Cow's milk is a complex biological fluid and a nutritive food regarding human health. Milk fat is composed of many neutral and polar lipids (Table 1) and is structured as fat globules emulsified in the aqueous phase. The milk fat globules are surrounded by a native biological membrane (Fig. 1) composed mainly of phospholipids, proteins and enzymes, cholesterol, glycoproteins and vitamins: the milk fat globule membrane (MFGM; Danthine et al. Reference Danthine, Blecker, Paquot, Innocente and Deroanne1999; Mather, Reference Mather2000). The role of milk fat in contributing to health and disease is quite controversial (Berner, Reference Berner1993a ; Gurr, Reference Gurr and Fox1995; Maijala, Reference Maijala2000; German & Dillard, Reference German and Dillard2006). SFA represent 60–70 g/100 g total fatty acids in cow's milk and unsaturated fatty acids 30–35 g/100 g total fatty acids (mainly MUFA; Jensen, Reference Jensen2002). While SFA and cholesterol contents are suspected to take part in the risk of CHD, some milk lipids such as conjugated linoleic acids, sphingomyelin (from the MFGM) and butyric acid would present anticarcinogenic properties (Parodi, Reference Parodi1997; Pfeuffer & Schrezenmeir, Reference Pfeuffer and Schrezenmeir2000; Lee et al. Reference Lee, Lee, Cho and Kim2005; Rombaut & Dewettinck, Reference Rombaut and Dewettinck2006). Regarding proteins, four casein classes coexist (αs1, αs2, β, κ), organized as so-called micelles, the structural models of which are actively discussed (Horne, Reference Horne2006). The κ-casein is mainly located at the surface of the micelle, held together by calcium phosphate bonds (Walstra, Reference Walstra1990). The soluble proteins, also called whey proteins, represent only 15–22 % of milk proteins but form a complex group consisting of albumins, Ig and proteose-peptones. β-Lactoglobulin (β-Lg) is the most abundant whey protein (51 %) and can bind and transport small hydrophobic molecules such as retinol (Whitney, Reference Whitney and Wong1988; Jensen, Reference Jensen1995). Individual milk proteins are reported to present a wide range of beneficial health and functional effects via bioactive peptides, such as anticarcinogenic effects, enhancement of certain physiological functions (Meisel, Reference Meisel2005; Silva & Maltaca, Reference Silva and Maltaca2005), improved iron bioavailability (Bouhallab & Bougle, Reference Bouhallab and Bougle2004), lowered blood pressure (Pfeuffer & Schrezenmeir, Reference Pfeuffer and Schrezenmeir2000; Murakami et al. Reference Murakami, Tonouchi, Takahashi, Kitazawa, Kawai, Negishi and Saito2004) or prevention of dental caries (Aimutis, Reference Aimutis2004) and might be used as nutraceuticals in the treatment of diarrhoea, thrombosis or immunodeficiency (Séverin & Wenshui, Reference Séverin and Wenshui2005). On the other hand, milk proteins are also reported to be important food allergens (Host, Reference Host2002) and are suspected to be involved in some diabetes cases (Schrezenmeir & Jagla, Reference Schrezenmeir and Jagla2000). In this respect, the issue of milk as a fully healthy or potentially harmful foodstuff remains controversial in many aspects (Maijala, Reference Maijala2000; Pfeuffer & Schrezenmeir, Reference Pfeuffer and Schrezenmeir2000) and can display variability among individuals of different ages and ethnic groups.
Table 1 Gross composition of milk lipids (adapted from Walstra et al. Reference Walstra, Geurts, Noomen, Jellama and van Boekel1999; Jensen, Reference Jensen2002; Michalski & Januel, Reference Michalski and Januel2006)

MFGM, native milk fat globule membrane.
* TAG reported to be found in the MFGM are likely to be residues from the core because MFGM separation procedures are imperfect (Walstra, Reference Walstra1974).
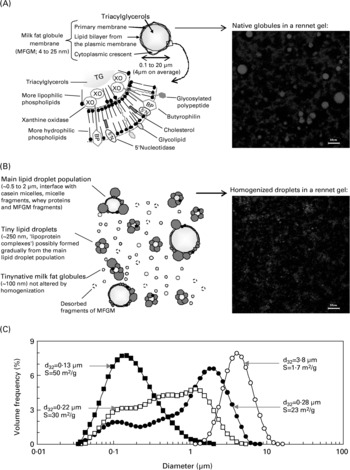
Fig. 1 (A) Ultrastructure of the native milk fat globules and their membrane (MFGM; not all proteins are shown here, see Table 2 and Mather, Reference Mather2000; not drawn to scale). Insert shows native milk fat globules (red) in the casein matrix (blue) of a rennet gel observed by confocal laser scanning microscopy (black areas are the serum pores of the gel; scale bar = 10 μm). (B) Proposed general organization of the lipid particles in homogenized milk:, native milk fat globule;, casein micelle;, fragment of casein micelle;, whey protein;, fragments of MFGM (not drawn to scale). Insert shows fat droplets of milk homogenized at 10 MPa in the casein matrix of a rennet gel observed by confocal laser scanning microscopy (scale bar = 10 μm). (C) Fat droplet size distribution, volume-surface mean diameter (d32) and specific surface area (i.e. surface area per g of fat, S) of: ○, raw whole milk; ●, whole milk homogenized at 5 MPa; □, whole milk homogenized at 10 MPa; ■, whole milk homogenized at 50 MPa. Adapted from Michalski et al. (Reference Michalski, Michel and Geneste2002a , Reference Michalski, Cariou, Michel and Garnier b , Reference Michalski, Michel, Briard and Anton c ) and Michalski & Januel (Reference Michalski and Januel2006).
Raw milk is subjected to various processing steps for preservation along the supply chain (Michalski & Januel, Reference Michalski and Januel2006). Particularly, commercial liquid milk is commonly homogenized and heat treated. Homogenization results in the most profound changes in the physical structure of milk fat and might result in altered health properties of milk, as often speculated. Homogenization consists of forcing hot pressurized milk between a valve needle and seat. This results in a dramatic reduction in fat globule size that greatly decreases the cream separation rate (Walstra, Reference Walstra2003). While the milk emulsion is thus more stable and shelf-life increases, intensive heat treatments (pasteurization or ultra-high temperature process (UHT)) are still necessary to preserve product microbiological quality (Hui, Reference Hui and Hui1993). Lacroix et al. (Reference Lacroix, Léonil, Bos, Henry, Airinei, Fauquant, Tomé and Gaudichon2006) observed no correlation between the nutritional quality of separated milk proteins in rats and the various heat treatments. However, heat treatments enhance the impact of homogenization on the organization of whole milk (Michalski & Januel, Reference Michalski and Januel2006) and possibly on its health properties.
