Introduction
Abnormal trilobites represent a primary means of understanding how a group of wholly extinct euarthropods responded to, and recovered from, injuries and developmental or genetic malfunctions (Owen, Reference Owen1985; Babcock, Reference Babcock1993, Reference Babcock, Mikulic, Landing and Kluessendorf2007; Bicknell and Pates, Reference Bicknell and Pates2020). The historical record of abnormal trilobites extends back to the middle nineteenth century (Portlock, Reference Portlock1845) with more detailed documentation arising during the later twentieth century (see Bergström and Levi-Setti, Reference Bergström and Levi-Setti1978; Šnajdr, Reference Šnajdr1978a, Reference Šnajdr1979, Reference Šnajdr1985; Rudkin, Reference Rudkin1979, Reference Rudkin1985; Conway Morris and Jenkins, Reference Conway Morris and Jenkins1985; Owen, Reference Owen1985; Babcock and Robison, Reference Babcock and Robison1989; Babcock, Reference Babcock1993). In the last decade alone, a marked surge in publications documenting these abnormal specimens has occurred. The majority of these works have considered abnormal specimens in the context of trilobite paleobiology and paleoecosystems (Budil et al., Reference Budil, Fatka, Zwanzig and Rak2010; Zamora et al., Reference Zamora, Mayoral, Esteve, Gámez-Vintaned and Santos2011; Fatka et al., Reference Fatka, Budil and Grigar2015, Reference Fatka, Budil and Zicha2021; Cheng et al., Reference Cheng, Han, Ou, Zhang, Guo and Zhang2019; Bicknell and Pates, Reference Bicknell and Pates2020; Bicknell and Smith, Reference Bicknell and Smith2021; Bicknell et al., Reference Bicknell, Smith, Bruthansová and Holland2021b; Foster, Reference Foster2021; Zong, Reference Zong2021a, Reference Zongb). Population-based data have been presented to explore injury patterns and lateralization in the trilobite fossil record (Pates et al., Reference Pates, Bicknell, Daley and Zamora2017; Bicknell et al., Reference Bicknell, Paterson and Hopkins2019; Pates and Bicknell, Reference Pates and Bicknell2019). Finally, limited publications have collated these observations to understand patterns of predation across the Paleozoic (Bicknell and Paterson, Reference Bicknell and Paterson2018; Vinn, Reference Vinn2018; Klompmaker et al., Reference Klompmaker, Kelley, Chattopadhyay, Clements, Huntley and Kowalewski2019). To further the examination of abnormal trilobites, and bolster evidence that is useful for large meta-analyses, we present seven new examples of abnormal specimens: one Lyriaspis sigillum Whitehouse, Reference Whitehouse1939 from the Wuliuan of Australia; one Zacanthoides sp. indet. (of Walcott, Reference Walcott1888) from the Wuliuan of the USA; two Asaphiscus wheeleri Meek, Reference Meek1873, and two Elrathia kingii (Meek, Reference Meek1870) from the Drumian of the USA; and one Ogygiocarella debuchii (Brongniart, Reference Brongniart1822) from the Darriwilian of Wales.
Geological context
The Lyriaspis sigillum specimen (AM F33980 and AM F34209) was collected from the Beetle Creek Formation, Georgina Basin at the type section (proximal to the Templeton River headwaters), Mt. Isa, western Queensland, Australia. There the unit consists of 15 m of siliceous shale, fine sandstone, thin calcareous beds, and chert horizons that were likely deposited below a storm wave base, at the oceanward shelf edge of a large epeiric sea (Fleming, Reference Fleming1977; Kruse, Reference Kruse2002). This portion of the formation is occasionally referred to as the ‘Lower Siltstone Member’ (Russell, Reference Russell1967), or the ‘undifferentiated Beetle Creek Formation’ (sensu Dunster et al., Reference Dunster, Kruse, Duffett and Ambrose2007). At the type locality, the unit unconformably overlies the Proterozoic rocks of the basin and is overlain by the Wuliuan-aged Inca Formation (Southgate and Shergold, Reference Southgate and Shergold1991; Dunster et al., Reference Dunster, Kruse, Duffett and Ambrose2007). Presence of the trilobite species Deiradonyx sp. aff. D. collabrevis Öpik, Reference Öpik1982 (likely D. collabrevis) suggests placement in the Pentagnostus praecurrens Biozone—the latter taxon co-occurs with the eponym in the ‘White Shale’ member of the Coonigan Formation, Gnalta Shelf, western New South Wales (originally described as P. veles Öpik, Reference Öpik1979, later synonymized by Laurie, Reference Laurie1988). Further support comes from close faunal ties to the Jigaimara Formation (Laurie, Reference Laurie2006b), Arafura Basin, Northern Territory and the lithological correlations to the lower Arthur Creek Formation, southeastern Georgina Basin (Laurie, Reference Laurie2004, Reference Laurie2006a; Dunster et al., Reference Dunster, Kruse, Duffett and Ambrose2007). The Australian Pentagnostus praecurrens Zone sits within early Templetonian Stage, equivalent to the Wuliuan Stage (Sundberg et al., Reference Sundberg, Geyer, Kruse, McCollum, Pegel, Zylinska and Zhuravlev2016, Peng et al., Reference Peng, Babcock, Ahlberg, Gradstein, Ogg, Schmitz and Ogg2020 and references therein).
