Introduction
CVD, including CHD and stroke, are one of the leading causes of death globally. In 2017, the WHO reported that 18 million individuals had died from CVD worldwide which represents 31 % of deaths(Reference Mathers and Loncar1). Abnormally raised blood pressure, defined as greater than 140 (systolic)/90 (diastolic) mmHg, is an independent risk factor for CVDs and this silent killer is associated with a three-fold higher risk of having a stroke or developing heart failure(Reference Isaura, Chen and Yang2,Reference Weir, Juhasz and Puelles3) . High blood pressure affects more than one in four adults in England, about 12·5 million individuals. However, the prevalence of hypertension appears to differ between the sexes, with 31 % reported amongst men and 26 % amongst women(Reference Public Health4). Dysfunction of the endothelium, which controls vascular tone and is strongly associated with hypertension, is now recognised as an early, but potentially reversible, step in the development of CVD(Reference Bryan5).
The control of vascular function is known to be influenced by dietary factors, with nitrate-rich vegetables considered an important modulator(Reference Jones, Tong and Monsivais6–Reference Serra-Majem, Roman and Estruch8). This has been demonstrated in many observational and cohort studies which have shown consumption of nitrate- and nitrite-rich foods to significantly improve cardiovascular health(Reference Bhupathiraju, Wedick and Pan9) such as lowering blood pressure(Reference Bahadoran, Mirmiran and Kabir10) in both healthy(Reference Larsen, Ekblom and Sahlin11) and hypertensive individuals(Reference Broxterman, La Salle and Zhao12), reducing endothelial dysfunction(Reference D’El-Rei, Cunha and Trindade13–Reference Blekkenhorst, Bondonno and Liu17) and inflammation(Reference Khambata, Ghosh and Rathod18), protection from ischaemia–reperfusion injury(Reference Webb, Patel and Loukogeorgakis19), and improved exercise performance in patients with heart failure(Reference Eggebeen, Kim-Shapiro and Haykowsky20). A prospective cohort study has also concluded that an increased adherence to a diet high in nitrate is accompanied by a significant reduction in the risk of suffering both cardiovascular complications and death due to any cause(Reference Liu, Bondonno and Russell21). Clinically, nitrate supplementation or use of nitrate as a medication to increase the bioavailability of nitrite and NO can reduce blood pressure(Reference Gee and Ahluwalia22). The interest in using dietary nitrates as a treatment for lowering blood pressure is growing but mechanisms underlying the effects are unclear, which limits their current application as a dietary treatment for hypertension(Reference Gee and Ahluwalia22). Furthermore, there is some evidence to suggest that high dietary nitrate intakes are associated with negative effects on health, which has led to the development of the acceptable daily intake for nitrate of 3·7 mg/kg body weight per d and for nitrite of 0·07 mg/kg body weight per d(Reference Koutsoumanis, Allende and Alvarez-Ordóñez23). The acceptable daily intake for nitrate is based on the risk of methaemoglobinaemia commonly known as blue baby syndrome, which can occur following high nitrate intake in some babies, and can be fatal(Reference Knobeloch, Salna and Hogan24). In addition, some epidemiological studies have reported an association between dietary nitrite intake and colorectal cancer. However, the weight of evidence only supports a significant relationship between cancer and red and processed meat(25), with little known about vegetables and drinking water. The nitrate and nitrite within processed meat may be a contributing factor in the association with cancer, although this needs further confirmation.
Humans are naturally colonised by an array of micro-organisms, such as commensal or symbiotic communities, whose metabolic activity is important for host physiology and health. Commensal oral bacteria and those residing in the gastrointestinal tract play an important role in converting dietary nitrate to nitrite and the potent vasodilator NO(Reference Bryan, Tribble and Angelov26–Reference Al Khodor, Reichert and Shatat31). Up to 85 % of ingested nitrate is reduced to nitrite by the nitrate-reducing bacteria in the oral cavity(Reference Ma, Hu and Feng32), raising the salivary nitrite concentration to 1000 times that of plasma(Reference Hezel and Weitzberg28). A cohort study conducted in 281 volunteers found that the high abundance of nitrate-reducing bacteria was associated with blood pressure in normotensive individuals, although this association was not found in those with hypertension(Reference Goh, Trinh and Colombo33). To date, very little is known about the role of these oral bacteria in the control of vascular function, and the variation in composition that exists between individuals. The aim of this review is to present the current evidence on the potential role of dietary nitrate and the oral microbiome on vascular function including blood pressure and vascular tone. Important determinants of the number and composition of the oral bacteria between individuals will also be described. However, the impact of dietary nitrate interventions on vascular function only will not be specifically addressed in this instance due to the large number of review articles which already exist in this research area(Reference D’El-Rei, Cunha and Trindade13–Reference Blekkenhorst, Bondonno and Liu17). Before presentation of the methodology and results of the literature review, we provide a general overview of dietary nitrate sources, the pathways for the conversion of dietary nitrate and nitrite to NO, location and type of nitrate-reducing bacteria in the oral cavity and their potential role in regulating vascular tone.
Nitrate, nitrite and nitric oxide sources and nitric oxide pathway
NO, the most effective form of nitrate, was first recognised in 1998 as an important signalling molecule in the cardiovascular system(Reference Raju34). NO plays a significant role in virtually all organs in the body, and higher circulating concentrations are associated with a lower CVD risk(Reference Parthasarathy and Bryan35). In additional to the dietary (exogenous) sources of nitrate and nitrite which leads to the production of nitrite, and subsequently NO, via the oral bacteria, the body can also derive NO endogenously (Fig. 1). The endogenous pathway can occur in a number of different tissues in the body using three forms of NO synthase (NOS) enzyme: neuronal NOS (nNOS), endothelial NOS (eNOS) and inducible NOS (iNOS). eNOS was initially discovered in endothelial cells and is important in modulating vascular tone and upholding endothelial integrity. However, eNOS can also be expressed in various tissues and requires the presence of oxygen, Ca and calmodulin to be activated(Reference Xu, Huso and Dawson36). Within the endothelium, l-arginine undergoes a five-electron oxygen-dependent oxidation to produce NO and l-citrulline, catalysed by the synthase enzymes. Five cofactors required by the NOS enzymes are FAD, FMN, tetrahydrobiopterin (BH4), NADPH and haeme Fe(Reference Hezel and Weitzberg28). Once produced in the endothelial cell, NO rapidly diffuses to the underlying smooth muscle layer where it mediates blood vessel vasodilation. Any NO remaining in the circulation is rapidly converted to nitrate by oxyHb or superoxide before it enters the enterosalivary pathway. Therefore, the NO produced has a relatively short half-life in the order of seconds to minutes(Reference Kelm37).
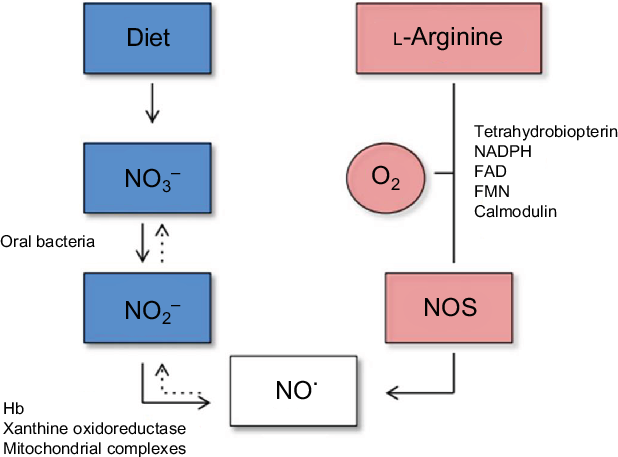
Fig. 1. Diagram of the endogenous generation of nitric oxide (NO) by NO synthase (NOS) (right panel), and exogenous generation of NO from the diet (left panel)(Reference Hezel and Weitzberg28). In biological fluids, NO is oxidised to nitrite (NO2) and nitrate (NO3) (dashed arrows). For a colour figure, see the online article.
