Introduction
Modern amphibians and their ancient relatives could hardly be more distinct in size, morphology, and ecological features. Whereas the three extant clades (frogs, salamanders, and caecilians) are generally small creatures with feeble skeletons, most of which feed on small invertebrates, their likely stem-group, Paleozoic temnospondyls, encompasses 1–2 m long, heavily ossified predators. The evolutionary transition between the Paleozoic giants and the dwarfed modern forms has long been sought among the Dissorophoidea, a speciose clade of mainly terrestrial and presumably insect-eating Carboniferous–Triassic temnospondyls that were usually smaller and had less-massive skeletons than their fish-eating fellows (Watson, Reference Watson1940; Romer, Reference Romer1947; Reig, Reference Reig1964), but alternative scenarios are still debated (Laurin and Reisz, Reference Laurin and Reisz1997; Marjanovic and Laurin, Reference Marjanovic and Laurin2008, Reference Marjanovic and Laurin2009, Reference Marjanovic and Laurin2013; Pardo et al., Reference Pardo, Small and Huttenlocker2017). In recent time, new important stem-taxa were discovered (Bolt, Reference Bolt1969; Anderson et al., Reference Anderson, Reisz, Scott, Fröbisch and Sumida2008a, Reference Anderson, Henrici, Sumida, Martens and Bermanb), which have made the origin at least of batrachians from within temnospondyls more likely and put the dissorophoids into focus of rigorous phylogenetic research (Milner, Reference Milner1988, Reference Milner1993; Trueb and Cloutier, Reference Trueb and Cloutier1991; Schoch and Milner, Reference Schoch and Milner2004; Ruta and Coates, Reference Ruta and Coates2007; Anderson, Reference Anderson2008; Fröbisch and Schoch, Reference Fröbisch and Schoch2009a; Sigurdsen and Green, Reference Sigurdsen and Green2011; Maddin et al., Reference Maddin, Jenkins and Anderson2012; Pérez Ben et al., Reference Pérez Ben, Schoch and Báez2018).
Dissorophoids themselves were only slowly recognized as a clade, because they fall into several highly distinct groups: (1) the ~50 cm long, heavily built olsoniforms, comprising the monophyletic armoured Dissorophidae and the Trematopidae (Carroll, Reference Carroll1964; Berman et al., Reference Berman, Reisz and Eberth1985; Dilkes, Reference Dilkes1990; Reisz et al., Reference Reisz, Schoch and Anderson2009; Schoch, Reference Schoch2012); (2) the 10–20 cm long terrestrial Amphibamidae (Bolt, Reference Bolt1969; Daly, Reference Daly1994; Schoch and Rubidge, Reference Schoch and Rubidge2005; Anderson et al., Reference Anderson, Henrici, Sumida, Martens and Berman2008b; Fröbisch and Reisz, Reference Fröbisch and Reisz2008); (3) the 10–30 cm long aquatic gill-bearing Micromelerpetidae (Boy, Reference Boy1972, Reference Boy1995, Reference Boy2002a, Reference Boyb; Werneburg, Reference Werneburg1991, Reference Werneburg1994; Witzmann and Pfretzschner, Reference Witzmann and Pfretzschner2003); and finally (4) the larval-neotenic, gill-bearing, up to 15 cm long Branchiosauridae (Boy, Reference Boy1972, Reference Boy1978, Reference Boy1987; Werneburg, Reference Werneburg1986, Reference Werneburg1987, Reference Werneburg1991, Reference Werneburg2001; Schoch, Reference Schoch1992; Schoch and Milner, Reference Schoch and Milner2008). Although each of these groups has been hypothesized as a clade, an inclusive test of all of these hypotheses on a broader range of dissorophoid taxa has yet to be conducted.
The first dissorophoid skeleton discovered was that of a poorly preserved branchiosaurid from the lower Permian of Münsterappel in Germany, reported by Meyer (Reference Meyer1848) as Apateon pedestris. This superficially salamander-like branchiosaurid was soon complemented by more frog-like skeletons from Pennsylvanian rocks from from Mazon Creek, Illinois, which were referred to as Pelion (Amphibamus) grandiceps (Wyman, Reference Wyman1858; Cope, Reference Cope1865). After Moodie (Reference Moodie1909, Reference Moodie1916), Romer (Reference Romer1930), and Steen (Reference Steen1931, Reference Steen1938) had reported a range of similar taxa from additional Pennsylvanian deposits in the United States and Czech Republic, Watson (Reference Watson1940) wrote a review paper on the origin of anurans in which he sought the ancestry of frogs among amphibamids, notably the tiny, lightly built Amphibamus grandiceps Cope, Reference Cope1865. The enlarged squamosal embayment (otic notch) of these taxa was given particular attention, as were the delicate stapes and the reduced nature of some dermal bones. These of course were features long known by students of temnospondyls to be shared with anurans, as exemplified by Quenstedt’s (Reference Quenstedt1850, p. 14) exclamation “true frog ears!” (German “wahre Froschohren”) upon his discovery of the otic notch in the large capitosauroid temnospondyl Cyclotosaurus.
Almost three decades after Watson, Bolt (Reference Bolt1969) reported the tiny amphibamid Doleserpeton annectens, phylogenetically the sister taxon to Amphibamus, but with two additional characters then unknown from any other temnospondyl: pedicellate teeth and cylindrical pleurocentra in the trunk vertebrae (Bolt, Reference Bolt1977). This was an important step forward because the cylindrical pleurocentra had long been considered a major argument against a temnospondyl relationship of lissamphibians, later upheld by Gardiner (Reference Gardiner1983) who argued for a close relationship among aïstopods, nectrideans, and lissamphibians on this basis. This was one reason why lepospondyls had been seen as the most plausible stem-group of extant amphibians, especially salamanders and caecilians (Romer, Reference Romer1945; Schmalhausen, Reference Schmalhausen1968; Carroll and Currie, Reference Carroll and Currie1975; Carroll and Holmes, Reference Carroll and Holmes1980). However, this diphyletic hypothesis (temnospondyl anurans versus leopospondyl salamanders and caecilians) was never consistent in that anurans share the cylindrical vertebrae with other lissamphibians, but were nevertheless regarded temnospondyl descendants by most authors (Danto et al., Reference Danto, Witzmann and Fröbisch2016).
Later, Bolt (Reference Bolt1977) discovered pedicely also in Amphibamus, but not Platyrhinops, which shares with the two others and lissamphibians tooth crowns with two cusps (bicuspidity). The clade formed by Doleserpeton and Amphibamus was since conceived the most plausible stem-group of Lissamphibia by many authors (Milner, Reference Milner1988, Reference Milner1993; Trueb and Cloutier, Reference Trueb and Cloutier1991; Ruta et al., Reference Ruta, Coates and Quicke2003; Schoch and Milner, Reference Schoch and Milner2004; Ruta and Coates, Reference Ruta and Coates2007), except for those upholding a non-temnospondyl origin for lissamphibians (Laurin and Reisz, Reference Laurin and Reisz1997; Laurin, Reference Laurin1998; Vallin and Laurin, Reference Vallin and Laurin2004; Marjanovic and Laurin, Reference Marjanovic and Laurin2008, Reference Marjanovic and Laurin2009, Reference Marjanovic and Laurin2013).
Jenkins and Walsh (Reference Jenkins and Walsh1993) discovered skeletons of a Jurassic stem-caecilian with rudimentary limbs, which they named Eocaecilia micropodia. In the course of studying this interesting stem taxon, Jenkins et al. (Reference Jenkins, Walsh and Carroll2007) and Maddin et al. (Reference Maddin, Jenkins and Anderson2012) discovered numerous plesiomorphic characters in the skeleton of Eocaecilia, most notably the retention of dermal bones in the skull that are lost in extant lissamphibians. This demonstrated that batrachians (salamanders and frogs) and caecilians must have lost these elements convergently.
A further milestone was taken by Anderson et al. (Reference Anderson, Reisz, Scott, Fröbisch and Sumida2008a) who named and described the lower Permian amphibamid Gerobatrachus hottoni from Texas. This taxon retains a full complement of dermal bones in the skull contrasting the reduced set of dermal bones in batrachians. However, these elements cover much smaller areas than in other temnospondyls, giving expanded orbits and highly reduced strut-like palatal elements, much like in salamanders and frogs. At the same time, the cylindrical vertebrae are reduced to 17 presacrals, the lowest count among temnospondyls and just slightly higher than that of batrachians. Because of its possession of a basale commune in the tarsus, which is a feature of salamanders, Gerobatrachus has been considered a stem-batrachian (Anderson et al., Reference Anderson, Reisz, Scott, Fröbisch and Sumida2008a) rather than ancestral to all lissamphibians, which was since found as an alternative placement (Fröbisch and Schoch, Reference Fröbisch and Schoch2009a).
Recently, Pardo et al. (Reference Pardo, Small and Huttenlocker2017) reported a tiny stereospondyl temnospondyl, Chinlestegophis jenkinsi Pardo, Small, and Huttenlocker, Reference Pardo, Small and Huttenlocker2017, which they argued shares derived features with caecilians and Eocaecilia. On this basis, they proposed a new diphyletic origin of lissamphibians, with batrachians having evolved from amphibamid dissorophoids, but caecilians from brachyopoid stereospondyls. This remains an open question that needs an inclusive analysis to be carried out in the future.
At any rate, the discovery of Eocaecilia and Gerobatrachus brought an end to the long-practiced separate treatment of temnospondyls and lissamphibians, because both Eocaecilia and Gerobatrachus retained dermal bones that are not present in any extant lissamphibian, but are well known from temnospondyls. The alternative placements of Gerobatrachus indicate that—given the temnospondyl hypothesis of lissamphibian origin is true—some amphibamids are most probably more closely related to lissamphibians (or batrachians) than others. The Amphibamidae has therefore come increasingly into the focus of studies on the origin of lissamphibians. This is irrespective of whether all extant taxa or only batrachians were derived from amphibamids, a discussion that is still in a state of flux (Anderson, Reference Anderson2008; Pardo et al., Reference Pardo, Small and Huttenlocker2017). Amphibamid relationships within dissorophoids therefore form a central problem that also involves the nature of the Branchiosauridae and the primitive condition of Dissorophoidea as a whole.
Objectives
This study aims to resolve or constrain the following major questions of dissorophoid phylogeny:
What does the large-scale phylogeny of Dissorophoidea look like?
What is the position of the enigmatic taxon Perryella?
Do Micromelerpetidae form a clade and where do they nest?