The Zacanthoides sp. indet. specimen (FHPR 17618) was collected from the Half Moon Mine locality, Chisholm Formation, Lincoln County, eastern Nevada. The Chisholm Formation there consists of 17–35 m of tan to gray to light reddish calcareous shale with some thin (0.05–3 m) interbedded carbonates (Sundberg, Reference Sundberg2011). It is a relatively thin, slope-forming unit overlying the Lyndon Limestone and underlying the Highland Peak Formation. The examined specimen co-occured with trilobite genera, e.g., Amecephalus Walcott, Reference Walcott1924, Athabaskia Raymond, Reference Raymond1928, and Glossopleura Poulsen, Reference Poulsen1927; echinoderms (Gogia Walcott, Reference Walcott1917); and the edrioasteroid Totiglobus Bell and Sprinkle, Reference Bell and Sprinkle1978 (Sundberg, Reference Sundberg2011; Foster and Gaines, Reference Foster and Gaines2016). This fauna indicates that, at the Half Moon Mine, the Chisholm Formation is from the Glossopleura walcotti and Pentagnostus praecurrens biozones of the Wuliuan Stage (Sundberg, Reference Sundberg2011; Peng et al., Reference Peng, Babcock, Ahlberg, Gradstein, Ogg, Schmitz and Ogg2020).
The Asaphiscus wheeleri and Elrathia kingii specimens were collected from the Wheeler Formation, Millard County, western Utah, USA. The A. wheeleri (FHPR 16702, MWC 9973) and one E. kingii (MWC 9972) are from the House Range (Wheeler Amphitheater site), whereas another E. kingii (FHPR 16640) is from the Wheeler Formation in the Drum Mountains. The Wheeler Formation is up to 277 m of gray to olive to pinkish calcareous shale (Hintze and Davis, Reference Hintze and Davis2002, Reference Hintze and Davis2003). The formation overlies the Swasey Limestone and underlies the Marjum Formation in the House Range and the Pierson Cove Formation (a carbonate-rich, Marjum Formation equivalent) in the Drum Mountains. Restriction of the Wheeler Formation to the House Range and Drum Mountains reflects infilling of the middle Cambrian House Range Embayment—a fault-bounded, deep-water bathymetric feature of the Laurentian shelf that was surrounded on three sides by shallow carbonate belt deposits (Rees, Reference Rees1986; Foster and Gaines, Reference Foster and Gaines2016). The deeper-water setting of the Wheeler Formation within the embayment allowed preservation of articulated trilobites and soft-bodied taxa (Robison et al., Reference Robison, Babcock and Gunther2015). The examined specimens from the House Range are from the upper Wheeler Formation, and the Drum Mountains specimen is also from the upper portion of the same formation, ~7 m below the Pierson Cove Formation. This places the material in the Bolaspidella and Ptychagnostus atavus biozones of the Drumian Stage (Babcock et al., Reference Babcock, Robison, Rees, Peng and Saltzman2007; Peng et al., Reference Peng, Babcock, Ahlberg, Gradstein, Ogg, Schmitz and Ogg2020).
The Ogygiocarella debuchii specimen (AM F128230) originated from a Llanfawr quarry near the township of Llandrindod Wells, central Wales, UK. These quarries are known for complete O. debuchii specimens, as well as other well-preserved trilobites (Hughes, Reference Hughes1969, Reference Hughes1971, Reference Hughes1979; Owens, Reference Owens1981, Reference Owens2002; Sheldon, Reference Sheldon1987a). The specimen was from the lower Llanfawr Mudstones (Sheldon, Reference Sheldon1987b; Owens, Reference Owens2002) of the Builth Inlier. The Llanfawr Mudstones layer is relatively thick (> 200 m at some localities) and dominated by fine mudstone and siltstone that were deposited in relatively quiet, middle to outer shelf depths (Fortey and Owens, Reference Fortey and Owens1987; Owens, Reference Owens2002). Ogygiocarella debuchii ranges through the entire formation, between the upper Didymograptus murchisonii and lower Nemagraptus gracilis biozones. However, the specimens reported here are likely from the regional Llandeilian Stage (Hustedograptus? teretiusculus Biozone) (Sheldon, Reference Sheldon1987b), correlating with the upper Middle Ordovician, Darriwilian (Bettley et al., Reference Bettley, Fortey and Siveter2001; Cooper and Sadler, Reference Cooper, Sadler, Gradstein, Ogg, Schmitz and Ogg2012; Bicknell et al., Reference Bicknell, Smith, Bruthansová and Holland2021b).
