INTRODUCTION
Between 1985 and 1991, increasing numbers of incidents of unusual mortality of the common frog (Rana temporaria) in Britain were reported unsolicited to one of the authors (A.A.C.). We studied this phenomenon from 1992 to 1997 and showed recurring annual epidemic mortalities of frogs occurred across much of Britain. These mortality events, which appear to kill many tens of thousands of frogs each year, occurred most commonly in the south and east of England, but extended to the north and west of the country [Reference Cunningham1, Reference Cunningham2]. Our investigations, including a detailed questionnaire survey of the owners of >3400 frog-breeding ponds, indicate that the mortalities had not been seen prior to the mid-1980s and are due to a previously unknown disease in Britain [Reference Cunningham1, Reference Cunningham2]. We identified two main disease syndromes: one characterized by skin ulceration [ulcerative syndrome (US)] and one characterized by systemic haemorrhages [haemorrhagic syndrome (HS)] [Reference Cunningham2]. Frogs also were found with lesions common to both of these syndromes (U+HS).
The HS and U+HS disease syndromes are consistent with ‘red-leg’ disease of amphibians, which has historically been regarded as having a primary bacterial (usually Aeromonas hydrophila) aetiology [Reference Anver, Pond, Fox, Cohen and Loew3–Reference Green, Wright and Whitaker5]. There has been speculation, however, that the bacteria isolated are post-mortem invaders [Reference Glorioso4, Reference Green, Wright and Whitaker5] or secondary pathogens [Reference Glorioso4–Reference van der Waaij, Cohen and Nace9]. Our investigations detected A. hydrophila more frequently in haemorrhagic frogs than in those with skin ulceration only, but iridovirus was detected in diseased tissues from frogs with each syndrome [Reference Cunningham2, Reference Drury, Gough and Cunningham10]. The association of iridovirus with lesions indicated that this virus group was implicated in the aetiology of the disease syndromes observed, possibly with A. hydrophila co-infection [Reference Cunningham2]. Subsequent molecular analyses confirmed the presence of virus belonging to the genus Ranavirus, family Iridoviridae, from both disease syndromes [Reference Cunningham1, Reference Hyatt11]. Ranaviruses were unknown in the British Isles prior to our investigations (we first identified the pathogen in Britain in 1995 [Reference Drury, Gough and Cunningham10]), but ranaviruses are known as important pathogens of fish and amphibians elsewhere, where they generally cause outbreaks of fatal systemic haemorrhagic disease [Reference Hyatt11, Reference Daszak12]. Ranaviruses are currently associated with amphibian mortality, but not long-term population declines [Reference Daszak, Cunningham and Hyatt13]. The potential for ranaviruses to impact amphibian populations, especially those previously naive to infection, however, should not be discounted. Molecular studies on the ranaviruses isolated in Britain indicate that the British viruses originate from North America [Reference Hyatt11].
In this paper, a series of transmission experiments is described to test whether the two disease syndromes described from frogs in Britain are (a) transmissible; (b) caused by ranavirus cultured from naturally diseased frogs; (c) caused by a common agent, by two distinct agents, or by a combination of agents and, (d) whether A. hydrophila is required for the production of either or both of these diseases.
METHODS
Animals
Adult common frogs were wild-caught in County Cavan, Eire for the transmission experiments. No reports of epidemic disease in wild amphibians, or of frogs with lesions consistent with ranavirus infection, have been reported from Ireland.
Following collection, the frogs were maintained in the laboratory for a minimum of 2 months prior to use to ensure they were disease-free. During this period, each frog was anaesthetized by immersion in a 0·2% aqueous solution of tricaine methane sulphonate (MS222, Thomson & Joseph Ltd, Norwich, UK) corrected to pH 7·0 using a 0·5 m aqueous solution of NaHCO3 [Reference Verhoeff-de Fremery, Griffin, Macgregor and Poole14], and was bled via cardiocentesis. Approximately 150 μl of blood was removed from each animal, and the serum was tested for anti-ranavirus antibodies using a competitive antibody capture ELISA [Reference Zupanovic15], for which the antibodies had been raised against epizootic haematopoietic necrosis virus (EHNV) and had been shown to react against all known ranaviruses [Reference Hyatt11, Reference Zupanovic15, Reference Hengstberger16]. Also, a random selection of sera was cross-checked via classical antibody decoration, as described by Zupanovic et al. [Reference Zupanovic15]. All animals were antibody-negative and suitable for use in the transmission experiments. The experiments were conducted under a Home Office Project Licence and with the approval of the Zoological Society of London's Ethics Committee.
