Eggshell is a complex structure with important biological functions for protection of inner content of egg after deposition as well as for chicken embryo development(Reference Sirri, Zampiga and Berardinelli1). There also exists a high risk of eggshell breakage and bacterial infection during collection, classification, packaging, transportation, storage and processing(Reference Li, Gong and Zhan2). It has been estimated that loss from damaged eggshell accounts for 8–11 % of total egg loss(Reference Dunn, Joseph and Bain3). The cracking of eggshells is a costly problem in the egg industry and gets worse with age-related reduction in shell quality(Reference Zhang, Zhang and Wang4). Hence, eggshell quality is a major concern for sales of eggs and development of poultry production.
To improve eggshell quality, great efforts have been taken in the genetics, environment and nutrition, especially in the micromineral nutrition(Reference Manangi, Vazques-Anon and Richards5–Reference Zhang, Wang and Zhang8). Zn, for example, is an essential metal micronutrient with particular physiological functions in all animals(Reference Salgueiro, Zubillaga and Lysionek9). Zn is necessary for maintenance of structural and functional integrity of biological membranes, facilitation of gene expression and protein synthesis, enzyme structure and function, appetite regulation and good food utilisation(Reference Cao, Henry and Davis10,Reference Park, Birkhold and Kubena11) . Zn is closely linked to eggshell formation(Reference Tsai, Mao and Li6,Reference Min, Liu and Qi12,Reference Martin13) . Especially, Zn plays an important role as a cofactor of carbonic anhydrase (CA) which involves in catalysing hydration of metabolic CO2 to ${\rm{HCO}}_3^{-}$ and supplying carbonate ions during eggshell formation(Reference Zhang, Zhang and Wang4). In addition, Zn can be incorporated into the growth step during calcite crystal formation in vitro (Reference Elzinga and Reeder14) and thus may affect the crystal and texture morphologies of the shell(Reference Zhang, Zhang and Wang4).
Traditionally, the major sources of Zn in mineral supplements of animal feeding diets have been its inorganic salts such as zinc sulphate (ZnSO4), zinc oxide, zinc chloride, etc.(Reference Garg, Mudgal and Dass15) However, it has been reported that the Zn-methionine chelate (Zn-Met) would be more stable and better resist to interference from pH, phytate or other ligands in the digestive tract and then could arrive at the absorptive site in the small intestine as an intact structure and thus might have more Zn absorption and a higher Zn bioavailability compared with ZnSO4(Reference Suo, Lu and Zhang16). Moreover, it is known that Met is considered to play main roles in body(Reference Elnesr, Elwan and Xu17), such as protein synthesis, a glutathione precursor, reactive oxygen species elimination and methylation reaction of DNA(Reference Kidd18). Thus, the combination between Zn and Met in this form (Zn-Met) exhibits more powerful influence than each addition in the alone form. It has been suggested that the amount of supplemental Zn as an organic form may be lowered to replace the utilisation of inorganic Zn(Reference Gheisari, Rahimi-fathkoohi and Toghyani19), thereby reducing the excretion of trace minerals that potentially pollute the environment. Moreover, it was reported that supplementation of organic Zn could increase eggshell quality by enhancing eggshell thickness and CA activity in the plasma and eggshell gland (ESG) of aged layers(Reference Zhang, Zhang and Wang4). Nevertheless, the mechanism of organic Zn enhancing eggshell quality of laying hens was not clear.
The eggshell is structurally composed of organic and inorganic components. Stefanello et al. (Reference Stefanello, Santos and Murakami20) reported that inorganic component of the eggshell consists of approximately 96 % calcium carbonate (CaCO3) crystals. Ca from the diet (CaCO3) through the intestine into the plasma is then either stored in the bones or transported directly to uterus (or avian ESG for synthesising into eggshell CaCO3)(Reference Gheisari, Rahimi-fathkoohi and Toghyani19). It is hypothesised that dietary Zn supplementation might increase eggshell quality on laying hens by enhancing the Ca deposition in eggshell formation of ESG. Therefore, the objective of the present study was to explore whether Zn-Met can enhance eggshell quality of laying hens and the mechanism of affecting eggshell formation would be related to Ca deposition by investigating the effects of Zn-Met on eggshell quality, Zn content, activities of Zn-containing enzymes and expression of shell matrix proteins in ESG of laying hens.
Methods
All experiments performed on animals were conducted in accordance with the Animal Welfare Committee guidelines and approved by the Animal Science College of Zhejiang University (Hangzhou, China), and the manuscript conformed to the Animal Research Reporting In Vivo Experiments Guidelines for Reporting Animal Research. The World Medical Association Declaration of Helsinki can be extended to animal trials and experimentation; however, alternatives exist and commenting on adherence would not go amiss.
