Introduction
The works undertaken by the hydroelectric company d’Emosson S.A. on the Glacier d’Argentière in order to divert and utilize the subglacial stream (Reference Vivian and ZumsteinVivian and Zumstein, 1969; Reference VivianVivian, 1970, Reference Vivian1971) have allowed access to a number of natural cavities, elongated in the longitudinal direction, between the rock bed and the base of the glacier. These cavities, which one rediscovers at the same level (altitude 2170 m a.s.l.) are situated on the down-hill slope of a rock bar in a region of steep gradient (40°−60°) corresponding on the surface to the Lognan ice fall, below 100 m of ice and 2 km up-glacier from the glacier snout (Fig. 1). The first cavity discovered has been the object of observations and systematic measurements since the beginning of the year 1970. Others although more spectacular have not been exploited scientifically both because they were only discovered recently (October 1972) and also because the artificial access to these cavities has been quickly obstructed by incoming ice.

Fig. 1. Location of the subglacial cavities of the Glacier d’Argentière: (a) in section, (b) in plan.
Description of the Subglacial Cavities
1. The floor formed by the rock bed. This consists of crystalline schists and the floors have different profiles according to the case, but all of them have a certain number of characteristics in common (Fig. 2):
The longitudinal slopes are high, between the extreme values of 40° and 80°. The less steep sections are to be found at the level where the ice regains contact with the rock, this reduction of slope having been observed following the melting of ice with warm water into two of the cavities (B and D).
According to the shape of the cavities and the mean slope of the rock bed, the irregularities of the latter take two different forms:
-
1. In small holes (e.g. B) one finds juxtaposed glacial abrasion forms (striae, grooves) and water erosional forms: solution cups, scallops, pot-holes (Reference ThomasThomas, 1971). The general appearance is that of a succession of concave profiles cutting each other at the level of smooth crests.
-
2. In larger cavities, the long profile does not result from the types of erosion described above. The slopes are steeper, convex profiles are more frequent, and the rock shows no signs of glacial erosion forms, but reflects solely interactions between the lithology and the tectonics.
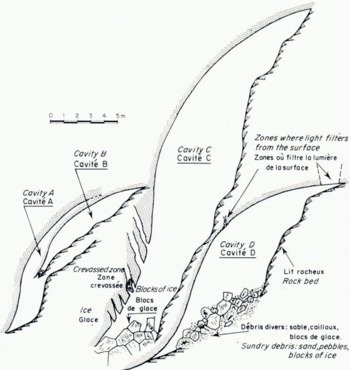
Fig. 2. Longitudinal profile of the different cavities studied.
Despite an ambient temperature which is slightly positive and which varies a little (around +0.5° C) the rock is covered discontinuously by a coating of ice whose thickness increases down-stream from 1−8 cm. This ice sometimes contains a little solid matter, but sometimes is very loaded with sand taken up by the glacier or falling from the ice ceiling.
2. The ceiling of the cavities corresponding to the bottom of the glacier. In all cases this presents a very curved longitudinal form of generally hyperbolic shape and with a long radius of curvature (Fig. 3a). In detail the ice vaults are not however always perfectly regular:
-
1. In the longitudinal sense, at approximately two-thirds of its length, the vault has a point of inflexion separating a long uphill sector with concave profile from a short downhill sector with convexity towards the rock. This convexity is more or less marked; in cavity C, the largest, it is accompanied by an intense cracking.
-
2. The transverse section of the glacial vault retains the detail of the irregularities of the topography of the rock bed on which the ice is moulded.

Fig. 3. (a) Partial view of cavity B. Note the detail of the forms on the vault—spicules, chips, protuberance of a block in the course of expulsion—and the refrozen layer on the rock bed. (b) Initiation of separation in cavity B. Note the ice chips and spicules on the ice vault.
Beneath the Glacier d’Argentière, the amount of solid matter evacuated by the glacier is not very large,Footnote * the majority of the mass of material below the glacier consisting of fine sand (median 0.25 to 1 mm) which covers the vault and which one finds again in the interior of the glacier in the form of strata.
The rare blocks of protogine coming from the accumulation basin, which are trapped in the base of the glacier, are progressively expelled by three processes: subglacial erosion, increase of the stresses (locally between the stone and the air-ice interface and at the place where the ice–rock contact is approached down-stream from the cavity), fracture by compression of the layer of ice containing the block. Under the effect of stress disequilibrium, the rejection of the block occurs (Fig. 4b).