Housing and husbandry
Animals were individually housed in cages containing damp autoclaved sphagnum moss and which were tilted to create a pool of distilled water. To prevent possible cross-infection, a new pair of disposable gloves was worn each time a frog was handled and when each cage was cleaned. A temperature of 18–22°C with a 12 h light cycle was maintained in order to replicate field conditions found at site investigations during months of peak mortality [Reference Cunningham1]. The frogs were fed on purpose-bred crickets (Gryllus bimaculatus).
Transmission experiments
During each experiment, great care was taken to avoid cross-contamination between frogs. Following the initial experimental procedure, each frog was inspected briefly on a daily basis and examined clinically every 3 days. Any animal found in distress was euthanized by immersion in a 0·4% aqueous solution of MS222 until anaesthetized, followed by stunning and pithing. All frogs exposed to virus or tissue homogenate were examined systematically at post-mortem examination and all such animals still alive at the end of each experiment [30 days post-exposure (p.e.)] were euthanized; due to logistical constraints, some frogs were not killed until several days after the end of the experiment. Two animals from each group of five control frogs used in experiments 1 and 2, and one control frog from experiment 3, were similarly killed and examined at the end of the respective experiments. Post-mortem examinations were conducted immediately following euthanasia or within 12 h of death. A summary of the infection regimes can be found in Table 1.
Table 1. Summary of infection regimes

US, Homogenate of tissue from frogs with ulcerative syndrome; HS, homogenate of tissue from frogs with haemorrhagic syndrome; with B, homogenate contains bacteria, including Aeromonas hydrophilia; without B, homogenate treated with antibiotics and bacteriologically sterile; RUK11, ranavirus isolated from a frog with HS (1, 11); RUK13 and isolate no. 23, ranavirus isolates from frogs with US (1, 11); I, immersion; W, wounded skin; i.p.+s.c., intraperitoneal and subcutaneous inoculation; i.v., intravenous inoculation.
Experiment 1. Exposure to lesions from naturally diseased frogs
Tissue samples (spleen, kidney, liver and oviduct) were pooled from 11 naturally diseased frogs collected from three separate sites (Ashford, Kent; Reigate, Surrey; Shoreham-by-Sea, West Sussex) of mortality where HS was the only disease found. These tissues, which had been archived at −20°C, were homogenized and suspended 20% w/v in 20 ml of sterile isotonic saline. The resulting homogenate was diluted in isotonic saline to make 320 ml stock (1/15) tissue suspension. (Thus, for each disease syndrome, the dilution of the original tissues in the stock suspension was 1/75.) This stock tissue suspension was split into two aliquots of 160 ml, to one of which was added gentamicin to a concentration of 0·05% and penicillin/streptomycin to a concentration of 1%. Similar tissue suspensions were produced using pooled tissues (ulcerated skin, spleen and kidney) from eight naturally diseased frogs from two separate sites (Croydon, Surrey; Weybridge, Surrey) of mortality where US was the only disease found.
For each tissue suspension (HS and US), the absence of bacteria in the aliquot containing antibiotics, and the presence of bacteria (including A. hydrophila) in the untreated aliquot, was confirmed using routine bacterial culture. The ranavirus titre was evaluated for each tissue suspension by inoculating a ten-fold dilution series in 24-well plates containing confluent layers of fathead minnow epithelial (FHM) cells [European Collection of Cell Cultures (ECACC) cell line no. 88102401]. Virus caused complete cell lysis within 12 days post-inoculation. The titre of the stock HS tissue suspension was 102 TCID50/ml, whilst that of the stock US suspension was 101·5 TCID50/ml. Two modes of exposure were used (immersion and wounded skin) in order to replicate possible natural routes of disease transmission.