Bird management, experimental design and diets
In the present study, 38-week-old Hyline Grey laying hens (n 384) with similar performance (laying rate: 88·65 (SEM 1·79) %; body weight: 1·42 (SEM 0·07) kg) were randomly allocated to four treatments: a control group fed without additional Zn supplementation in premix, the first experimental group fed lowered level of organic Zn as Zn-Met (40 mg Zn per kg of diet) (rate of chelation: 99·0 %, purity: 96·00 %, 16·00 % of Zn content, 80·00 % of Met; Novo International Trading Co., Ltd), the second experimental group fed recommended levels of organic Zn as Zn-Met (80 mg Zn per kg of diet) and the group receiving Zn in 100 % of daily recommended dose from ZnSO4 monohydrate (80 mg Zn per kg of diet) (≧99·00 % in purity; Sinopharm Chemical Reagent Co. Ltd). Each of the groups consisted of six replications in four different cages (four birds per cage). The size of each cage (equipped with two nipple drinkers and one feeder) was 50 × 60 × 60 cm3. An enclosed, ventilated and conventional house with 16 h lighting was provided for all laying hens to keep them in the same environmental conditions. Feed and water were offered ad libitum. The experiment lasted 12 weeks, consisting of a 2-week adaption period and a 10-week experimental period. During the adaption period from 38 to 40 weeks of age, all laying hens were fed the normal diet with 80 mg/kg Zn (China feed standard) to make them similar with regard to healthy laying performance.
The basal maize–soyabean meal diet (Table 1) was formulated to meet or exceed the requirements for laying hens (National Research Council, 1994)(21). Different Zn sources were supplemented in the premixes. Differential contents of Met in these groups were caused by addition of Zn-Met in premix, which was corrected by the addition of extra Met (dl-methionine) in the premix. Hence, all nutrients were kept at the same levels except for Zn content that met the nutritional requirements in the basal diet, which was in accordance with NY/T 33-2004 (Chicken Feeding Standard, Agricultural Industry Standard of the People’s Republic of China). Approximately 200 g of each diet was collected and stored at −20°C for analysis of the Zn content according to the method of atomic absorption spectrometry using an atom absorption spectrophotometer. The analysed Zn contents in the four experimental diets were 24·99, 65·35, 105·33 and 105·22 mg/kg for control, Zn-Met (40 and 80 mg/kg), and ZnSO4, respectively.
Table 1. Composition of the experimental basal diet

* The premixes provided the following per kg of diet: vitamin A 7500 IU, vitamin D3 2500 IU, vitamin E 25 mg, vitamin K3 2·5 mg, vitamin B1 1·5 mg, vitamin B2 4·5 mg, vitamin B6 3 mg, vitamin B12 0·02 mg, niacin 25 mg, folic acid 1·1 mg, calcium pantothenate 8 mg, biotin 0·12 mg, choline chloride 400 mg, Cu 20 mg, Mn 60 mg, Fe 90 mg, iodine 0·8 mg, Se 0·3 mg.
† The premix in the control diet provided per kg of diet: 0 mg of Zn/kg; the premixes in another two groups provided per kg of diet: 40, 80 mg Zn/kg as Zn-methionine (Zn-Met), respectively; the premix in the last group provided per kg of diet: 80 mg Zn/kg as zinc sulphate monohydrate. Different Zn sources were supplemented in the premixes. Differential contents of methionine in these groups were caused by the addition of Zn-Met in the premix, which was corrected by the addition of extra methionine (dl-methionine) in the premix. Hence all nutrients were kept at the same levels except for Zn content which met the nutritional requirements in the basal diet, which was in accordance with NY/T 33-2004 (Chicken Feeding Standard, Agricultural Industry Standard of the People’s Republic of China).
‡ Metabolisable energy based on calculated values; others were analysed values.
Sample collection
At the end of the experiment, thirty-six eggs from each treatment group (six eggs per replication and six replications per treatment) were randomly collected at 5-week and 10-week experimental periods for egg quality determination as reported previously(Reference Zhang, Wang and Zhang8). Twelve hens from each treatment group (two hens per replication) were randomly selected and marked. After 12-h feed withdrawal (water was offered ad libitum), hens were killed humanely by carbon dioxide asphyxiation. The ovary, magnum, and ESG samples were collected immediately. A set of tissue sub-samples was snap-frozen in liquid N2 and then stored in −80°C for the mRNA level analysis. Another set of sub-samples was kept on ice and stored at −20°C for subsequent measurements of Zn content, Ca, P, total protein (TP) and albumin (Alb) levels as well as the activities of alkaline phosphatase (ALP) and CA. Blood samples collected from the wing vein were centrifuged for 10 min (3000 g ) to separate out serum. Pure serum samples were aspirated by pipette, stored in 1·5-ml tubes at −80°C until analyses and thawed at 4°C before analysis. All serum samples from two birds in each replicate were pooled into one sample in equal ratios before analysis as previously reported by Liao et al. (Reference Liao, Li and Zhu22).