After summarizing the consequences of homogenization on milk structure known to date, we will thus review the possible effects of homogenization on the health properties of milk through available literature evidence or suggestions.
Consequences of homogenization on milk components and organization
The structure of fat globules and the composition of their MFGM is altered by homogenization and heat treatments (Mulder & Walstra, Reference Mulder and Walstra1974). Indeed, the rupture of fat globules creates a new interface that cannot be entirely covered by the MFGM so that other surface active components adsorb and form a new membrane around the fat droplets (Darling & Butcher, Reference Darling and Butcher1978). Casein micelles are reported to be the major protein fraction adsorbed: they would spread onto the fat surface when colliding during homogenization, even if part of the native MFGM remains associated with the fat droplets (Jackson & Brunner, Reference Jackson and Brunner1960; Michalski & Januel, Reference Michalski and Januel2006). Fig. 1 shows a schematic of the organization of the native milk fat globule and the MFGM (Fig. 1(A)) and of the newly created fat droplets in homogenized milk (Fig. 1(B)) and shows corresponding visualizations by confocal microscopy in dairy gels (Fig. 1 inserts). Fig. 1(C) presents examples of fat droplet size distributions. The native milk fat globule size distribution usually spans from < 1 μm to ~20 μm, with an average volumic diameter initially around 3–5 μm. Upon homogenization, the latter is reduced to around 1 μm and the interface between fat droplets and the aqueous medium increases dramatically (Fig. 1(C); Michalski et al. Reference Michalski, Michel and Geneste2002a ). In commercial pasteurized or UHT full-fat milk, the reported volume moment mean diameter (d43) is in the range 0·39–0·43 μm (Lopez, Reference Lopez2005). Favé et al. (Reference Favé, Coste and Armand2004) report d43 = 0·46–1·02 μm in half-skimmed commercial UHT milk v. 4·36 μm in whole pasteurized organic milk.
Furthermore, various interactions and complex formations occur between the different caseins and whey proteins upon milk heating as detailed by Michalski & Januel (Reference Michalski and Januel2006). During homogenization some MFGM components are displaced to the skim milk phase (Fig. 1(B); Keenan et al. Reference Keenan, Moon and Dylewski1983). Bovine xanthine oxidase (BXO) and butyrophilin are covalently bound to fatty acids, contributing to preserve the affinity of these MFGM proteins with the interface. If milk is homogenized then pasteurized, caseins (mainly β and κ) represent 99 % of adsorbed proteins (Zahar & Smith, Reference Zahar and Smith1996). In infant formula, pasteurization favours β-Lg–κ-casein interactions and late homogenization favours the interaction of caseins and whey proteins with the fat droplet membrane (Guo et al. Reference Guo, Hendricks and Kindstedt1998). When pasteurized milk is homogenized, whey proteins make up about 5 % of adsorbed proteins and about 20 % of the surface area covered (Sharma & Dalgleish, Reference Sharma and Dalgleish1994). The following can occur, depending on whether heat treatment is performed before (1) or after (2) homogenization: (1) denatured whey proteins can interact with the native MFGM proteins and micellar caseins (particularly κ-casein), and the casein–whey protein complexes adsorb on to the lipid droplet interface, or (2) the semi-intact casein micelles or micellar fragments can cover the fat droplet interface, and the denatured whey proteins link to the native MFGM proteins and to adsorbed caseins via disulphide bonds (Michalski & Januel, Reference Michalski and Januel2006). These structural changes can affect the health value of milk components.
Impact of homogenization on milk digestion?
The structure of milk is likely to affect the digestion process. Indeed, during digestion of homogenized milk, a simultaneous coagulation of caseins and lipid droplets can occur in the stomach. This reaction would differ from untreated milk digestion, because the structure of coagulated matter is much finer and the casein-coated lipid droplets are likely to interact with the casein matrix of the gastric clot as observed during milk gelation (Michalski et al. Reference Michalski, Cariou, Michel and Garnier2002b ; inserts in Fig. 1(A, B)). There is some evidence that the protein transfer to the small intestine may be easier. For example in subjects suffering from intestinal disease, homogenized milk is reported to be more easily digestible than untreated milk (Sieber et al. Reference Sieber, Eyer and Luginbuhl1997). Using a minipig model, Meisel & Hagemeister (Reference Meisel and Hagemeister1984) show that raw milk and pasteurized milk give a very firm curd and present slower gastric emptying rate than pasteurized homogenized milk, UHT milk or cultured milk. With a soft curd as given by UHT milk, the milk constituents empty much more rapidly from the stomach. Higher acidity, higher pepsin activity and increased amount of peptic degradation products were also observed compared with raw milk or pasteurized milk, using the minipig model (Meisel & Hagemeister, Reference Meisel and Hagemeister1984). This also results in a greater absorption rate from UHT milk (Kaufmann, Reference Kaufmann1984). Moreover, the proteolysis of casein is enhanced with pasteurized homogenized milk and UHT milk (Pfeil, Reference Pfeil1984). Still using the minipig model, Buchheim (Reference Buchheim1984) shows that a firm coagulation of casein occurs with raw and pasteurized non-homogenized milk. When pasteurized homogenized milk is fed, the direct incorporation of fat globules in the network structure limits the aggregation of casein. In Buchheim's study, UHT homogenized milk showed a reduced tendency to aggregation of casein and fat globules during its presence in the stomach. However, it is difficult to dissociate the effects of heat treatment and homogenization.