Do Branchiosauridae form a clade and where do they nest?
What is the relationship of Doleserpeton and Gerobatrachus to Lissamphibia/Batrachia?
What is the most likely evolutionary scenario for the origin of the Dissorophoidea and the origin and early diversification of Lissamphibia/Batrachia?
The olsoniforms, which were studied in detail elsewhere (Schoch, Reference Schoch2012; Maddin et al., Reference Maddin, Fröbisch, Evans and Milner2013; Schoch and Sues, Reference Schoch and Sues2013), are not represented by a complete set of known taxa, but a smaller selection representative of their diversity.
Phylogenetic analysis
Taxon sampling
The goal of the phylogenetic analysis was to cover as wide a range of well-studied dissorophoid taxa as possible, with the focus on the origin and diversification of this clade and the in-group relationships of amphibamids. Other questions have been addressed elsewhere, such as the placement of dissorophoids among temnospondyls (Schoch, Reference Schoch2013) or the evolutionary history of olsoniforms (Schoch, Reference Schoch2012; Holmes et al., Reference Holmes, Berman and Anderson2013; Maddin et al., Reference Maddin, Fröbisch, Evans and Milner2013; Schoch and Sues, Reference Schoch and Sues2013). Although kept relatively large, the number of taxa has been restricted to address the specific questions formulated above. To this end, datasets for amphibamids (Schoch and Rubidge, Reference Schoch and Rubidge2005; Anderson et al., Reference Anderson, Reisz, Scott, Fröbisch and Sumida2008a, Reference Anderson, Henrici, Sumida, Martens and Bermanb; Fröbisch and Reisz, Reference Fröbisch and Reisz2008; Sigurdsen and Bolt, Reference Sigurdsen and Bolt2010), dissorophids (Schoch, Reference Schoch2012; Holmes et al., Reference Holmes, Berman and Anderson2013; Maddin et al., Reference Maddin, Fröbisch, Evans and Milner2013), and branchiosaurids (Schoch and Milner, Reference Schoch and Milner2008; Fröbisch and Schoch, Reference Fröbisch and Schoch2009a) have been combined, recoded in some places (indicated in Supplemental Dataset), and additional new characters were added. Throughout, the data set from which the coding was adopted is cited. In order to elucidate the origin of lissamphibians, three representative stem taxa were chosen, interpreted to reflect the primitive condition, the salientian (stem-anuran) Triadobatrachus, the urodele (stem-caudate) Karaurus, and the apodan (stem-gymnophionan) Eocaecilia, which are also fairly consistent with the primitive condition, as assessed by analysis of character distribution in the crown-groups (Schoch, Reference Schoch2014a). The analysis of the recently reported Chinlestegophis is spared for a separate study in the future.
The following taxa have been included in the phylogenetic analysis.
Outgroup:
Dendrysekos helogenes Steen, Reference Steen1931 (Holmes et al., Reference Holmes, Carroll and Reisz1998; Schoch and Milner, Reference Schoch and Milner2014).
Ingroups:
1. Trimerorhachis insignis Cope, Reference Cope1878 (Case, Reference Case1935; Milner and Schoch, Reference Schoch2013).
2. Sclerocephalus haeuseri Goldfuss, Reference Goldfuss1847 (Boy, Reference Boy1988; Schoch and Witzmann, Reference Schoch and Witzmann2009).
3. Acanthostomatops vorax (Credner, Reference Credner1883) (Boy, Reference Boy1989; Witzmann and Schoch, Reference Witzmann and Schoch2006).
4. Perryella olsoni Carlson, Reference Carlson1987 (Case, Reference Case1935; Ruta and Bolt, Reference Ruta and Bolt2006).
5. Micromelerpeton credneri (Bulman and Whittard, Reference Bulman and Whittard1926) (Boy, Reference Boy1972, Reference Boy1995; Schoch and Milner, Reference Schoch and Milner2014).
6. Limnogyrinus elegans Fritsch, Reference Fritsch1881 (Werneburg, Reference Werneburg1994).
7. Branchierpeton amblystomum (Credner, Reference Credner1881) (Werneburg, Reference Werneburg1991).
8. Eimerisaurus graumanni (Boy, Reference Boy1980) (Boy, Reference Boy2002b).
9. Branchiosaurus salamandroides Fritsch, Reference Fritsch1876 (Boy, Reference Boy1987; Werneburg, Reference Werneburg2012; Schoch and Milner, Reference Schoch and Milner2014).
10. Apateon gracilis (Boy, Reference Boy1987; Werneburg, Reference Werneburg1991; Schoch and Fröbisch, Reference Schoch and Fröbisch2006).
11. Leptorophus tener Schönfeld, Reference Schönfeld1911 (Boy, Reference Boy1986, Reference Boy1987).
12. Schoenfelderpeton prescheri Boy, Reference Boy1986 (Boy, Reference Boy1987).
13. Amphibamus grandiceps (Milner, Reference Milner1982; Bolt, Reference Bolt1969, Reference Bolt1979).
14. Doleserpeton annectens Bolt, Reference Bolt1969 (Bold, 1977; Sigurdsen and Bolt, Reference Sigurdsen and Bolt2010).
15. Gerobatrachus hottoni Anderson et al., Reference Anderson, Reisz, Scott, Fröbisch and Sumida2008a.
16. Georgenthalia clavinasica Anderson et al., Reference Anderson, Henrici, Sumida, Martens and Berman2008b.
17. Eoscopus lockardi Daly, Reference Daly1994.
18. Platyrhinops lyelli (Wyman, Reference Wyman1858) (Carroll, Reference Carroll1964; Clack and Milner, Reference Clack and Milner2010).
19. Micropholis stowi Huxley, Reference Huxley1859 (Broili and Schröder, Reference Broili and Schröder1937; Boy, Reference Boy1985; Schoch and Rubidge, Reference Schoch and Rubidge2005).
20. Tersomius texensis Case, Reference Case1910 (Carroll, Reference Carroll1964; Maddin et al., Reference Maddin, Fröbisch, Evans and Milner2013).
21. Pasawioops mayi Fröbisch and Reisz, Reference Fröbisch and Reisz2008.
22. Fedexia striegeli Berman et al., Reference Berman, Henrici, Brezinski and Kollar2010.
23. Acheloma dunni Polley and Reisz, Reference Polley and Reisz2011.
24. Phonerpeton pricei Dilkes, Reference Dilkes1990.
25. Ecolsonia cutlerensis Berman et al., Reference Berman, Reisz and Eberth1985.
26. Broiliellus texensis Williston, Reference Williston1914 (Carroll, Reference Carroll1964; Schoch, Reference Schoch2012).
27. Dissorophus multicinctus Cope, Reference Cope1895 (Carroll, Reference Carroll1964; DeMar, Reference DeMar1968; Schoch, Reference Schoch2012).
28. Cacops morrisi Reisz et al., Reference Reisz, Schoch and Anderson2009.
29. Conjunctio multidens Carroll, Reference Carroll1964 (Schoch and Sues, Reference Schoch and Sues2013).
30. Triadobatrachus massinoti Piveteau, Reference Piveteau1936 (Rage and Roček, Reference Rage and Roček1989; Ascarrunz et al., Reference Ascarrunz, Rage, Legreneur and Laurin2016).
31. Karaurus sharovi Ivakhnenko, Reference Ivakhnenko1978.
32. Eocaecilia micropodia Jenkins and Walsh, Reference Jenkins and Walsh1993 (Jenkins et al., Reference Jenkins, Walsh and Carroll2007).
Character sampling
Many previously used characters were revised and others excluded because they were uninformative for the present range of taxa. For instance, characters 30, 46, and 47 of Schoch and Rubidge (Reference Schoch and Rubidge2005), and character 51 of Anderson et al. (Reference Anderson, Henrici, Sumida, Martens and Berman2008b) have been omitted because they were found not to be defined clearly enough; otherwise the characters added by Anderson et al. (Reference Anderson, Henrici, Sumida, Martens and Berman2008b) are included in the present in data set, albeit with some modified coding. In addition, I have added 38 characters from other sources, including some newly formulated ones. Altogether, the dataset includes 108 discrete morphological characters sampled from all over the temnospondyl skeleton. The complete list of characters is given in the Supplemental Dataset.
Analysis
The parsimony analysis was performed in TNT 1.5 (available at http://www.cladistics.org/tnt.html) using the New Technology Search option, which generated 10 equally most parsimonious trees (tree length [TL]=282 steps; consistency index [CI]=0.429; retention index [RI]=0.711). Multistate characters (1, 45, 53, 67, 75, 94) were ordered. When these six characters were left unordered, the topology of the strict consensus tree remained the same. Dendrysekos (=Dendrerpeton of many former authors who referred to Holmes et al., Reference Holmes, Carroll and Reisz1998) has served as only outgroup taxon. The non-dissorophoid ingroup taxa are Trimerorhachis (for Dvinosauria), Sclerocephalus (for Eryopiformes), and Acanthostomatops (for Zatracheidae).
Results
The resulting 10 trees produce seven polytomies in the strict consensus. Homoplasies are common. The Dissorophoidea (sensu Schoch and Milner, Reference Schoch and Milner2014), Micromelerpetidae (for spelling, see Schoch and Milner, Reference Schoch and Milner2014), Xerodromes, Olsoniformes, Dissorophidae, and the Amphibamiformes+Lissamphibia clade each form stable nodes (see Bremer support values given in the following tree description). The main instabilities are located: (1) within the higher Micromelerpetidae (Eimerisaurus, Branchierpeton, Limnogyrinus); (2) the Trematopidae (Ecolsonia, Fedexia, and advanced trematopids); (3) the base of the Amphibamidae-Lissamphibia clade (Eoscopus, Platyrhinops, all higher taxa); and (4) the higher Amphibamiformes (phylogenetic definition see next scetion), which encompass an unresolved polytomy between Georgenthalia, Branchiosauridae, Gerobatrachus and Lissamphibia. The following nodes were found in the main analysis of 33 taxa and 108 characters (see also Fig. 1.1):
(1) Rhachitomi. Bremer Support: >6. Within Rhachitomi, and above Trimerorhachis, Sclerocephalus, Acanthostomatops, and all higher taxa form an unresolved polytomy.
(2) Perryella + Dissorophoidea. Bremer Support: 2. Synapomorphies: 9, 21, 38.
(3) Dissorophoidea. Bremer Support: 3. Synapomorphies: 24, 30, 32.