Egg quality determination
Eggshell thickness was measured without membrane using a digital micrometre at three different points (air cell, sharp end and any side of the equator) and estimated by the average of three different thickness measurements from each egg(Reference Xiao, Zhang and Wu23,Reference Yilmaz Dikmen, Sözcü and İpek24) . Egg weight, eggshell strength, albumen height, yolk colour and Haugh unit were measured using an Egg Quality Analyzer (DET-6000; Nabel Co., Ltd)(Reference Li, Zhang and Gong25). After egg quality determination, yolk and egg white were separated and placed in 50 ml tubes. The eggshell, egg white and egg yolk were collected and frozen (at −20°C) for further analysis.
Eggshell ultrastructure and energy-dispersive X-ray spectroscopy determination
The eggshell collected was washed with distilled water to remove the dirt and the inner shell membrane and then dried at room temperature (25 ± 2°C) for at least 48 h(Reference Dunn, Rodriguez-Navarro and Mcdade26). Three parts of sample peels from the equator were separated and prepared for field emission scanning electron microscope (SU8010) analysis. Two parts of samples (0·5–1 cm2) were used for the analysis of the external and internal surface of the shell, the third part was used for the assessment of the transversal surface. The samples were glued on an aluminium support (stub), metalized with gold and analysed in the electron microscope(Reference Stefanello, Santos and Murakami20). For the internal surface analysis, the fibre diameter and fibre gap area were measured using the field emission scanning electron microscope ruler. For the transversal surface analysis, the effective total thickness (combined mammillary layer, palisade, vertical crystal and cuticle sections, μm), the mammillary thickness (μm), the palisade layer thickness (μm) and width of the mammillary knobs (μm) were measured using the field emission scanning electron microscope ruler(Reference Dunn, Rodriguez-Navarro and Mcdade26). The percentage of the palisade layer thickness and the mammillary thickness were obtained by calculating the percentage of the thickness of each layer to the effective total thickness. Five scanned images were obtained from each sample (six samples per group) using 200× magnification (200 μm, external and internal surface) and 100× magnification (500 μm, transversal surface). Furthermore, the eggshell peels (six samples per treatment) without metallisation by gold were analysed for elemental composition and distribution with an Oxford energy-dispersive X-ray spectroscopy operated at 20 kV and at a working distance (WD) of 20 mm. The energy-dispersive X-ray spectroscopy was calibrated using a pure Co sample operated at 20 kV and at WD 20 mm. The spectrum of elemental peaks was cross examined and data values (relative atomic weight percentages) were normalised. The determination of energy-dispersive X-ray spectroscopy contained line scan and mapping scan of transversal surface of eggshell at a magnification of 200× (200 μm). The same area was determined in transversal surfaces of all groups and contained all kinds of layers in eggshell (average the testing values of repeated measurements).
Zinc contents in eggshell, egg white, egg yolk, serum and eggshell gland
Eggshells were ground to a fine powder, then ashed (550°C, for 14 h) and solubilised in 10 ml hydrochloric acid (6 M) and 30 ml of demineralised water at a high temperature using a sand heater (300°C for 15 min). After filtration (Whatman filter), the volume was increased to 50 ml with demineralised water prior to analysis, according to the method of Gheisari et al. (Reference Gheisari, Sanei and Samie27); egg white or yolk was defrosted, mixed and 0·5 g was placed in a plastic high-pressure tube for digestion (7 ml of nitric acid and 2 ml of hydrochloric acid) using an organic program. After cooling, the samples were transferred to 100 ml flasks and brought to 100 ml volume with deionised water for the homogenisation(Reference Rubio Zapata28); Zn contents in serum, ovary, magnum and ESG were measured according to the method of Chen et al. (Reference Chen, Hogstrand and Luo29). Flame atomic absorption spectrophotometry was used in the determination.
Activities of carbonic anhydrase and alkaline phosphatase, the concentrations of calcium, phosphorus, total protein and albumin
The ESG was homogenised in 10 % (w/v) ice-cold physiological saline and then centrifuged for 10 min at 4°C (3000 g ). The supernatant collected and the serum were used to determine the concentrations of Ca, P, TP and Alb and the activities of ALP using analysis kit (Nanjing Jiancheng Bioengineering Institute), and CA activity was measured by ELISA kit (Shanghai mlbio Institute). A microplate reader (Bio-tek ELX800; Biotek Instruments, Inc.) was used in the determination.
RNA extraction, reverse transcription and real-time quantitative PCR
Total RNA was extracted from ESG using Trizol reagent (Invitrogen) according to the manufacturer’s instruction. The concentration of each extracted RNA sample was determined using a Nano Drop Spectrophotometer (ND-2000; Gene Company Ltd), and the integrity of the RNA was checked using denatured RNA electrophoresis. A total of 1 μg RNA was used to obtain complementary DNA by reverse transcription using the HiScript α Q RT SuperMix RT (Vazyme). Real-time quantitative PCR reactions were performed on an ABI 7500 real-time quantitative PCR system with a 10-μl reaction volume containing 5 μl of 2 × ChamQTM Universal SYBR-Green qPCR Master Mix (Vazyme), 0·2 μl of 10 µM each of forward and reverse primers, 1 μl diluted complementary DNA template (10-fold) and 3·6 μl double-distilled water. The primer sequences for osteopontin (OPN), ALP, CA, ovocleidin-17, ovocleidin-116, Ca-binding protein-d28k (CaBP-D28k) and β-actin are given in Table 2. The protocol of PCR is as follows: denaturation at 95°C for 30 s, followed by 40 cycles of 95°C for 10 s and 60°C for 30 s. All reactions were performed in duplicates and each reaction was verified to contain a single product of the correct size using agarose gel electrophoresis. The 2−ΔΔCT was used to calculate the mRNA level of each target gene(Reference Livak and Schmittgen30). The internal reference genes (β-actin) were used to normalise the expression level of the targeted gene.
