Introduction
The most frequent nematodes identified in donkeys are members of the Strongylidae family, the subfamily Cyathostominae and, to a lesser extent, the subfamily Strongylinae (Matthee et al., Reference Matthee, Krecek and Milne2000; Matthews & Burden, Reference Matthews and Burden2013). Donkey intestinal strongyles are known to lower the host's fertility, body condition score and cause milk production losses (Matthews & Burden, Reference Matthews and Burden2013). Anthelmintic compounds that have proven to be effective for the treatment of cyathostomins are moxidectin, ivermectin and other macrocyclic lactones (Sanna et al., Reference Sanna, Pipia, Tamponi, Manca, Varcasia, Traversa and Scala2016). However, a lack of licensed anthelmintic drugs for donkeys, together with the off-label use of drugs intended for horses and ruminants, inadequate dosage and too frequent administration of anthelmintics, are considered to cause a rapid increase in anthelmintic resistance (Elsheikha, Reference De Falco, Mancini, Roscigno, Mignola, Taglialatela-Scafati and Senatore2019). Until now, resistance in donkeys has been reported for moxidectin, pyrantel, fenbendazole and ivermectin (Trawford et al., Reference Thienpont, Rochette and Vanparijs2005; Lawson et al., Reference Madhusudan, Ramachandra, Udaykumar, Sharnagouda, Nagraj and Jagjivan2015; Buono et al., Reference Buono, Roncoroni, Pacifico, Piantedosi, Neola, Barile, Fagiolo, Várady and Veneziano2018; Hamed et al., Reference Hamed, El-Allawy and Hassnein2019). As there are no alternatives available on the market, anthelmintic resistance represents a threat not only to the animal's health and well-being, but also to the quantity and quality of milk production and industries relying on it.
Nowadays, an increasing interest in donkey milk can be observed in the countries of Western Europe and Italy, due to its multiple usages, such as human consumption and cosmetics (Camillo et al., Reference Camillo, Rota, Biagini, Tesi, Fanelli and Panzani2018; FAO, 2020). Considered highly similar to human milk, it is given to infants, and because it has a protein composition different from cow's milk, it can also be consumed by people with lactose intolerance (Madhusudan et al., Reference Lawson, Burden and Elsheikha2017). Therefore, it is important to limit the anthelmintic drug residues, assuring the safety and quality of donkey milk.
The rapid development of anthelmintic resistance, together with the need for new safe alternatives, has caused an increased interest in the therapeutic potential of medicinal plants. To date, only a limited number of studies have been conducted worldwide to test the anthelmintic activity of plants used in ethnoveterinary practices or their bioactive compounds against gastrointestinal (GI) parasites of horses and donkeys. Condensed tannins (CTs) isolated from sainfoin have been reported to exhibit good in vitro anthelmintic activity, reducing horse strongyle egg hatching by 82%, while in vivo tests on donkeys’ and horses’ CTs showed little to no anthelmintic effects (Couto et al., Reference Couto, Santos, Laborda, Nóvoa, Ferreira and Madeira de Carvalho2016; Collas et al., Reference Coté, Boucher, Pichette and Legault2018). Maestrini et al. (Reference Maestrini, Tava, Mancini, Salari and Perrucci2019) reported that saponins and prosapogenins possess strong ovicidal activity against donkey GI nematodes, showing >80% inhibition of egg hatching. Crude aqueous extract of Trachyspermum ammi has shown good in vivo anthelmintic activity against donkey GI nematodes, the faecal egg count reduction being 71.2% (Imani-Baran et al., Reference Imani-Baran, Abdollahi, Akbari, Jafarirad and Moharramnejad2020). However, a herbal dewormer commercially available in Italy, which contains Mallotus philippinensis, Carduus marianus, Urtica urens, Genziana lutea and Eucalyptus globulus extracts, failed to exhibit any anthelmintic effect in vivo in donkeys – proof that in vitro and in vivo studies of the medicinal plants before their administration are required (Papini et al., Reference Papini, Orsetti and Sgorbini2020).
The present study was conducted to evaluate the in vitro anthelmintic activity of six plants commonly used in traditional Romanian medicine for the treatment of parasitic diseases in both humans and domestic animals: Achillea millefolium, Artemisia absinthium, Centaurium erythraea, Gentiana asclepiadea, Inula helenium and Tanacetum vulgare, using an egg-hatching assay (EHA) and larval development assay (LDA). This study is part of ongoing work on anthelmintic resistance detection in equid species and the search for plant-based treatment alternatives (Cernea et al., Reference Cernea, Cristina, Stefanut, Madeira de Carvalho, Taulescu and Cozma2015).