The gastric step is of tremendous importance for lipid digestion because it is known to facilitate the subsequent TAG hydrolysis by the pancreatic lipase (Favé et al. Reference Favé, Coste and Armand2004). This is particularly important in infants and in adult patients suffering from pancreatic insufficiency. In minipigs, Buchheim (Reference Buchheim1984) observed by electron microscopy extended lamellar structures seeming to escape from fat droplets in the gastric coagulum of pasteurized milk (homogenized as well as non-homogenized) and of UHT milk, but only rarely in raw milk and in cultured milk. These lamellae are reported by Buchheim (1984) to be typical of monoacylglycerols, providing direct evidence for lipolysis which occurs to a considerable extent in these milks. Regarding subsequent gastric lipolysis in minipigs, after feeding raw milk and cultured milk that were both pasteurized and homogenized, only slight lipolysis is observed (Timmen & Precht, Reference Timmen and Precht1984). Feeding homogenized pasteurized milk, non-homogenized pasteurized milk and UHT milk results in production of both partial glycerides and NEFA of up to 15–30 % of the total fat (Timmen & Precht, Reference Timmen and Precht1984). Armand and colleagues (Armand et al. Reference Armand, Pasquier, André, Borel, Senft, Peyrot, Salducci, Portugal, Jaussan and Lairon1999; Favé et al. Reference Favé, Coste and Armand2004) have shown in man that the lipid droplet size is a key physico-chemical factor governing fatty acid bioavailability: smaller droplets result in greater lipolysis providing a larger interface area. We can thus postulate that the small droplet size in homogenized milk would thus favour milk fat lipolysis. However, the composition of the fat droplet interface is also important, because lipases must gain access to the TAG through this interface. For example, in premature infants, human milk fat globules (surrounded by a human native MFGM similar to that schematized in Fig. 1) are reported to result in a more efficient gastric lipolysis than the much smaller homogenized lipid droplets of infant formula (Favé et al. Reference Favé, Coste and Armand2004). Human milk fat globules are larger in colostrums (volume-surface mean diameter d32 = 4·3 μm) than in mature breastmilk (d32 = 3·5 μm), and much larger in general than infant formula droplets (d32 = 0·3 μm; Michalski et al. Reference Michalski, Briard, Michel, Tasson and Poulain2005a ). The ultrastructure of milk fat droplets appears thus to be of utmost importance (Favé et al. Reference Favé, Coste and Armand2004), it can be related to the physico-chemical changes due to homogenization and heating that could affect lipase access to the interface. We have recently shown in the rat that consumption of dairy creams with 20 % fat containing small homogenized fat droplets results in a slower TAG metabolization than consuming creams containing large phospholipid-coated droplets or than consuming unemulsified fat plus skimmed milk (Michalski et al. Reference Michalski, Leconte, Briard, Fauquant, Maubois and Goudédranche2005b , Reference Michalski, Michel and Geneste2006a ). In man, small droplets are more efficiently lipolysed (Armand et al. Reference Armand, Pasquier, André, Borel, Senft, Peyrot, Salducci, Portugal, Jaussan and Lairon1999) but the slower metabolization can be linked to the delayed gastric emptying due to the gastric clot structure. We recently described a microfiltration process that provides a milk fraction containing native milk fat globules of smaller size than in usual raw milk (d43~2–2·5 μm; Michalski et al. Reference Michalski, Leconte, Briard, Fauquant, Maubois and Goudédranche2006b ; also Goudédranche et al. Reference Goudédranche, Maubois and Fauquant1998). The digestibility of milk containing small native milk fat globules has to be compared with that of milk containing homogenized fat droplets of similar size. The latter can also be produced by homogenizing anhydrous milk fat with native micellar casein and whey protein isolate. This would allow discrimination between size and interface composition effects (Michalski et al. Reference Michalski, Soares, Lopez, Leconte, Briard and Géloën2006a ). The difference in gastric emptying with the minipig studies may be due to the different animals used and the different fat content of the gastric clots. Long-term effects of these metabolization differences compared with untreated milk fat globules are still to be investigated.
Impact of homogenization on atherosclerosis and CHD?
Atherosclerosis
Atherosclerosis is the partial or total obstruction of arteries due to the formation of plaques rich in cholesterol at the internal face of the vascular wall. However, the role of cholesterol- and SFA-rich foods such as butter, milk and fatty meat in promoting atherosclerosis is still controversial. Obviously, the effects of any single fat source will be diluted when in a mixed diet (Berner, Reference Berner1993b ). Furthermore, by considering only three fatty acid groups (SFA, MUFA and PUFA), the fact that within one group different fatty acids do not act the same way is ignored. Berner (Reference Berner1993b ) also highlights that the impact of milk products on plasma lipids and on the risk of CVD is different from expected considering their lipid content and composition.
An exaggerated postprandial lipaemic response is a risk factor for development of atherosclerosis. In this respect, some studies have recently shown that different structured dairy products result in different lipaemia profiles. Consequently, it may not be justified to qualify milk as anti- or pro-atherogenic regarding solely its lipid composition. Clemente et al. (Reference Clemente, Mancini, Nazzaro, Lasorella, Rivieccio, Palumbo, Rivellese, Ferrara and Giacco2003) have shown that milk, mozzarella cheese and butter in test meals did not result in the same timing of TAG peak in type 2 diabetic patients. Sanggaard et al. (Reference Sanggaard, Holst, Rehfeld, Sandstrom, Raben and Tholstrup2004) compared the effect of whole milk with fermented milk (certainly both homogenized although not stated) on postprandial lipid metabolism in man. Fermented milk resulted in a slower gastric emptying rate than regular milk, and in a greater increase and a quicker decrease of the TAG content in all lipoprotein fractions. Controlled dietary studies in man have shown no difference in the effect on plasma cholesterol of milk and butter with equal fat content and adjusted regarding lactose and casein content (Tholstrup et al. Reference Tholstrup, Høy, Normann Andersen, Christensen and Sandström2005). In a careful review of recent observational and human intervention trial findings on dairy products and CVD, Tholstrup (Reference Tholstrup2006) concludes that there is no strong evidence that dairy products (i.e. including homogenized milk) increase the risk of CVD in healthy men of all ages or young and middle-aged healthy women. Moreover, there seems to be a neutral effect of regular hard cheese consumption regarding plasma cholesterol. We should point out that hard cheese, like most cheeses, is produced from unhomogenized milk as opposed to commercial liquid milk. However, to date there are no studies available simply comparing unhomogenized and homogenized milk products in man. Different types of cheese should also be compared. Moreover, hard cheese may contain beneficial bioactive peptides released by enzymes and bacteria during cheesemaking (Gobbetti et al. Reference Gobbetti, Stepaniak, De Angelis, Corsetti and Di Cagno2002). Finally, the lipid ultrastructure of hard cheese, in which fat globules are partly destroyed (Lopez, Reference Lopez2005), as well as its high calcium content might play a role. Barba et al. (Reference Barba, Troiano, Russo, Venezia and Siani2005) recently reported a significant inverse association between the frequency of whole milk consumption and BMI in children that can subsequently have an impact on obesity and atherosclerosis. They highlight that this association was no longer significant when children consuming skimmed milk were included in the analysis. Barba et al. considered their results as the possible beneficial role of bioactive peptides and calcium, but we wonder whether homogenization might have had an effect. We recently found in rats that plasma TAG appear faster, and as a sharper peak, after consumption of anhydrous milk fat plus skimmed milk than after consumption of unhomogenized dairy cream or homogenized dairy cream of similar composition (Michalski et al. Reference Michalski, Soares, Lopez, Leconte, Briard and Géloën2006a ). A hint of a higher TAG peak was observed for unhomogenized cream compared to homogenized cream, but these results remain to be confirmed in man. Sodium caseinate added to a fatty meal was also found to lower NEFA in the postprandial and postabsorption phases, and also to reduce moderately postprandial lipaemia, probably via its insulinotropic activity (Westphal et al. Reference Westphal, Kastner, Taneva, Leodolter, Dierkes and Luley2004). However, we must point out that sodium caseinate may result in different effects from casein micelles. Since homogenization affects casein organization among the aqueous and fat phases, the hypothesis of different casein effects on postprandial lipaemia depending on milk treatment should be tested. No difference was found between skimmed milk and whole milk (commercial thus obviously homogenized) regarding their glycaemic and insulinaemic indexes in man (Hoyt et al. Reference Hoyt, Hickey and Cordain2005). However, these parameters should be measured after raw whole milk consumption in order to test for a possible processing effect. Overall, studies are needed in man to investigate the effect of homogenization on the anti- or pro-atherogenic properties of milk.