Table 2. Primers used for real-time quantitative fluorescence PCR analysis

OPN, osteopontin; ALP, alkaline phosphatase; CA, carbonic anhydrase; OC-17, ovocleidin-17; OC-116, ovocleidin-116; CaBP-D28k , Ca-binding protein-d28k.
Calculations
Total Met content of premix (2 %) was calculated ((0·34–0·24 %)/5 %) on the basis of removing the Met content (0·24 %) in the maize, soyabean meal and fishmeal from the criteria of Met (0·34 %).
Addition of extra Met in the premix (g/kg) = 2 % × 103 – Zn addition in premix (g/kg) × quality fraction of Met in Zn sources (80 %).
Statistical analyses
The results were presented as means with standard errors and analysed statistically by one-way ANOVA using SPSS 20.0 except the percentage of relative atomic weight in transversal surface of eggshell (means and standard deviation). Differences among all treatments were separated by the Tukey test for multiple comparisons. The Pearson correlation test (two tails) were used to analyse and examine the relationship between eggshell strength and ultrastructure-related parameters for the 80 mg/kg Zn-Met group. Values of P < 0·05 were considered significant. All the graphs were made using the GraphPad Prism 5.01.
Results
Egg quality determination
Throughout the experiment, albumen height, Haugh unit and yolk colour showed no significant difference (P > 0·05) between the four groups (Fig. 1(B)–(D)). However, at the 5-week experimental period, egg weight (P < 0·01) and eggshell strength (P < 0·05) were numerically the highest in the 80 mg/kg Zn-Met group but showed no significant differences (P > 0·05) between the control and ZnSO4 treatment (Fig. 1(A) and (F)). At the 10-week experimental period, shell thickness and shell strength increased in the 80 mg/kg Zn-Met group compared with the control and ZnSO4 groups (control v. Zn-Met 80: P < 0·01; ZnSO4 v. Zn-Met 80: P < 0·05) while the statistically significant increase (P < 0·05) in the Zn-Met groups was observed in egg weight when compared with the control (Fig. 1(A)–(F)).

Fig. 1. Effects of dietary zinc-methionine (Zn-Met) supplementation on egg quality of laying hens at 5-week and 10-week experimental periods. Values are means (six eggs per replication, six replications per treatment) with standard errors represented by vertical bars. a,b,c Mean values with unlike letters were significantly different among the four treatments (P < 0·05). , 5 week;
, 10 week. Zn-Met 40, Zn-Met at 40 mg/kg; Zn-Met 80, Zn-Met at 80 mg/kg; ZnSO4 80, zinc sulphate at 80 mg/kg.
Eggshell ultrastructure and Pearson correlations between eggshell strength and ultrastructure-related parameters for the 80 mg/kg zinc-methionine group
Laying hens fed with supplemented Zn diets had a great width of mammillary cones in transversal surface as well few cracks on the external surface, dense fibre nodules and small fibre gap in internal surface compared with those fed the control diet (Fig. 2). As for internal surface (Fig. 3(A) and (C)), fibre diameter with 80 mg/kg Zn-Met treatment increased compared with the control (P < 0·001) and ZnSO4 treatment (P < 0·05) but there was no difference in area of fibre gap (P < 0·05) among all groups. In the transversal surface (Fig. 3(B) and (D)), 80 mg/kg Zn-Met treatment reduced mammillary knob width (P < 0·001) compared with the control and ZnSO4 groups but increased mammillary thickness (P < 0·01), effective total thickness (P < 0·001) compared with control. There was no difference (P > 0·05) in the percentage of the thickness of each layer (mammillary layer or palisade layer) to the effective total thickness among all treatments.

Fig. 2. Scanning electron microscope photographs of external surfaces, internal surface and transverse surface of eggshells on laying hens. Scale bars: external and internal surface: 200 μm; transversal surface: 500 μm. A sample of six eggs per treatment was used to observe eggshell ultrastructure. Zn-Met 40, zinc-methionine at 40 mg/kg; Zn-Met 80, zinc-methionine at 80 mg/kg; ZnSO4 80, zinc sulphate at 80 mg/kg.