(4) Micromelerpetidae. Bremer Support: 3. Synapomorphies: 40, 74, 77, 84.
(5) Eimerisaurus + Branchierpeton + Limnogyrinus. Bremer Support: 3. Synapomorphies: 5, 7, 38, 51. The relationships among the three genera remain unresolved.
(6) Xerodromes. Bremer Support: 5. Synapomorphies: 18, 53, 88.
(7) Olsoniformes. Bremer Support: >6. Synapomorphies: 16, 19, 20, 28, 29, 35, 60, 90, 92.
(8) Dissorophidae. Bremer Support: 5. Synapomorphies: 4, 10, 54, 57, 59.
(9) Dissorophinae. Bremer Support: 5. Synapomorphies: 12, 22, 30, 61, 65.
(10) Cacopinae. Bremer Support: 4. Synapomorphies: 17, 40, 49, 50, 55.
(11) Trematopidae. Bremer Support: 1. Synapomorphies: 39. This node contains an unresolved polytomy consisting of Ecolsonia + Fedexia + higher Trematopidae.
(12) Phonerpeton + Acheloma. Bremer Support: 3. Synapomorphies: 27, 40, 51.
(13) Amphibamiformes. Bremer Support: 5. Synapomorphies: 11, 12, 75, 93.
(14) Micropholidae. Bremer Support: 1. Synapomorphies: 34, 51.
(15) Pasawioops + Micropholis. Bremer Support: 1. Synapomorphies: 33.
(16) Post-micropholid Amphibamiformes. Bremer Support: >6. Synapomorphies: 6, 40, 86, 91, 101.
(17) Amphibamidae. Bremer Support: 1. Synapomorphies: 71, 89, 102.
(18) Higher Amphibamiformes. Bremer Support: 1. Synapomorphies: 7, 30, 84, 100. This node contains an unresolved polytomy consisting of Georgenthalia + Branchiosauridae + Gerobatrachus + Lissamphibia.
(19) Branchiosauridae. Bremer Support: 3. Synapomorphies: 12, 74, 80. This node contains an unresolved polytomy consisting of Branchiosaurus + Apateoninae + Leptorophinae.
(20) Leptorophinae. Bremer Support: 3. Synapomorphies: 17, 83, 95, 96.
(21) Lissamphibia. Bremer Support: >6. Synapomorphies: 71, 94, 105, 107, 108.
(22) Batrachia. Bremer Support: >6. Synapomorphies: 4, 72, 89, 98, 104, 106.

Figure 1 Phylogeny of dissorophoids as found by present analysis. Strict consensus of ten MPTs, 282 steps, CI=0.429, RI=0.711. (1) Main data set (108 characters); (2) variant analysis, giving slightly better resolved Amphibamiformes (omitting characters 17 and 30).
Variant analyses
Because two of the characters (17, 30) of Schoch and Rubidge’s (Reference Schoch and Rubidge2005) data set were criticized as mutually overlapping or non-independent (Holmes et al., Reference Holmes, Berman and Anderson2013), they were omitted in variant analyses, giving a significantly higher resolution (Fig. 1.2). Exclusion of character 30 alone resulted in four MPTs, whereas omission of character 17 or both characters 17 and 30 likewise resulted in only two MPTs. In both variants, the higher Amphibamiformes were almost completely resolved, with Gerobatrachus + Georgenthalia forming the sister taxon to Eocaecilia + (Karaurus + Triadobatrachus), and in turn with the branchiosaurids forming the sister taxon of the latter clade; at the base of the Amphibamiformes, Eoscopus and Platyrhinops form successive sister taxa of Amphibamidae and the aforementioned clades.
Robustness of nodes
The robustness of nodes and sources of instability were studied by the variant analysis of selected subsets of taxa. When Acanthostomatops is omitted, Dissorophoidea nests with Sclerocephalus rather than Trimerorhachis (seven trees). When Perryella is omitted in addition to Acanthostomatops, the result remains the same (seven trees).
Systematic paleontology
Temnospondyli Zittel, Reference Zittel1888
Rhachitomi Watson, Reference Watson1919, sensu Schoch, Reference Schoch2013
Dissorophoidea Bolt, Reference Bolt1969
Diagnosis
Laterally exposed palatine (LEP) (1); dorsal quadrate process (DQP) (2); stapes directed mostly laterally (24), postparietal four times wider than long (30), posterior projection of vomer absent (32), short preorbital region (51), jugal anteriorly blunt (97); medial quadratojugal process (99).
Occurrence
Moscovian–Induan (Moscovian–Recent, if some or all lissamphibians are dissorophoids).
Definition (node)
The least inclusive clade containing Micromelerpeton credneri and Dissorophus multicinctus (Schoch, Reference Schoch2013).
Diversity
Dissorophoidea in its present form contains 52 genera and 85 species (without Lissamphibia).
Micromelerpetidae Boy, Reference Boy1972
Diagnosis
Basipterygoid joint unossified (74), elevated presacral count (77), and unossified pubis (84); additional characters: lateral line sulci present even in large adults, bony ventral scales with thickened, knob-bearing posterior margin (Boy, Reference Boy1972).
Occurrence
Moscovian–Sakmarian.
Definition (node)
The least inclusive clade containing Micromelerpeton credneri and Branchierpeton amblystomum. Range: Moscovian–Artinskian.
Diversity
Within the frame of the present analysis, Micromelerpetidae encompasses Micromelerpeton (three species), Eimerisaurus (two species), Limnogyrinus (two species), Nyranerpeton milneri Werneburg, Reference Werneburg2012 (not included in analysis), and Branchierpeton (three species).
Xerodromes Schoch and Milner, Reference Schoch and Milner2014
Diagnosis
Squamosal-supratemporal suture shortened by extension of squamosal embayment (18), distance squamosal embayment-orbit foreshortened (53), trunk ribs throughout shorter (88).
Occurrence
Moscovian–Induan (Moscovian–Recent, if some or all lissamphibians are dissorophoids).
Definition (stem)
The most inclusive clade containing Apateon pedestris, Amphibamus grandiceps, and Dissorophus multicinctus, but not Micromelerpeton credneri (Schoch and Milner, Reference Schoch and Milner2014).
Diversity
Xerodromes in its present form contains 48 genera and 76 species (without Lissamphibia).
Amphibamiformes new taxon
Diagnosis
Palatine and ectopterygoid reduced to narrow struts (11), interpterygoid vacuity greatly expanded laterally (12), humerus length:waist ratio 6:10 (75), basioccipital and supraoccipital absent (93).
Occurrence
Moscovian–Induan (Moscovian–Recent, if some or all lissamphibians are dissorophoids).
Etymology
Derived from the genus Amphibamus, which it contains.
Definition (stem)
The most inclusive clade containing Amphibamus grandiceps, but not Dissorophus multicinctus (Schoch and Milner, Reference Schoch and Milner2014).
Diversity
Amphibamiformes in its present form contains 12 genera and 15 species.
Remarks
The new name Amphibamiformes is here proposed for three reasons: (1) it encompasses a much larger group than the family-level name Amphibamidae suggests; in its extreme version, which has become more likely with the discovery of Doleserpeton and Gerobatrachus, it includes the more than 7,846 extant lissamphibian species (www.amphibiaweb.org as of April 18, 2018); (2) it contains the Branchiosauridae, which according to all cladistic analyses so far are retained as a clade; and (3) Amphibamiformes is a more apt sister taxon to Olsoniformes than Amphibamidae, considering that even in its smallest form (without Lissamphibia) it is comparably more speciose than its sister group.
Micropholidae Watson, Reference Watson1919
Diagnosis
Vomer with additional fang pair posterior to midvomerine depression (8), postparietal more than four times as wide as long (30), palatine bordering interpterygoid vacuity along its entire lateral margin (34), preorbital length foreshortened (51).
Occurrence
Sakmarian–Induan.
Definition (node)
The least inclusive clade containing Tersomius texensis and Micropholis stowi. In the present analysis, Micropholidae includes Tersomius, Micropholis, and Pasawioops.
Remarks
This clade, which has been found by some former authors (Schoch and Rubidge, Reference Schoch and Rubidge2005; Sigurdsen and Bolt, Reference Sigurdsen and Bolt2010), probably also contains Rubeostratilia and possibly Plemmyradytes. It is in many respects more plesiomorphic than the Amphibamidae and Branchiosauridae, as discussed below. Eoscopus may be a stem taxon of Micropholidae, sharing the additional vomerine fang pair with the clade.
Amphibamidae Moodie, Reference Moodie1909
Diagnosis
Anterior trunk ribs not widened distally (71); choana medially expanded (89), patch of tiny denticles on vomer replacing tusk pair (102); additional character: squamosal posteriorly shortened, compensated by the quadratojugal.
Occurrence
Moscovian–Artinskian.
Definition (node)
The least inclusive clade containing Amphibamus grandiceps and Doleserpeton annectens.
Remarks
This definition is admittedly very restrictive, confining the family to a minimum of two taxa, but potentially including a still undescribed taxon from the Pennsylvanian Kinney Brick Quarry site in New Mexico (R. Werneburg, personal communication, 2017).
Branchiosauridae Fritsch, Reference Fritsch1879
Diagnosis
Palatine Y-shaped, posterior part separate from maxilla; ectopterygoid completely separate from maxilla; branchial denticles single, without bony base, often with brush-like tips.
Occurrence
Moscovian–Artinskian.
Definition (node)
The least inclusive clade containing Branchiosaurus salamandroides and Schoenfelderpeton prescheri.
Diversity
Branchiosauridae in its largest form contain five genera and 19 species (without Eumicrerpeton, Milnererpeton, and Tungussogyrinus).
Remarks
The incompletely known taxa Milnererpeton huberi (Hunt, Lucas, and Berman, Reference Hunt, Lucas and Berman1996) Tungussogyrinus bergi, and Eumicrerpeton parvum Moodie, Reference Moodie1909 have been omitted, but their possession of branchiosaurid characters makes them likely members of the clade rather than larvae of some other dissorophoid taxa, as has been proposed by former authors (Hunt et al., Reference Hunt, Lucas and Berman1996; Werneburg, Reference Werneburg2009; Milner in Schoch and Milner, Reference Schoch and Milner2014).