Nitrate metabolism, enterosalivary circulation and gastrointestinal tract
High levels of inorganic nitrate are found in vegetables (such as beetroot and spinach) as well as drinking water, and these dietary sources accounts for 80 % of the daily intake. In contrast, the intake of dietary nitrite is very low, being approximately 100 times lower(Reference Hord, Tang and Bryan38) than that of nitrate(Reference Brkić, Bošnir and Bevardi39). Although the process of re-circulation of nitrates in the body has been known since the 1970s, the importance of the oral nitrate-reducing bacteria in the enterosalivary circulation has only recently been recognised(Reference Govoni, Jansson and Weitzberg27) (Fig. 2). The key role that these bacteria play in nitrate reduction was supported by a previous human study in which a significant correlation was found between high abundance of oral nitrate-reducing bacteria and nitrite level in saliva(Reference Burleigh, Liddle and Monaghan40). Nitrate secretion from the salivary glands leads to a 10-fold rise in salivary nitrate levels(Reference Kapil, Haydar and Pearl41) and this nitrate-enriched saliva appears to be a supportive environment for the growth of the oral bacteria particularly the nitrate-reducing bacteria on the tongue(Reference Doel, Benjamin and Hector42). These bacteria are mostly facultative anaerobes which use nitrate as an alternative electron acceptor for their respiration(Reference Hyde, Andrade and Vaksman43). A symbiotic relationship therefore exists between the oral commensal bacteria in which they receive nitrate from the host for their own respiration and in return produce nitrite required by the host(Reference Doel, Benjamin and Hector42). This relationship is particularly important for nitrite bioavailability since humans are unable to complete this process independent of the nitrate-reducing bacteria, with 80 % of nitrates swallowed and present in the stomach produced by the oral commensals(Reference Koch, Gladwin and Freeman44). Once in the stomach, contact with the gastric acidity leads to the protonation of nitrites to form nitrous acid (HNO2), which then decomposes into not only NO but also several other nitrogen oxides(Reference Iijima, Henry and Moriya45) which have localised benefits on maintaining the gastric mucosa layer(Reference Sobko, Huang and Midtvedt46) and enhancing mucosal blood flow(Reference Iijima, Henry and Moriya45) which increases the thickness of the mucosal layer(Reference Petersson, Phillipson and Jansson47). This process is referred to as non-enzymic conversion which does not require bacteria. However, the presence of Helicobacter pylori can contribute to a more acidic environment within the stomach and increase non-enzymic conversion(Reference Waldum, Kleveland and Sørdal48). Residual nitrates and nitrites are then absorbed in the small intestine with the half-life of circulating nitrate in the bloodstream of about 5–6 h(Reference Gilchrist, Shore and Benjamin49). In contrast, plasma nitrite concentrations start to increase within 15 min of nitrate ingestion and reach a peak level in 2 h(Reference Lundberg and Weitzberg50). A large proportion, approximately 70–75 % of the plasma nitrate, is excreted in the urine whereas the remaining 25 % is stored in the salivary gland and then recycled in the enterosalivary pathway(Reference Bos, Wedel and Hezel51).
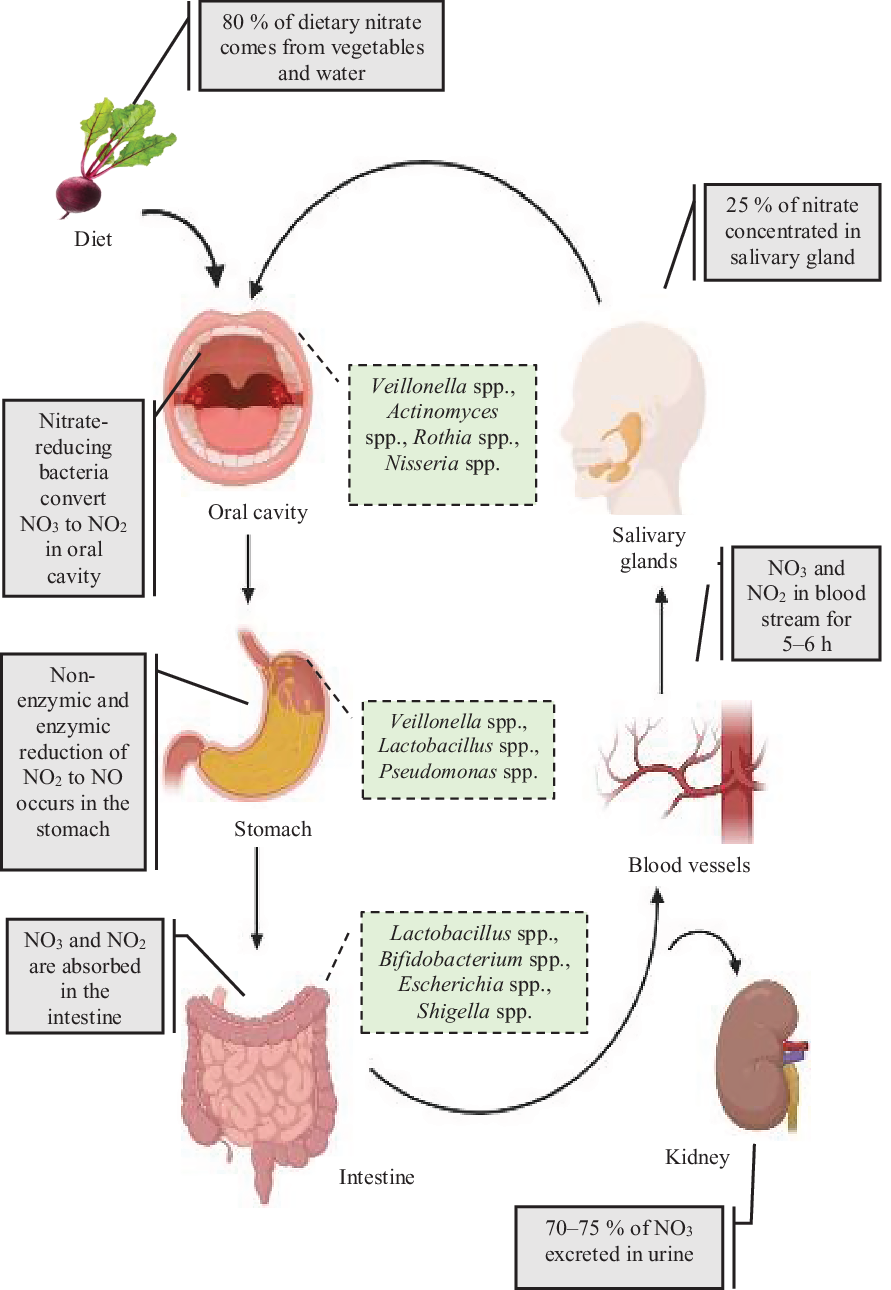
Fig. 2. Overview of the nitrate enterosalivary circulation and nitrate metabolism in humans. Ingested inorganic nitrate is converted to nitrite in the oral cavity by nitrate-reducing bacteria with reduction to nitric oxide (NO) and nitrogen oxides occurring within the acidic environment of the stomach. Remining nitrate and other nitrate components are then rapidly absorbed into the bloodstream via the small intestine. A large proportion of nitrate is then excreted by the kidneys into the urine, with up to 25 % being recycled by the salivary glands and then concentrated in saliva. For a colour figure, see the online article.
The role of the nitrate-reducing bacteria can persist past the oral cavity as most of these bacteria move into the stomach with both swallowed food and saliva. Limited studies have investigated the existence of these bacteria in the stomach and have confirmed that the gastric acidity is not a germ-free environment(Reference Lundberg52). Although the gastric pH is below 5, some bacteria species can tolerate the stomach acidity, with a culture-based study reporting Clostridium spp., Veillonella spp. and Lactobacillus spp. as the most predominant gastric species(Reference Petra, Rus and Dumitrașcu53), with Veillonella spp. identified as the most abundant nitrate-reducing bacteria(Reference Hyde, Andrade and Vaksman43). There are many factors that can influence gastric acidity such as inflammation and long-term use of proton pump inhibitors. The pH level has been found to have a positive impact on nitrate and nitrite concentration in the gastric juice. In a study conducted in ninety-nine patients with dyspepsia, results showed that when the pH level of the mucosal surface increased there was a comparable increase in both nitrate and nitrite concentrations. Another study conducted in participants with achlorhydria, in which gastric pH ranged from 6 to 8, reported three genera of nitrate-reducing bacteria (Streptococci, Neisseriae and Haemophili) to be responsible for the nitrite accumulation in the gastric secretions(Reference Forsythe, Dolbyt and Websters54).