Fig. 3. Effects of dietary zinc-methionine (Zn-Met) supplementation on eggshell ultrastructure of laying hens. Values are means (six scanned images per sample, six samples per treatment) with standard errors represented by vertical bars. a,b,c Mean values with unlike letters were significantly different among the four treatments (P < 0·05). (B) , Mammillary knob width;
, mammillary thickness;
, palisade layer thickness;
, effective total thickness; (D)
, mammillary layer;
, palisade layer. Zn-Met 40, Zn-Met at 40 mg/kg; Zn-Met 80, Zn-Met at 80 mg/kg; ZnSO4 80, zinc sulphate at 80 mg/kg.
Correlation between eggshell strength and ultrastructure-related parameters (eggshell thickness, effective total thickness, mammillary knob width, mammillary thickness, palisade layer thickness, fibre diameter) for the 80 mg/kg Zn-Met group can be seen in Fig. 4. There was a significantly inverse correlation (P < 0·01) between eggshell strength and mammillary knob width for the 80 mg/kg Zn-Met group (Fig. 4(C)).

Fig. 4. Pearson correlation between eggshell strength and ultrastructure-related parameters ((A) eggshell thickness; (B) effective total thickness; (C) mammillary knob width; (D) mammillary thickness; (E) palisade layer thickness; (F) fibre diameter) for the 80 mg/kg zinc-methionine group.
Energy-dispersive X-ray spectroscopy determination
Fig. 5(A) illustrates the distribution of elements and Fig. 5(B) showed that C, O and Ca were the most dominant elements in transversal surface of eggshell, while relative atomic weight percentage of Ca was the highest in the eggshell of laying hens fed the 80 mg/kg Zn-Met diet. As for the linear distribution of Ca in the transversal surface of eggshell (Fig. 5(C)), the 80 mg/kg Zn-Met group had stronger signal intensity of Ca among the line (across mammillary layer, palisade layer, vertical crystal and cuticle sections) compared with the control and 40 mg/kg Zn-Met groups. Furthermore, the typical Ca in the control and ZnSO4 groups was unevenly distributed throughout all layers of eggshell compared with Zn-Met treatments (Fig. 5(D)).

Fig. 5. (A, B) The spectrum of elemental peaks energy-dispersive X-ray spectroscopy (EDS) and the percentage of relative atomic weight (calcium, oxygen, carbon, nitrogen, phosphorus, zinc). (C) Linear distribution of calcium in transversal surface of eggshell. Y: signal intensity of calcium, counts per s. (D) Planar distribution of calcium in transversal surface of eggshell. The same area was determined in transversal surfaces of all groups and contained all kinds of layers in eggshell (average the testing values of repeated measurements). Scale bars: transversal surface: 200 μm. Values are means (six samples per treatment). (B) , Calcium;
, oxygen;
, carbon;
, nitrogen;
, phosphorus;
, zinc. Zn-Met 40, zinc-methionine at 40 mg/kg; Zn-Met 80, zinc-methionine at 80 mg/kg; ZnSO4 80, zinc sulphate at 80 mg/kg.
Zinc contents in eggshell, egg white, yolk, serum, ovary, magnum and eggshell gland
Zn contents in the eggshell, egg white, yolk, serum and magnum significantly (P < 0·001) increased with increasing Zn-Met levels, except in ovary and ESG (Fig. 6(A) and (B)). Moreover, Zn contents in yolk (P < 0·05) and serum (P < 0·01) were highest in the 80 mg/kg Zn-Met group. In the 80 mg/kg Zn-Met and ZnSO4 diets, Zn contents had the same effects (P > 0·05) in eggshell, egg white, ovary, magnum and ESG.

Fig. 6. Effects of dietary zinc-methionine (Zn-Met) supplementation on zinc contents in eggshell, egg white, egg yolk, serum, ovary, magnum and eggshell gland of laying hens. Values are means (six samples per treatment) with standard errors represented by vertical bars. a,b,c,d Mean values with unlike letters were significantly different among the four treatments (P < 0·05). (A) , Egg white;
, egg yolk;
, eggshell; (B)
, serum;
, ovary;
, magnum;
, eggshell gland. Zn-Met 40, Zn-Met at 40 mg/kg; Zn-Met 80, Zn-Met at 80 mg/kg; ZnSO4 80, zinc sulphate at 80 mg/kg.
Activities of carbonic anhydrase and alkaline phosphatase, the concentrations of calcium, phosphorus, total protein and albumin in serum and eggshell gland
The activities of CA and ALP and the concentrations of Ca, P, TP and Alb are listed in Fig. 7. Ca and Alb levels in ESG (Fig. 7(A) and (D)) were the highest (P < 0·05) for laying hens fed the 80 mg/kg Zn-Met diet and showed no significant differences (P > 0·05) between the 40 mg/kg Zn-Met and 80 mg/kg ZnSO4 groups as well as CA activity in serum (Fig. 7(E)). Laying hens fed the Zn-Met diet had higher (P < 0·001) ALP activity in serum compared with those fed the other two diets (Fig. 7(F)).

