Introduction
Grain sorghum is a popular crop to include in a crop rotation, because it permits the effective use of atrazine for control of many weeds, particularly broadleaf weeds (Owen and Powles Reference Owen and Powles2010). However, producers still face weed control issues in grain sorghum because of the limited POST chemical options for weedy grass control once the crop has emerged (Smith and Scott Reference Smith, Scott, Espinoza and Kelley2015). More specifically, johnsongrass [Sorghum halepense (L.) Pers.], fall panicum (Panicum dichotomiflorum Michx.), barnyardgrass [Echinochloa crus-galli (L.) Beauv.], broadleaf signalgrass (Urochloa platyphylla Nash), and Texas panicum [Panicum texanum (Buckl.) R. Webster] can be the most troublesome grass species.
The ALS chemistry has not been available in grain sorghum until now because of the strong genetic similarities between johnsongrass and grain sorghum (Bowers et al. Reference Bowers, Abbey, Anderson, Chang, Draye, Hoppe, Jessup, Lemke, Lennington, Li, Lin, Liu, Luo, Marler, Ming, Mitchell, Oiang, Reischmann, Schulze, Skinner, Wang, Kresovich, Schertz and Paterson2003). However, with the discovery of nicosulfuron-resistant weedy sorghum, researchers were able to cross-pollinate nicosulfuron resistance into grain sorghum, allowing for safe use of nicosulfuron in the crop (Anonymous 2016; Tuinstra and Al-Khatib Reference Tuinstra and Al-Khatib2008).
Johnsongrass can reproduce through seed as a summer annual or through rhizomes as a perennial. Johnsongrass can produce up to 28,000 seeds and up to 90 m of rhizomes in one season of grain sorghum growth (Horowitz Reference Horowitz1973). Not only does johnsongrass reduce grain sorghum yield by competing with the crop, but it also produces allelopathic chemicals that inhibit grain sorghum growth (Mueller et al. Reference Mueller, Lewis, Green and Burns1993). Johnsongrass alone can reduce corn (Zea mays L.) yields 74% to 100% (Bendixen Reference Bendixen1986). Control of the troublesome weed has been achieved in other crops such as soybean (Glycine max L.) by using selective herbicides such as dinitroanilines and acetyl-coenzyme A carboxylase-inhibiting herbicides (Langemeier and Witt Reference Langemeier and Witt1986; McWhorter Reference McWhorter1977; Riley and Shaw Reference Riley and Shaw1988). The evolution of resistance, however, has reduced these options (Heap Reference Heap2020). The introduction of glyphosate-resistant corn, cotton, and soybeans provided a more effective option for control with glyphosate. Prior to the introduction of glyphosate-resistant corn, nicosulfuron was the primary option for POST control of grasses in corn.
With the Inzen™ technology introduced to the market, questions have been proposed as to whether or not the herbicide-resistant trait will confer resistance to other Weed Science Society of America (WSSA) Group 2 ALS-inhibiting herbicides. ALS-inhibiting herbicides, as defined by the WSSA (2017), primarily starve the plant of branched-chain amino acids, specifically leucine, isoleucine, and valine. The five families composing herbicides that inhibit ALS function include sulfonylureas, imidazolinones, pyrimidinylthiobenzoics, triazolinones, and triazolopyrimidines.
Cross-resistance across a suite of ALS-inhibiting herbicide families was documented by Tranel and Wright (Reference Tranel and Wright2002). However, their research found that ALS resistance generally is grouped into one of three categories: sulfonylurea resistant, imidazolinone resistant, or a broad cross-resistance. Resistance to ALS herbicides was first discovered only 5 yr after the introduction of the first sulfonylurea herbicide chlorsulfuron. The mechanism of resistance to chlorsulfuron was reduced sensitivity of the target ALS enzyme to inhibition by the herbicide (Mallory-Smith et al. Reference Mallory-Smith, Thill and Dial1990). Currently, there are nine confirmed ALS enzyme mutations that confer resistance to ALS-inhibiting herbicides: Ala122, Pro197, Ala205, Asp376, Arg377, Trp574, Ser653, Val560, and Gly654. The Ala122 mutation is the most common one documented to confer cross-resistance (Tranel et al. Reference Tranel, Wright and Heap2016). PCR screening of Inzen™ sorghum revealed a double mutation of Val560Ile and Trp574Leu (Tuinstra and Al-Khatib Reference Tuinstra and Al-Khatib2008). The Trp574Leu point mutation has been found in Palmer amaranth (Amaranthus palmeri S. Watson), which resulted in ALS cross-resistance to all five families in the species (Molin et al. Reference Molin, Nandula, Wright and Bond2016). The Val560Ile point mutation has also been documented conferring ALS cross-resistance to all five ALS-inhibiting herbicide families in johnsongrass (Werle et al. Reference Werle, Jhala, Yerka, Dille and Lindquist2016). Another mechanism of ALS-inhibiting herbicide resistance is increased herbicide metabolism resulting in detoxification of the herbicide. However, enhanced metabolism only results in cross-resistance to ALS herbicides less than 10% of the time (Hall et al. Reference Hall, Holtum, Powles, Powles and Holtum1994). Therefore, the objective of this research was to evaluate the cross-resistance of Inzen™ grain sorghum to PRE and POST applications of various WSSA Group 2 ALS-inhibiting herbicides.