Fig. 4. (a) Longitudinal section of cavity B, (b) expulsion of a block, (c) formation of a refrozen scar.
The fallen blocks slide on the rock bed and tend to accumulate at the level where ice-rock contact is taken up. There they are slowly taken back into the glacier.
The difference in speed between the ice and the transported block causes, where the ice and the blocks are under pressure on the rock bed, fusion phenomena on the uphill side and refreezing on the downhill side of the blocks. Thus at the level of the cavity one can observe downhill of the blocks an elongated scar in the direction of flow of the ice and corresponding, according to the degree of evolution, either to refrozen ice—very pure and thus nicely differentiated from the sandy ice around it—or to a little pocket of water if the refreezing is incomplete, particularly down-stream from large blocks, or to a void when this pocket of water for various reasons (expulsion of a stone, expansion phenomena of ice) has been punctured (Fig. 4c).
The vault also carries ice efflorescences following on the phenomena of decompression on the ice-air interface: particularly abundant ice chips at the start of the opening (Fig. 3b), bending of the ice surrounding blocks of rock in the course of expulsion, stalactites and ice spicules (already described by Reference Kamb and LaChapelleKamb and LaChapelle, 1964) at various points. All of these formations are carried along by the general movement of the glacier (Fig. 4a).
3. The line of rejoined contact between ice and rock. Below the cavity this is characterized by two series of phenomena which underline the effects of vertical creep in the ice: (i) uphill movement against the slope of “ice wedges” (Fig. 4a), (ii) puddles of water which can persist for from several hours to several days at the back of the vault where it presses on the rock. This water comes either from the basal melting of the glacier or from overflowing of the subglacial stream. In this second case, the volume of water is therefore somewhat larger.
The Basal Ice Layer
The deep ice of the glacier, that of the level at which the cavitation phenomena occur and where, sliding happens, is not the same as that of the remainder of the glacier. This ice for which we propose a name of basal ice layer represents an enigma in several ways.
The study and observation of this ice made possible by the melting of ice by hot water (35° to 38° C) carried out by Emosson S.A. has allowed us to observe certain of its physical characteristics. The results will form the basis of a separate publication by Monique Ricq, but the main lines of this study are of interest in the context of this paper because the origins of this deep ice are no doubt related to the cavitation phenomena.
The basal ice layer consists primarily of an ice relatively charged with sand containing within its mass some rare pebbles of modest size (5 to 6 cm in long axis) associated or not with little pockets of water. More often these water pockets are isolated in the ice where they can reach larger volumes (from a half to one cubic metre). The basal ice shows marked lamination in places, certain ice beds being separated by very thin layers of water.
The deep ice is very often stratified: bands of pure ice alternating with bands of ice containing solid debris visible in section on the walls, but also on ice cores extracted from the base of the glacier. These strata have a mean thickness of 8 to 10 cm (Fig. 5). Stratification is not general at all places under the glacier, but constitutes a discontinuous phenomenon. It seems that it must be connected with the classical phenomena of freeze-thaw related to the overcoming of obstacles on the rock bed, but even more to temperature differences (some tenths of a degree is sufficient) existing from time to time at the level of the beginning of the cavities (for reasons which we shall return to later). Three years of observations in cavity B have allowed us to establish during certain visits the formation under the vault of refrozen ice some centimeters in thickness whereas at other times melting assists in the concentration of the sandy fraction in very dirty layers. The discontinuous distribution of the stratification results from a double discontinuity: spatial (as a function of the obstacles and their disposition on the rock bed) and temporal (as a function of the phases of penetration of cold waves with the assistance of the subglacial cavities).