Fig. 7. Effects of dietary zinc-methionine (Zn-Met) supplementation on the concentration of calcium (A), phosphorus (B), total protein (TP) (C) and albumin (Alb) (D), activities of alkaline phosphatase (ALP) (E) and carbonic anhydrase (CA) (F) in serum and eggshell gland of laying hens. Values are means (six samples per treatment) with standard errors represented by vertical bars. a,b Mean values with unlike letters were significantly different among the four treatments (P < 0·05). , Serum;
, eggshell gland. Zn-Met 40, Zn-Met at 40 mg/kg; Zn-Met 80, Zn-Met at 80 mg/kg; ZnSO4 80, zinc sulphate at 80 mg/kg.
RNA extraction, reverse transcription and real-time quantitative PCR
No significant (P > 0·05) effects among all treatments were observed in mRNA levels of OPN, ALP, ovocleidin-17 and ovocleidin-116 in ESG (Fig. 8(A)–(E)). The mRNA levels of CA and CaBP-D28k in the 80 mg/kg Zn-Met group were the highest (P < 0·001). In addition, mRNA levels of CA and CaBP-D28k showed no significant difference (P > 0·05) between the 40 mg/kg Zn-Met and 80 mg/kg ZnSO4 groups (Fig. 8(C) and (F)).
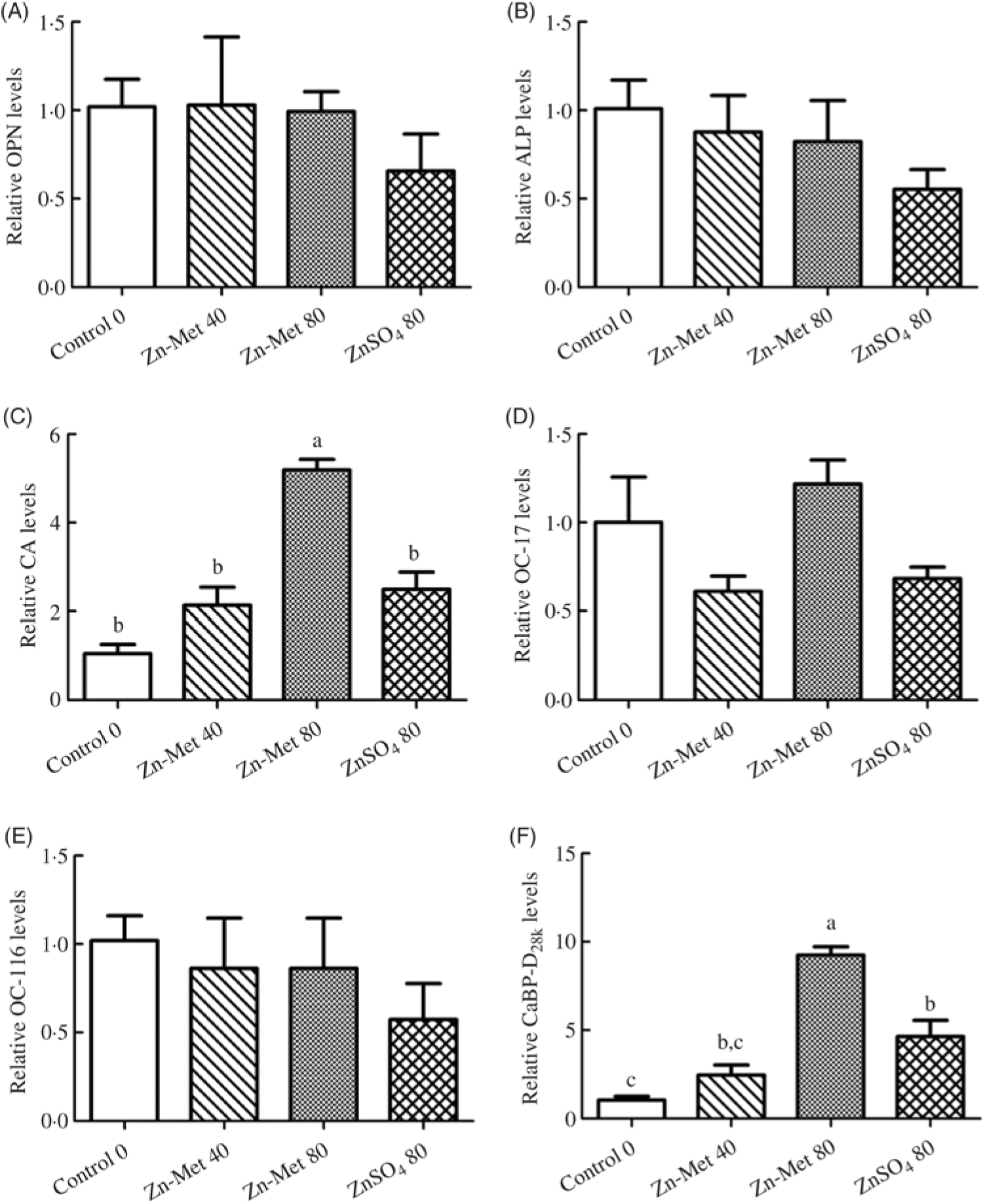
Fig. 8. Effects of dietary zinc-methionine (Zn-Met) supplementation on the mRNA levels of genes involved in eggshell quality in eggshell gland of laying hens. Values are means (six samples per treatment) with standard errors represented by vertical bars. OPN, osteopontin; ALP, alkaline phosphatase; CA, carbonic anhydrase; OC-17, ovocleidin-17; OC-116, ovocleidin-116; CaBP-D28k, calcium-binding protein-d28k. a,b,c Mean values with unlike letters were significantly different among the four treatments (P < 0·05). Zn-Met 40, Zn-Met at 40 mg/kg; Zn-Met 80, Zn-Met at 80 mg/kg; ZnSO4 80, zinc sulphate at 80 mg/kg.