We must point out that Oster (Reference Oster and Dhalla1972) found BXO antibodies in the serum of patients with atherosclerotic disease. Thus, Oster hypothesized that BXO released from the MFGM due to homogenization would favour atherosclerosis and other coronary diseases. Indeed, BXO is present in high amounts in milk, associated with the MFGM (Briley & Eisenthal, Reference Briley and Eisenthal1975; Fig. 1(A)). BXO activity is heat resistant up to 78°C and increases with homogenization pressure (Demott & Praepanitchai, Reference Demott and Praepanitchai1978). The enzyme would become more available due to the reduced size and increased number of fat droplets after homogenization. BXO adsorbed onto homogenized fat droplets would then cross the intestinal wall and enter the systemic circulation with subsequent access to arterial walls and the myocardium, possibly contributing to deleterious effects such as myocardial infarction (Oster, Reference Oster and Dhalla1972). However, this hypothesis is criticized for many reasons such as inactivation at acidic pH like in the stomach (Mangino & Brunner, Reference Mangino and Brunner1976). Ho & Clifford (Reference Ho and Clifford1977) have also shown that high amounts of BXO injected intravenously to rabbits do not result in enzyme detection in the heart, aorta, liver or kidneys. Other recent studies emphasize the role of BXO in the generation of reactive oxygen species in the cardiovascular system (Berry & Hare, Reference Berry and Hare2004). By catalysing the oxidation of aldehydes into acids, BXO causes the release of hydrogen peroxide that can generate highly reactive radicals. All these reactive oxygen species are formed in trace amounts under physiological conditions but are eliminated quickly by the cell antioxidant system. When their production increases and/or antioxidant defences are no longer sufficient, reactive oxygen species can attack various cellular targets (lipids, proteins, DNA) and cause multiple injuries. Therefore, the possibly harmful effects of BXO have been the subject of questions to the European Parliament in September 2000 and April 2001 (written questions E-2907/00 and E-0864/02), to which the Commission responded not to have sufficient proof. Today, even if BXO has not been proven to display any major role in atherosclerosis, this hypothesis remains under discussion (Hajjar & Leopold, Reference Hajjar and Leopold2006) and insidious long-term effects in some individuals, maybe promoted by milk processing, cannot be ruled out.
CHD
CHD is due to the development of arteriosclerosis in coronary arteries, characterized by the deposit of calcified plaques in the internal wall of the coronay arteries leading to stenosis. In an extensive review of the functional peptides derived from casein and present in milk, Silva & Maltaca (Reference Silva and Maltaca2005) explain that some casein-derived peptides present antithrombotic and antihypertensive features. The κ-casein fragment f103-111 can prevent blood clotting through inhibition of platelet aggregation. Also, Elwood et al. (Reference Elwood, Pickering, Hughes, Fehily and Ness2004) recently reviewed cohort studies and reported that milk drinking may be associated with a small but worthwhile reduction in heart disease and stroke risk, even though residual confounding from unidentified factors could not be ruled out. Particularly, the definition of ‘milk intake’ presents some difficulties and according to Tholstrup (Reference Tholstrup2006), the study would have been more valuable if a proper meta-analysis had been carried out. Warensjö et al. (Reference Warensjö, Jansson, Berglund, Boman, Ahren, Weinehall, Lindahl, Hallmans and Vessby2004) demonstrated that milk product intake, estimated by the proportion of ruminant-derived odd size fatty acids 15:0 and 17:0 in serum lipid esters, was negatively associated with CVD risk factors. No unfavourable effect of dairy products was found, although the negative correlation disappeared after adjustment for clinical risk factors. All these studies concern the consumption of commercial, heat-treated and homogenized milk. Since homogenization and heat treatments involve the reorganization of casein components within the milk structure, the effect of each milk processing factor should be examined with respect to the desirable bioactivity of casein-derived peptides.