Exposure via immersion
For each of the four tissue suspensions, five frogs were immersed in 250 ml of a 10% solution of stock tissue suspension in distilled water. Each frog was placed in a separate container with enough solution to cover its body, but not its head. A screw lid containing air holes was then secured over the top of the container. In addition, ten control frogs were mock-infected via immersion in distilled water only, at the same pH range as above. The animals were kept in these containers for 8 h before being placed into their respective cages.
Exposure via wounded skin
Exposure via wounded skin was used by Kunst & Valpotic [Reference Kunst and Valpotic17] to experimentally transmit a suspected iridoviral disease of the edible frog. For each of the four stock tissue suspensions, five frogs were anaesthetized (by immersion in a 0·2% solution of MS222, as above) and each had 1 cm2 areas of skin over the left femoral region and caudal right dorsum scarified using a new sterile scalpel blade for each frog. Approximately 1 ml of tissue suspension was immediately rubbed into each area of scarified skin. The same procedure was repeated on ten control frogs, with distilled water being used in place of tissue suspension. The sites of scarification were chosen because they are common sites for skin lesions in the natural disease [Reference Cunningham2].
Experiment 2. Exposure to ranavirus cultured from naturally diseased frogs
Two British ranavirus isolates were used in this series of experiments: isolate RUK11, cultured from the kidney of a wild frog naturally diseased with HS, and isolate RUK13, cultured from the femoral skin of a wild frog naturally diseased with US [Reference Hyatt11]. Both isolates had been cultured for three passages (P3) in FHM cells. The harvested cell culture fluid used contained 106·8 TCID50/ml for virus RUK11 and 106·2 TCID50/ml for virus RUK13. The isolates were not plaque-purified, as similar work with other viruses had shown this process can alter the pathogenicity of virus to the original host species [Reference Loeber and Dorries18]. Each of the following exposure methods was carried out for virus RUK11 and repeated for virus RUK13.
Exposure via immersion
Using individual containers, each of five frogs were immersed for 8 h in 250 ml of a 1% aqueous solution (using distilled water) of harvested virus in cell culture fluid, exposing the frogs to 104·8 TCID50/ml for virus RUK11 and 104·2 TCID50/ml for virus RUK13. Five frogs were similarly exposed to fluid harvested from equivalent wells of FHM cells without virus.
Exposure via intraperitoneal (i.p.) and subcutaneous (s.c.) inoculation
Five frogs were each inoculated intraperitoneally and subcutaneously (0·25 ml per site) with harvested virus in cell culture fluid, exposing the frogs to 106·2 TCID50 for virus RUK11 and 105·6 TCID50 for virus RUK13. Five frogs were similarly inoculated with fluid harvested from equivalent wells of FHM cells without virus.
Exposure via intravenous (i.v.) inoculation
Five frogs were each inoculated intravenously (sublingual vein) with 0·05 ml of harvested virus in cell culture fluid, exposing the frogs to 105·5 TCID50 for virus RUK11 and 104·9 TCID50 for virus RUK13. Five frogs were similarly inoculated with 0·05 ml fluid harvested from equivalent wells of FHM cells without virus. To facilitate venepuncture, the animals were anaesthetized by immersion in a 0·2% solution of MS222, as described above.