Materials and methods
Trilobite specimens within the AM, FHPR, and MWC were reviewed for abnormalities by RDCB and JRF. A total of seven specimens were identified. Specimens were coated in magnesium oxide and photographed under low angle LED light with a Canon EOS 5DS (AM specimens) or a Canon EOS 5D Mark IV (FHPR and MWC specimens). All measurements of specimens were made using ImageJ (Schneider et al., Reference Schneider, Rasband and Eliceiri2012).
Repositories and institutional abbreviations
AM = Australian Museum, Sydney, New South Wales, Australia; FHPR = Utah Field House of Natural History State Park Museum, Vernal, Utah, USA; MWC = Museums of Western Colorado, Dinosaur Journey Museum, Fruita, Colorado, USA.
Results
The Lyriaspis sigillum specimen is articulated internal (AM F34209) and external (AM F33980) molds, and AM F33980 is more complete (Fig. 1). The specimen has a U-shaped indentation on the left thoracic pleural lobe (Fig. 1.1, 1.2). The indentation impacts thoracic segments 3–6, truncates pleurae by 1 mm, and is 2.6 mm long. The abnormality margin is cicatrized and thoracic segments 4 and 5 are pinched distally (Fig. 1.1, 1.4).
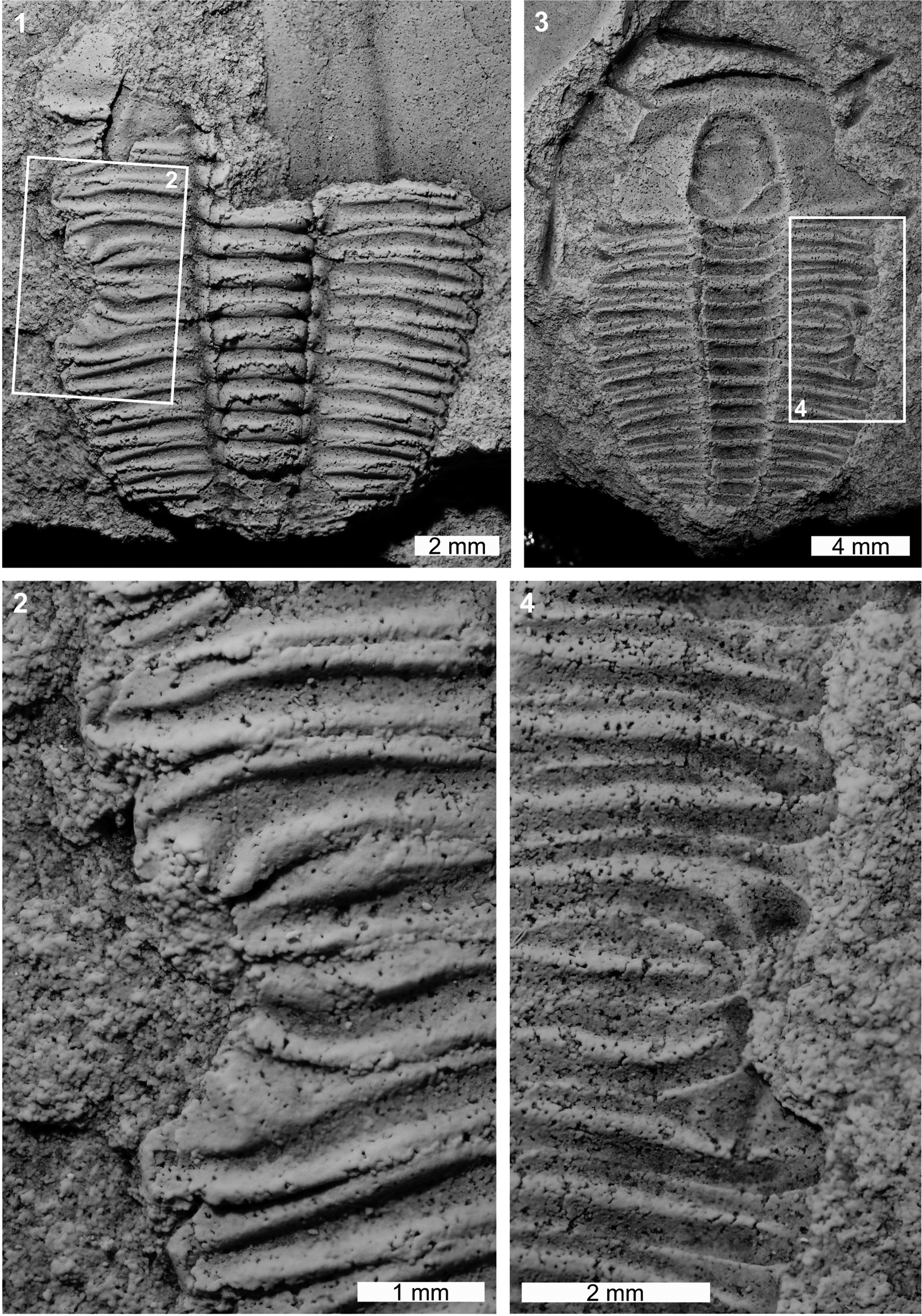
Figure 1. Malformed Lyriaspis sigillum Whitehouse, Reference Whitehouse1939, Beetle Creek Formation (Miaolingian, Wuliuan): (1, 2) AM F34209: (1) complete specimen; (2) detail of abnormality in box in (1) showing U-shaped injury; (3, 4) AM F33980: (3) complete specimen; (4) detail of abnormality in box in (3) showing injury. All specimens coated. All images converted to grayscale.