The small intestine and colon contain many different species of bacteria including both facultative and obligate anaerobes which are involved in the bioconversion of nitrite to NO, although they are not necessarily the same as the nitrate-reducing bacteria found in the oral cavity(Reference Tiso and Schechter55). A study conducted in germ-free and normal rats has shown that NO can be produced by the bacteria resident in the small intestine of normal rats, but not in germ-free rats(Reference Sobko, Reinders and Norin56). Furthermore, two studies have identified lactobacilli, bifidobacteria(Reference Sobko, Reinders and Norin56), Escherichia coli and Shigella as the predominant nitrate-reducing bacteria in the large intestine(Reference Parham and Gibson57). However, an in vitro study which used pure strains of gut bacteria incubated in agar media with nitrate then nitrite, found that in the presence of nitrite, both bifidobacteria and lactobacilli generated large amounts of NO, up to 5000 parts per billion (ppb), but only approximately 35 ppb of nitrate(Reference Sobko, Reinders and Jansson58). Interestingly, Sobko et al. (Reference Sobko, Huang and Midtvedt46) reported that the NO formed was being utilised by E. coli and Staphylococcus aureus. These authors speculated that these gut bacteria may consume NO in order to help adapt to their environment in this in vitro experiment. Therefore, it appears that the presence of NO and other nitrate metabolites in the large intestine may be dependent on the relevant abundance of these bacteria species and their production and utilisation of NO(Reference Briskey, Tucker and Johnson59). Localised effects of the NO could include altering blood flow which could potentially increase the uptake of nitrate and nitrite in the proximal small intestine where the majority are absorbed(Reference Koch, Gladwin and Freeman44). However, the NO level in the gastrointestinal tract could also be influenced by other factors such as pH level, inflammation, oxygen tension and the level of dietary nitrate intake of an individual. Further studies are needed to determine the direct effects of nitrate and nitrite on gut bacteria composition and nitrate metabolism.
Bacterial nitrate reduction in the oral cavity, composition and location
A continuous flow of saliva, specialised mucosal surfaces and teeth in the human oral cavity provide a unique microbial habitat for bacteria. Most of these bacteria are found on the dorsum (surface) of the tongue and around the teeth where a wash of 1 ml of saliva can contain up to 107–108 micro-organisms(Reference Koch, Gladwin and Freeman44). However, only 700 species have currently been identified(Reference Koch, Gladwin and Freeman44). The majority of these bacteria shelter in the gingival crevices between teeth which represent a conducive anaerobic environment. Here, the gingival crevicular fluid bathes the bacteria within a nutritionally rich medium supporting their proliferation(Reference Marsh, Head and Devine60). In contrast, the smoother surfaces of teeth have much lower levels of bacteria due to the forces that act on these areas during eating and drinking. However, the nitrate-reducing bacteria are found predominately on the rear dorsum of the tongue, with a higher proportion of Gram-negative bacteria found within the papillae of the tongue compared with the surface. Some studies have identified the genus and species of these bacteria that can produce nitrate reductases and nitrite reductases that aid in the production of NO. These include: Veillonella atypica, V. dispar, Actinomyces eslumdii, A. odontolyticus, Staphylococcus epidermids, Neisseria flarescens, Haemophilus, Porphyromonas, Rothia mucilaginosa, R. dentocarisa, Prevotella and Leptotrichia (Reference Doel, Benjamin and Hector42,Reference Hyde, Andrade and Vaksman43) . The two major groups of oral nitrate-reducing bacteria are the strict anaerobes such as V. atypica and V. dispar and the facultative anaerobes such as A. odontolyticus and R. mucilaginosa (Reference Doel, Benjamin and Hector42) (see Table 1). Facultative anaerobes are mostly prevalent on the surface of the tongue, with a study stratifying participants according to oral nitrate-reduction capacity observing a higher abundance of Streptococcus, Granulicatella, Prevotella, Neisseria and Haemophilus on the posterior surface of the tongue compared with Actinomyces (Reference Hyde, Andrade and Vaksman43). Interestingly, although lower in prevalence, Actinomyces have been reported to be more efficient reducers of dietary nitrates under anaerobic conditions.
Table 1. Commonly reported nitrate-reducing bacteria species found in the oral cavity

↑, Increased; ↑↑, highly increased.
Mechanisms by which bacteria may convert nitrate to nitrite
The three mechanisms through which nitrates are converted to nitrites and other components by bacteria are denitrification, assimilation and dissimilation. The first process, denitrification, occurs in the oral cavity under aerobic conditions(Reference Ji, Yang and Zhu61) and is also called the respiratory nitrate reduction process. During microbial respiration, oxygen is replaced by nitrogen oxides as terminal electron acceptors and ultimately reduces nitrate to nitrous oxide or free nitrogen(Reference Schreiber, Stief and Gieseke62). Most of the bacteria which have genes for respiratory nitrate reductases (nirS and nirK) prefer aerobic conditions(Reference Takaya, Catalan-Sakairi and Sakaguchi63) such as Rothia spp. and Neisseria spp. However, some denitrification species of bacteria also reside in anaerobic conditions(Reference Koch, Gladwin and Freeman44), such as Veillonella. The specialised surface of the tongue dorsum therefore represents a microaerophilic environment which allows denitrification to occur under both aerobic and anaerobic conditions. In the oral cavity, nitrite (NO2) is initially formed from salivary nitrate (NO3) by some oral bacteria such as Actinomyces (Reference Hyde, Andrade and Vaksman43) that are considered to possess the nitrate reductase enzyme (nar) and further converts nitrite to NO through either enzymic (nir) or non-enzymic denitrification. The latter process is a well-established step in the gastric environment of the stomach. NO is then converted to nitrous oxide (N2O) by NO reductase (nor) and finally to nitrogen (N2) by nitrous oxide reductase (nos). The nitrogen oxides and enzymes that participate in the process of denitrification are as follows:

In the second pathway known as dissimilation, nitrate is reduced to ammonia (NH4 +) by periplasmic nitrate reductase (nap), with the intermediate product being nitrite(Reference Sparacino-Watkins and Stolz64). This two-step process is strictly anaerobic and occurs in the human gut by the facultative anaerobes(Reference Tiso and Schechter55):

Assimilation, which occurs predominantly in plants, water and soil(Reference Herrero, Flores, Imperial, Schmidt, Cambridge, Press and Elsevier65), is the third pathway. Similar to denitrification, the conversion of nitrate to ammonia occurs, but, during this pathway, the enzyme cytoplasmic nitrate reductase (nas) is used(Reference Herrero, Flores, Imperial, Schmidt, Cambridge, Press and Elsevier65). In this biosynthetic anabolic pathway, nitrite is further reduced to ammonia, which can then undergo ammonium assimilation by incorporating the amino acid glutamine(Reference Koch, Gladwin and Freeman44). The assimilation and dissimilation processes are therefore important in the utilisation of nitrates. Nitrifying bacteria (including Nitrobacter, Nitrococcus and Nitrosomonas)(Reference Dodsworth, Hungate and Hedlund66) are responsible for the dissimilation and ammonification of nitrates and oxidise ammonium salts and nitrites to nitrates in a process called nitrification. It has been hypothesised that this process might happen in the gut, but, to date, this has not been described(Reference Tomasova, Konopelski and Ufnal67).
In humans, nitrate reduction seems to occur either directly, such as in assimilatory nitrate reduction, or during a series of reactions during respiratory nitrate reduction. Notably, the latter process needs more than one enzyme for further reduction which is mediated by the bacterial communities(Reference Koch, Gladwin and Freeman44). This suggests that nitrate-reducing capacity of nitrate-reducing bacteria is related to the bacterial species, cellular location of enzymes and environmental conditions such as oxygen level. Therefore, dissimulation would occur more in the gut and denitrification in the oral cavity(Reference Tomasova, Konopelski and Ufnal67). Although the role of oral bacteria in mediating the beneficial effect of nitrate on vascular function is poorly understood, this review aims to address this knowledge gap by focusing on studies that used antibacterial mouthwash and toothpaste to determine the importance of the presence of oral microbiome on blood pressure and vascular tone.