Moss & Freed (Reference Moss and Freed2003) recently studied the link between CHD occurrence and circulating antibodies against the MFGM. They found that death rates due to CHD were positively correlated with milk consumption and might be linked to the circulating antibodies against the MFGM proteins; the latter link to lymphocytes and platelets, which would cause their aggregation. Moss & Freed (Reference Moss and Freed2003) conclude that the MFGM proteins could be atherogenic. This hypothesis was recently criticized by Spitsberg (Reference Spitsberg2005) on analytical grounds regarding the relevance of the tested MFGM protein antibodies. Since homogenization changes the organization of the MFGM and the exposure of its proteins, we suggest that this treatment could influence the putative atherogenic effect of these proteins. However, studies remain to be performed to elucidate this point. Also, hard cheese consumption is negatively correlated with CHD (Moss & Freed, Reference Moss and Freed2003; Tholstrup, Reference Tholstrup2006) even if this product is rich in MFGM. Though, as previously noted, hard cheese is produced from unhomogenized milk. The protein and mineral composition may also play a role that should be elucidated. Regarding other health effects of MFGM proteins (Table 2 Michalski & Januel, Reference Michalski and Januel2006), Riccio (Reference Riccio2004) claims that these proteins could possibly be involved in autoimmune and neurological diseases, such as multiple sclerosis and autism. Indeed, butyrophilin was reported to induce inflammatory responses in the central nervous system when injected alone into animals. This author advises cautious removal of MFGM from dairy products. On the contrary, the thorough review by Spitsberg (Reference Spitsberg2005) highlights many health benefits of MFGM components including (1) anticancer effects via the absorbed peptides from the proteins fatty acid binding protein and BRCA1, the β-glucuronidase inhibitor and the phospholipids (mainly sphingomyelin), (2) anticholesterolaemic effects mainly via phospholipids, and (3) prevention of gastric diseases via sialylated glycoproteins. Spitsberg (Reference Spitsberg2005) suggests the use of MFGM as a potential nutraceutical, as also proposed regarding other bioactive peptides from milk (Séverin & Wenshui, Reference Séverin and Wenshui2005). The issue of MFGM components contributing to health and disease still appears controversial. During homogenization, a rearrangement of the MFGM components occurs and some fragments are released to the aqueous phase (Fig. 1), while in whole milk the MFGM is native at the globule surface. Due to its lipophilicity, butyrophilin should remain at the lipid interface. It would be important to investigate the effect of MFGM fragments on the beneficial or deleterious health effects mentioned earlier when dispersed in an aqueous phase or adsorbed at a lipid interface. Indeed, changes in the physico-chemical arrangement of bioactive molecules may affect their activity (either in a positive or negative way) and suggest different effects of unhomogenized and homogenized milk in this respect. Milk containing small native milk fat globules, in which the MFGM interface is doubled while kept in its native state (Michalski et al. Reference Michalski, Leconte, Briard, Fauquant, Maubois and Goudédranche2006b ), may also be useful to study the physiological impact of the MFGM quantity when consuming unhomogenized and homogenized milks.
Table 2 Major proteins of the bovine milk fat globule membrane: functions and health effects claimed in the literature (adapted from Michalski & Januel, Reference Michalski and Januel2006)

* (+), benefits; ( − ), harmful effects.
† Spitsberg (Reference Spitsberg2005).
‡ Riccio (Reference Riccio2004).
§ Kvistgaard et al. (Reference Kvistgaard, Pallesen, Arias, López, Petersen, Heegaard and Rasmussen2004).
Finally, the A1 variant of β-casein in cow's milk (and the B–β-casein that appears less frequently), but not the A2–β-casein, produces β-casomorphin 7 (Elliott et al. Reference Elliott, Harris, Hill, Bibby and Wasmuth1999; Jinsmaa & Yoshikawa, Reference Jinsmaa and Yoshikawa1999). β-Casomorphin 7 is a bioactive peptide, source of tyrosyl radical having thus the ability to catalyse the oxidation of LDL, which in turn have been widely implicated in the cardiovascular risk when taken up by the endothelium-bound macrophages (Allison & Clarke, Reference Allison and Clarke2006). β-Casomorphin 7 has a much longer half-life than other exomorphins and has a much higher activity than exomorphins encoded in human milk proteins (Allison & Clarke, Reference Allison and Clarke2006). Consequently, McLachlan (Reference McLachlan2001) hypothesized that the A1 variant of β-casein might be a risk of CHD due to correlations between A1–β-casein consumption and mortality from heart disease in 17 countries. However, in a review, Truswell (Reference Truswell2005) is very clear in his conclusion that there is no convincing or probable evidence that A1–β-casein is a factor causing CHD. Particularly, Truswell (Reference Truswell2005) argues that McLachlan (Reference McLachlan2001) used a too short a lag time of 5 years and that there could be counfounding factors such as the uncertainty of milk consumption evaluation and of socioeconomic or environmental differences. Truswell also stresses the unreliability of correlation studies of food intake and chronic disease. However, Woodford (Reference Woodford2006) argues that opponents of the A1 variant hypothesis cited in Truswell's review may have influenced conclusions due to their links with dairy companies. Allison & Clarke (Reference Allison and Clarke2006), who represent a corporation promoting breed selection producing A2 milk, also raise doubts. Based on immunological, pharmaceutical and clinical evidences, Woodford (Reference Woodford2006) thinks that the A1/A2–β-casein variant effect on CHD should be taken seriously. In a detailed reply to these criticisms, Truswell (Reference Truswell2006) reports a recent double-blind crossover human experiment where the same dose of A1 or A2 variant of β-casein was consumed for 12 weeks each way (Chin-Dusting et al. Reference Chin-Dusting, Shennan, Jones, Williams, Kingwell and Dart2006). No evidence was found from the study that supplementation with A1–β-casein had any cardiovascular health disadvantage over consumption of A2–β-casein (Chin-Dusting et al. Reference Chin-Dusting, Shennan, Jones, Williams, Kingwell and Dart2006). To date, no results are available about longer-term effects of this consumption, especially when initiated during infancy. Since homogenization has an effect on milk structure, particularly regarding casein distribution, the impact of milk processing on the distribution and bioactivity of different β-casein variants should be elucidated.
Results from recent observational studies regarding the association between dairy products and CHD mortality are conflicting. A weakness of observational studies is that they exclusively provide information about associations, not of causality. Furthermore, the estimation of milk intake can be flawed by neglecting milk as an ingredient in manufactured products (e.g. ice cream or milk chocolate) and there may be residual confounding as a result of unknown factors (Tholstrup, Reference Tholstrup2006). The variety of dairy products using different processes leading to various fat ultrastructures is also important. For example, consumption of cheeses manufactured with unhomogenized milk is high in France where coronary mortality is low, whereas in Scandinavian countries with high coronary mortality, homogenized milk consumption is high (Tholstrup, Reference Tholstrup2006). More human intervention studies are needed to elucidate the role of differently processed milks and their derived dairy products, together with the impact of formulation and interactions among ingredients, with respect to the risk of CHD.
Impact of homogenization on diabetes?