The Zacanthoides sp. indet. specimen is a partial internal mold showing the anterior right cephalic and thoracic regions (FHPR 17618; Fig. 2.3, 2.4). The first thoracic pleural spine of the specimen has been truncated by 1.3 mm (Fig. 2.3, 2.4). The spine also has a U-shaped indentation with a reduced, posteriorly directed spine (Fig. 2.4). This reduced section likely records abnormal regrowth of the segment through subsequent molting events.
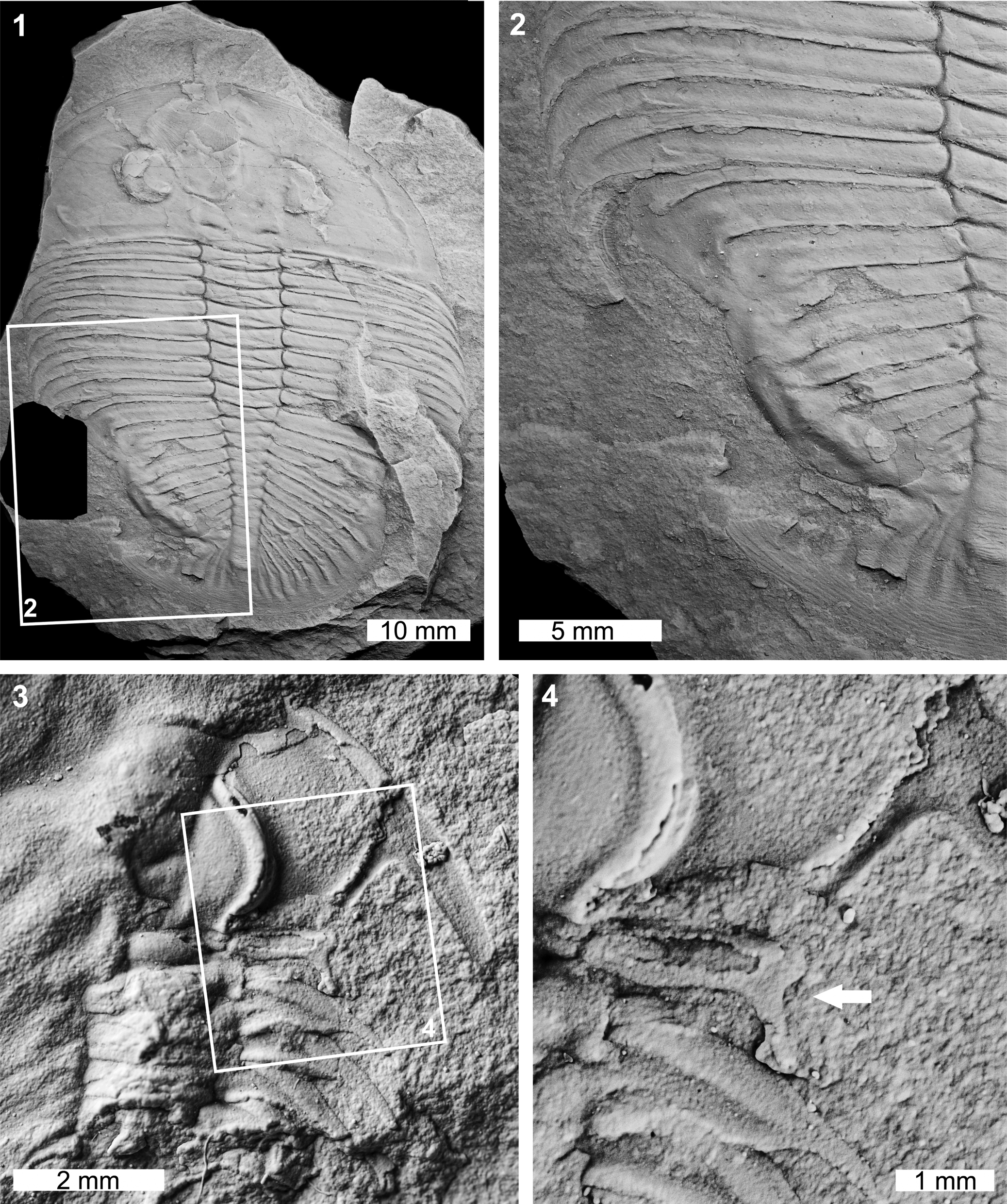
Figure 2. Malformed Ogygiocarella debuchii (Brongniart, Reference Brongniart1822) and Zacanthoides sp. indet. (of Walcott, Reference Walcott1888): (1, 2) Ogygiocarella debuchii from the Llanfawr Mudstones (Middle Ordovician, Darriwilian), AM F128230: (1) complete specimen; (2) detail of abnormality in box in (1) showing W-shaped indentation on the left side of the pygidium; (3, 4) Zacanthoides sp. indet. from the Half Moon Mine, Chisholm Formation (Miaolingian, Wuliuan), FHPR 17618: (3) complete specimen; (4) detail of abnormality in box in (3) showing abnormally developed pleural spine (white arrow). All specimens coated. All images converted to grayscale.