Materials and Methods
Research was conducted at the Lon Mann Cotton Research Station (LMCRS) near Marianna, AR (34.44°N, 90.45°W), and the Arkansas Agricultural Research and Extension Center (AAREC) in Fayetteville, AR (36.05°N, 94.55°W), in 2016 and 2017, and the Pine Tree Research Station (PTRS) near Colt, AR, (35.07°N, 90.10°W), in 2016 to evaluate the scope of cross-resistance of Inzen™ sorghum herbicides to WSSA Group 2. The soil texture at LMCRS was a Calloway silt loam (fine-silty, mixed, active, thermic Aquic Fraglossudalfs) with 2% sand, 82.3% silt, and 15.6% clay, a pH of 5.5, and 2.2% organic matter (OM). The AAREC soil texture was a Captina silt loam (fine-silty, siliceous, active, mesic Typic Fragiudults) with 22% sand, 64% silt, and 14% clay, a pH of 5.8, and 1.8% OM. The PTRS soil texture was a Calloway silt loam (fine-silty, mixed, active, thermic Aquic Fraglossudalfs) with 10.6% sand, 68.6% silt, and 20.8% clay, a pH of 7.5, and 1.3% OM.
Inzen™ (Pioneer, Johnston, IA) and a Dekalb (Monsanto Co., St. Louis, MO) conventional grain sorghum cultivar (‘DKS 53-67’) were planted in the PRE experiment at all locations (Figures 1–3). Cultivars were planted at 217,000 seeds ha–1 to a 2.5- to 3-cm depth in both years. All plots consisted of two rows, 8.5 m long. Plots to evaluate ALS resistance PRE were arranged as a split-plot design where the whole-plot factor was ALS herbicide applied (22 herbicides), and the sub-plot factor was seed technology planted (Inzen™ or conventional). The POST experiment was planted to Inzen™ sorghum only and treated POST with multiple ALS-inhibiting herbicides. The experimental design was a randomized complete block with the fixed effect being herbicide treatment and random effects consisting of site-year and replication.

Figure 1. Planting/application timing and rainfall at Marianna, AR (LMCRS) in 2016 and 2017.
PRE herbicide applications were made immediately following planting, and POST herbicide applications were made when sorghum reached the V4 growth stage using an air-pressurized tractor-mounted spray boom at LMCRS and a CO2-pressurized backpack sprayer at PTRS and AAREC. Both sprayers were equipped with TeeJet® Air Induction XR 110015 nozzles (TeeJet® Technologies, Glende Heights, IL). All treatments were applied with a ground speed of 4.8 km h–1. Herbicides were applied in a spray volume of 112.2 L ha–1 at LMCRS and 140 L ha–1 at PTRS and AAREC. Herbicide treatments and corresponding rates are listed in Table 1.
Table 1. Herbicides and rates applied for PRE and POST acetolactate synthase (ALS)–inhibiting herbicide sensitivity experiments in 2016 and 2017.a–c

a Sensitivity experiment conducted near Colt, AR, in 2016.
b Sensitivity experiment conducted near Marianna, AR, in 2016 and 2017.
c Sensitivity experiment conducted in Fayetteville, AR, in 2016 and 2017.