Experiment 3. Exposure, via wounded skin, to cultured virus or to lesions, following equalization of virus titres
In the experiments above, differences in disease outcomes were observed depending upon whether the source of virus was diseased tissue or cell culture. To determine if this difference was due to the different virus titres used (the virus titre from cell culture was approximately five orders of magnitude greater than the virus titre in homogenized tissues), frogs were exposed, via wounded skin, to equalized titres of cultured virus and virus in tissue homogenate. To do this, a 20% w/v suspension of tissue homogenate in isotonic saline was constituted (as above) from the ulcerated skin of a freshly dead wild common frog (ref. 937/96, from Worcester Park, Surrey) naturally diseased with US and the homogenate was stored at +4°C. Fresh homogenate was made as the RUK13 homogenate was no longer available by the time this experiment was conducted. Homogenate and cultured virus from a case of US was used as previous administration of US homogenate had produced only skin ulceration while virus cultured from US homogenate produced both skin ulcers and systemic haemorrhages. In contrast, both HS homogenate and virus cultured from HS homogenate produced both skin ulcers and systemic haemorrhages. Ranavirus (isolate no. 23) was cultured from an aliquot of the fresh US homogenate, passaged in FHM cells four times (P4), harvested and stored at +4°C. The virus titre was determined for both the tissue homogenate suspension and the harvested cell culture fluid. Differential dilutions in distilled water were made to provide equal virus titres (103·0 TCID50/ml) for each virus source. For each solution, five frogs were exposed to 1 ml via wounded skin using the methods described above. In addition, one frog was exposed to 1 ml of sterile saline only, via wounded skin.
Bacteriology
Samples of liver, spleen and small intestine were taken from each frog necropsied using aseptic techniques (skin, spleen and small intestine were taken from frogs used in experiment 3) and these were cultured aerobically for bacteria for 24 h and 48 h on 5% horse blood agar at 25°C. Bacteria isolated were identified using colony morphology, Gram's stain and the API biochemical strip system (API-bioMérieux UK Ltd, Basingstoke, Hampshire, UK).
Virology
Samples of kidney were taken from each frog necropsied, fixed in 2·5% glutaraldehyde or stored frozen, and examined for the presence of viruses using both transmission electron microscopy (TEM) and cell culture. Samples of ulcerated skin were taken for TEM from most frogs which developed this lesion.
Statistical analyses
The results of each experiment were tested for associations between disease production and exposure to tissue homogenates or to isolated virus using the χ2 test [Reference Kirkwood19]. Where the numbers involved were small, analyses were repeated using Fisher's exact test for small sample sizes [Reference Kirkwood19].
RESULTS
Experiment 1. Exposure to lesions from naturally diseased frogs
This experiment was conducted to find out if either of the two disease syndromes found in the wild (US and HS) are transmissible via routes that might occur naturally and, if so, if A. hydrophila is required for the production of either or both of these diseases. The results of this experiment are presented in Tables 2 and 3.
Table 2. Outcomes following exposure, via immersion, of frogs to lesions from naturally diseased frogs, with and without the presence of bacteria

* Euthanized; −, negative; +, positive
† viscera not including the gastrointestinal tract.
Table 3. Outcomes following exposure, via wounded skin, of frogs to lesions from naturally diseased frogs, with and without the presence of bacteria
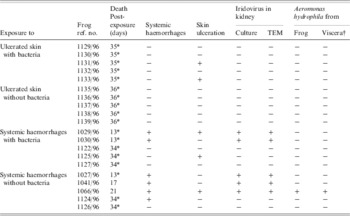
* Euthanized; −, negative; +, positive; † viscera not including the gastrointestinal tract.
Exposure via immersion
No frogs exposed to HS homogenate developed disease, regardless of the presence or absence of bacteria within the homogenate. Of frogs exposed to US homogenate, three developed US: one exposed to homogenate containing antibiotics, two exposed to untreated homogenate (Fig. 1, Table 2). Mock-infected frogs remained healthy.

Fig. 1. Frog ref. 1080/96 with ulcerated right tympanic membrane 24 days post-exposure, via immersion, to homogenized tissues from frogs with ulcerative skin syndrome. This frog also developed ulceration of the left maxilla and left rostrum.
Exposure via wounded skin
Seven frogs exposed to HS homogenate developed disease: four exposed to homogenate containing antibiotics, three exposed to untreated homogenate (Table 3). Lesions comprised those of HS (four frogs), US (one frog) and U+HS (two frogs). Of the frogs exposed to US homogenate, two developed US (Fig. 2): both frogs were exposed to untreated tissue homogenate. No mock-infected frogs developed disease.