Materials and methods
Plant materials
The species of plants included in this study were selected based on the composition of anthelmintic tea mixtures available on the Romanian market with respect to their claimed ethno-therapeutic use, according to the available literature in this domain. Plant materials were purchased from a local licensed herbal store that had collected the plants from Prahova County (45.1°N, 26.02°E), Bistrița-Năsăud County (47°10′N, 24°30′E) and Botoșani County (47°50′N, 26°49′E), Romania in July 2019. The details and data in terms of the plants scientific and empirical names, family, the plant parts used, their main active compounds and biological activity are summarized in table 1.
Table 1. List of plants used for aqueous extraction with details of plant species, family, plant parts used, main active compounds and biological activity.
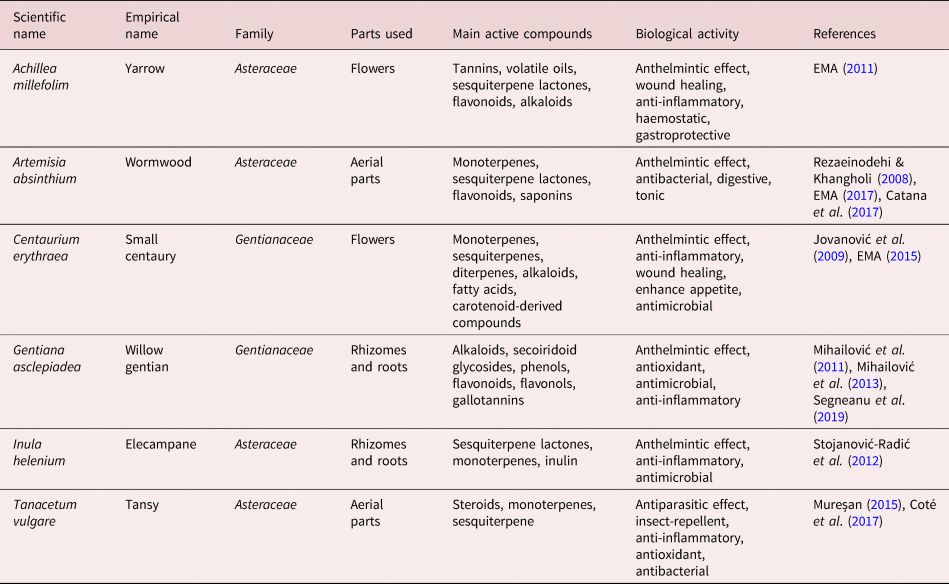
Preparation of aqueous extracts
The aqueous plant extracts were prepared by adding 12.5 g of powdered plant material to 50 ml of distilled water heated to 100°C, mixing and then boiling them for 30 min in a water bath at a temperature of 100°C. The resulting extracts were filtered through an extra-fine sieve, followed by filtration through Whatman No. 1 filter paper, and stored at 4°C for further use.
Egg recovery and preparation
Seventeen dairy jennies from a family farm located in Cluj County, Romania were selected for the study. The selected donkeys had never received anthelmintic treatments, and, therefore, were considered naturally infected with strongyles. Faecal samples were collected directly from the rectum using sterilized gloves and collecting bags. Faecal samples were thoroughly mixed with warm distilled water (37°C) to obtain the first egg suspension, which was filtered through a sieve battery and comprised the following sizes: 250, 125 and 25 μm. According to their size, as stated by Thienpont et al. (Reference Trawford, Burden and Hodgkinson1986), strongyle eggs were retained on the last sieve used (25 μm), from where they were washed and collected under pressure, using distilled water. The final egg suspension was obtained by centrifugation of the egg/water mixture at 4000 RPM for 7 min, followed by supernatant removal. In the end, the average number of eggs/100 μl, determined by the McMaster counting method (Thienpont et al., Reference Trawford, Burden and Hodgkinson1986), was 300.