Atrazine (Aatrex; Syngenta Crop Protection, LLC, Greensboro, NC) plus S-metolachlor (Dual; Syngenta Crop Protection, LLC, Greensboro, NC) were applied at planting to help maintain weed-free plots. Any escapes from the PRE application were controlled by a POST application of the same mixture. Further escapes were hand-weeded. Recommendations from soil sample results analyzed at the soil testing and research laboratory in Marianna, AR, were followed for fertility management. Pest management decisions were based on University of Arkansas Extension recommendations (Espinoza Reference Espinoza2015; McLeod and Greene Reference McLeod, Greene, Espinoza and Kelley2015). Traditional furrow irrigation was used to provide soil moisture for all tests, except in Fayetteville where overhead irrigation was used.
Visible ratings for crop injury and sorghum heights were recorded at 2 and 4 wk after planting (WAP) and POST application (WAA). Visible ratings of herbicide application were on a 0 to 100% scale, with 0 being no injury and 100% equaling plant death (Frans et al. Reference Frans, Talbert, Crowley and Camper1986). Five random plants in each plot were measured to estimate an average of sorghum height and then divided by the average sorghum height of nontreated plots so as to obtain relative sorghum heights. Because of an issue of sterile seed in 2016, yield data were only collected in 2017. Both rows of Inzen™ and conventional sorghum were harvested using a small-plot combine, and grain moisture was adjusted to 14%. Harvested plots were recorded as kg ha–1 and converted to relative yield by dividing each plot by the average yield of the nontreated plots.
All data collected were subjected to ANOVA using JMP (JMP Pro13, SAS Institute Inc., Cary, NC), with significant means separated using Fisher’s protected LSD (α = 0.05). As the nontreated plot in each replication was used to convert sorghum height and yield to a percentage of the nontreated, data from these plots were not included in the analysis. All treatments containing Inzen™ technology were excluded from the statistical analysis of visible crop injury, because no injury was observed. Therefore, in the PRE experiment visible injury to the conventional hybrid was analyzed as a randomized complete block. All data between site-years were analyzed together, with locations considered random.
Results and Discussion
Sensitivity to PRE Applications of ALS-Inhibiting Herbicides
All experiments received adequate rainfall within 7 d of application, with the exception of LMCRS in 2016 (Figures 1–3). Soil moisture is important for activation of soil-applied herbicides (Curran Reference Curran2001). Because herbicides applied to the soil are absorbed by the hypocotyl of plants during germination, the soil must receive adequate rainfall or irrigation within 7 d of application for herbicide activity. To combat the lack of rainfall, an irrigation application was applied on May 18, 2016 at LMCRS. Activation of herbicides by irrigation did not result in a difference of visible injury 2 WAP (P = 0.1725) or 4 WAP, (P = 0.3930) between locations.
For the response variable visible injury at 2 WAP, a main effect of herbicide was found (P = 0.0309) (Table 2). At 2 WAP, flumetsulam-methyl and bispyribac-Na caused the least injury (6%) to grain sorghum, and thiencarbazone-methyl caused the most injury (96%). Bispyribac-Na is used for POST control of weeds (Anonymous 2012) and does not have residual activity. Inzen™ grain sorghum at 2 WAP had a high degree of resistance to all herbicides applied based on no more than 1% injury observed (data not shown). Nicosulfuron, which is labeled in Inzen™ grain sorghum, caused 49% injury to conventional sorghum (Table 3). These results were consistent with previous studies of injury to conventional grain sorghum applied with nicosulfuron, which showed levels ranging from 19% to 67% depending upon rate applied (Matocha and Jones Reference Matocha and Jones2015).

Figure 2. Planting/application timing and rainfall at Fayetteville, AR (AAREC) in 2016 and 2017.