Fig. 5. (a) A view of the basal ice layer of the Glacier d’Argentière. (b) The basal ice layer of the Mer de Glace.
The freeze–thaw phenomena under the glacier cause important changes in the chemical and physical–chemical properties of the basal ice as compared with those of ice in the firn field. These changes, studied by Monique Ricq, are related to the displacement of solid impurities (redistribution as a function of the speed of refreezing) and the enrichment in salts below the ice through the action of the sand in suspension (important enrichment in the melting phase). The first results show that the basal ice differs from ice in the firn region by a higher pH and a stronger mineral content, but Reference Souchez, Souchez, Lorrain and LemmensSouchez and others (1973) have proved that the ice in the chips has a still higher mineral content than that of the basal ice. The basal ice layer, for which crystallographic study reveals a very large deformation of the crystals,Footnote * is of variable thickness. However, one does not know a very exact value for this: it has been recognized to be over 1.5 m at the Glacier d’Argentière and more than 2 m at the Mer de Glace (observations by R. Vivian), but it is not impossible to think that its thickness could attain a higher maximum value approaching some 10 m. Its identification allows us perhaps to give a reasonable explanation of certain results obtained during seismic soundings (on the Z’Mutt, Findelen, Unteraar, and Gurgler glaciers) which produce evidence for the existence at the base of these glaciers of a reflecting level defining a deep zone which seems to be endowed with specific properties different from those of the ice above. This deep zone has generally been assumed up to now to be bottom moraine. The multiplication of the number of sites accessible below glaciers in the Alps permits us today rather to think of an ice with a different nature (that for which we have here given several physical characteristics) which has an accumulation truly morainic in form.
Glacier Sliding and the Phenomenon of Glacial Cavitation
The glacier sliding velocities were recorded by means of a cavitometerFootnote † installed in cavity B since the winter 1970/71 (Fig. 6), and are of the order of 3 cm/h on average (by way of comparison Electricité de France have measured, beneath the Mer de Glace and under a similar thickness of 100 m of ice, mean velocities of 5−6 cm/d, and this virtually prohibits all cavitation).

Fig. 6. The Argentière cavitometer.
The flow of the glacier does not occur in time in a regular manner. The annual study of variations shows on the one hand a seasonal variation: a period of high velocities of average over 3 cm/h (capable of reaching sometimes 5 cm/h), in June through July to the beginning of August, and a period marked by smaller average (below 2.5 cm/h) in winter, at the beginning of spring and in the autumn; on the other hand, sharp deceleration exists (actual complete halting of the glacier, which seems to be blocked) for from several hours to 1, 2 or 3 d in duration, alternating with accelerating phases very often occuring at the end of the day and during the first hours of the night. Seven days of continuous observations with systematic measurements every hour made by three observers shut underneath the glacier (“Glaciolab 72” experiment) have allowed us to make a more detailed analysis of the successive changes in the subglacial cavity, these in turn reflect variations in the speed of sliding (Fig. 7, where one can establish a very large variability in hourly velocities).

Fig. 7. Experimental study of velocities of displacement and deformation in cavity B.
Along a single flow axis of the glacier, the velocities recorded vary from up-stream to down-stream (2.9 cm/h at the beginning of the cavity, 2.5 cm/h in the central part, 2.1 cm/h at the point where ice–rock contact is resumed). Other observations show that in the neigh-bourhood of this contact internal stresses in the ice increase, precipitating the expulsion of solid materials (rejection phenomenon) contained in the basal part of the glacier or causing the uphill flow of “ice wedges”, a continuing phenomenon under the Glacier d’Argentière. This allows us to understand the previous observations made under the glacier. It underlines the continuity of the line where ice-rock contact is found down-stream of the cavity, whereas on the uphill side the line where the cavity begins is locally variable. Everything occurs as if the glacier, being braked down-stream in a sill of the rock bed, buckles itself, the cleavage which results from this lack of uniformity of sliding grows in the down-stream–up-stream direction into the form of regressive glacial cavitation.
Apart from the differences in velocity observed in the longitudinal direction, there are even slight variations in the transverse direction: they have been measured both on a section situated at 2180 m a.s.l. and at 2 km up-glacier from the front. They accompany the more or less pronounced cleavages of the glacier and explain the discontinuity of the phenomenon of glacial cavitation from one bank to the other of the valley. A study of the three-dimensional deformations of geometrical patterns enscribed on the glacial vault illustrate perfectly their differential interplay and also the interference effects related to the proximity of contact with the bedrock.
Sliding of the glacier reflects numerous secondary deformations due to the creep of ice. Figures 7 and 8, which analyse the temporal deformations of the segments constituting the geometrical figures, show that from 20 to 26 October 1972, the deformations were stronger on the sides perpendicular to the direction of flow of the ice (influential role of the topography of the cavity when it is regaining contact between ice and rock) whereas the other segments, sub-parallel to the direction of flow of the glacier, only have limited deformations. The segment situated most down-stream, nearest to the rock, is registering two different behaviours of the ice: at f (more perturbed location) the advance of the glacier is more jerky; at e the flow is more regular for this point is located less close to the contact. This explains the very irregular shape of the curve ef—when the geometrical figure arrives at the end of the cavitation—which is justified by the elastic properties of the ice.