Fig. 2. Frog ref. 1133/96 showing ulceration of the dorsal left thigh 35 days post-exposure, via wounded skin, to homogenized tissues from frogs with ulcerative skin syndrome.
Bacteriology
The non-gastrointestinal viscera of most of the frogs were sterile. A. hydrophila was not detected in any of the control frogs examined and was detected in only two frogs exposed to tissue homogenate: in the intestine of one that remained healthy throughout the experiment and in the spleen of one found dead with systemic haemorrhages. Neither of these animals had been exposed to the bacterium experimentally.
Virology
Of the animals exposed to tissue homogenate via immersion, no viruses were detected in the renal tissue, but ranavirus was found in ulcerated skin from one (frog ref. 1151/96) of three frogs examined with this lesion. Of the frogs exposed to tissue homogenate via wounded skin, ranavirus was detected in the kidneys of five of six animals that developed systemic haemorrhages and in ulcerated skin from two (refs 1029/96 and 1066/96) of five frogs examined that developed this lesion. No mock-infected frogs were positive for ranavirus.
Statistical analyses
There was an association between exposure to homogenized tissues from sick frogs and the development of disease (χ21=7·5, P<0·01), with 12 of 40 frogs thus treated developing lesions and none of 20 mock-infected frogs developing lesions. Statistical analyses were conducted to investigate the effects of (a) the method of exposure used (immersion vs. wounded skin), (b) the source of tissue homogenate used (US vs. HS) and (c) the presence of bacteria, specifically A. hydrophila, on the production of lesions. Neither of the last two parameters was found to affect the likelihood of disease development, but the method of exposure was significant, or close to significance, with exposure to tissue homogenate via wounded skin being more likely to result in disease than exposure via immersion (χ21=4·3, P<0·05; Fisher's exact test=0·08). Further analyses showed that, although the method of exposure did not affect the outcome for US homogenate (Fisher's exact test=1·00), it did significantly affect the outcome for HS homogenate (Fisher's exact test=0·003).
Although the source of homogenate did not affect the development of disease, it significantly affected the type of lesion produced (χ21=5·8, P<0·05; Fisher's exact test=0·03). Exposure to US homogenate produced US, but exposure to HS homogenate produced all three disease syndromes (HS, US and U+HS) observed in the field, with all but one of the affected animals developing systemic haemorrhages. Other possible causes (presence of bacteria, method of exposure) of producing this variation in lesions were insignificant.
Ranavirus was detected in tissues only from frogs that developed lesions post-exposure (Tables 2 and 3). These results demonstrate a strong association between the development of lesions and the presence of virus (χ21=20·6, P<0·001; Fisher's exact test <0·001).
Experiment 2. Exposure to ranavirus cultured from naturally diseased frogs
This experiment was conducted to find out if ranavirus cultured from each type of lesion (US and HS) found in the wild was the aetiological agent of these lesions. The results of this experiment are presented in Table 4.
Table 4. Outcomes following exposure of frogs to iridovirus cultured from naturally diseased frogs

* Euthanized; −, negative; +, positive; n.e., not examined; † viscera not including the gastrointestinal tract; i.p., intraperitoneal; s.c., subcutaneous; i.v., intravenous.
Exposure via immersion
All frogs exposed to virus RUK11 and four of five frogs exposed to virus RUK13 developed HS (Fig. 3), US, or U+HS, and died or were euthanized on welfare grounds within 19 days p.e. The remaining frog exposed to virus RUK13, and all mock-infected frogs, stayed healthy.

Fig. 3. Frog ref. 1022/96 which died of haemorrhagic syndrome 13 days post-exposure, via immersion, to virus RUK13. Note the petechial haemorrhages over the dorsal surface of the tongue. A few petechiae are also visible within the skin of the ventrum.
Exposure via i.p. and s.c. inoculation
All frogs exposed to virus developed systemic haemorrhages and died, or were euthanized, within 13 days p.e. One frog (ref. 0983/96) exposed to virus RUK13 also developed skin ulceration. The mock-infected frogs remained healthy.