Two abnormal Asaphiscus wheeleri specimens were considered. The first specimen is a complete internal mold (MWC 9973; Fig. 3.1–3.3). MWC 9973 has two abnormalities on the left thoracic pleural lobe. The anterior abnormality truncates the thoracic pleurae 1–4 by ~1.5 mm when compared to the right pleural lobe and shows an L-shaped morphology (sensu Bicknell and Pates, Reference Bicknell and Pates2020). This region shows evidence of possible regrowth of the anteriormost pleurae. Further, the fourth thoracic pleura has a pinched region (Fig. 3.2). The posterior abnormality is a U-shaped indentation that impacts thoracic pleurae 7–9 and the anteriormost pygidium. The edge of this indentation shows marked cicatrization (Fig. 3.3) and truncates pleurae by 2.5 mm. The second specimen is an internal mold with a damaged cephalon (FHPR 16702; Fig. 3.4, 3.5). The specimen has a single segment injury (SSI) on the eighth pleura on the right pleural lobe. This truncates the pleural spine by 1.7 mm.
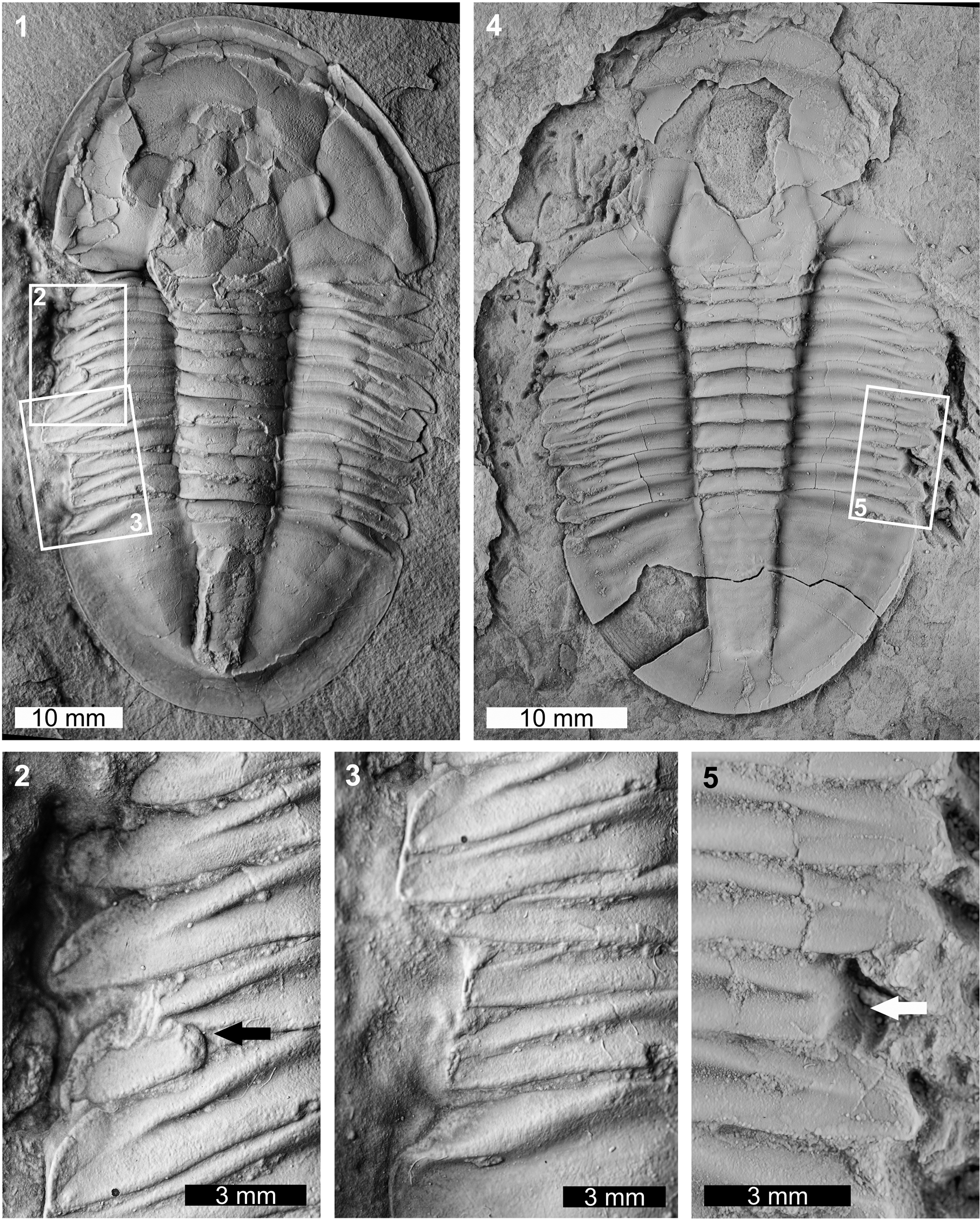
Figure 3. Malformed Asaphiscus wheeleri Meek, Reference Meek1873, House Range, Wheeler Formation (Miaolingian, Drumian): (1–3) MWC 9973: (1) complete specimen; (2) detail of abnormality in box in (1) showing L-shaped injury and pinched pleural region (black arrow); (3) detail of abnormality in box in (1) showing U-shaped injury; (4, 5) FHPR 16702: (4) complete specimen; (5) detail of abnormality in box in (4) showing SSI (white arrow). All specimens coated. All images converted to grayscale.