Fig. 8. Deformation of a geometrical pattern inscribed on the ice vault in the course of seven days of observation 20–26 October 1972.
These new observations on subglacial cavitation suggest at least two comments:
-
1. On the scientific level, cavitation is related to a diminution in ice velocity, a phenomenon resulting from irregularities in the long-profile of the rock bed rather than to a relation between velocity and slope at the moment where the cleavage begins as one had hitherto thought. The glacier does not cleave beginning from a fixed departure point situated up-stream, but deforms itself beginning from a constant point of contact situated down-stream and this constitutes a zone of maximum friction.
-
2. On the practical level, the resumption of ice-rock contact at the down stream end of a cleavage, the generating point of the subglacial cavitation, could be considered as the cornerstone of the whole phenomenon. Its natural destruction (by crevasses or deep shear cracks) or artificially (melting by hot water in order to open subglacial tunnels) risks breaking the tectonic equilibrium of the glacier and from that could create a very perturbed situation, which could be highly dangerous.
Cavitation and Subglacial Micro-Climatology
Thus below the Glacier d’Argentière the steep slopes of the rock bed down-stream from the Lognan rock bar favour the development of subglacial cavities. These are interconnected one with another by a veritable network of channels more or less open (Fig. 9); very significant air currents indicate the existence of exchanges between these different cavities. In the month of December 1972, after the first snow falls, a cavity (D) situated near the left bank, where the thickness of ice is least, still allowed, at the level of two accidents to its vault, light to filter in coming from the outside through crevasses. It seems therefore that subglacial cavities communicate either totally or in part, permanently or temporarily, one with the others and are therefore equally open to external influences. These exchanges can modify very appreciably the micro-climatic characteristics of the subglacial spaces.

Fig. 9. Interconnecting channel between cavities A and B.
The experiment “Glaciolab 72” has allowed us to be more precise about several possible effects of this air circulation under the glacier. The inclusion of three people in cavity B for seven days could not help transform the micro-climate of the cavity. The increase of temperature was from 0.3 to 0.4 deg (from 0.4 to 0.8° C) a sufficient elevation to eliminate all forms of spicules and ice chips and to accelerate melting at the level of cleavage. This very localized increase in the temperature of the cavity appears to have had no influence on the dynamics of the glacier proper. On the contrary all differences were the consequences of a subglacial reheating of some tenths of a degree, a general reheating following on a sudden increase in external temperature. The streams which in summer flow with high outfall (10 to 15 m3/s) play the role of an air pump, force air circulation in the cavities, and facilitate the penetration of warm currents below the glacier.
It therefore seems that we are here in the presence of factors favourable to ice falls. For the last two large disasters of this type in the Alps, a definite elevation in temperature had been recorded a little before the release of the catastrophies (Reference Kamb and LaChapelleGuichonnet, 1950; Reference VivianVivian, 1966). More than some tenths of a degree suffice to accelerate basal melting. This melting causes the formation at the base of the glacier of a horizon of thickness several centimetres, rich in sand and in water, a sort of mud which lacks cohesion, reducing friction, and favouring sliding when contact is resumed between the glacier and its bed. There, where the glacier is again in contact on the rock, the role of the liquid element remains essential, thanks to the existence of a film of water measured at 0.2 mm in thickness on the Glacier d’Argentière (Reference Vivian and ZumsteinVivian and Zumstein, in press). The increase in pressure at the level of the resumption of contact between ice and rock causes a melting which is added to a thermal melting at the level of the cleavages: this therefore causes a complete upsetting of the regime of velocities, characteristic of a sliding facilitated by an elimination of friction, which can be the origin of spectacular falls of ice.
Acknowledgements
We particularly wish to thank for their help and advice Messieurs P. Veyret, Director of the Institut de Géographie Alpine and M. Pardé, Professeur de Potamologie, R. Weller, Director of Emosson S.A., E. Chardonnet, Chef des Services Études EDF-REH Alpes Nord, P. Courdouan, Chef d’Aménagement EDF at Chamonix, also Dr. J. W. Glen who has undertaken the translation into English of the French text.
We also wish to thank the members of “Glaciolab 72” and all the University organizations public and private which took part in the experiment.