Methods
A systematic approach was used to identify the relevant human and animal studies which investigated the role of dietary nitrate and the oral microbiome on blood pressure. PubMed and Web of Science were used for the literature search which included all relevant articles published in English from January 1950 until September 2019. There were three stages in the selection process. The combinations of the key terms used in the search strategy were as follows: (‘Nitrate’ OR ‘Nitrite’ OR ‘Nitric Oxide’) AND (‘Oral Bacteria’ OR ‘Oral Microbiom’ OR ‘Nitrate-Reducing Bacteria’) AND (‘Blood Pressure’ OR ‘Hypertension’ OR ‘Cardiovascular’ OR ‘Vascular Function’) AND (‘Mouth Wash’ OR ‘Antiseptic’ OR ‘Antibacterial’). The titles and abstracts of the identified papers were screened by one member of the review team (H. S. A.) who identified potentially relevant papers. This review was restricted to animal studies and human studies which used antibacterial mouthwash or toothpaste to determine the effects on oral nitrate reduction on blood pressure and vascular tone. Only published peer-reviewed literature was considered and ‘grey’ literature such as dissertations, conference proceedings, reports, letters to editors and other non-peer-reviewed research was excluded. After duplicates were removed, the abstract and full papers were screened for eligibility. In addition, a hand-search of the bibliographies of the articles found from the electronic database searches was also conducted. An overview of the literature search is shown in Fig. 3.

Fig. 3. Flow of information through the different phases of the literature review.
The quality of the included randomised controlled trials in human subjects and animal studies were assessed for the risk of bias using the Cochrane risk of bias tool(Reference Higgins, Sterne and Savović68) for human studies and SYRCLE’s tool(Reference Hooijmans, Rovers and De Vries69) for animal studies.
Results and discussion
The systematic search identified 160 publications. Of these, twelve relevant publications were included, with nine describing studies conducted in human subjects and three in animals. The risk of bias assessment summaries for each study are presented in online Supplementary Tables 1 and 2, respectively. Animal studies will be discussed before studies including human participants. This will be followed by discussion of the non-modifiable and modifiable factors affecting inter-individual variability in number and composition of oral bacteria, with potential mechanisms of action.
Animal studies
Of the fourteen animal studies which have investigated the effect of nitrate on blood pressure, only three studies have determined whether oral bacteria are important in mediating the improvements in blood pressure and endothelial function (Table 2). Formation of bioactive NO takes place within the gastric environment of the stomach as a result of the enterosalivary circulation of nitrate, as well as systemically in the blood vessels. In 2009, Petersson et al. (Reference Petersson, Carlström and Schreiber70) reported daily mouthwash treatment for 7 d in rats to attenuate both the gastroprotection provided by NO and the diastolic blood pressure-lowering effect of sodium nitrate. A similar pattern was also evident for the mean arterial pressure in the rats treated with mouthwash and nitrate, but the lack of an effect in the rats treated with mouthwash and nitrite suggested that oral bacteria play an important role in the metabolism of nitrate to NO and mediated vasodilation. Furthermore, these rats also had reduced oral bacteria, suggesting that nitrite could bypass the reduction step by the oral bacteria and was being reduced in the circulation or within endothelial cells to NO, or via effects on the formation of the intermediate nitrosothiols(Reference Petersson, Carlström and Schreiber70). However, dietary nitrite intake is generally lower than that of nitrate, and the half-life in plasma shorter (seconds v. hours), which suggests that even if nitrite directly stimulates NO signalling, the quantity and kinetics of nitrite v. nitrate indicate that the critical aspect of this mechanism is the reduction of nitrate. Therefore, the role that dietary nitrite plays in blood pressure lowering may be more limited relative to nitrate.
Table 2. Animal studies investigating the importance of oral nitrate-reducing bacteria on blood pressure in response to nitrate intake
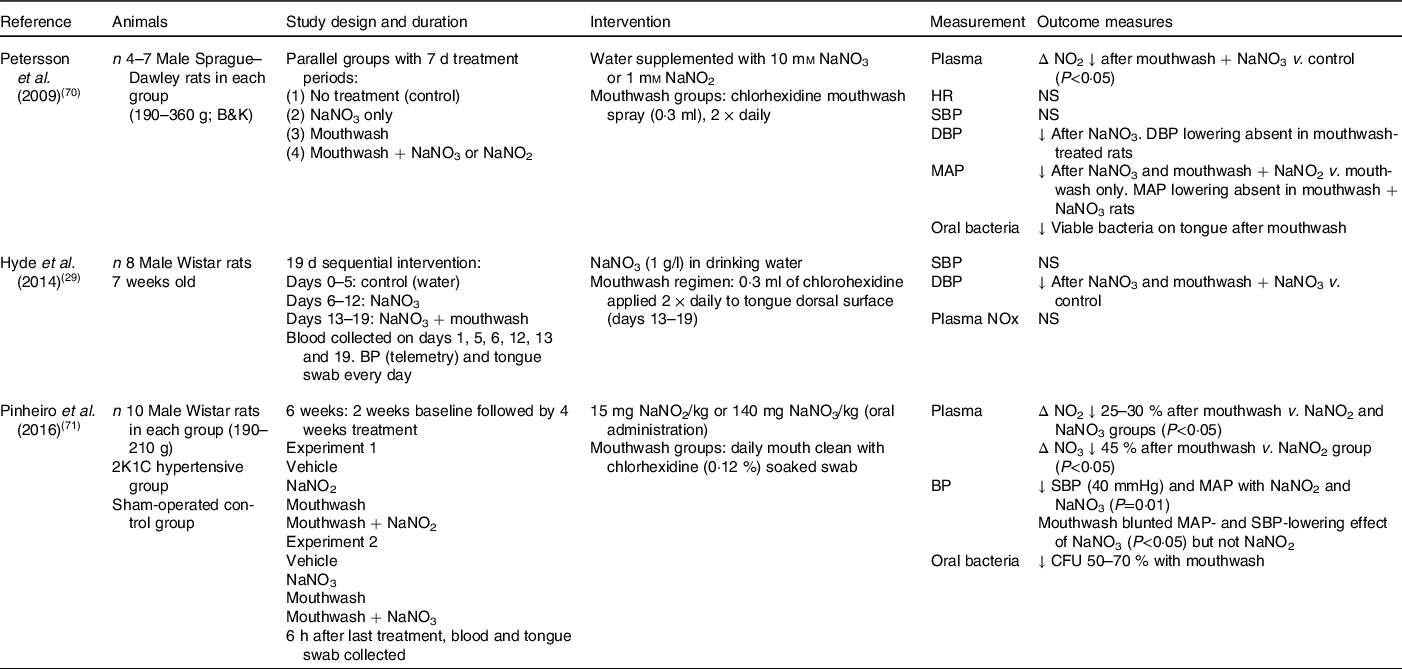
NO2, nitrite concentration; HR, heart rate; SBP, systolic blood pressure; DBP, diastolic blood pressure; MAP, mean arterial pressure; BP, blood pressure; NOx, sum of nitrate and nitrite; 2K1C, 2 kidney, 1 clip; NO3, nitrate concentration; CFU, colony-forming units (number of viable bacteria).
In agreement, Hyde et al. (Reference Hyde, Luk and Tribble29) also reported a significant reduction in diastolic blood pressure and increase in plasma nitrite concentrations following the addition of sodium nitrate to drinking water in male Wistar rats. However, in this study, mouthwash treatment was unable to diminish the blood pressure-lowering effects of the nitrate supplementation. The authors speculated that the direct application of the chlorhexidine-based mouthwash (Vedco) to the tongue surface using a swab might not have enabled sufficient time for the mouthwash to exert its full extent on the bacteria relative to mouthspray(Reference Hyde, Luk and Tribble29). A novel aspect of this longer-term supplementation study was the focus on the changes in the microbiota composition on the rat tongue in response to the treatments. Compared with baseline, there was a greater relative abundance of nitrate-reducing bacteria (Haemophilus spp. and Steptococcus spp.) after 6 d of sodium nitrate consumption, and of these Haemophilus parainfluenzae has also been identified as one of fourteen species contributing to nitrate reduction in the oral cavity of healthy adults. Co-supplementation of mouthwash with nitrate was found to increase the diversity of the oral bacteria present relative to nitrate intake only, with increases found in the low abundance taxa such as Enterobacteriaceae, Corynebacterium and Morganella. Therefore, the use of mouthwash appeared to disturb the oral microbiome by reducing the abundance of the normally dominant taxa but not completely to impact nitrate reduction. These findings suggest that the lower abundance taxa which were evident after mouthwash treatment may be functionally important in the bioactivation of dietary nitrate. However, the authors did caution against translating these findings on the oral bacteria composition to humans since the oral human microbiome has been shown to be more diverse and of a differing composition compared with the rat(Reference Hyde, Luk and Tribble29).