Type I diabetes
Type I diabetes is an auto-immune disease initially characterized by the infiltration of Langerhan's islets by macrophages and lymphocytes. Consequently, the insulin-producing cells (pancreatic β-cells) are selectively destroyed, causing an absolute and definitive insulin deficiency. The trigger of the auto-immune disruption process is reported to be controlled by genetic and environmental factors. Besides viral infections, dietary factors such as milk consumption have been discussed as being involved (Schrezenmeir & Jagla, Reference Schrezenmeir and Jagla2000). Kostraba et al. (Reference Kostraba, Cruiskshanks, Lawler-Heavner, Jobim, Rewers, Ga, Chase, Klingensmith and Hamman1993) stress that 50 % of type I diabetics consumed cow's milk before the age of 3 months. Sipetic et al. (Reference Sipetic, Vlajinac, Kocev, Bjekic and Sajic2005) found that the risk of type I diabetes is higher for children who were breast fed less than 4 months and who received cow's milk at younger than 5 months, and highlight the importance of interactions between these factors. Early exposure to cow's milk was not found to be associated with increased risk of type I diabetes development in low-risk subjects, but on the other hand, several epidemiological studies prompted Dahl-Jorgensen et al. (Reference Dahl-Jorgensen, Joner and Hanssen1991) and Fava et al. (Reference Fava, Leslie and Pozzilli1994) to hypothesize that cow's milk consumption is a risk factor in subjects genetically prone to diabetes. However, this hypothesis was not confirmed by the study of Meloni et al. (Reference Meloni, Marinaro, Mannazzu, Ogana, La Vecchia, Negri and Colombo1997). Karjalainen et al. (Reference Karjalainen, Martin, Knip, Robinson, Savilahti, Akerblom and Dosch1992) hypothesized that a particular region of bovine serum albumin (a 17-residue peptide called ABBOS) would be the reactive, trigger epitope for genetically prone subjects. According to Strand (Reference Strand1994), heating milk above 85°C would be sufficient to denature bovine serum albumin, more specifically its reactive epitope regarding type I diabetes. More recently, the implication of A1–β-casein in type I diabetes incidence has been suggested, particularly in 0–14-year-olds (Elliott et al. Reference Elliott, Harris, Hill, Bibby and Wasmuth1999; McLachlan, Reference McLachlan2001). As mentioned regarding CHD, Truswell (Reference Truswell2005) rules out the hypothesis of differing effects of A1 and A2 variants of β-casein on type I diabetes. However, his viewpoint is criticized by others (Allison & Clarke, Reference Allison and Clarke2006; Woodford, Reference Woodford2006), primarily because studies often fail to provide a true control devoid of diabetogenic effects. Also, type I diabetic patients present antibodies against A1-β–casein while their parents have antibodies against the A2 variant (Padberg et al. Reference Padberg, Schumm-Dräger, Petzoldt, Becker and Federlin1999; Allison & Clarke, Reference Allison and Clarke2006). The lower consumption of A1–β-casein at 2 years of age is suspected to explain the lower incidence of type I diabetes in Iceland than in Scandinavia (Birgisdottir et al. Reference Birgisdottir, Hill, Thorsson and Thorsdottir2006). However, whether there is a definite health benefit to milk containing the A2 variant requires further investigation (Hill & Boland, Reference Hill and Boland2001; Bell et al. Reference Bell, Grochoski and Clarke2006). In a review, Schrezenmeir & Jagla (Reference Schrezenmeir and Jagla2000) point out the need for intervention studies in man, and particularly, what impact consumption of cow's milk has beyond infancy. Studies usually deal with the consumption of commercial homogenized milk. Further studies appear to be necessary to definitely conclude whether cow's milk consumption is linked to the development of type I diabetes and to examine the impact of homogenization and other technological treatments of milk products.
Type II diabetes
Type II diabetes is part of the so-called metabolic syndrome which besides diabetes comprises abdominal obesity, hypertension, dyslipoproteinaemia, insulin resistance, prothrombotic and proinflammatory states (Sarti & Gallagher, Reference Sarti and Gallagher2006). Type II diabetes is characterized by the insulinoresistance of peripheral tissues associated with hyperinsulinaemia, followed by the secondary qualitative and quantitative deficiency of pancreatic insulin secretion due to glucose (Schrezenmeir & Jagla, Reference Schrezenmeir and Jagla2000). The early stage of type II diabetes is characterized by insulinoresistance of peripheral muscular and adipose tissues. Insulin resistance usually corresponds to the reduced hypoglycaemic effect at a usually efficient concentration. High SFA consumption is associated with glucose intolerance or insulin resistance and type II diabetes. Milk contains 60–70 g SFA/100 g total fatty acids. On the contrary, unsaturated fatty acids (30–35 g/100 g total milk fatty acids) are inversely associated to diabetes risk (Mann, Reference Mann2002), and milk also contains beneficial minor components such as conjugated linoleic acid (Schrezenmeir & Jagla, Reference Schrezenmeir and Jagla2000). Epidemiological data concerning a relationship between milk consumption and type II diabetes are rare (Schrezenmeir & Jagla, Reference Schrezenmeir and Jagla2000). Moreover, milk processing parameters are not controlled. Lawlor et al. (Reference Lawlor, Ebrahim, Timpson and Davey Smith2005) point out that randomized controlled trials are required to establish whether milk avoidance is causally associated with the lower occurrence of type II diabetes that they observed in non-milk consumers. It is known that the various heat treatments and storage favour the formation of glycation products (also called non-enzymatic glycosylation or Maillard reaction, often involving lysine; Morgan et al. Reference Morgan, Leonil, Molle and Bouhallab1999). There is evidence that glycation products formed in the human body may be linked with complications of diabetes; an effect of food-derived glycated molecules should be investigated further. Moreover, glycation in food products impairs proteolysis, which makes digestion more difficult (Evangelisti et al. Reference Evangelisti, Calcagno, Nardi and Zunin1999). The latter study was conducted with commercial milks subjected to various heat treatments and very likely homogenization. However, homogenization parameters are not specified and the authors do not take it into account in their discussion. Many parameters affect glycation and particularly the availability of amine groups within protein structure. Since homogenization dramatically alters the organization of proteins in milk, it would be interesting to compare the potential glycation sites of proteins from homogenized or unhomogenized milk, and to distinguish between the impact of homogenization and heat treatments.
Impact of homogenization on milk intolerance and allergy?