Two abnormal Elrathia kingii specimens were identified. The first specimen is a complete internal mold (MWC 9972; Fig. 4.1, 4.2). MWC 9972 has a large W-shaped indentation on the left thoracic pleural lobe impacting thoracic pleurae 1–7. The indentation extends up to 4.3 mm into the specimen and shows evidence of cicatrization (Fig. 4.2). The second specimen is an internal mold and likely represents a molt (Daley and Drage, Reference Daley and Drage2016; Drage et al., Reference Drage, Holmes, García-Bellido and Daley2018; Drage, Reference Drage2019) (FHPR 16640; Fig. 4.3, 4.4). The specimen has a V-shaped indentation with limited cicatrization that impacts thoracic pleurae 7 and 8 on the right pleural lobe (Fig. 4.4). This indentation truncates pleurae by 4.6 mm.
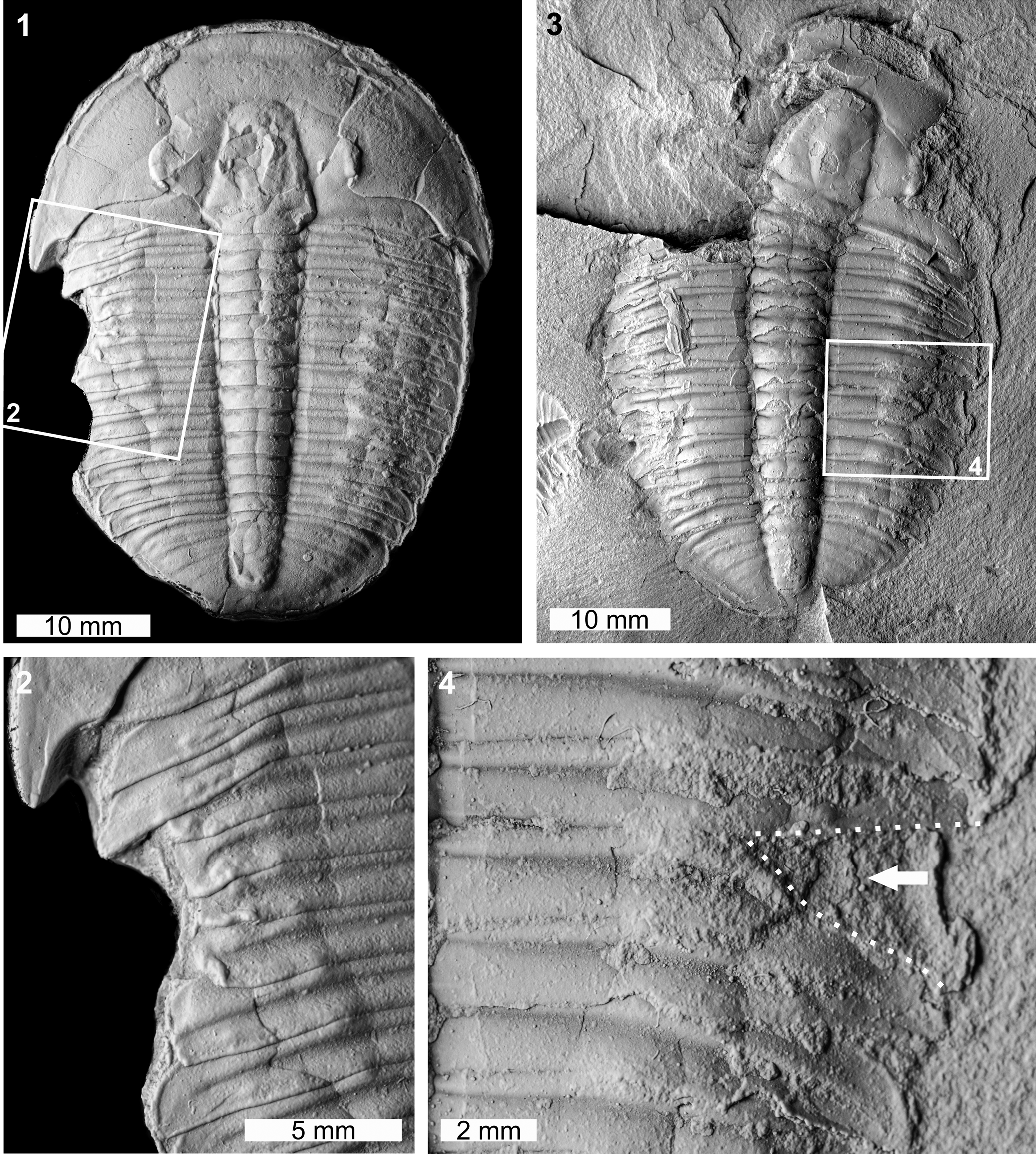
Figure 4. Malformed Elrathia kingii (Meek, Reference Meek1870), Wheeler Formation (Miaolingian, Drumian): (1, 2) MWC 9972, House Range outcrop: (1) complete specimen; (2) detail of abnormality in box in (1) showing large W-shaped indentation; (3, 4) FHPR 16640, Drum Mountains outcrop: (3) complete specimen; (4) detail of abnormality in box in (3) showing V-shaped indentation (dotted white line, white arrow). All specimens coated. All images converted to grayscale.
The Ogygiocarella debuchii specimen is an articulated internal mold (AM F128230; Fig. 2.1, 2.2). The specimen has a large W-shaped indentation on the left side of the pygidium that extends across ~75% of the pygidium (Fig. 2.1, 2.2). The malformation is 17.1 mm long and the margin shows extensive fusion of the damaged pygidium. This results in an inflated pygidial border relative to other exoskeletal regions (Fig. 2.2).
Discussion