Exposure via i.v. inoculation
All frogs exposed to virus developed HS (Fig. 4) and died, or were euthanized, within 15 days p.e. The mock-infected frogs remained healthy.

Fig. 4. Frog ref. 1020/96 which died of haemorrhagic syndrome 11 days post-exposure, via intravenous inoculation, to virus RUK13. (a) Note the diffusely haemorrhagic viscera and enlarged, congested spleen (centre of picture). (b) Close-up view of the caudal viscera: the urinary bladder (arrowhead) is diffusely haemorrhagic and one of the testes (arrow), which is erythematous with small haemorrhages over the surface, can be seen to the right of the intestine.
Bacteriology
The non-intestinal viscera of most frogs were sterile. A. hydrophila was detected in the small intestine only of five frogs (including one mock-infected frog) and in the non-intestinal viscera of two additional frogs.
Virology
Ranavirus was detected in renal tissue from each animal that developed disease. In addition, ranavirus was detected in ulcerated skin from all four frogs (refs 0983/96, 1035/96, 1040/96 and 1053/96) examined with this lesion. No mock-infected frogs were positive for ranavirus.
Statistical analyses
Of 30 frogs exposed to cultured virus, 29 developed disease and died or were euthanized, while all 30 mock-infected frogs stayed healthy (χ21=56·1, P<0·001). Ranavirus was detected from the kidney of all 29 diseased frogs, but not from the virus-exposed frog that remained healthy or from any of the mock-infected frogs examined. There was a strong positive association between the presence of virus and the development of lesions (χ21=42, P<0·001). The type of lesion produced was not affected by the presence of A. hydrophila, by the virus origin or by the method of exposure.
Experiment 3. Exposure via wounded skin to virus and to lesions, following equalization of virus titres
In the above experiments, exposure to virus cultured from skin ulcers produced systemic haemorrhages whereas exposure to virus within homogenized skin ulcers did not. As the virus titre in the homogenized tissues was much lower than in the tissue culture, experiment 3 was conducted using equalized virus titres from each source to find out if the difference in virus dose caused the difference in clinical outcomes. A summary of the results of this experiment is presented in Table 5. All five frogs exposed to cultured virus became diseased and died (two were euthanized) within 21 days p.e. Three animals died with HS, while two developed U+HS. In the latter, the skin ulceration was only at the site of femoral scarification. Only two frogs exposed to homogenate became sick. One died 16 days p.e. with systemic haemorrhaging and ulceration of both the femoral and dorsum areas of scarified skin. The other affected frog, which was killed at the end of the experiment, had relatively minor lesions, comprising a small (∼3×2 mm) area of skin ulceration between the left eye and nostril. The mock-infected frog remained healthy.
Table 5. Outcomes following exposure of frogs to equal titres of iridovirus from (1) diseased tissues and (2) culture virus (ref. no. 23) from naturally diseased frogs via wounded skin

* Euthanized; −, negative; +, positive; n.e., not examined; † viscera not including the gastrointestinal tract.
Bacteriology
Generally, a mixed bacterial growth was isolated from the skin and small intestine, whilst there was either a mixed culture or no growth from the spleen. A. hydrophila was isolated from the spleens of two frogs exposed to tissue homogenate and from two of the frogs exposed to cultured virus.
Virology
Ranavirus was detected in all of the tissues examined using TEM from four of the five frogs exposed to cultured virus. No virus was found in the remaining frog (ref. 588/98) in this group. Four of the five frogs exposed to homogenized tissue were virus-negative, but ranavirus was found in all tissues examined using TEM from the one frog (ref. 576/98) in this group that developed systemic disease. Corresponding results were obtained on virus culture. No virus was detected in the mock-infected frog tissues.
Statistical analyses
All animals exposed to cultured virus developed lesions (3 HS, 2 U+HS) and only two frogs exposed to tissue homogenate became sick (1 US, 1 U+HS). Frogs exposed to cultured virus were more likely to develop systemic haemorrhages than were frogs exposed to virus in homogenized US lesions (χ21=6·67, P=0·01; Fisher's exact test=0·048), but the likelihood of exposed frogs developing skin ulceration was not affected by the treatment given.