Evolution of major dissorophoid clades
The evolution of dissorophoids and their relationship to extant amphibians have been analyzed by numerous authors in the 20th century, with the first detailed account given by Watson (Reference Watson1940), who elucidated the origin of anurans based on the examination of Amphibamus grandiceps. Subsequent seminal studies on dissorophoids were conducted by Carroll (Reference Carroll1964) and Bolt (Reference Bolt1969, Reference Bolt1977, Reference Bolt1979), but these were mostly confined to aspects of character evolution, such as Bolt’s discovery of pedicely in Doleserpeton and Amphibamus. Resting on Parsons Reference Parsons and Williamsand Williams’ (1962, Reference Parsons and Williams1963) findings, Bolt (Reference Bolt1969) proposed a common origin of Lissamphibia from within the dissorophoids. This was the first time that substantial data were provided to link extant amphibians with a single group of Paleozoic tetrapods, after lissamphibians had been considered polyphyletic by authors from different schools of thought (Holmgren, Reference Holmgren1933; Romer, Reference Romer1945; Schmalhausen, Reference Schmalhausen1968). In his thorough reexamination of Amphibamus grandiceps, Bolt (Reference Bolt1979) also considered developmental aspects, and developed a scenario in which r-selection might have driven the origin of lissamphibians.
The first inclusive phylogenetic analyses of dissorophoids, based on cladistic principles, were carried out by Jürgen Boy, initially focused on branchiosaurids and micromelerpetids (Boy, Reference Boy1972), then expanded on dissorophoids as a whole (Boy, Reference Boy1981). This author had studied the ontogeny of dissorophoids and other temnospondyls in unprecedented detail (Boy, Reference Boy1974, Reference Boy1978, Reference Boy1988), and incorporated many of these data into his evolutionary scenarios. Having recognized the monophyletic status of branchiosaurids, he suggested that they either formed a neotenic clade or a group of larval forms whose terrestrial adults simply remained unknown. Boy also clearly distinguished micromelerpetids from branchiosaurids and amphibamids, although he considered the paedomorphic, aquatic micromelerpetids and branchiosaurids as close relatives. He was also the first author who recognized micromelerpetids and branchiosaurids as dissorophoids, after these groups had been neglected in other surveys (Romer, Reference Romer1947; Carroll, Reference Carroll1964; Bolt, Reference Bolt1969).
More inclusive than any previous accounts were Milner’s (Reference Milner1988, Reference Milner1990, Reference Milner1993) analyses and discussions, which also employed scenarios of heterochrony and paleoecology. This author was the first to widen the perspective by including all lissamphibians, finding that their closest relatives among Paleozoic tetrapods were indeed Amphibamus and Doleserpeton as forming successive sister taxa towards the crown, thus synthesizing the variant hypotheses of Watson (Reference Watson1940) and Bolt (Reference Bolt1969). Milner was the first author who knew both the Central European branchiosaurids and the North American terrestrial dissorophoids by first-hand examination and in sufficient detail to find robust support for their relationship, as well as the close relationship between branchiosaurids and lissamphibians (Milner, Reference Milner1993). He therefore went further in developing the first inclusive phylogeny of temnospondyls plus lissamphibians (Milner, Reference Milner1990, Reference Milner1993), and most of his hypotheses have been confirmed by computer-assisted cladistic analyses (Yates Reference Yates and Warrenand Warren, Reference Yates and Warren2000; Ruta and Coates, Reference Ruta and Coates2007; Schoch, Reference Schoch2013).
The first large-scale cladistic analysis of early tetrapods and lissamphibians was performed by Laurin and Reisz (Reference Laurin and Reisz1997), who presented the most radical alternative to Bolt’s (Reference Bolt1969) and Milner’s (Reference Milner1988) Temnospondyl Hypothesis in proposing that all lissamphibians were derived from lysorophians, a small clade within lepospondyl microsaurs. Naturally, this analysis included various dissorophoids and notably Doleserpeton, but omitted branchiosaurids with reference to their then-believed completely immature status. In their Lepospondyl Hypothesis, Laurin and Reisz (Reference Laurin and Reisz1997) employed numerous lepospondyl features shared by sirenid, proteid, and amphiumid salamanders as well as caecilians. The implications of this study were analyzed by Schoch and Milner (Reference Schoch and Milner2004) who argued that the lysorophian-lissamphibian similarities constitute convergences produced by repeated events of neoteny (salamanders) and burrowing (caecilians). Although this new perspective was highly stimulating for further research, it primarily failed to explain the close resemblance particularly of anurans with temnospondyls, such as pedicely, the auditory apparatus, and various characters in the palate (Ruta and Coates, Reference Ruta and Coates2007; Sigurdsen and Green, Reference Sigurdsen and Green2011)—despite efforts to surmount these problems (Laurin, Reference Laurin1998; Vallin and Laurin, Reference Vallin and Laurin2004; Marjanovic and Laurin, Reference Marjanovic and Laurin2008). At any rate, this intensified phylogenetic controversy had an enormous stimulating influence on the description and revision of dissorophoid taxa.
The last decade has brought various new and increasingly refined phylogenetic studies of dissorophoids, triggered by the examination of long-known taxa (Micropholis: Schoch and Rubidge, Reference Schoch and Rubidge2005; Cacops: Reisz et al., Reference Reisz, Schoch and Anderson2009; Perryella: Ruta and Bolt, Reference Ruta and Bolt2006; Doleserpeton: Sigurdsen and Bolt, Reference Sigurdsen and Bolt2010; Mordex: Werneburg, Reference Werneburg2012; Tersomius: Maddin et al., Reference Maddin, Fröbisch, Evans and Milner2013) and the discovery of new species and genera (Eimerisaurus: Boy, Reference Boy2002b; Plemmyradytes: Huttenlocker et al., Reference Huttenlocker, Pardo and Small2007; Pasawioops: Fröbisch and Reisz, Reference Fröbisch and Reisz2008; Rubeostratilia: Bourget and Anderson, Reference Bourget and Anderson2011; Nyranerpeton: Werneburg, Reference Werneburg2012; Reiszerpeton: Maddin et al., Reference Maddin, Fröbisch, Evans and Milner2013; Scapanops: Schoch and Sues, Reference Schoch and Sues2013). These studies provide a much broader platform of taxa, characters, and hypotheses, which will form the basis of the following discussion.
The origin of the Dissorophoidea
Dissorophoids must have evolved well before the Westphalian D (Moscovian: 307–315 Myr), because at that time the major clades were already established (Schoch and Milner, Reference Schoch and Milner2014). Morphologically, there is a considerable gap between dissorophoids and other temnospondyls. Departing from the primitive condition (edopoids, Dendrysekos, Balanerpeton), dissorophoids or certain taxa among Xerodromes with plesiomorphic traits (basal amphibamiforms, basal trematopids) have a short preorbital region, a longer postorbital skull table with elongate rectangular supratemporals, and enlarged orbits in adults, which give most taxa a juvenile appearance.
Most importantly, the enlargement of the orbits, which separated the jugal and lacrimal, correlates a dorsal expansion of the palatine (LEP), a structural innovation that evolved convergently but in a rather different morphological framework within the higher dvinosaurians (Shishkin, Reference Shishkin1973). In dvinosaurians, the orbits were not enlarged, but shifted to the side, and only in later Permian–Triassic taxa an LEP was established, whereas the lower Permian forms retained a regular jugal-lacrimal contact.
A second consistent character, the dorsal quadrate process, has been argued to have evolved in the enlarged tympanic notch of dissorophoids (Bolt and Lombard, Reference Bolt and Lombard1985). In the palate, the interpterygoid vacuities expanded even more substantially than the orbits, pushing the pterygoid, palatine, and ectopterygoid to the margin (Watson, Reference Watson1940; Carroll, Reference Carroll1964). This probably correlated with enlarged eye musculature (Witzmann and Werneburg, Reference Witzmann and Werneburg2017). The pectoral girdle of dissorophoids is much lighter built and the limb bones are more slender and well ossified compared to in most other temnospondyls (Carroll, Reference Carroll1964; DeMar, Reference DeMar1968; Boy, Reference Boy1972; Daly, Reference Daly1994), although the distal end of the humerus has a modified condyle, which is still not widely known but may be another synapomorphy of dissorophoids and lissamphibians (Sigurdsen and Bolt, Reference Sigurdsen and Bolt2009).
The placement of the Dissorophoidea within the large-scale phylogeny of temnospondyls remains controversial. According to the existing hypotheses, the immediate sister taxon of dissorophoids may either be: (1) the zatracheids (Schoch, Reference Schoch1997, Reference Schoch2013; Holmes et al., Reference Holmes, Carroll and Reisz1998), (2) some or all eryopiforms (Milner, Reference Milner1990; Yates Reference Yates and Warrenand Warren, Reference Yates and Warren2000), or (3) the dvinosaurians (Ruta and Bolt, Reference Ruta and Bolt2006, albeit with Dendrysekos interpreted as basal dissorophoid). In an inclusive cladistic analysis, Schoch (Reference Schoch2013) found: (1) Dvinosauria; and (2) Zatracheidae to form successive sister taxa of Dissorophoidea, although only the support for Zatracheidae was robust. The implications of a dvinosaurian-zatracheid-dissorophoid clade would leave a somewhat paradoxical situation: features shared by dvinosaurians and basal dissorophoids, such as the long posterior skull table and the laterally placed orbits, would have to be reversed in zatracheids, but these appear to have retained the primitive condition exemplified by eryopiforms. Additional shared derived characters, such as the LEP, are as mentioned before not strictly similar in dvinosaurians and dissorophoids, do not occur in zatracheids, and evolved only late in the dvinosaurian lineage, because basal taxa (Trimerorhachis, Neldasaurus, and Erpetosaurus) do not have these features (Milner and Schoch, Reference Schoch2013; Schoch, Reference Schoch2018), whereas they are present even in the basal-most dissorophoids. In turn, the character-states shared by zatracheids and dissorophoids do not require reversals within dissorophoids, and otherwise do not appear anywhere in temnospondyls (see next section). Therefore, I regard the relationship between dvinosaurians and dissorophoids as unsettled, whereas the likelihood for a zatracheid-dissorophoid clade as relatively high, as measured by the number of synapomorphies shared by the two.
In line with the aforementioned evidence, the present analysis finds that zatracheids form an unresolved trichotomy with dissorophoids and eryopiforms. Dvinosaurians are found outside this clade in all variant analyses. Interestingly, the omission of zatracheids (represented by Acanthostomatops) results in a closer placement of eryopiforms with dissorophoids rather than dvinosaurians. By phylogenetic definition, taxa below Micromelerpetidae fall outside Disorophoidea (Schoch and Milner, Reference Schoch and Milner2014), which puts Perryella on a grade outside this taxon.