Milk intolerance
Milk allergy and lactose intolerance should not be confused. Dietary intolerance (e.g. to lactose) is a physiological reaction following ingestion of a food product or dietary additive. This is normally due to a deficiency in some digestive enzymes, thus impairing the complete digestion of the implied food. In this respect, lactose intolerance results from a lack of lactase, the enzyme necessary for its proper digestion. Other rare causes can explain lactose intolerance including abnormal permeability of the stomach wall, galactose transportation or galactose metabolism (Dahlqvist, Reference Dahlqvist and Delmont1983). Milk fat content was not found to affect the symptoms of lactose intolerance (Vesa et al. Reference Vesa, Korpela and Korpela1997). Delayed gastric emptying has been proposed as one possible explanation for improved lactose tolerance after ingestion of milk with a meal instead of milk on its own (Vesa et al. Reference Vesa, Marteau and Korpela2000). Therefore, it would be possible that homogenization could present a beneficial effect in this respect for dairy creams since it delays gastric emptying (Armand et al. Reference Armand, Pasquier, André, Borel, Senft, Peyrot, Salducci, Portugal, Jaussan and Lairon1999; Michalski et al. Reference Michalski, Briard, Desage and Géloën2005b , Reference Michalski, Soares, Lopez, Leconte, Briard and Géloën2006a ), while it could accelerate gastric emptying for milk (Meisel & Hagemeister, Reference Meisel and Hagemeister1984). However, results linking gastric emptying with lactose intolerance are not straightforward and no clearcut conclusion can be drawn (Korhonen & Korpela, Reference Korhonen and Korpela1994; Vesa et al. Reference Vesa, Marteau and Korpela2000). Paajanen et al. (Reference Paajanen, Tuure, Poussa and Korpela2003) studied adults tolerant to lactose, with a subjective tolerance to unhomogenized milk but describing subjective intolerance to homogenized milk. However, their study revealed no differences in symptoms between consumption of homogenized and unhomogenized milk. A following study dealt with adults intolerant to lactose (Korpela et al. Reference Korpela, Paajanen and Tuure2005). No significant difference is observed in the symptomatic response between unprocessed organic milk and processed milk. Even though some subjects subjectively experience a better tolerance of unhomogenized than homogenized milk, this is not the case in lactose-intolerant subjects in general (Korpela et al. Reference Korpela, Paajanen and Tuure2005). Moreover, Paajanen et al. (Reference Paajanen, Korpela, Tuure, Honkanen, Järvelä, Ilonen, Knip, Vaarala and Kokkonen2005a ) report that food-related gastrointestinal symptoms in a cohort of young adults were caused by unspecific and unknown traits of altered mucosal immune response rather than by cow milk intolerance, as is often subjectively suspected by the patient.
Milk allergy
Cow's milk protein allergy is a reaction linked to the immune system. The incidence of allergy in early childhood varies between 2 and 3 % depending on the countries (Host, Reference Host2002). Within the first year, 56 % of initial allergy occurrences among children are resolved, 87 % of initial allergy occurrences are found resolved at the age of 3 (i.e. 71 % of the remaining occurrences after 1 year of age) and 97 % of initial allergy occurrences are found resolved at 15 years of age (i.e. 80 % of the remaining occurrences after 3 years of age) (Host et al. Reference Host, Halken, Jacobsen, Christensen, Herskind and Plesner2002). Cow's milk protein allergies are abnormal reactions of the immune system to the proteins contained in cow's milk. The major allergizing proteins are reported to be β-Lg, α-lactalbumin and caseins. They cause anaphylactic reactions (group of immune phenomena induced by a type-1 hypersensitivity reaction to the IgE mediation). The symptoms affect digestion, skin and the respiratory system. Decreasing levels of milk-specific IgE might signify allergy resolution. Recent studies also emphasize the role of the regulative cytokine IL-10 in milk tolerance and of defective T cell-mediated dendritic cell apoptosis in cow's milk allergy (Sicherer & Leung, Reference Sicherer and Leung2005). Paajanen (Reference Paajanen2005) reports that delayed-type cow's milk protein allergy could be a local intestinal immune-activation state showing activation of both Th1 and Th2 lymphocytes. Moreover, Paajanen suggests that an autoimmune state cannot be ruled out, at least in a subgroup of the affected subjects. We must point out that an impact of sensitization to IgE during foetal life depending on the mother's diet cannot be ruled out.
In animal models, it seems that homogenization favours hypersensitivity. Homogenization and pasteurization are reported to enhance the humoral immune response of rats charged intraperitoneally with milk (Feng & Collins, Reference Feng and Collins1999). Homogenized milk orally fed to hypersensitive mice is reported to induce an anaphylactic shock (Poulsen & Hau, Reference Poulsen and Hau1987), increase milk-specific IgE production (Nielsen et al. Reference Nielsen, Poulsen and Hau1989), increase the mass of intestinal segment and induce mastocyte degranulation (cells whose cytoplasm contains many basophilic granulations, rich in mediators such as heparin, serotonin, histamine; Poulsen et al. Reference Poulsen, Nielsen, Basse and Hau1990). Moreover, the allergenicity of homogenized milk in mice is reported to increase with increasing fat content (Poulsen et al. Reference Poulsen, Hau and Kollerup1987). On the other hand, unhomogenized cow's milk induces few or no such symptoms and immune responses. However, when milk is given intravenously (Poulsen & Hau, Reference Poulsen and Hau1987) or subcutaneously (Poulsen et al. Reference Poulsen, Nielsen, Basse and Hau1990), the same reactions are observed regardless of milk treatment.
In North European countries, many consumers state that they tolerate untreated cow's milk and pasteurized non-homogenized milk, but not homogenized milk. The parents of allergic children relate the same phenomenon. One explanation may be that during homogenization, the organization of allergenic proteins is changed. The milk fat presents a dramatically increased surface onto which allergenic milk proteins can adsorb. In untreated milk, many of the antigenic proteins are located inside casein micelles. In homogenized milk, the amount of exposed antigenic proteins is reported to increase (Poulsen & Hau, Reference Poulsen and Hau1987). Furthermore, there is also some release of MFGM proteins (listed in Table 2) in the aqueous phase (the latter were suggested to be potential allergens, though with no clearcut evidence, by Riccio, Reference Riccio2004). However, the amount and exposure of allergenic proteins in untreated milk appear to be sufficient to induce allergic reactions in some subjects. Moreover, it has not been proven that homogenization induces hypersensitivity in man. Clinical studies revealed no difference between homogenized and unhomogenized milk in either children allergic to milk (Host & Samuelsson, Reference Host and Samuelsson1988) or in adults intolerant to lactose or hypersensitive to milk (Pelto et al. Reference Pelto, Rantakokko, Lilius, Nuutila and Salminen2000). In one study, homogenized pasteurized milk was found to be less suitable than unhomogenized milk in 10 % of children with milk protein allergy (Hansen et al. Reference Hansen, Host and Osterballe1987). But few studies concern subjects with normal milk tolerance. Paajanen et al. (Reference Paajanen, Tuure, Vaarala and Korpela2005b ) recently found no difference in the immunological responses to homogenized and unhomogenized milk in healthy adults with a good tolerance of milk, according to their production of specific antibodies against casein, β-Lg and bovine insulin during 1 month for each type of milk. Accordingly, a recent review points out that homogenization does not change the allergenic potency of cow's milk (Paschke & Besler, Reference Paschke and Besler2002). However, Paajanen et al. (Reference Paajanen, Tuure, Vaarala and Korpela2005b ) highlight that their results with milk-tolerant adults do not exclude the possibility that homogenized and unhomogenized milk could induce different types of primary immunization to cow's milk antigens in immunologically intact individuals, i.e. in infants. Updated data provided by Wal (Reference Wal2004) show that most milk proteins, even minor proteins, are potential allergens and that no single allergen or particular structure can account for a major part of milk allergenicity. This can explain why the effect of homogenization may be difficult to observe, since individuals can be sensitive to various epitopes, and since some human groups can be more sensitive than others. Wal (Reference Wal2004) also highlights that the available evidence is not sufficient to reliably predict the effect of food processing on the allergenic potential of milk proteins.