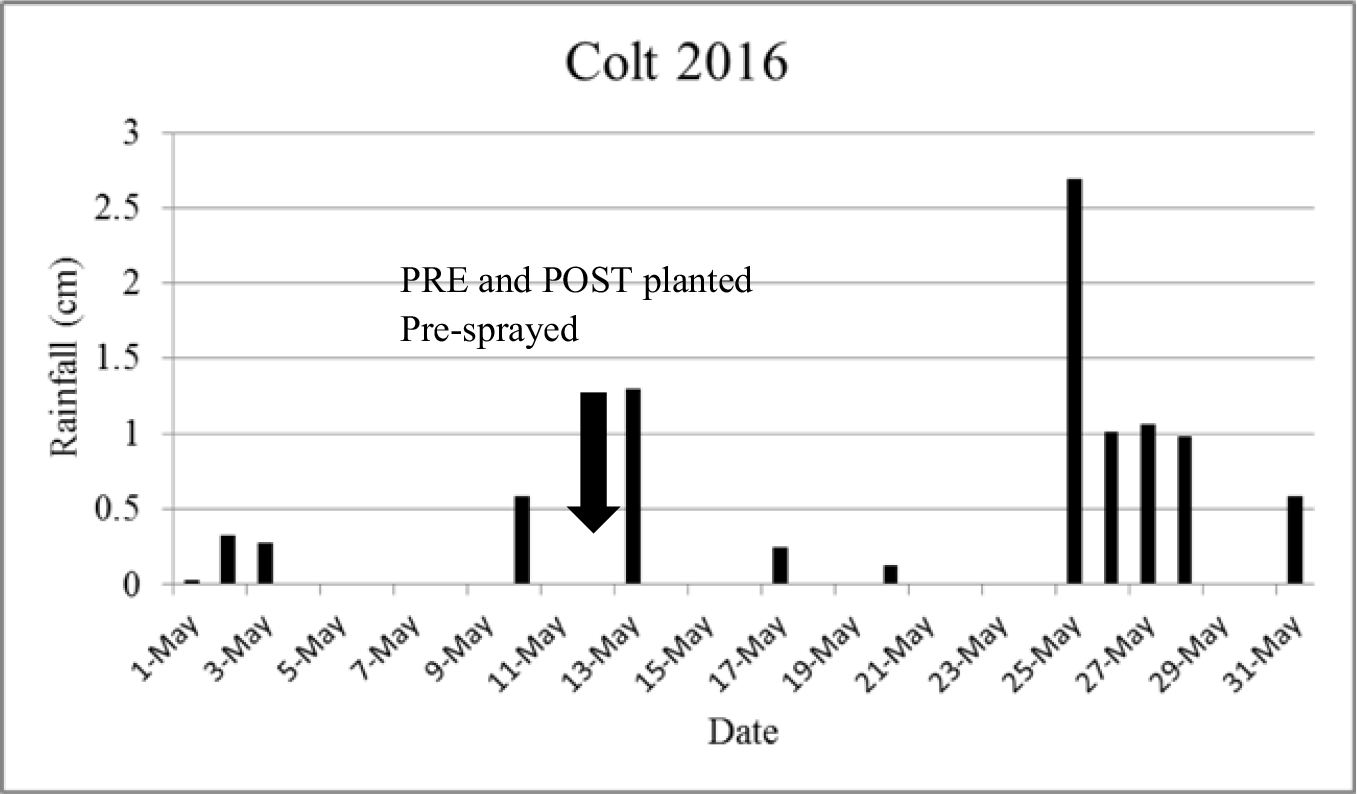
Figure 3. Planting/application timing and rainfall at Colt, AR (PTRS) in 2016.
Table 2. ANOVA for PRE acetolactate synthase–inhibiting herbicides to grain sorghum from 2016 and 2017.a–d

a Sensitivity experiment conducted near Colt, AR, in 2016.
b Sensitivity experiment conducted near Marianna, AR, in 2016 and 2017.
c Sensitivity experiment conducted in Fayetteville, AR, in 2016 and 2017.
d Abbreviations: DF, degrees of freedom; WAP, weeks after planting.
e *Denotes significance.
Table 3. Visible injury (%) from PRE applications of acetolactate synthase–inhibiting herbicides to conventional grain sorghum in 2016 and 2017.a–d
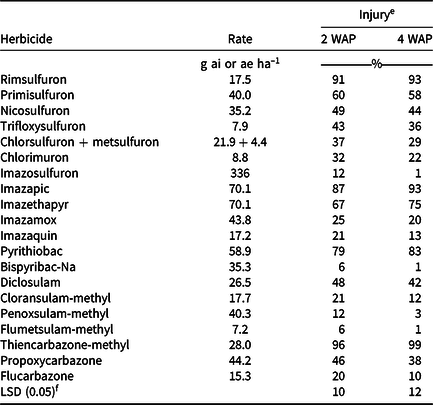
a Sensitivity experiment conducted near Colt, AR, in 2016.
b Sensitivity experiment conducted near Marianna, AR, in 2016 and 2017.
c Sensitivity experiment conducted in Fayetteville, AR, in 2016 and 2017.
d Abbreviations: WAP, weeks after planting.
e Visual injury ratings were conducted on a scale of 0 to 100%, with 0% being no injury and 100% denoting total plant death.
f Means within a column can be compared using Fisher’s protected LSD (α = 0.05).
