situation. Given the high diversity and adaptive potential of Lolium, management practices must involve diverse tactics within an integrated weed management framework. Additionally, managing Lolium populations occurring in field edges, roadsides, and other noncultivated areas may be a useful stewardship practice. Information generated from this study provides an improved understanding of adaptive trait diversity in L. perenne ssp. multiflorum and association among them, offering valuable insights into species persistence and informing management considerations. Elucidating the physiological, biochemical, and molecular mechanisms underpinning the correlation between various plant morphological traits and herbicide resistance warrants further investigation.
Acknowledgments
The Netaji Subhas-Indian Council of Agricultural Research International Fellowship (ICAR-IF) and the Tom Slick Fellowship from the College of Agriculture and Life Sciences, Texas A&M University, provided to AM are gratefully acknowledged. We thank Seth Abugho for assisting with the field survey and all graduate students, student workers, and interns of the Texas A&M Weed Science Research program for assisting with the characterization of the Lolium populations. The authors declare that no conflicts of interest exist.