Zatracheidae as plausible sister group
The lower Permian Zatracheidae encompasses only three genera: Acanthostomatops (Saxony, Germany), Zatrachys (Texas, New Mexico, Oklahoma, and Virginia), and Dasyceps (Texas, England, and Czech Republic). In their examination of the basal taxon Acanthostomatops, Boy (Reference Boy1989) and Witzmann and Schoch (Reference Witzmann and Schoch2006) reported most developmental changes to be consistent with eryopiforms, whereas certain morphological features are shared with dissorophoids. These include the small squarish interclavicle, slender clavicles, the wide squamosal embayment, and the intervomerine and internarial fontanelles. The shared character-states are consistent with the observation that the ontogeny of both Acanthostomatops and many dissorophoids involved some metamorphosis, as outlined by Schoch (Reference Schoch2009). As in most dissorophoids, zatracheids probably had fully terrestrial adults, whereas the juveniles were gill-bearing larvae similar to those of eryopiforms and the basal dissorophoid Micromelerpeton (Boy, Reference Boy1988, Reference Boy1989, Reference Boy1990; Schoch, Reference Schoch2003; Witzmann and Pfretzschner, Reference Witzmann and Pfretzschner2003; Witzmann and Schoch, Reference Witzmann and Schoch2006). In Acanthostomatops, metamorphosis was more gradual and stretched-out than in the branchiosaurid Apateon gracilis, which underwent a drastic transformation that is more similar to, but less pronounced than that of extant lissamphibians (Schoch and Fröbisch, Reference Schoch and Fröbisch2006). The light-built pectoral girdle, stout humerus, and the absence of lateral line sulci indicate that adults of zatracheids were terrestrial, and the remodeling of the hyobranchium as well as shortening of the trunk and extreme broadening of the skull during metamorphosis (Witzmann and Schoch, Reference Witzmann and Schoch2006), which are unique features of the clade, are quite consistent with that. Zatracheids evidently underwent some substantial separate evolution since their ancestors diverged from those of dissorophoids in the early Pennsylvanian.
Perryella as basal taxon
The small temnospondyl Perryella olsoni, from the lower Permian of Oklahoma, was first described by Carlson (Reference Carlson1987) who considered it incertae sedis, and later referred it to the Dissorophoidea by Milner (Reference Milner1990). Ruta and Bolt (Reference Ruta and Bolt2006) revised the taxon and provided a masterly detailed description. Schoch and Milner (Reference Schoch and Milner2014) considered Perryella an “amphibamid” (amphibamiform in the present framework), based especially on the narrow ectopterygoid and short palatine ramus of the pterygoid; these features are not well exposed, however. Perryella instead forms a plausible candidate for a basal dissorophoid, sharing several plesiomorphies with micromelerpetids. These include the elongate posterior skull table, with supratemporal and squamosal having a long suture, despite a substantial squamosal embayment. The orbits are markedly smaller than in dissorophoids, although the general appearance of the skull roof is similar to large specimens of Micromelerpeton and Eimerisaurus. As in large Micromelerpeton and Limnogyrinus, the postorbital is elongate and posteriorly pointed. The palatal bones bear numerous small teeth, and the parasphenoid is covered by a long denticle field on the parasphenoid. The basal plate is large, and the foramina for the internal carotid artery are placed lateral to the denticle field, which is close to micromelerpetids and amphibamiforms. Moreover, the wide jugal, the supinator, and the relatively large quadrangular interclavicle are plesiomorphic features consistent with the primitve condition of dissorophoids.
Characters shared by Perryella and Dissorophoidea are the anteriorly reduced palatine ramus and the lost prefrontal-postfrontal contact, admittedly two features known to have evolved in parallel repeatedly within xerodromes, as well. Due to its more elaborate squamosal embayment and the aforementioned characters, Perryella appears to nest outside Dissorophoidea sensu stricto, which makes it an interesting taxon to study the stepwise acquisition of dissorophoid characters. The three most important features of this clade, LEP, DQP, and medial quadratojugal process (MQP), were well established.
Micromelerpetidae
This clade of small paedomorphic dissorophoids, known since Credner’s (Reference Credner1881, Reference Credner1886) reports on the Saxon lower Permian deposits, had long been lumped together with true branchiosaurids and larvae of seymourimorphs under the name “branchiosaurs” (Fig. 3). Micromelerpetids were first recognized as distinct from true branchiosaurids by Bulman and Whittard (Reference Bulman and Whittard1926), and their morphology was described in detail by Boy (Reference Boy1972, Reference Boy1995, Reference Boy2002a, Reference Boyb) and Werneburg (Reference Werneburg1988, Reference Werneburg1991, Reference Werneburg1994). They are mostly represented by larval or immature specimens, which co-occur with the smaller branchiosaurids and larvae of eryopiforms (Sclerocephalus, Onchiodon, Actinodon) in Central European lake deposits of lower Permian age (Boy and Sues, Reference Boy and Sues2000). Their possession of an LEP and other dissorophoid characters was discovered rather late after larger adult specimens had been found, (Branchierpeton: Werneburg, Reference Werneburg1991; Micromelerpeton: Boy, Reference Boy1995), which clearly demonstrated their dissorophoid affinities. Unlike branchiosaurids, micromelerpetids had elongate bodies with 26–29 presacral vertebrae, elongate ribs, well-established lateral line sulci, and a larger quadrangular interclavicle. These features indicate aquatic locomotion and sensation, suggesting the clade formed an early aquatic dissorophoid offshoot. However, it remains an open question whether all taxa of this clade led an aquatic adult life, as large specimens of Micromelerpeton credneri lack lateral line sulci and have enlarged eyes, indicated by the sclerotic ring (Boy, Reference Boy1995; F. Witzmann, personal communication, 2018).
Bulman and Whittard (Reference Bulman and Whittard1926) only knew small adults of Micromelerpeton from the Odernheim lake deposit in Germany, whose adult skull length was in the 20–25 mm range. After new excavations had been carried out in stratigraphically older formations, Boy (Reference Boy1995) reported much larger specimens, which had proportionately longer skulls (45 mm), with expanded posterior skull tables and polygonal ornamentation on the dermal bones (Schoch and Milner, Reference Schoch and Milner2014, fig. 27A). This morphology is consistent in many features with Perryella, which is nonetheless found to nest at the base of Dissorophoidea rather than with micromelerpetids.
Branchierpeton, which was confined to the eastern part of Germany, is known from a stratigraphic sequence of species that probably spanned the Pennsylvanian-Permian boundary (Werneburg, Reference Werneburg1989, Reference Werneburg1991). In that series, the most ancient species, B. saalense Werneburg, Reference Werneburg1990, has a slightly narrower skull with a longer postorbital skull table, which resembles that of Eimerisaurus and Micromelerpeton. This pattern is consistent with the present phylogeny, which contradicts the traditional interpretation of Werneburg (Reference Werneburg1989), Milner (Reference Milner1993), and Boy (Reference Boy2002a) in which Limnogyrinus was the basal taxon and Micromelerpeton the most advanced. These early phylogenies were based on a few characters only, and in this case the prefrontal-postfrontal contact in Limnogyrinus was given much weight. Yet the frequent reversals of this character, as exemplified by Perryella, trematopids, branchiosaurids, and amphibamiforms, cast doubt on referring exclusively to this feature. Instead, Limnogyrinus and its close relative Nyranerpeton (Werneburg, Reference Werneburg2012), are similar in most features to Branchierpeton, and the phylogenetic sequence suggested by the present analysis may be read as a trend towards stronger paedomorphosis. This trend also can be traced within single lineages, such as Branchierpeton (B. saalense–B. reinholdi Werneburg, Reference Werneburg1988–B. amblystomum of Werneburg, Reference Werneburg1989) and stratigraphically arranged morphotypes of Micromelerpeton credneri (Niederkirchen–Odernheim–Humberg of Boy, Reference Boy1995). The ancient micromelerpetid is thus likely to have looked more like the large, long-skulled Micromelerpeton credneri from late Niederkirchen than more paedomorphic Limnogyrinus. However, character conflicts cannot be resolved because Limnogyrinus has a lower presacral vertebral count than the other taxa, which appears more plesiomorphic.
Micromelerpetids form a clade of early dissorophoids, which successfully explored larger, nutrition-rich lakes by extending the larval period or abandoning metamorphosis (neoteny). Their larvae were less evolved than those of true branchiosaurids (Witzmann and Pfretzschner, Reference Witzmann and Pfretzschner2003), which had specialized marginal teeth, branchial dentition, and often retained an open cheek well within late larval stages (Boy, Reference Boy1987; Schoch and Milner, Reference Schoch and Milner2008). In micromelerpetids, metamorphosis was not as drastic as in branchiosaurids and neotenic specimens are less clearly distinguished from metamorphosed ones (Schoch, Reference Schoch2009). As indicated by the well-known larvae of eryopiforms, micromelerpetid larvae are likely to form the primitive condition for dissorophoids, a point to which I shall return to below when discussing branchiosaurids.
Xerodromes
Xerodromes form the higher, post-micromelerpetid dissorophoids, which share elongate limb bones, short trunk ribs, and light-built pectoral girdles with a small interclavicle (Fig. 4). Many xerodromes have a disproportionately wide skull, but not necessarily an extended interorbital distance. The postfrontal, postorbital, jugal, and squamosal are the main elements affected by this widening, contrasting markedly with the condition in micromelerpetids, which does not share these features. What is more, the orbits are proportionately larger than in micromelerpetids and the enlarged squamosal embayment wedges deeply between the squamosal and supratemporal, extending onto a large, rounded, unornamented area—this is found in taxa as different as Dissorophus, Platyrhinops, Apateon, and Doleserpeton; the trematopid squamosal embayment is to be regarded secondarily modified (Schoch, Reference Schoch2012).
The ancestral xerodrome morphology is not readily assessed because basal taxa are unknown and both olsoniforms and amphibamiforms fall into quite disparate groups with substantial numbers of autapomorphies (Maddin et al., Reference Maddin, Fröbisch, Evans and Milner2013; Schoch and Milner, Reference Schoch and Milner2014). Among those representatives with the largest number of plesiomorphies are the amphibamiforms Tersomius, Eoscopus, and Platyrhinops and the olsoniforms Ecolsonia, Mordex, and Phonerpeton. These share with micromelerpetids the elongate postorbital skull table (supratemporals long rectangular, also in Broiliellus) and a posteriorly extended basal plate of the parasphenoid. The palatal elements were covered almost throughout with denticles, as retained in Platyrhinops, Phonerpeton, and Cacops. The pterygoid reached far anterior to contact the vomer, as exemplified by Phonerpeton, Acheloma, and Ecolsonia. Reduction of the anterior portion of the palatine ramus appears to have occurred in parallel in amphibamiforms and dissorophids, also because the resulting morphology of the palatine ramus differs: in amphibamiforms pushed laterally, often contacting only the ectopterygoid, and in dissorophids aligned anteriorly, with the palatine firmly sutured to the blunt anterior end of the pterygoid. Ancient xerodromes appear to have had well-sutured prefrontal and postfrontal, a feature retained in Platyrhinops and Amphibamus, as well as Mordex.