Discussion
Studies on eggshell in laying hens have shown that supplementation of organic Zn, a Zn chelate, increased both eggshell thickness and eggshell strength in older hens(Reference Swiatkiewicz and Koreleski31,Reference Manangi, Vazques-Anon and Richards5) . The present study also showed that Zn-Met increased shell thickness and shell strength compared with ZnSO4 with the same addition (80 mg/kg) in the diet, while there was no difference in eggshell thickness and shell strength between the 40 mg/kg Zn-Met group and the ZnSO4 group. It was suggested that Zn-Met with lower supplementation in the diet could be used to replace the utilisation of inorganic Zn, given eggshell quality. Nevertheless, it was necessary to explore the material properties (inorganic constituent: ultrastructure; organic constituent: shell matrix proteins) that determine the increase in eggshell quality caused by Zn-Met supplementation.
It is known that the deposition of eggshell contains several stages(Reference Li, Zhang and Gong25). The mammillary knob layer follows the outer surface of the outer shell membrane and is the first calcified layer deposited, with its tips embedded in the outer shell membrane(Reference Carnarius, Conrad and Mast32). The palisade layer forms as the mammillary knobs fuse, and the vertical crystalline layer is the last calcified layer deposited, consisting of short crystals running perpendicular to the shell membrane(Reference Solomon33,Reference Hunton34) . Studies implied that shell quality is dependent upon the mammillary layer(Reference Bain35), which is opposite to the results of Fathi et al. (Reference Fathi, Zein El-Dein and El-Safty36) who suggested that the organisation of the columns of the palisade layer is one of the major determinants of the rigidity of the shell and the strength and shell resistance of the eggs. In our study, it was clearly shown that eggshell with the 80 mg/kg Zn-Met treatment had smoother external surface and thicker fibre diameter in internal surface as with smaller mammillary cones compared with the control and ZnSO4 groups. As for the transversal surface of eggshell, laying hens fed the Zn-Met diets had similar effects on the thickness of mammillary layer, palisade layer and effective total thickness compared with those fed the ZnSO4 diet. There was no difference in the percentage of the thickness of each layer (mammillary layer, palisade layer) to the effective total thickness. To explore more important factor for eggshell quality, the Pearson correlation between eggshell strength and ultrastructure-related parameters was performed. There was a significantly inverse correlation between eggshell strength and mammillary knob width for the 80 mg/kg Zn-Met group. Hence, the eggshell quality increased by Zn-Met treatments was mostly related to the mammillary cone width in our study.
Following the distribution of elements, it was found that Ca was one of dominant elements in transversal surface of eggshell, and the relative atomic weight percentage of Ca was the highest in the 80 mg/kg Zn-Met treatments in which Ca was more evenly distributed in transversal surface of eggshell. It was hypothesised that the differences in the eggshell quality may be eventually caused by Zn-induced changes in Ca deposits in eggshell, and the addition of Zn increases the utilisation of Ca in hens to improve the qualitative parameters of the eggshell(Reference Simons37). Consequently, in order to confirm the hypothesis, deeper examination of the Zn contents in eggshell, white, yolk, serum and tissues was undertaken.
In the present study, laying hens fed the 80 mg/kg Zn-Met diet had significant effects on Zn contents in eggshell, white, yolk, serum, ovary, magnum and ESG compared with those fed the control diet. This suggested that the changes in eggshell induced by Zn-Met supplementation were also reflected in Zn contents. Moreover, the better effects on Zn contents in serum and yolk were found in the 80 mg/kg Zn-Met group compared with the ZnSO4 group, which indicated that Zn-Met had greater bioavailability than inorganic Zn sources. To explore the mechanism of Zn-induced increased eggshell quality, we further analysed Ca, P, TP, Alb concentrations and activities of Zn-containing enzymes in eggshell formation.