The impact of mouthwash on chronic changes in blood pressure in response to nitrate or nitrite supplementation was further examined by Pinheiro et al. (Reference Pinheiro, Ferreira and Amaral71) in both control and hypertensive rats. After 4 weeks, significant reductions in mean arterial pressure and systolic blood pressure were evident in both the nitrate and nitrite groups, with concordant increases found in circulating plasma nitrate and nitrite levels. Interestingly, co-supplementation with mouthwash attenuated the rise in plasma nitrite levels by 25–30 % in both groups but was only found to blunt the blood pressure-lowering effect of nitrate, with little impact found on blood pressure in the mouthwash and nitrite group. In agreement with Petersson et al. (Reference Petersson, Carlström and Schreiber70), these findings suggested that anti-hypertensive effects of nitrite were potentially occurring via non-enzymic reactions within the gastric environment after swallowing this ion independently of the enterosalivary pathway. Analysis of the endogenously produced vasodilatory compound S-nitrosothiol and levels of vascular nitrosylation revealed mouthwash to reduce nitrosylation responses to nitrate only, leading the authors to speculate that S-nitrosylation was an important mediator of the blood pressure-lowering effects of both nitrate and nitrite(Reference Petersson, Carlström and Schreiber70,Reference Pinheiro, Ferreira and Amaral71) . Studies have also reported that the foods consumed with dietary nitrites, such as conjugated fatty acids, are also a target of nitrating species in the stomach leading to the formation of nitro-fatty acids (such as nitro-conjugated linoleic acid). These electrophiles have been shown to have anti-hypertensive effects independent of S-nitrosothiols, suggesting that they may also play a role in mediating the effects of nitrate and nitrite on blood pressure(Reference Delmastro-Greenwood, Hughan and Vitturi72). Antiseptic mouthwash was proposed to attenuate the beneficial effects of dietary nitrate intake on blood pressure by reducing the amount of nitrite formation by the oral bacteria and therefore reaching the stomach, inhibiting gastric formation of S-nitrosothiols. However, the positive benefits on blood pressure of raised S-nitrosothiols was only found in the hypertensive rats, supporting previous observations in both animals and human subjects that raised blood pressures often show a greater sensitivity to the anti-hypertensive effects of medication and/or dietary modification.
Studies performed in animals may provide useful insights into the mechanisms underlying the effects of oral bacteria in the bioactivation of nitrate. However, findings in rats and mice need to be interpreted with caution due to differences in physiology and dependence on nitrate as a source of NO between organisms. In contrast to human subjects, rats and mice do not recirculate nitrate in saliva(Reference Montenegro, Sundqvist and Nihlén73) and so salivary nitrate concentrations never exceed those levels found in plasma(Reference Ahmed, Nichols and Honavar74) and they also have other nitrate-reducing mechanisms that may work in tandem with nitrate reduction by the oral bacteria to control nitrite and NO level(Reference Montenegro, Sundqvist and Nihlén73).
Human studies
The publications describing the human studies were divided into those which examined (1) the association between oral bacteria with nitrate/nitrite levels and/or blood pressure (n 5; Table 3) and (2) the combined effects of nitrate ingestion and oral bacteria on nitrate/nitrite levels and/or blood pressure (n 4; Table 4). The role of the oral bacteria in mediating systemic nitrite production after nitrate intake has been primarily investigated with the use of an antiseptic mouthwash to remove the bacteria before the measurement of the outcomes of interest. The type of mouthwash has been shown to be important, with the strong antibacterial chlorhexidine-based mouthwash (Corsodyl) found to be more effective at reducing V. dispar (nitrate-reducing bacteria) in the oral cavity than Listerine (mixture of essential oils), Isodine and Cepacol (antibacterial) in healthy adults(Reference Mitsui and Harasawa75). In support of these findings, gargling with 10 ml of chlorohexidine mouthwash (Corsodyl) twice for 1 min was also found to reduce the bacterial count of nitrate-reducing bacteria by approximately 80 % and virtually abolish the oral nitrate-reducing capacity compared with no mouthwash in healthy subjects(Reference Govoni, Jansson and Weitzberg27). Although nitrate accumulated in saliva after ingestion of sodium nitrate in both studies, a significant reduction in the conversion of salivary nitrate to nitrite after mouthwash was associated with 30 % lower plasma nitrate concentrations at 3 h post-ingestion, compared with no prior use of mouthwash. In contrast, a randomised cross-over study found an antibacterial toothpaste to have no effect on salivary or plasma nitrate concentrations in sixteen women after consuming 400 mg of nitrate before brushing their teeth with antibacterial toothpaste (0·3 % triclosan) or toothpaste containing no antibacterial agent(Reference Bondonno, Croft and Puddey76). The lack of an effect observed with the antibacterial toothpaste may reflect either the lower prevalence of the nitrate-reducing bacteria on the surface of the teeth, relative to the tongue, or the less efficient removal of the bacteria sheltering within the gingival crevices between the teeth compared with mouthwash.
Table 3. Human studies determining the effects of oral bacteria on salivary and plasma nitrite concentrations, and/or blood pressure in response to nitrate intake
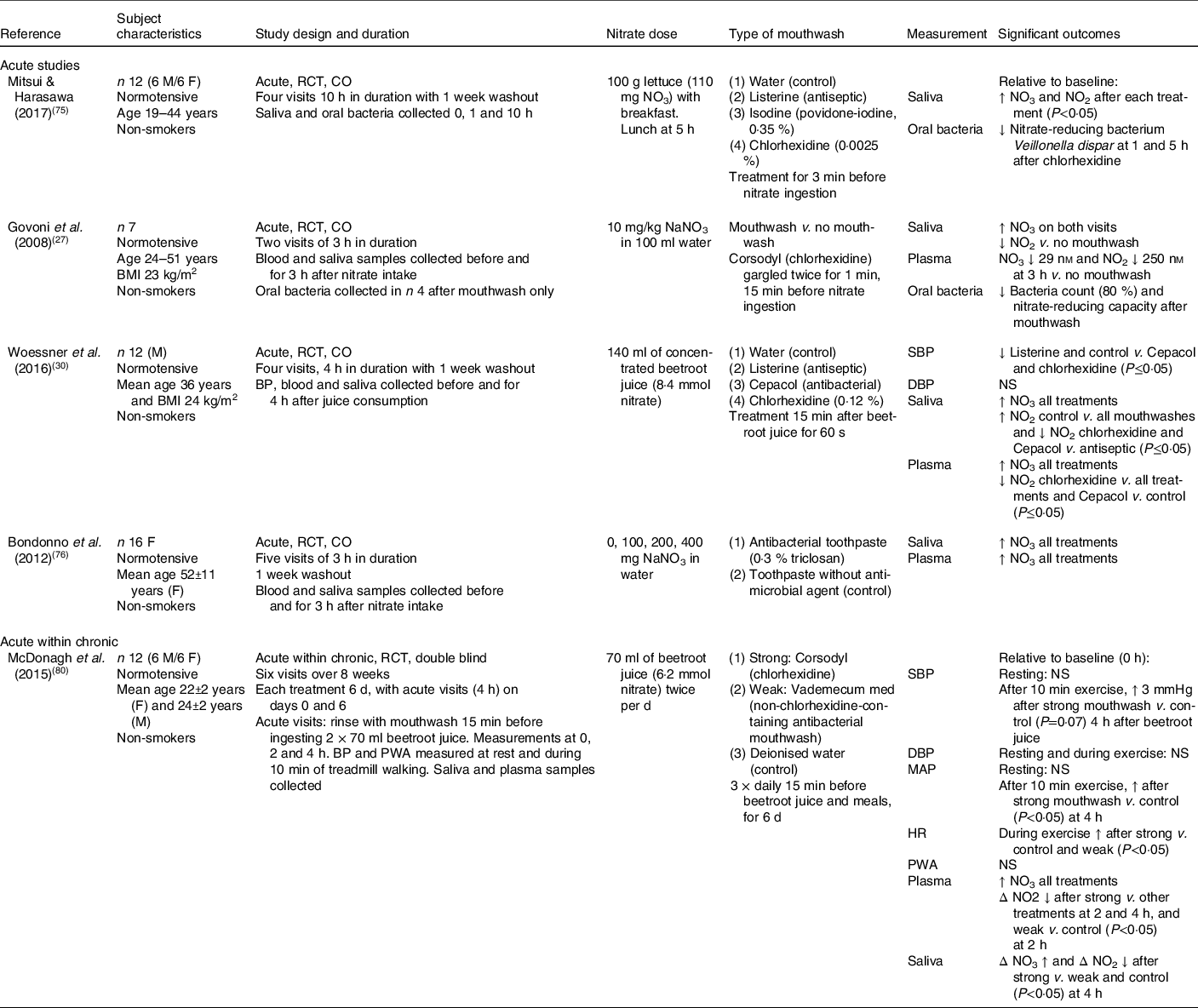
M, male; F, female; RCT, randomised controlled trial; CO, cross-over; NO3, nitrate concentration; SBP, systolic blood pressure; DBP, diastolic blood pressure; BP, blood pressure; PWA, pulse wave analysis; MAP, mean arterial pressure; HR, heart rate.