Adult xerodromes were apparently advanced over micromelerpetids in having a more definitive terrestrial existence; the two only known clades represent alternative evolutionary strategies to enable enhanced terrestriality: amphibamiforms became small salamander-like insectivores that eventually developed bicuspid pedicellate teeth, whereas olsoniforms evolved into larger predators with enlarged fangs and heavy dermal armor in some forms, as the only dissorophoids to reach sizes around a meter. In both clades, ossification of the braincase and axial skeleton was reinforced as compared with outgroups, with amphibamiforms evolving the cylindrical pleurocentrum, whereas olsoniforms strengthened the intercentrum instead and evolved uncinate processes on their ribs for further reinforcement (Bolt, Reference Bolt1969; Berman et al., Reference Berman, Reisz and Eberth1985; Daly, Reference Daly1994). The larval life of xerodromes is only known from branchiosaurids, which evolved neoteny and specialized larval characters; the question how widespread branchiosaurid larval features were in post-micromelerpetid taxa remains controversial. Milner (Reference Milner2007) and Werneburg (Reference Werneburg2012) reported branchiosaurid-like larvae in Mordex, but these specimens are more likely to fall within the population of Branchiosaurus salamandroides after reexamination. At any rate, the lissamphibian characters evolved mostly within amphibamiforms, with xerodromes only sharing the shortened ribs, light-built dermal pectoral girdle, and the tendency to form a wide skull with modern amphibians—features that were much accentuated in later amphibamiform evolution.
Olsoniformes
The monophyly of Trematopidae and Dissorophidae had long been questioned (Milner, Reference Milner1993), but was eventually confirmed after reexamination of postcranial characters and the description of much better preserved skull material in both groups (Reisz et al., Reference Reisz, Schoch and Anderson2009; Polley and Reisz, Reference Polley and Reisz2011; Schoch, Reference Schoch2012; Maddin et al., Reference Maddin, Fröbisch, Evans and Milner2013; Schoch and Sues, Reference Schoch and Sues2013). The present analysis confirms the placement and monophyletic status of the Olsoniformes, a clade that was named by Anderson et al. (Reference Anderson, Henrici, Sumida, Martens and Berman2008b). A detailed discussion of olsoniform relationships and their monophyly was given by Schoch (Reference Schoch2012).
Amphibamiformes
This clade is rather uncontroversial and has so far mostly been referred to as Amphibamidae (Schoch and Rubidge, Reference Schoch and Rubidge2005; Huttenlocker et al., Reference Huttenlocker, Pardo and Small2007; Fröbisch and Reisz, Reference Fröbisch and Reisz2008; Bouget and Anderson, 2011; Maddin et al., Reference Maddin, Fröbisch, Evans and Milner2013; Schoch and Milner, Reference Schoch and Milner2014). Basal amphibamiforms as found in the present study (Platyrhinops, Eoscopus) have rather long presacral vertebral columns in which the pleurocentra reach somewhat farther ventral than in Micromelerpeton, as Daly (Reference Daly1994) has shown. The interclavicle of these forms is quadrangular like in micromelerpetids, but much smaller compared with the skull or vertebral column. In the trunk, the ribs are shorter than in any other group (including olsoniforms), but the first five thoracic ribs are still substantially longer and more robust than the following ones. Basal amphibamiform skulls are heavily ornamented with many small polygonal ridges, and the circumorbital elements remain relatively large with well-established sutures. The posterior skull table houses elongate supratemporals as in outgroups, but the squamosal embayment is huge, and the distance between orbits and squamosal embayment is minimized but usually strengthened by ornament.
There are two alternative phylogenetic hypotheses found by the present analysis that produce equally plausible evolutionary scenarios: (1) Platyrhinops and Eoscopus form basal taxa that branched off before micropholids and higher amphibamiforms diverged, or (2) Platyrhinops nests at the base of Amphibamidae and Eoscopus at the base of Micropholidae. Because the palate of Platyrhinops retains many plesiomorphies (wide pterygoid, palatine and ectopterygoid, vomer completely covered by denticles, basal plate), this taxon has a rather ancestral appearance. On the other hand, the postorbital skull table is not as elongate as in Micropholidae and Eoscopus, which appear more plesiomorphic in this respect. In addition, the squamosal embayment is medially expanded much like in Amphibamidae sensu stricto, resulting in a laterally restricted supratemporal and tabular. Eoscopus, on the other hand, has a very wide skull due to enormously enlarged orbits, which resembles that of Georgenthalia, branchiosaurids, and especially Gerobatrachus; but these are probably convergences, as indicated by multiple lines of conflicting evidence. On the other hand, Eoscopus shares only plesiomorphies with micropholids, such as the morphology of the basal plate and posterior skull table, and with Platyrhinops it is consistent in the completely dentigerous vomer, a feature also retained in Doleserpeton and Amphibamus.
Branchiosauridae
Over decades, the main “branchiosaur” question was: Why are branchiosaurids not simply the larvae of other amphibamiforms? The short answer to this question is: because adult branchiosaurids are known, and they differ from all other dissorophoids. Adult metamorphosed specimens were described by Werneburg (Reference Werneburg1991) and Schoch and Fröbisch (Reference Schoch and Fröbisch2006), who found that during metamorphosis the main branchiosaurid synapomorphies were conserved, and the adult morphology was unlike that of Amphibamus or Doleserpeton. Instead, branchiosaurids share derived features with Gerobatrachus, such as the morphology of the pterygoid and vomer, and the lack of tooth patches on these bones. These features were formerly referred to immaturity, but their presence in adult branchiosaurids, as well as their absence even in immature specimens of Platyrhinops (Clack and Milner, Reference Clack and Milner2010) and Amphibamus (Milner, Reference Milner1982) indicate that these features were independent of developmental changes.
First recognized by Fritsch (Reference Fritsch1879) as a distinct group, then described in detail by Credner (Reference Credner1881, Reference Credner1886), the Branchiosauridae were eventually identified as a separate clade by Boy (Reference Boy1972). This was an important step beyond the confusion that existed since Romer (Reference Romer1939, Reference Romer1947) had proposed them as larvae of eryopiform temnospondyls. Boy (Reference Boy1971) first suggested that branchiosaurids were not simply larvae of other clades, but that in most branchiosaurid species, adults retained a larval morphology, and concluded by analogy with extant salamanders that they were neotenic forms. Boy (Reference Boy1987) and Werneburg (Reference Werneburg1991) reported adult specimens in one species, Apateon gracilis, which were apparently metamorphosed adults. Whereas Boy (Reference Boy1987, p. 76) referred to them as “matured,” Werneburg (Reference Werneburg1991) highlighted their metamorphosed (transformed) appearance. The different developmental pathways of branchiosaurid ontogenies were then studied in more detail by Schoch and Fröbisch (Reference Schoch and Fröbisch2006: metamorphosis) and Fröbisch and Schoch (Reference Fröbisch and Schoch2009b: neoteny). Finally, Sanchez et al. (Reference Sanchez, De Ricqles, Schoch and Steyer2010) were able to confirm the neoteny hypothesis on the basis of skeletochronological and paleohistological data, revealing that sexual maturity was reached in animals retaining a larval morphology. The Branchiosauridae, as defined by Boy (Reference Boy1987) on the basis of synapomorphies, were first found by a cladistic analysis of Schoch and Milner (Reference Schoch and Milner2008) and later, in the somewhat extended analyses of Fröbisch and Schoch (Reference Fröbisch and Schoch2009a), they were found as a clade, nesting within the amphibamiforms. The present analysis confirms this hypothesis, based on a much larger set of taxa and characters.
Branchiosaurids have a wide, abbreviated basal plate much like in Amphibamidae, but in contrast to these it lacks a denticle field. Consequently, the internal carotid foramina are located more medially and are more closely set, whereas they are located much farther laterally in larval Platyrhinops and Amphibamidae, where the denticle field pushes them far away from the central part of the basal plate. Furthermore, branchiosaurids all share a wide, Y-shaped palatine, which is present throughout ontogeny and not found in other dissorophoids (Boy, Reference Boy1972, Reference Boy1987; Werneburg, Reference Werneburg1987, Reference Werneburg1989, Reference Werneburg1991, Reference Werneburg2001; Schoch, Reference Schoch1992). The broad lateral wing of the palatine forms the only contact of the palatine with the maxilla, and the ectopterygoid is completely separate from the maxilla. Consequently, the interpterygoid vacuities are narrower than in other amphibamiforms, resembling those of trematopids and micromelerpetids. The palatine ramus of the pterygoid reaches much farther anteriorly than in most other amphibamiforms, which is quite possibly a derived feature that lends more strength to the otherwise strongly reduced palate. Again, this palatal architecture does not only characterize branchiosaurid larvae and neotenes, but also metamorphosed adults, as exemplified by Apateon gracilis (Schoch and Fröbisch, Reference Schoch and Fröbisch2006).
Milner (Reference Milner1982) suggested that adult Amphibamus developed from a branchiosaurid larva, after he had described larval specimens from Mazon Creek originally reported by Moodie (Reference Moodie1909) and Gregory (Reference Gregory1950). Some of the early larvae he referred to Branchiosauridae proper (Branchiosaurus), others to Amphibamus. These specimens, although potentially of high significance, are challenging because of poor preservation. My own examination of this material, especially Eumicrerpeton parvum, has made me doubtful of this conclusion. The large specimen of Eumicrerpeton (USNM 4400; Schoch and Milner, Reference Schoch and Milner2014) clearly has a branchiosaurid palate architecture, and development into adult Amphibamus is conceivable only when some kind of resorption and reorientation of the palatine, ectopterygoid, and pterygoid had taken place. Although resorption and reorientation of palatal elements is well known in salamanders and probably forms the primitive condition of the clade (Lebedkina, Reference Lebedkina1979; Schoch, Reference Schoch1998), the ontogenetic changes of the palate in branchiosaurid metamorphosis were minimal, consisting of a broadening of the palatine, but no reorientation; the palatine ramus of the pterygoid was as long as in larvae, contacting most of the palatine medially.