DISCUSSION
The three disease syndromes (US, HS and U+HS) found at mortality incidents investigated in the field were successfully replicated in the laboratory following exposure of frogs to either tissue homogenate or to cultured ranavirus from naturally diseased frogs. The progress of the diseases in the laboratory mimicked those found in the wild: frogs that developed HS died in the acute or peracute stages of the disease, whereas frogs with US developed lesions more slowly [Reference Cunningham1, Reference Cunningham2].
The HS and U+HS disease syndromes are consistent with descriptions of a disease of amphibians in North America called ‘red-leg’, which has historically been regarded as having a primary bacterial (usually A. hydrophila) aetiology [Reference Anver, Pond, Fox, Cohen and Loew3–Reference Green, Wright and Whitaker5]. In the current study, however, we have shown ranavirus to be the aetiological agent for these lesions and that neither disease outcome nor disease progression was influenced by the presence of bacteria, including A. hydrophila. Molecular studies suggest that ranavirus was introduced to Britain from North America [Reference Hyatt11]. Although ranaviruses have recently been shown to be important causes of amphibian mortality [Reference Daszak, Cunningham and Hyatt13, Reference Chinchar20, Reference Green, Converse and Schrader21], so far they have not been implicated as causative agents of amphibian population declines [Reference Daszak, Cunningham and Hyatt13]. However, further studies are required to elucidate the effects of this emerging infectious disease on frog populations in Britain.
While the experiments demonstrated that the disease syndromes seen in the field are transmissible and are caused by ranavirus alone, they also showed unexpected differences in the disease outcomes following either exposure to HS tissue homogenate via different routes or infection with ranavirus from different sources (Fig. 5). Although the disease outcomes from exposure to US tissue homogenate were not affected by the method of exposure (i.e. immersion vs. wounded skin), exposure to HS tissue homogenate via wounded skin was significantly more likely to result in disease than exposure via immersion (χ21=10·8, P=0·001; Fisher's exact test=0·003).

Fig. 5. Summary of the outcomes obtained following the experimental exposure of frogs to either homogenized tissues or cultured virus from naturally diseased frogs with each type of disease syndrome.
A greater proportion of animals developed disease, and more frogs died with systemic haemorrhages, following exposure to cultured virus than following exposure to homogenized tissues (Tables 2–4). As the method of exposure influenced the disease outcome for the HS tissue homogenate experiments but not the experiments using cultured virus, valid overall comparisons between these two types of treatment could not be made, but further comparisons between the results obtained from virus in US lesions and virus cultured from US lesions could be conducted.
A significantly greater proportion of animals became diseased following exposure to virus cultured from US tissues (RUK13) than to homogenized US tissues (χ21=16·13, P<0·001; Fisher's exact test <0·001). Also, exposure to RUK13 was more likely to cause the development of systemic haemorrhages than was exposure to homogenized US tissues (χ21=31·11, P<0·001; Fisher's exact test <0·001). No differences were found between the treatment used and the development of skin ulceration. One explanation for this could be differences in the amount of virus the frogs were exposed to; the virus titre in the stock suspension of US tissue homogenate (101·5 TCID50/ml) was five orders of magnitude lower than that in the tissue fluid harvested from cell culture (106·2 TCID50/ml) and perhaps higher doses of virus are more likely to lead to systemic infections that rapidly kill the host, while low doses of virus lead to chronic skin infections. However, when frogs were exposed to the same titre of virus via either homogenized US tissues or virus cultured from this same homogenate, once again exposure to cultured virus was more likely to produce systemic haemorrhages than exposure to homogenized tissues, while there was no difference in the likelihood of exposed frogs developing skin ulcers.