Table 4. Chronic human studies investigating the involvement of oral bacteria in the blood pressure-lowering effect of nitrate

F, female; M, male; BP, blood pressure; SBP, systolic blood pressure; DBP, diastolic blood pressure; NO3, nitrate concentration; NO2, nitrite concentration; RCT, randomised controlled trial; CO, cross-over; ABP, arterial blood pressure; A-SBP, ambulatory SBP; A-DBP, ambulatory DBP; HR, heart rate.
Four studies have determined the impact of mouthwash on changes in oral nitrate-reducing capacity and blood pressure (Table 4). Compared with no mouthwash, Kapil et al. (Reference Kapil, Haydar and Pearl41) reported that using 0·2 % chlorhexidine twice daily for 7 d significantly increased systolic and diastolic blood pressure measured using three different techniques (clinic, ambulatory and home measurements) by approximately 3 and 2 mmHg, respectively, in nineteen healthy normotensive subjects. Interestingly, the effects of mouthwash treatment on blood pressure were evident after only a single use of the chlorhexidine mouthwash and was maintained for the following 6 d. The rise in blood pressure was significantly correlated with the significant reduction in plasma nitrite levels, with only a trend for a relationship with the salivary nitrite, highlighting the potential importance of the oral nitrate-reducing bacteria in blood pressure modulation.
In fifteen subjects treated with anti-hypertensive medication, the attenuation found in oral nitrate-reducing capacity after daily use of chlorhexidine mouthwash for 3 d was associated with an increase in systolic blood pressure of 2·3 mmHg, but only a trend for a decrease in plasma nitrite concentrations compared with the control (tap water)(Reference Bondonno, Liu and Croft77). The lack of a significant effect on the plasma nitrite response relative to Kapil et al. (Reference Kapil, Haydar and Pearl41) was thought to be due to the study visit being performed 12 h after prior use of the mouthwash treatment or related to the age or medication use of the hypertensive participants. In order to determine the mechanism underlying the effects of dietary nitrate intake on blood pressure, plasma cGMP (cyclic guanosine monophosphate), a mediator of NO-dependent smooth muscle relaxation in the endothelium and a good marker of NO production, can be measured. Although increases in plasma nitrite and cGMP after dietary nitrate intake have been previously associated with blood pressure lowering, no effects were evident on cGMP concentrations after 3 d of using mouthwash. This may be related to the lack of a nitrate challenge on the study visit (which provides an important source of NO under hypoxic conditions) but could also suggest that dietary nitrate may have an impact on vascular tone via direct effects on smooth muscle function.
In contrast to these two studies, Tribble et al. (Reference Tribble, Angelov and Weltman78) reported use of chlorhexidine mouthwash twice daily for 7 d to be associated with a highly variable effect on clinic systolic blood pressure (an increase of at least 5 mmHg found in nine subjects whereas a decrease was observed in four subjects) in an orally healthy cohort. Post hoc data analysis revealed the inclusion of tongue cleaning as part of the daily dental hygiene routine to play a significant role in the responses observed both on blood pressure and the diversity of the oral bacteria at baseline and during the study. Specifically, regular tongue cleaning was associated with a greater ability to reduce nitrite to NO whereas the lack of tongue cleaning resulted in an oral microbiome composition which favoured conversion of nitrite to ammonia and not NO. The authors speculated the use of chlorhexidine mouthwash was having a chemo-stimulatory effect on the oral bacteria, with the temporary loss of bacterial numbers proposed to stimulate a rapid population recovery and increase in bacterial nitrate reductase activity. However, these effects may also reflect a protective up-regulation of the nitrate-, nitrite- and NO-regulating mechanisms in the microbiota suddenly detached from their biofilms during tongue cleaning and warrants further investigation.
In a cross-over study, treatment with chlorhexidine (0·2 %) for 3 d was shown to have no effect on clinic or 24 h ambulatory blood pressure in seventeen young females compared with a placebo mouthwash(Reference Sundqvist, Lundberg and Weitzberg79). Although a reduction in salivary nitrite- and oral nitrate-reducing capacity was found after the antibacterial mouthwash, comparable changes were not evident in either the plasma or urine samples collected. The lack of effects observed relative to other studies may reflect the short intervention time with the mouthwash treatments or inclusion of female participants only. Based on a previous study conducted by the same research group in athletes, they speculated that cross-talk may exist between the enterosalivary nitrate–nitrite–NO pathway and eNOS, with a greater intake of dietary nitrate associated with a lower eNOS activity. However, whether a reduction in nitrate–nitrite–NO with antibacterial mouthwash leads to an up-regulation in eNOS is yet to be established.
In the studies presented in Table 3, measures of blood pressure have been related to salivary and plasma nitrate/nitrite levels following nitrate intake and use of mouthwash. In agreement with previous findings, Woessner et al. (Reference Woessner, Smoliga and Tarzia30) found antibacterial mouthwashes to attenuate postprandial salivary and plasma nitrite concentrations following dietary nitrate intake (concentrated beetroot juice) compared with the weaker antiseptic mouthwash and control. Although changes in clinic systolic blood pressure 0–3 h after the treatments were not related to plasma/salivary nitrite or nitrate levels, systolic blood pressure at 4 h was 2–5 mmHg higher after chlorhexidine and Cepacol mouthwashes compared with control and Listerine mouthwash. These findings potentially suggest an important role of the nitrate–nitrite–NO enterosalivary pathway, but should be interpreted with caution due to the small sample size, inclusion of male subjects only and the short duration of the study visit relative to the expected peak in plasma nitrite concentrations (approximately 3 h). Furthermore, these findings may have been influenced by the large inter-individual variability observed in blood pressure responses following the mouthwash treatments.
In the study of McDonagh et al. (Reference McDonagh, Wylie and Winyard80), consumption of 2 × 70 ml shots of concentrated beetroot juice and daily use of strong or weak antibacterial mouthwash for 6 d were found to have limited effects on baseline blood pressure and salivary and plasma nitrate/nitrite levels compared with the control (water). However, differences were evident 2–4 h after drinking the beetroot juice, with the rise in plasma nitrite found to be attenuated after use of the strong and weak mouthwash for 6 d. These changes were associated with a reduced oral nitrate-reducing capacity after the strong mouthwash, with lower nitrite levels compared with both the weak and placebo mouthwashes. Although changes in resting measures of blood pressure (supine and seated) and pulse wave analysis (arterial stiffness) after the juice were not influenced by the strength of the mouthwash used, differences were evident in blood pressure during low-intensity activity on the treadmill. In particular, there was a greater increase in systolic blood pressure and mean arterial pressure after rinsing with the strong (chlorhexidine) compared with the control (water) mouthwash. The lack of effect on arterial stiffness even in the presence of lower salivary and plasma nitrite levels after the strong mouthwash indicates that either the availability of NO was not altered sufficiently over the 4 h acute test period in these young active participants or that their higher physical active level may have masked any effects of the mouthwash on the vascular function measures. However, this is one of the only studies to incorporate a measure of blood vessel elasticity to determine the role of oral bacteria in mediating the beneficial effects of beetroot juice on vascular function, and so further studies are needed in which to compare these findings and determine the underlying mechanisms.
As highlighted in the human studies, oral bacteria composition appears to vary between individuals, with both non-modifiable (for example, age, sex, genetics and tongue physiology) and modifiable (for example, diet, health conditions, lifestyle and dental hygiene routine) factors considered to have an impact on the abundance and prevalence of nitrate-reducing bacteria in the oral cavity. These factors are important to consider during interpretation of the study findings and for informing the design of future studies exploring the role of oral nitrate-reducing bacteria on the regulation of vascular function. The following section summarises the main factors identified from the human studies.