In the present analysis, the genera Tungussogyrinus (?late Permian of Tunguska region, central Siberia) and Milnererpeton (Pennsylvanian of New Mexico) were omitted because they are not sufficiently known to be placed with reasonable confidence (Hunt et al., Reference Hunt, Lucas and Berman1996; Shishkin, Reference Shishkin1998; Schoch and Milner, Reference Schoch and Milner2008). This said, both Tungussogyrinus and Milnererpeton are now known to share the Y-shaped palatine and free ectopterygoid (Werneburg, Reference Werneburg2009; Werneburg et al., Reference Werneburg, Schneider and Lucas2013) with Branchiosauridae. Both taxa also share the single branchial denticles with Branchiosauridae, first reported by Credner (Reference Credner1881), restored by Stadtmüller (Reference Stadtmüller1936), and described in detail by Boy (Reference Boy1972). These form a modification of the plesiomorphic branchial dentition, which consists of small bony plates located on the branchial arches, bearing rows or patches of teeth, occurring in temnospondyl outgroups (eryopiforms, dvinosaurians), colosteid stem-tetrapods (Hook, Reference Hook1983; Witzmann, Reference Witzmann2013), and many osteichthyans. In branchiosaurids, the bony plates were lost and the single teeth were much thinner and often had brush-like tips, which has been interpreted as a filter-feeding device (Boy, Reference Boy1972). This character forms a larval synapomorphy of branchiosaurids (Boy, Reference Boy1987), but, unlike the palatal features, their distribution cannot be traced well within Dissorophoidea because unequivocal larval specimens are still only known from micromelerpetids and branchiosaurids (Boy, Reference Boy1974; Schoch, Reference Schoch2009). Single branchial denticles were also mentioned by Milner (Reference Milner1982) to occur in larval Amphibamus. Branchiosaurids may thus have shared the branchial denticles with some or all amphibamiforms, and they are also known to occur in salamandroid caudate Beiyanerpeton from the Late Jurassic of Liaoning, China (Gao and Shubin, Reference Gao and Shubin2012) and in the Paleogene caudate Seminobatrachus (Skutschas and Gubin, Reference Skutschas and Gubin2012). The distribution of this character indicates that the single denticles were probably more widespread among dissorophoids, potentially occurring throughout amphibamiforms or even xerodromes.
Amphibamidae proper
The two genera Doleserpeton and Amphibamus share a range of derived characters (Fig. 5), among which are the posteriorly foreshortened squamosal (compensated for by the quadratojugal), the loss of palatine and ectopterygoid tusks, extreme reduction in the width of the ectopterygoid, and reduction of the tabular to a tiny element without distinct horn. In all these features, amphibamids are more derived than branchiosaurids, but not necessarily more similar to lissamphibians. Conversely, the palatal denticles, which cover the complete vomer, pterygoid, palatine, and large areas of the parasphenoid, are a consistent feature of Amphibamus and Doleserpeton, and even found in juveniles much smaller in size than branchiosaurids. In these features, amphibamids are consistent with Platyrhinops, which developed a fully dentigerous palate early in ontogeny (Clack and Milner, Reference Clack and Milner2010). The small larval specimens referred to Amphibamus by Milner (Reference Milner1982) indeed have a triangular denticle field on the parasphenoid, which does not occur in branchiosaurids, and the tabular is tiny as in adults; the palatal elements are too poorly preserved to show whether the palatine was Y-shaped or not. Within Amphibamidae, the number of presacral vertebrae was reduced from 24 (Doleserpeton) to 18 (Amphibamus), a trend that was paralleled within Micropholidae (Micropholis having 19) and Branchiosauridae (22–19), where the presacral count is particularly variable within species (Boy, Reference Boy1972). A strict identification of small larvae on the basis of presacral numbers alone, as attempted by Werneburg (Reference Werneburg2012) to distinguish taxa, may therefore be misleading. Amphibamids were more lightly built than branchiosaurids, which is also apparent in the more delicate anterior trunk ribs in the former, as well as the less-intense ornament on the dermal skull bones. At the same time, branchiosaurids contrast amphibamids in the more-reduced areas occupied by circumorbital elements, a derived character shared with Georgenthalia and Gerobatrachus. Furthermore, amphibamids are also plesiomorphic in the retention of dentigerous palatal ossicles, which is consistent with the presence of extensive tooth patches on the vomer and palatine, whereas such ossicles are absent even in large adult branchiosaurids.
Georgenthalia and Branchiosauridae
The incompletely known small amphibamiform Georgenthalia clavinasica shares a few characters with branchiosaurids. Although the exposed palate is more consistent with micropholids and amphibamids proper, the skull roof closely resembles that of large specimens of Branchiosaurus and Apateon, having large orbits with correlated broad postorbitals, and a much shortened posterior skull table. Also shared with branchiosaurids is the retention of substantial tabular horns. Also alternatives are equally parsimonious, Georgenthalia may form a plausible sister taxon to Branchiosauridae, in which the palatal architecture of branchiosaurids was not yet established.
Origin of lissamphibians
The discovery of Doleserpeton, Eocaecilia, and Gerobatrachus has added substantial data to the controversy over the origin of lissamphibians, yielding some unexpected information on character evolution before and during the early diversification of lissamphibians, or at least batrachians.
Doleserpeton was essential in demonstrating that two lissamphibian characters evolved within amphibamiforms: pedicely and cylindrical pleurocentra, which gave rise to the single cylindrical centrum in Lissamphibia (Bolt, Reference Bolt1969). A third feature, the absence of the ectopterygoid, is now controversial: it was proposed by Bolt (Reference Bolt1969) and retained in the new reconstruction of Sigurdsen and Bolt (Reference Sigurdsen and Bolt2010), whereas a tiny strut-like ectopterygoid was figured in the same paper (Sigurdsen and Bolt, Reference Sigurdsen and Bolt2010, fig. 2). This is also a problematic character, because Gerobatrachus, which is closer to lissamphibians in a full range of characters, retains quite a substantial ectopterygoid. The only shared character-state of amphibamids and Gerobatrachus is the absence of teeth on the ectopterygoid, a feature that also evolved in parallel within branchiosaurids, namely the leptorophine genera Melanerpeton, Leptorophus, and Schoenfelderpeton (Boy, Reference Boy1987).
Pedicely and bicuspidity were later also found in Amphibamus (Bolt, Reference Bolt1979) and some ontogenetic stages of Tersomius (Bolt, Reference Bolt1977; Fig. 6). The existence of fused pedicels in the branchiosaurid Apateon pedestris remains unclear (Boy, Reference Boy1978), whereas the report of pedicely in adults of the same taxon by Schoch and Carroll (Reference Schoch and Carroll2003) may be caused by breaks produced by an underlying mandible. Even in Gerobatrachus, pedicely remains controversial; the maxilla teeth of this taxon were shown to have a narrow zone that may have formed by the fusion of pedicellate crowns with their bases (Anderson et al., Reference Anderson, Reisz, Scott, Fröbisch and Sumida2008a).
According to the evidence from Doleserpeton and Tersomius, pedicely originated in juveniles, after the peg-like larval dentition had been replaced and before fully mineralized, labyrinthodont teeth were produced (Bolt, Reference Bolt1977, Reference Bolt1979). It is conceivable that this stage was not always established, or preserved, because it may have been confined to brief developmental phases. Unlike in many other morphological features, pedicely is a condition that may be derived by incomplete mineralization of a tooth, resulting from the existence of two separate centers of mineralization (Vasilieva and Smirnov, Reference Vasilieva and Smirnov2001). Thus, it may well have been absent as a discrete morphology in some stem taxa, whereas the mechanism of tooth production inherently carried pedicely as an option. Eventually it became the standard condition in lissamphibians, but even in these it was repeatedly lost—either because larval teeth were retained by neoteny, or alternatively in taxa whose feeding strategy required stronger conical teeth.
In contrast to pedicely, the distribution of cylindrical pleurocentra in the current phylogenetic topology carries less conflicting evidence. Having been long known in Doleserpeton and Amphibamus, they have been found in adults of the branchiosaurid Apateon gracilis (R. Werneburg, personal communication, 2017), closely resembling those of Doleserpeton. In Gerobatrachus, as in lissamphibians, the pleurocentrum has become the dominating element.
Gerobatrachus has a parabolically wide skull, huge posteriorly extended orbits, and a disproportionately small skull table. These are batrachian features, and along with the basale commune in the tarsus (an exclusive salamander character) have been cited to support Gerobatrachus as a stem-batrachian (Anderson et al., Reference Anderson, Reisz, Scott, Fröbisch and Sumida2008a). However, all other derived features of this taxon are consistent with the hypothesis that it forms a stem-lissamphibian, which was found alternatively by Fröbisch and Schoch (Reference Fröbisch and Schoch2009a) and in the present analysis.
Unlike amphibamids proper, Gerobatrachus retains a remarkably branchiosaurid-like tabular horn, more massive anterior trunk ribs, and a large but autapomorphic ectopterygoid. The latter is unique among dissorophoids in expanding posteromedially to support the palatine ramus of the pterygoid, which is aligned just like in anurans, salamanders, and Eocaecilia. Like in branchiosaurids and lissamphibians, the pterygoid is entirely edentulous and its rami are reduced to thin struts, contrasting the shelved and denticle-bearing pterygoid of Doleserpeton and Amphibamus. Conversely, the palatine is much reduced and has almost lost contact to the ectopterygoid, with its vomerine process broadened much like in anurans (in adult salamanders, the palatine is absent). The rounded maxilla with its wide shelf is consistent with that of branchiosaurids as well as lissamphibians.
Considering character-states shared only by Branchiosauridae, Gerobatrachus, and Lissamphibia (see below), the Amphibamidae have lost their exclusive status as immediate lissamphibian sister taxon. This was indicated by Fröbisch and Schoch (Reference Fröbisch and Schoch2009a) and (implicitly) by Sigurdsen and Bolt (Reference Sigurdsen and Bolt2010) who included Gerobatrachus in their analysis, where it nested with branchiosaurids rather than amphibamids proper. Gerobatrachus is clearly advanced over amphibamids proper and branchiosaurids in the reduced presacral count (17 instead of 18 or 19) and it has a wide parabolic skull shape consistent with that of Triadobatrachus, Karaurus, and many crown batrachians, although the primitive condition of caecilians is not yet fully known, but the skull of Eocaecilia is certainly more consistent with that of amphibamiforms in the structure of the palate than crown caecilians would suggest (e.g., the arrangement of the pterygoid, the shape of the parasphenoid, and the structure of the palatine). The teeth are much smaller, more closely set, and more numerous in Gerobatrachus and lissamphibians, as contrasted with all other amphibamiforms. Moreover, the premaxilla has a thin dorsal (alar) process, again more consistent with that of lissamphibians than with branchiosaurids or amphibamids.