The abnormal trilobites documented here show evidence of removal of exoskeletal sections. Furthermore, no specimens have abnormal growths indicative of genetic malformations (Owen, Reference Owen1980, Reference Owen1985; Babcock, Reference Babcock1993; Bicknell and Smith, Reference Bicknell and Smith2021) or circular/ovate structures indicative of pathologies (Šnajdr, Reference Šnajdr1978b; Babcock, Reference Babcock1993; De Beats et al., Reference De Baets, Budil, Fatka, Geyer, De Baets and Huntley2021). These specimens therefore show examples of injuries (Owen, Reference Owen1985; Babcock, Reference Babcock1993; Pates et al., Reference Pates, Bicknell, Daley and Zamora2017; Bicknell et al., Reference Bicknell, Smith, Bruthansová and Holland2021b) that occurred through failed predation, a molting complication, or some other traumatic injury. Trilobites with overdeveloped thoracic spines, e.g., Nevadia weeksi (Walcott, Reference Walcott1910), likely experienced molting complications and would have damaged the hypertrophied spines (Conway Morris and Jenkins, Reference Conway Morris and Jenkins1985; Bicknell et al., Reference Bicknell, Holmes, Pates, García-Bellido and Paterson2022). The examined specimens lack these morphologies. Furthermore, L-, U-, V-, and W-shaped indentations are commonly attributed to failed predation (Šnajdr, Reference Šnajdr1981, Reference Šnajdr1985; Owen, Reference Owen1985; Babcock, Reference Babcock1993, Reference Babcock, Mikulic, Landing and Kluessendorf2007; Fatka et al., Reference Fatka, Szabad and Budil2009, Reference Fatka, Budil and Grigar2015; Pates et al., Reference Pates, Bicknell, Daley and Zamora2017; Bicknell and Holland, Reference Bicknell and Holland2020). As such, the indentations likely record failed predation.