Inter-individual variability in number and composition of oral bacteria: non-modifiable factors
Geographical location and culture have both been suggested to have an impact on oral bacteria composition. Findings from a study including participants from Northern and Southern Europe reported a higher abundance of Rothia and unclassified Gemellaceae in Finnish populations compared with Spanish while Lactococcus, Fusobacterium and Porphyromonas genera were significantly higher in Spanish compared with Finnish groups(Reference Sandell and Collado81). Comparing findings of this study with another study which investigated the differences in oral bacteria between individuals living in Africa, Alaska and Germany showed that oral bacteria composition is highly variable between countries(Reference Li, Li and Rzhetskaya82). These differences may represent the sex and age distributions of these different populations, genetic make-up and habitual food preferences(Reference Li, Li and Rzhetskaya82,Reference Hansen, Kern and Bak83) .
Moreover, the dorsal surface of the tongue plays a major role in nitrate reduction and represents a highly papillated surface area. The papillary structure of the human tongue is unique in nature and supports a higher bacterial density than the mucosal surface, accumulating oral debris and anaerobic bacteria on the rear of tongue(Reference Doel, Benjamin and Hector42). There are three kinds of papillae on the tongue: fungiform, circumvallate and foliate papillae. The fungiform papillae have a mushroom shape and are found predominately on the dorsal surface of the tongue covering up to two-thirds of the surface. Their shape supports a higher bacterial density(Reference Eldeghaidy, Thomas and Skinner84). However, the shape and number of papillae varies between individuals which has been related to differences in oral bacteria composition. Studies have shown that a number of factors can affect the papillary number on the tongue including ageing (with lower number of papillae observed in those individuals over 60 years), genetic make-up, ethnicity(Reference Sandell and Collado81), demographics and environment(Reference Eldeghaidy, Thomas and Skinner84).
Within the oral cavity, the presence of teeth increases the bacterial density compared with those with permanent tooth loss since the gingival crevices between teeth represent a greater surface area and environment for bacterial growth(Reference Kishi, Ohara-Nemoto and Takahashi85). Other important factors considered to make an impact on the variety of nitrate-reduction bacteria present in the oral cavity are ageing and sex. However, in a recent human study conducted in nine participants < 22 years and nine participants > 70 years, a similar salivary microbiome at baseline and after placebo beetroot juice was found in both groups. Comparable changes in bacterial composition (increases in Rothia and Neisseria) were also evident in both age groups in response to consuming 70 ml of beetroot juice (≈ 6·2 mmol nitrate) daily for 10 d(Reference Vanhatalo, Blackwell and L’Heureux86), suggesting that age was not an important modulator of the oral bacteria composition in this study. Few studies have determined differences in oral bacteria composition between men and women. In order to address this knowledge gap, Kapil et al. (Reference Kapil, Rathod and Khambata87) examined the impact of sex on nitrate-reducing bacteria abundance in thirteen male and thirteen females aged 18–45 years. Oral bacteria samples were collected before and after nitrate supplementation and all samples were analysed by 16S ribosomal RNA sequencing. Significant sex-dependent effects on oral nitrate-reducing bacteria composition were not found in this study. However, sub-group analysis indicated females to have a non-significant tendency for a higher activity of nitrate-reducing bacteria than men(Reference Ahmed, Nichols and Honavar74,Reference Kapil, Rathod and Khambata87) , but these findings need to be confirmed in a suitably powered study.
Inter-individual variability in number and composition of oral bacteria: modifiable factors
Several modifiable factors have been reported to influence and change the oral nitrate-reducing bacteria composition, with dietary nitrate intake considered to be one of the most important factors(Reference Govoni, Jansson and Weitzberg27,Reference Liddle, Burleigh and Monaghan88) . In a recent cross-over study conducted in eighteen volunteers assigned to receive a nitrate supplement or a placebo for 10 d, an increase in the abundance of some nitrate-reducing bacteria, particularly Rothia and Neisseria, was linked with the ability of an individual to reduce the nitrate supplement. However, changes were not observed with the Prevotella and Veillonella species(Reference Vanhatalo, Blackwell and L’Heureux86). Interestingly, these results corroborate findings from another study which reported the reduction in Prevotella and Veillonella species in the oral cavity of elderly adults following dietary nitrate intake to be associated with a lower mortality risk in this population(Reference Liddle, Burleigh and Monaghan88). Furthermore, the increased prevalence of Rothia and Neisseria species relative to the Prevotella and Veillonella species was linked to higher NO bioavailability in both saliva and plasma(Reference Vanhatalo, Blackwell and L’Heureux86). These findings imply that the oral bacteria community is responsive to changes in the level of dietary nitrate intake(Reference Koopman, Buijs and Brandt89). However, the authors also reported that individuals with a higher abundance of Campylobacter concisus and Prevotella melaninogenica in their oral cavity at baseline may not be as responsive to dietary nitrate intake than those with a lower proportion of these bacteria(Reference Vanhatalo, Blackwell and L’Heureux86). This might reflect the fact that both C. concisus and P. melaninogenica are predominately nitrite, but not nitrate, reducers in the oral cavity. Therefore, dietary nitrate availability may affect the growth and composition of particular groups of oral bacteria which can be related to improved cardiovascular health(Reference Koopman, Buijs and Brandt89). Of particular note, drinking beetroot juice rich in dietary nitrate can increase the oral cavity pH from 7·0 to 7·5, which is close to the optimal pH of 8 required for nitrate reductase activity(Reference Hohensinn, Haselgrübler and Müller90). Therefore, the effect of pH is also important in terms of the proliferation and inhibition of different populations within the oral bacterial community(Reference Vanhatalo, Blackwell and L’Heureux86).
In a similar fashion, some health conditions have also been reported to influence the oral bacterial composition, with a lower density of nitrate-reducing bacteria and a different bacterial composition found in individuals with raised blood pressure (hypertensives) than normotensive subjects(Reference Al Khodor, Reichert and Shatat31). A recent novel study has provided further evidence on the relationship between differences in oral bacteria composition with hypertension in postmenopausal women (n 446). This study analysed oral bacterial samples by using 16S RNA sequencing and found that the abundance of Prevotella oral species 317 and Streptococcus oralis were significantly lower in women with elevated blood pressure compared with those with normal blood pressure(Reference Gordon, LaMonte and Genco91). Furthermore, the differences in the oral bacteria communities between groups also seemed to be associated with the severity and progression of the hypertension(Reference Goh, Trinh and Colombo33). Conversely, a higher abundance of nitrate-reducing bacteria were observed in individuals who suffer from migraines (a vascular-driven process associated with changes in NO). Interestingly, the dominant nitrate-reducing bacteria in these individuals were Pseudomonas and Streptococcus which were not common in subjects who did not suffer with migraines. Oligotyping (the technique for differentiation between closely related microbial taxa)(Reference Stellato, Utter and Voorhis92) was performed for both genera to investigate the strain-level differences across the bacterial population. Pseudomonas decompose to two oligotypes (different strains of the same species) and have differential abundance patterns with significantly higher abundance in oligotype 2 in those suffering from migraines compared with non-sufferers(Reference Gonzalez, Hyde and Sangwan93). These results suggest that the type of these oral bacteria may be more prevalent in individuals with migraines. However, more work is needed to find the link and the mechanism to explain how these bacteria adapt genetically to their host environment. Therefore, there may be an optimum number and composition of nitrate-reducing bacteria which has beneficial effects, and a greater level may have a negative impact on conditions associated with blood vessel dilation such as migraine. However, it should be acknowledged that nitrate reduction and metabolism cannot be attributed to a single bacterial species as they are unlikely to express all of the enzymes required to decompose nitrate simultaneously. More likely, these individual nitrate-reducing bacteria are considered to work in synergy with other members of the microbial community. This has been demonstrated by Hyde et al. (Reference Hyde, Andrade and Vaksman43) who found that mixed colonies of high and low nitrate reducers showed a greater capacity for nitrate reduction than mixes of either multiple high reducers or individual nitrate-reducing bacteria. This highlights the complexity of the oral microbiome and the impact on dietary nitrate metabolism.