One explanation for the difference in disease outcomes following exposure to virus in tissue homogenate compared to exposure to cultured virus is that the presence of frog skin within the virus inoculum inhibits the ability of virus to infect systemically, even when given the opportunity via wounded skin. This could be, for example, due to the presence of antimicrobial peptides (AMPs) within the skin: inhibition of viruses, including the type ranavirus FV3, by peptides in frog skin has been demonstrated in vitro [Reference Chinchar22, Reference Van Compernolle23]. However, it would be improbable for AMPs to inhibit systemic infection but not cutaneous infection, as was seen in our experiments using homogenate. Also, inhibition by AMPs could not explain the differences in disease outcomes between exposure to virus in US tissue homogenate and to virus in HS tissue homogenate. In this case, the US virus (present in skin homogenate) was more virulent than HS virus (present in viscera homogenate) when exposure was via immersion. An alternative explanation for our findings is that the tissue tropism of US virus is altered upon passage in cell culture to resemble the tissue tropism of HS virus. This could occur if US ‘virus’ existed as a heterogeneous population of viruses of different genetic composition which underwent selection in vitro or if passage in cell culture altered the expression of a specific gene (or genes) involved in the recognition of systemic cell receptors.
A previous study on EHNV, a ranavirus of fish, showed that all 12 isolates examined had identical amino-acid sequences for the major capsid protein (MCP), but differed in their restriction fragment length polymorphism (RFLP) profiles [Reference Hyatt11]. It was concluded that EHNV exists within the environment as a heterogeneous population. It is possible that a similar situation exists with the British ranavirus, RUK. Studies on RUK11 and RUK13 have shown these viruses to be members of the genus, Ranavirus, and that both isolates are identical at the C′-terminal 163 amino-acid coding sequence of the MCP as well as at the 3′-terminal non-coding regions [Reference Hyatt11]. Subtle differences were observed, however, between RUK11 and RUK 13 on analysis of the virus genomes using RFLP profiles and sequencing and of the virus proteins using SDS–PAGE [Reference Cunningham1, Reference Hyatt11]. Thus, the genomes of these two isolates are not identical.
Genetic variation is a critical feature of all living organisms and the same is true for viruses. With respect to viruses, genetic variation provides potential to infect, and replicate in, new hosts [Reference Domingo24–Reference Vinuela31]. Many of the reported examples of closely related but not identical (variable) viral populations that evolve in this manner are for RNA viruses. Such heterogeneous populations of RNA viruses are frequently termed quasi-species [Reference Domingo25]. The variability is attributed to RNA polymerases, which lack proof-reading and error editing functions, resulting in a high rate of nucleotide mis-incorporation in RNA viruses [Reference Steinhauer and Holland32, Reference Steinhauer, Domingo and Holland33]. In contrast, DNA viruses possess proof-reading and repair functions to reduce the intrinsic error rate [Reference Parrish34]. However, as DNA viruses also replicate rapidly and prodigiously, viral populations of closely related genomes are generated [e.g. 25, 31, 34, 35] and studies with small DNA viruses have shown passage in cell culture to produce rapid selection of variants [Reference Loeber and Dorries18, Reference Markowitz36, Reference Sugimoto37]. Such genetic variation and adaptation can include alterations in tissue tropism, host-range and virulence [Reference Domingo24, 26–29, 38–Reference McKnight40]. Studies with small DNA viruses have shown passage in cell culture to produce rapid selection of variants [Reference Loeber and Dorries18, Reference Markowitz36, Reference Sugimoto37]. Within the current study, the pathogenicity of ranavirus from frogs with US changed (increased virulence, possible altered tissue tropism) during tissue culture to become similar to that of ranavirus from frogs with HS (Fig. 5). This phenomenon was unexpected and requires further investigation, including studies to determine the extent of genetic variation in the US and HS ranavirus populations and the rate at which genetic modifications might occur. Such knowledge will be important in understanding the virulence, host range and evolution of ranaviruses.
ACKNOWLEDGEMENTS
We thank Mike Lovett, Tracy Howard, Gill Bell and Shaheed Macgregor for technical assistance and Tom Langton for his support and advice. This work was approved by the Zoological Society of London's Ethics Committee and was partly funded by a seed grant from the IUCN Declining Amphibian Populations Task Force to A.A.C.
DECLARATION OF INTEREST
None.