As pointed out by Sanchez & Frémont (Reference Sanchez and Frémont2003), the number of publications on food allergy has recently increased dramatically. Food processing and interactions between constituents and additives are strongly suspected to be responsible for at least part of the increased occurrence of food allergies. Heat processing induces, in most cases, irreversible denaturation of proteins leading to aggregation. Such structural changes do not always correlate with decreased allergenicity. Depending on the system, heating may have no effect or it may decrease or increase allergenicity (Sanchez & Frémont, Reference Sanchez and Frémont2003). The existence of sequential and conformational epitopes, the demasking of new epitopes or the modification of epitopes can explain some of the results reported in the literature. Even in the absence of heating, interactions between proteins and other components of food can cause conformational changes in allergens, thereby affecting their thermal stability. In such an event, increased or decreased thermal aggregation of affected proteins can occur. The effect of such interactions on the allergenicity of proteins is currently unknown. In this respect, the fourth task of the European Research Program Protall concerns the impact of food processing (e.g. heating) and digestion on their allergenicity (Sanchez & Frémont, Reference Sanchez and Frémont2003). A review by Besler et al. (Reference Besler, Paschke and Paschke2001) also shows the influence of food processing on the allergenicity (capacity to bind IgE) of proteins. Drastic heating such as boiling skim milk for 10 min is reported to reduce greatly the allergenicity of caseins and whey proteins. On the contrary, the most important consequence of heating at common milk-processing temperatures seems to be the increased immunoreactivity of some milk allergens. Allergenicity increase by heating can be due to epitope exposure after conformational changes or to changes in amino acids due to Maillard reactions with sugars (Sanchez & Frémont, Reference Sanchez and Frémont2003). The latter reactions are known to occur in milk upon heating. Protein–fatty acid interactions can also change the secondary structure of β-Lg upon heating or even at room temperature with oleic acid (Ikeda et al. Reference Ikeda, Fögeding and Hardin2000). Since some β-Lg can be present at the lipid interface in homogenized milk, such interactions can occur and their effect on protein allergenicity needs to be investigated, regardless of heat treatments.
Conclusion and prospects for future research
Homogenization changes the organization of milk components by decreasing the size of milk fat globules, on which caseins become the main protein fraction adsorbed instead of the native MFGM. However, the present review shows that there is still no clearcut evidence about the impact of these structural changes on some health properties of milk such as CVD, diabetes and allergy. The effects of milk homogenization and heating regarding the bioactivity of casein peptides and MFGM proteins and lipids, and the cardiovascular impact of milk consumption, remain to be elucidated. Further studies should aim to dissociate heat treatment from homogenization effects. It also appears necessary to compare the digestion and health effects of milk products containing fat droplets with different size and interface composition (native MFGM or fragments thereof, native micellar casein, sodium caseinate, whey protein isolate, phospholipids etc.).
More generally, milk contains bioactive proteins, polypeptides, peptides, lipids (cholesterol, phospholipids such as sphingomyelin, conjugated linoleic acid, gangliosides and cerebrosides), oligosaccharides, hormones and growth factors, as well as additives (vitamin D, n-3 fatty acids in some brands), bacteria, and possible traces of endotoxins, antibiotics, detergents/disinfectants and pesticides/insecticides; however, their possible activation, alteration or inactivation due to dairy processes are not well known. In this respect, the effect of new alternative processes for milk preservation should be studied regarding their putative impact on milk-related health properties. The use of high hydrostatic pressure treatment is presented as an advantageous alternative to the usual heat treatments (Langley-Danysz, Reference Langley-Danysz1993; Trujillo et al. Reference Trujillo, Capellas, Salso, Gervilla and Guamis2002). Another widely used method in Canada and Scandinavia is removal of bacteria from skimmed milk using microfiltration (Pafylias et al. Reference Pafylias, Cheryan and Mehaia1996; Lindquist, Reference Lindquist1998; Saboya & Maubois, Reference Saboya and Maubois2000; Sachdeva et al. Reference Sachdeva, Jain, Ram and Agarwal2001). The fat source in microfiltered milk is still homogenized cream because using unhomogenized cream would rapidly lead to a cream layer disliked by most consumers. The impact of these technologies on milk health value needs to be further explored.
As suggested by Walzem et al. (Reference Walzem, Dillard and German2002), food and nutrition research should move beyond the description of food ingredients as delivering only essential nutrients and develop a mechanistic understanding of the interactions between dietary components and the metabolic and physiological properties of the gastrointestinal tract. In this respect, we feel that studies dealing with the health properties of milk and dairy products should take their physico-chemical properties and the ultrastructure of milk fat into account as relevant parameters, since they affect digestion metabolism. It is thus of the utmost importance in clinical studies that the technological treatments applied to the food products be described. This research would benefit from interdisciplinary studies with the aim of completing nutritional knowledge, further optimizing dairy processes (especially in the field of infant nutrition) and even developing adapted products for patients suffering from gastrointestinal disease, allergy or metabolic syndrome. In the current context of obesity and allergy outbreaks, studies on the impact of processing parameters (homogenization, heating, whipping, cheesemaking etc.) and physico-chemical structure (fat ultrastructure, interface and protein matrix composition etc.) on the nutritional and health value of milk and dairy products would help to design products meeting three major objectives: shelf-life, taste and health value.
Acknowledgements
The author thanks C. Januel and M. H. Famelart for their contribution in the literature search and Arilait Recherches for funding part of the work. J. L. Maubois and H. Vidal are acknowledged for their critical comments on the manuscript and M. Skilton for editing the English language. We apologize to some authors who were cited indirectly through reviews.