Once the ovum, with the germ cell attached, is released from the ovary, it takes roughly 4–6 h to lay down the albumen, 75 min in isthmus to formulate the membranes and 18–20 h in ESG to lay down the shell(Reference Hunton34). Thus, ESG spending most time among all sections of the oviduct is important to eggshell formulation. Zn is an important component of the CA and ALP, and CA is one of the key enzymes of CaCO3 deposition inside the ESG; the de-phosphorylation of ALP can regulate expressions of some phosphorylated shell matrix proteins in the ESG(Reference Klecker, Zeman and Jelinek38). Our results indicated that Ca and Alb levels in ESG were found highest in the 80 mg/kg Zn-Met group but similarly between the 40 mg/kg Zn-Met group and ZnSO4 group, which was corresponding to the result of the relative atomic weight percentage of Ca in eggshell. Furthermore, the changes in increased enzymatic activities of CA and ALP in serum of the Zn-Met treatments might be attributable to the increased Zn accumulation in serum which was corresponding to the result of greater Zn contents in serum of the 80 mg/kg Zn-Met group. Therefore, the effect of Zn-Met supplementation on the eggshell quality was confirmed partly by Zn-induced Ca deposition. The determination of mRNA expression of proteins involved in eggshell formation is necessary to get more evidence of increased eggshell quality caused by Zn-induced Ca deposition in eggshell formation.
It was reported that CaBP-D28k has been detected in the tubular gland cell of ESG, and the expression of CaBP-D28k mRNA in the ESG increases during eggshell calcification(Reference Bar39). In addition, the avian eggshell primarily consists of CaCO3 mineral (calcite) and matrix proteins. Hincke et al. (Reference Hincke, Nys and Gautron40) reported that the organic matrix of the eggshell also could play a role in determining shell quality. A complex array of proteins exists in the uterine fluid and eggshell extract of Gallus gallus: egg white proteins (lysozyme, ovotransferrin and ovalbumin); bone matrix proteins (OPN and sialoprotein); specific proteins in uterus (ovocleidins and ovocalyxins)(Reference Hincke, Nys and Gautron40–Reference Hincke, Gautron and Panheleux43). Ovocleidin-17 is the major protein component of the eggshell matrix and one of the best candidates to regulate mineral deposition(Reference Reyes-Grajeda, Moreno and Romero44), which is revealed by immunohistochemistry in the mammillary and palisade layers(Reference Nys, Gautron and Garcia-Ruiz45). ovocleidin-116 is a major component of the chicken eggshell matrix observed throughout the palisade layer and most abundant in uterine fluid during the intense eggshell calcification phase(Reference Hincke, Gautron and Rodriguez-Navarro46,Reference Hincke, Nys and Gautron47) . Previous study indicated that ovocleidin-17 and ovocleidin-116 are eggshell-specific matrix proteins that have previously been shown to modulate shell calcification(Reference Rose and Hincke48). OPN is a highly phosphorylated, acidic, mineral-binding glycoprotein(Reference Chien, Hincke and Vali49), which is thought to play an important role in regulating mineralisation of avian eggshell – initially proposed because OPN gene expression correlates with the daily egg production cycle and reaches its maximal expression during the period of eggshell calcification(Reference Pines, Knopov and Bar50). In the present study, the mRNA levels of CA and CaBP-D28k showed no significant difference between the 40 mg/kg Zn-Met and 80 mg/kg ZnSO4 groups. Furthermore, the mRNA levels of CA and CaBP-D28k in the 80 mg/kg Zn-Met group were up-regulated, which suggests that CA and CaBP-D28k might be involved in Zn-induced Ca deposition in eggshell formation of laying hens. However, the exact mechanisms how Zn affects the Ca deposition by regulating expressions of CA and CaBP-D28k need to be further studied in future experiments.
In conclusion, shell strength as one of eggshell qualities was mostly related to mammillary cone width in ultrastructure caused by the pattern of Ca deposition in eggshell formation. And the increase in Zn-Met-induced Ca deposition may be due to the increased Zn contents in serum and tissues, which were attributable to the increased CA concentrations in serum, Ca, Alb levels and up-regulated CA and CaBP-D28k mRNA levels in ESG. Our observations also revealed the similar effects on shell thickness, shell strength, Zn contents, CA activity in serum and Ca, Alb levels, CA and CaBP-D28k mRNA levels in ESG between the 40 mg/kg Zn-Met group and ZnSO4 group. However, inorganic Zn replaced by organic Zn should be further investigated from every aspect. In addition, a substantial number of questions remain in fully understanding the avian eggshell quality; the precise chemical formation of the crystalline structure of the eggshell, the distribution of pores and processes behind termination of calcification all remain relatively unknown(Reference Wilson51) and need to be further analysed.
Acknowledgements
The present study was supported by the earmarked fund for Modern Agro-Industry Technology Research System of China (no. CARS-41-K17).
The authors’ contributions were as follows: L. L. and L. M. designed the experiment; L. L. drafted the manuscript and conducted the experiments and analysed the data; L. L., L. M., M. Z. and L. W. conducted the feeding trial and sample collection; X. Z. revised the manuscript; all authors have read and approved the final version of the manuscript.
The authors declare that there are no conflicts of interest.