Figure 2 Phylogenetic definition of Dissorophoidea and its major constituent taxa, based on the results of the present analysis.
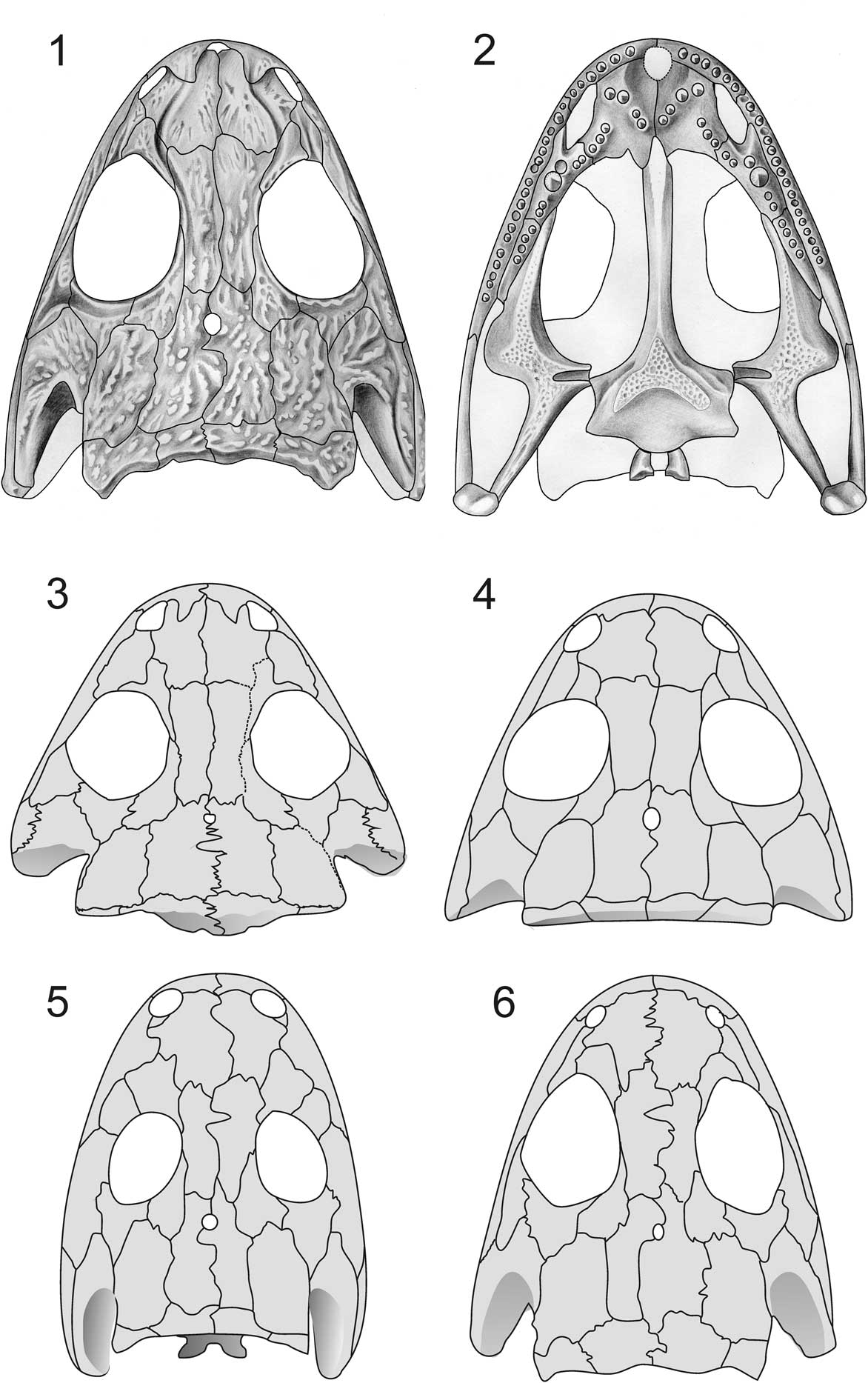
Figure 3 Morphology of basal dissorophoids (micromelerpetids) and stem taxon (Perryella). (1) Micromelerpeton credneri, skull roof (after Boy, Reference Boy1995; skull length 25 mm); (2) M. credneri, palate (after Boy, Reference Boy1995; skull length 22 mm); (3) Limnogyrinus elegans (personal observation; skull length 18 mm); (4) Branchierpeton amblystomum (after Werneburg, Reference Werneburg1991; skull length 20 mm); (5) Perryella olsoni (after Ruta and Bolt, 2006; skull length 41 mm); (6) Eimerisaurus graumanni (after Boy, Reference Boy1981; skull length 32 mm).

Figure 4 Phylogeny of basal dissorophoids (synapomorphies mapped, see Supplemental Dataset for character definitions).

Figure 5 Morphology of amphibamiforms. (1) Micromelerpeton credneri, skull roof (Schoch and Milner, Reference Schoch and Milner2014; skull length 40 mm); (2) Apateon caducus, skull roof of neotenic adult (Fröbisch and Schoch, Reference Schoch2009; skull length 29 mm); (3) Amphibamus grandiceps, skull roof (personal observation; skull length 18 mm); (4) Apateon gracilis, palate of metamorphosed adult (personal observation; skull length 18 mm); (5) Apateon caducus, palate of neotenic adult (after Schoch, Reference Schoch1992; skull length 16 mm); (6) Amphibamus grandiceps, palate (personal observation; skull length 18 mm).
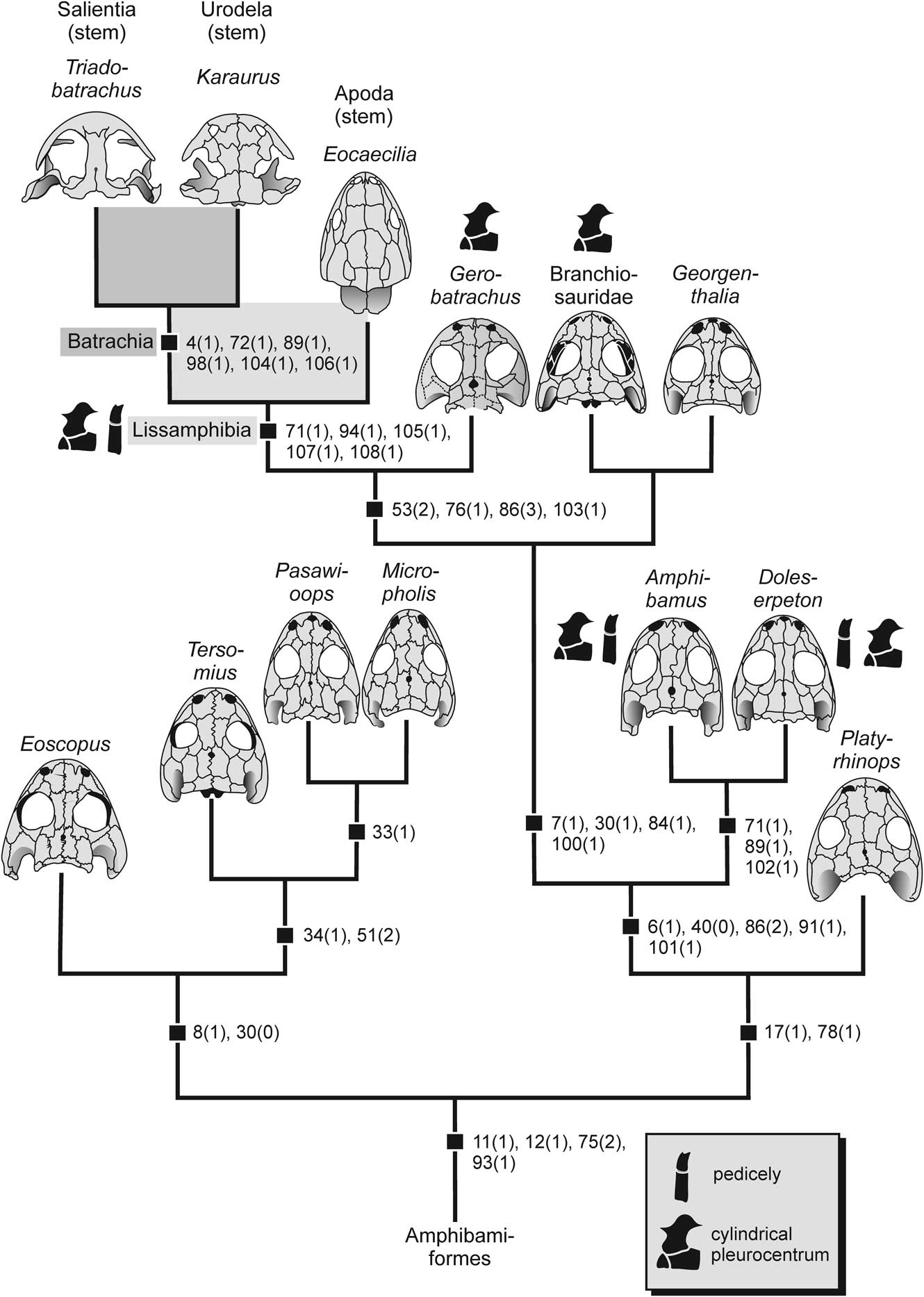
Figure 6 Phylogeny of derived dissorophoids and origin of lissamphibians according to the monophyletic temnospondyl hypothesis (synapomorphies mapped, see Supplemental Dataset for character definitions). Two lissamphibian characters (pedicely and vertebral centrum) mapped.
Acknowledgments
I thank I. Rosin, M. Kamenz, and N. Adorf for their skillful preparation of material and casts, J. Boy, A. Milner, and R. Werneburg for many discussions, and J. Anderson, N. Fröbisch, and F. Witzmann for their constructive and helpful reviews.
Accessibility of supplemental data
Data available from the Dryad Digital Repository: https://doi.org/10.5061/dryad.1n1p0b5.2