Cardiometabolic diseases including obesity, the metabolic syndrome and type 2 diabetes are major contributors to global CVD disease burden. Whilst some studies have reported plasma nitrate/nitrite levels to be negatively associated with waist circumference(Reference Kondo, Ueyama and Imai94), obesity(Reference Piva, Tatsch and De Carvalho95) and blood pressure, others have observed positive associations between plasma nitrite and BMI, fasting blood glucose(Reference Li, Lyn and Lapu-Bula96), systolic blood pressure and the fasting lipid profile. In support of these findings, Akram et al. (Reference Akram, Fuchs and Daue97), found plasma nitrite levels to be higher in individuals with both obesity and the metabolic syndrome followed by those with obesity alone, with the lowest levels in those with normal weight. Whether high plasma nitrite levels play a role in the worsening of the cardiometabolic risk markers is a public health issue since higher dietary nitrate intakes may also cause higher levels of plasma NOx (sum of nitrate and nitrite levels). Furthermore, these data are associations, which do not indicate whether cardiometabolic risk markers change in response to varying nitrate/nitrite intakes and do not prove cause and effect. Interestingly, a review of the evidence suggests the contrary, with dietary nitrate supplementation found to reverse or improve some of the features of the metabolic syndrome and be protective against the development of CVD(Reference Lundberg, Carlström and Weitzberg98). Although these beneficial effects may be related to improvements in NO metabolic pathways and glucose control, we cannot discount that favourable changes in the gut microbiota in response to dietary nitrate intake may also represent an important mechanism since dysbiosis (a term to describe microbial imbalance) is a common feature of the cardiometabolic diseases. However, very few studies have determined the impact of dietary nitrate supplementation on the gut microbiota in humans, with a very short-term study with nitrate-rich fruit and vegetable juice suggesting a reduction in the Firmicutes:Bacteroides ratio after 3 d which was related to higher plasma nitrate/nitrite levels(Reference Henning, Yang and Shao99). Furthermore, a 1-year intervention with the Mediterranean diet, rich in vegetables, was associated with increased abundance of specific taxa that were inversely associated with inflammatory markers(Reference Ghosh, Rampelli and Jeffery100). More studies are needed to address this research gap which also include analysis of the oral microbiome to determine whether increases in the abundance of nitrate-reducing bacteria are related to improvements in cardiovascular health.
Oral hygiene habits, including daily use of an antibacterial mouthwash or tongue scraper, have been found to not only reduce acute bacterial infection, but also numbers of bacteria present(Reference Woessner, Smoliga and Tarzia30). On the other hand, poor oral hygiene contributes to dysbiosis by accumulating a plaque biofilm which contains large number of microbes including nitrate-reducing bacteria(Reference Kilian, Chapple and Hannig101). This can cause dental infections and gingivitis by increasing pathogenic bacteria such as Porphyromonas gingivalis (Reference Kishi, Ohara-Nemoto and Takahashi85). Studies have shown that patients with periodontal disease to have higher levels of salivary nitrite which may be partly derived from the reduction of nitrates by the oral bacteria. Since nitrite has been shown to have an antimicrobial effect against gastrointestinal and oral pathogens, it has been speculated that the salivary glands may respond to the periodontal infection by enhancing the secretion of nitrate and production of nitrite by the nitrate-reducing bacteria as a host defence mechanism(Reference Qu, Wu and Pang102). This is thought to reduce the prevalence of the acidogenic bacteria which contribute to the development of dental caries. In agreement, Doel et al. (Reference Doel, Benjamin and Hector42) has reported a significant reduction in dental carries in study participants with high salivary nitrate concentration. Epidemiological studies have reported an association between periodontal disease with CVD. Although the cause-and-effect relationship has not been proven, studies have suggested that inflammation caused by the oral infection may contribute to the development and progression of the atherosclerotic plaque. Interestingly, periodontal pathogens have been identified in the atherosclerotic plaque, suggesting a direct role in CVD. However, to date, periodontal disease has not been considered to be a CVD risk marker(Reference Liccardo, Cannavo and Spagnuolo103). Lifestyle habits such as smoking can also influence oral bacteria composition(Reference Ahmed, Nichols and Honavar74). In a study conducted in nine non-smokers aged 20–45 years and five healthy active smokers (>20 cigarettes per week) aged 30–60 years, nitrate reduction activity was found to be over 80 % lower in smokers compared with non-smokers(Reference Ahmed, Nichols and Honavar74). However, the low numbers of individuals within each group may have influenced the results observed.
As previously mentioned, dietary nitrates have been shown to interact with other food components such as lipids(Reference Delmastro-Greenwood, Hughan and Vitturi72), with similar reports for polyphenols(Reference Lovegrove, Stainer and Hobbs104), alcohol(Reference McDonagh, Wylie and Morgan105) and proteins(25). In particular, foods and beverages rich in polyphenols including apple, tea and orange juice have been shown to lead to a 3-fold increase in NO production in the stomach(Reference Gago, Nyström and Cavaleiro106) and reduce endogenous N-nitrosamine formation. Along with polyphenols, the content of ethanol in red wine can also interact with nitrite forming ethyl nitrite which works as a nitrosation agent and may mediate NO effects. These interactions with other dietary components may therefore play a role in modulating the circulating NO levels and bioavailability of the nitrate and nitrite contained within foods.
In summary, a systematic approach was used to identify the studies that have determined the impact of oral bacteria on blood pressure in response to nitrate intake, from dietary sources or supplements. However, only a very limited number of human (n 2) and animal (n 3) studies have addressed this research question, with the remaining studies examining the importance of the oral bacteria on the nitrate-reducing capacity on circulating nitrite concentrations and blood pressure. Based on our observations from these studies, there is accumulating evidence to suggest that absence of nitrate-reducing oral bacteria was associated with increasing blood pressure even when accompanied by a high nitrate intake. However, some of the studies failed to see any effects, which may be due to type of mouthwash used in the human studies or the method of application of the mouthwash in the animal study(Reference Hyde, Luk and Tribble29). Sex, hypertension, and tongue cleaning were all found to be important potential determinants of the variability in the responses between participants. Of these, the dental hygiene practice of tongue cleaning, which is recommended by the American Dental Association, appeared to promote oral microbiota diversity and be associated with a greater ability to recover the tongue microbiome after mouthwash use. Potential mechanisms to explain the blood pressure-lowering effects of dietary nitrates included increases in plasma nitrite, S-nitrosothiols, nitro-fatty acids and vascular nitrosylation and cross-talk between the enterosalivary nitrate–nitrite–NO pathways and eNOS activity in the endothelial cells. However, the limited number of studies performed make it difficult to draw any firm conclusions from this literature review.
Conclusions
With the increasing prevalence of non-communicable diseases there is an urgent need for further studies to investigate the role of the oral bacteria on cardiovascular health in response to dietary nitrate intake, and to determine the underlying mechanisms. With vascular function now recognised as an important prognostic marker for future CVD risk, studies incorporating real-time measures of vascular reactivity and tone are required. Furthermore, the use of rigorous methods to determine changes in the abundance and composition of the oral bacteria in response to intake of dietary nitrate would help to identify important nitrate-reducing bacteria related to changes in vascular function and determine whether these bacterial groups are also evident in the gut microbiome, a proposed modulator of chronic disease risk. Diets containing nitrate-rich foods may contain other bioactive components which could also contribute to CVD risk reduction, including fibres, vitamins, minerals and flavonoids. Such diets may offer a number of advantages over nitrate/nitrite supplemental use, not only due to the availability of other bioactive components, but also because of reports of vascular adaptation and risk of marked acute hypotension after supplemental nitrate use, not found with nitrate-rich diets(Reference Omar, Artime and Webb107). With hypertension a major risk factor for CVD, more studies are needed to determine whether diets higher in nitrate-rich foods can be recommended for blood pressure lowering and disease prevention in healthy individuals and those at greater CVD risk.
Acknowledgements
H. S. A. was supported by a PhD studentship funded by King Saud University (Saudi Arabia). This research received no specific grant from any funding agency, and commercial.
The authors responsibilities were as follows: H. S. A., D. A. H., K. G. J. and J. A. L. contributed to the conception of the literature search strategy. H. S. A. undertook the literature review. D. A. H., K. G. J. and J. A. L. provided feedback and guidance on previous drafts of the review and J. A. L. was responsible for final content.
The authors have no conflicts of interest to declare.
Supplementary material
For supplementary material referred to in this article, please visit https://doi.org/10.1017/S0954422420000281