By 4 WAP, a main effect of herbicide was found (P < 0.0001) for the response variable visible injury (Table 2). By 4 WAP, Inzen™ grain sorghum exhibited no signs of injury from any of the herbicides (data not shown). The conventional grain sorghum seemed to recover from some of the injury by 4 WAP, similar to published results from other researchers (Matocha and Jones Reference Matocha and Jones2015). The conventional technology was injured only 1% by bispyribac-Na, whereas 96% injury was caused by thiencarbazone-methyl (Table 3). Nicosulfuron at 4 WAP resulted in 44% injury, which was similar to injury seen from trifloxysulfuron, diclosulam, and propoxycarbazone. Both rimsulfuron and imazapic caused 93% injury, which was not different from thiencarbazone-methyl or pyrithiobac. Observation of injury with imazethapyr (75%) and pyrithiobac (83%) is essential, because there is the potential of herbicide carryover in a crop rotation with rice or cotton (Table 3); however, no injury was observed on Inzen™ grain sorghum. These data prove that Inzen™ grain sorghum can be implemented as a safe option in a rotation that follows ALS-inhibiting herbicide applications and permits potential for weed control.
A two-way interaction between herbicide and technology was observed for sorghum height 2 WAP (P = 0.0071) (Table 2). A reduction in sorghum height was found in all plots containing the conventional sorghum 2 WAP, with the exception of imazosulfuron, imazamox, bispyribac-Na, penoxsulam-methyl, and flumetsulam-methyl. There was no reduction in Inzen™ grain sorghum height at 2 WAP for any of the herbicides applied. Comparing Inzen™ and conventional grain sorghum within each herbicide, a sorghum height reduction in the conventional variety was found following all herbicides, except imazosulfuron, imazamox, bispyribac-Na, penoxsulam-methyl, and flumetsulam-methyl (Table 4). Sorghum height was influenced by the interaction of herbicide applied and technology planted 4 WAP (P = 0.0035) (Table 2). Plots containing the conventional grain sorghum, 4 WAP, had a reduction in sorghum heights ranging from 1% to 68%. The greatest sorghum height reductions in the conventional sorghum were observed when pyrithiobac, imazapic, thiencarbazone-methyl, and rimsulfuron were applied (Table 4). No height reductions were seen in plots containing Inzen™ grain sorghum.
Table 4. Grain sorghum heights at 2 and 4 wk following PRE acetolactate synthase–inhibiting herbicide applications in Fayetteville, AR, and near Marianna, AR, in 2017.

a Average sorghum height of Inzen™ grain sorghum in nontreated plots was 32 cm at 2 WAP and 40 cm in nontreated conventional plots.
b Whole-plot LSD for herbicide at 2 WAP was 24 and sub-plot LSD for seed technology planted was 24.
c Average sorghum height of Inzen™ grain sorghum in nontreated plots was 45 cm at 4 WAP and 54 cm in nontreated conventional plots.
d Whole-plot LSD for herbicide at 4 WAP was 16 and sub-plot LSD for seed technology planted was 20.
No reduction in relative yield was found in Inzen™ plots. Differences in relative yield were found in plots of the conventional grain sorghum (P < 0.0001) with all herbicides except trifloxysulfuron, imazosulfuron, propoxycarbazone, flucarbazone, penoxsulam-methyl, and flumetsulam-methyl (Tables 2 and 5). When comparing technologies, a difference between conventional and Inzen™ grain sorghum was found with 13 of the herbicides tested (Table 5). These results for Inzen™ grain sorghum are consistent with those of Werle et al. (Reference Werle, Jhala, Yerka, Dille and Lindquist2016), where the double point mutation of Val560Ile and Trp574Leu provided broad cross-resistance to ALS-inhibiting herbicides.
Table 5. Relative yield from 2017 of Inzen™ and conventional grain sorghum following PRE acetolactate synthase–inhibiting herbicide applications in Fayetteville, AR, and near Marianna, AR.

a Whole-plot LSD for herbicide was 8, and sub-plot LSD for seed technology planted was 12.
b Nontreated plots containing the Inzen™ technology yielded 8,480 kg ha–1.
c Nontreated plots containing the conventional technology yielded 7,828 kg ha–1.
d Means within the LSD are not significantly different according to Fisher’s protected LSD (α = 0.05).