Explanations for SSIs are therefore worth considering. When SSIs are observed with larger injuries on the opposing side (Conway Morris and Jenkins, Reference Conway Morris and Jenkins1985; Nedin, Reference Nedin1999; Bicknell et al., Reference Bicknell, Holmes, Pates, García-Bellido and Paterson2022), they likely reflect a bilateral predatory attack. When SSIs are not identified with larger injuries, further explanation is needed. It seems unlikely that predators large enough to consume trilobites would damage only one segment (Babcock, Reference Babcock1993). However, smaller predators could have removed a single section while a trilobite was in a soft-shelled stage. Failed predation as an explanation for unpaired SSIs is therefore plausible. An SSI could occur during enrollment—if an individual enrolled too quickly or on an abnormal angle, a segment could be nicked. Furthermore, intraspecific competition during feeding or seeking communal shelter during the postecdysal, soft-shell phase could have resulted in minor damage. As such, consideration of broader paleoecology is needed when proposing explanations for SSIs.
The injured Lyriaspis sigillum documented here represents the first record of a malformed trilobite from the Beetle Creek Formation and the second example of predation from the deposit (see Jones and McKenzie, Reference Jones and McKenzie1980). The degree of exoskeletal warping around the injury in AM F34209/F33980 indicates that the specimen was damaged during a soft-shelled phase and also suggests that durophages were present in the Beetle Creek Formation biota. One of the co-occurring Xystridura spp. (four are currently known from the unit) is a possible injury-maker, given the size of the genus (Öpik, Reference Öpik1975). Appendage data is unknown for Xystridura Öpik, Reference Öpik1975; however, it was likely comparable to other predatory artiopodans and used gnathobasic spines on walking legs to masticate prey (Zacaï et al., Reference Zacaï, Vannier and Lerosey-Aubril2016; Bicknell et al., Reference Bicknell, Ledogar, Wroe, Gutzler, Watson and Paterson2018, Reference Bicknell, Holmes, Edgecombe, Losso, Ortega-Hernández, Wroe and Paterson2021a). Alternatively, large, nonbiomineralized artiopodans with gnathobasic spines might have produced the injury and the lack of soft-bodied preservation in the Beetle Creek Formation precludes the identification of these forms. Regardless, the rarity of injured L. sigillum reflects either an occasional diet of smaller trilobites, or the complete consumption of prey. Shelly coprolites from equivalent Xystridura-bearing units (e.g., the Giles Creek Dolostone in the neighboring Amadeus Basin, Northern Territory) would support the second theory (unpublished data, Smith, 2022).
Prior to documentation of the injured Asaphiscus wheeleri specimen considered here, only malformed Asaphiscus wheeleri pygidia were known (Vorwald, Reference Vorwald1984; Babcock, Reference Babcock1993; Bicknell and Paterson, Reference Bicknell and Paterson2018). The addition of thoracic injuries illustrates that Asaphiscus wheeleri was subject to more substantial predation than previously thought. Previous records of predation within the Wheeler Formation have been assigned to the activities of priapulid worms (Conway Morris and Robison, Reference Conway Morris and Robison1986), radiodonts (Babcock, Reference Babcock1993), and artiopodans with gnathobasic spines on walking legs (Bicknell and Pates, Reference Bicknell and Pates2020). However, biomechanical and functional morphological evidence suggest that the last group were the most effective at breaking exoskeletons (Bruton, Reference Bruton1981; Stein, Reference Stein2013; Zacaï et al., Reference Zacaï, Vannier and Lerosey-Aubril2016; Bicknell et al., Reference Bicknell, Ledogar, Wroe, Gutzler, Watson and Paterson2018, Reference Bicknell, Holmes, Edgecombe, Losso, Ortega-Hernández, Wroe and Paterson2021a; Holmes et al., Reference Holmes, Paterson and García-Bellido2020). Further examination of the morphology and predatory ability of other possible predators from these sites is needed to present more complete paleoecological reconstructions and aid in understanding the role of predation during the earliest marine ecosystems.
Acknowledgments
This research was funded by an Australian Research Council grant (DP200102005), a University of New England Postdoctoral Fellowship (to RDCB), a Karl Hirsch Memorial Grant (to RDCB), and an Australian Museum AMF/AMRI Visiting Research Fellowship (to RDCB). Field work facilitated in part by US Bureau of Land Management permits UT16-014S and N-86224 (to JRF), with thanks to field crew members A.R.C. Milner, R. Hunt-Foster, J. Lehane, M. Perry, and T. Lawrence. MWC specimens were collected by D. Dayvault, and we thank J. McHugh for loan of this material. We thank S. Pates for discussions regarding causes of trilobite injuries. Finally, we thank R.-W. Zong and D. Rudkin for their thorough reviews that substantially improved the text.