POST Inzen™ Grain Sorghum Evaluation
No visible injury, sorghum height reduction, or yield loss occurred in the Inzen™ sorghum treated with any of the PRE-applied ALS-inhibiting herbicides evaluated. However, bispyribac-Na POST caused a 12% sorghum height reduction and 35% yield loss in Inzen™ sorghum, but this yield loss did not represent a statistically significant impact on the crop (Table 6). These results are consistent with the Regiment® (bispyribac-Na) label, which states that typical ALS injury may be observed as early as 3 to 7 d after application. Injury from bispyribac results in temporary yellowing and a reduction in sorghum height (Anonymous 2012). By 4 WAA, no visible injury was observed in plots treated with bispyribac-Na.
Table 6. ANOVA of Inzen™ grain sorghum from POST-applied acetolactate synthase–inhibiting herbicides in 2016 and 2017.a–d

a Sensitivity experiment conducted near Colt, AR, in 2016.
b Sensitivity experiment conducted near Marianna, AR, in 2016 and 2017.
c Sensitivity experiment conducted in Fayetteville, AR, in 2016 and 2017.
d Abbreviations: DF, degrees of freedom; WAA, weeks after application.
e *Denotes significance.
Grain sorghum heights were reduced 28% by bispyribac-Na 2 WAA, and 12% by 4 WAA. All other plots contained plants with similar heights to the nontreated (Table 7).
Table 7. Relative sorghum heights of Inzen™ grain sorghum to POST-applied acetolactate synthase–inhibiting herbicides in 2017 at Fayetteville, AR, and near Marianna, AR.a

a Abbreviations: WAA, weeks after application.
b Average sorghum height of nontreated plots was 82 cm at 2 WAA.
c Average sorghum height of nontreated plots was 93 cm at 4 WAA.
d Means within the LSD are not significantly different according to Fisher’s protected LSD (α = 0.05).
Grain yield was not affected by ALS-inhibiting herbicide when applied to Inzen™ grain sorghum (P = 0.6118) (Table 6). Cross-resistance among ALS-inhibiting herbicides to Inzen™ sorghum is consistent with previous research that documented resistance to both sulfonylurea and imidazolinone herbicides when the double gene mutation of Val560Ile and Trp574Leu was present (Werle et al Reference Werle, Begcy, Yerka, Mower, Dweikat, Jhala and Lindquist2017).
Lack of visible injury, and reduction of sorghum height or yield with Inzen™ grain sorghum can be attributed to the ALS double gene mutation of Val560Ile and Trp574Leu. Trp574 is the second most documented ALS gene mutation and has been identified in 41 weed species. The specific substitution of Trp574Leu is the most documented and accounts for 38 of the 41 Trp574 substitutions. In all 38 documented cases of Trp574Leu, resistance to two or more of the five ALS families was confirmed (Heap Reference Heap2020). Palmer amaranth, barnyardgrass, and johnsongrass account for 3 of these 38 cases and are among the top five most problematic weeds in many Arkansas row crops (Hernandez et al. Reference Hernandez, Leon, Fischer, Gebauer, Galdames and Flgurea2015; Molin et al. Reference Molin, Nandula, Wright and Bond2016; Panozzo et al. Reference Panozzo, Scarabel, Tranel and Sattin2013; Singh et al. Reference Singh, Salas-Perez, Bagavathiannan, Lawton-Rauh and Roma-Burgos2019).
Practical Implications
As many weed species can evolve resistance to a specific site of action, it is important to note that within WSSA Group 2, resistance to a specific ALS-inhibiting herbicide does not necessarily constitute resistance to all ALS-inhibiting herbicides. The broad cross-resistance to multiple ALS-inhibiting herbicides seen in Inzen™ could potentially allow for these products to be used within the crop for weed control, along with reducing current plant-back intervals for grain sorghum to WSSA Group 2 herbicides.
These results may prove beneficial when developing a herbicide program for grain sorghum, as there is potential to incorporate other herbicides into the program, depending on weed species present. By enabling use of the ALS site of action, Inzen™ will allow more options for herbicide diversification, further delaying the development of weed resistance. However, Werle et al. (Reference Werle, Jhala, Yerka, Dille and Lindquist2016) confirmed cross-resistance to nicosulfuron and imazethapyr in populations of johnsongrass present in Kansas, Missouri, and Nebraska. The possibility of ALS-resistant johnsongrass spreading further emphasizes the necessity of proper stewardship of the Inzen™ technology.
Acknowledgments
Funding for this research was provided by the Arkansas Corn and Grain Sorghum Board. No conflicts of interest have been declared.