Larval identification
For the faecal culture preparation, individual samples of collected faeces were mixed with a small amount of distilled water and placed into plastic cups. The cups were covered with perforated aluminium foil and incubated for 14 days at 27°C. Every two days, a small amount of water was added to the faecal culture in order to maintain a relative humidity of approximately 60–80%. After 14 days, the third-stage larvae (L3) were collected, obtaining a mixed sample that was examined using optical microscopy (200×, 400×). The morphological identification of 200 L3, followed by their classification into Cyathostominae and Strongylinae subfamilies, was performed based on the length of the intestine, number, shape and pattern of intestinal cells (Cernea et al., Reference Cernea, Madeira de Carvalho, Cozma, Cernea, Răileanu, Silberg and Gut2008; Santos et al., Reference Santos, Madeira de Carvalho and Molento2018).
EHA
The EHA was carried out using the method described previously by Coles et al. (Reference Coles, Jackson, Pomroy, Prichard, von Samson-Himmelstjerna, Silvestre, Taylor and Vercruysse2006), with some modifications: 800 μl of distilled water, 200 μl of egg suspension and 1000 μl of aqueous plant extract were added to each testing well of 24-well cell culture plates (Eppendorf, Nitech, Cluj-Napoca, Romania). The final dilutions of aqueous plant extracts in the testing wells were 125.0, 62.5, 31.25, 15.63, 7.81, 3.91, 1.95, 0.98, 0.49 and 0.24 mg/ml. Five replicates were tested for each concentration, together with a total of 12 negative controls. In the control solution, the plant extract was replaced by 1000 μl of distilled water. The estimated number of eggs contained in 100 μl was 300. Plates were incubated at 27°C for 48 h. The number of eggs and first-stage larvae (L1) were counted using an optical microscope (Motic BA310, Nitech, Cluj-Napoca, Romania) at 40× and 100×. The percentage of hatched eggs in each well was calculated using the following formula:

LDA
The LDA was performed in order to test the potential larvicidal effects of the aqueous plant extracts. As with the EHA, LDA was performed using ten serial dilutions, with concentrations ranging from 125.0 to 0.24 mg/ml, having five replicates for each dilution and a total of 12 wells with negative controls. For 48 h, the mix of 800 μl of distilled water and 200 μl of egg suspension was incubated at 27°C. Afterwards, 1000 μl of plant extract were added to each well of 24-well cell culture plates (Eppendorf) and the incubation continued for another 14 days (Craven et al., Reference Craven, Bjørn, Barnes, Henriksen and Nansen1999; Tandon & Kaplan, Reference Tandon and Kaplan2004). In the negative control, the same protocol was applied, except the plant extracts were replaced by distilled water. The larval development percentage was calculated using the following formula:

Statistics
The mean reduction (%) of egg hatch and larval development compared to the negative controls (distilled water) was calculated for each plant extract and for each dilution. All data were analysed by repeated measures analysis of variance, using the GraphPad InStat 3.10 software, http://graphpad-instat.win7dwnld.com/download-new-version/. Differences, with the indication of P-value, were determined using Tukey's multiple comparison test. Probit regression analysis was performed to calculate the median lethal concentration (LC-50) with respective 95% confidence intervals (95% CIs) for each plant extract. If the data did not accurately fit the probit model (bad fit), the LC-50 value was not calculated (Hintze, Reference Hintze and Hintze2007; Peachey et al., Reference Peachey, Pinchbeck, Matthews, Burden, Mulugeta, Scantlebury and Hodgkinson2015).
Results
Larval identification
Cyathostomin species represented 74% of the total larvae number. Cyathostomum sensu latum type A (27%), type C (20%) and Trichostrongilus axei (10%) were the most frequent, followed by cyathostomins type D (6%), type B (5%), type G (2%), Gyalocephalus capitatus (2%), cyathostomins type E (1%) and type F (1%). Five species (26% of all species recovered) belonged to the Strongylinae subfamily: Strongylus vulgaris (8%), Oesophagodontus robustus (8%), Craterostomum acuticaudatum (7%), Trichodontophorus serratus (2%) and Strongylus edentatus (1%).
EHA
The EHA results of the six aqueous plant extracts are presented in table 2. From all of the tested plants, five have shown a strong ovicidal activity at concentrations ranging between 125 and 1.95 mg/ml, as compared to C. erythraea, which exhibited a low and non-significant egg-hatching inhibition. The aqueous extract of I. helenium showed the most significant effects (P < 0.01), inhibiting egg hatching at concentrations between 125 and 0.976 mg/ml, and the lowest LC-50 value of 0.041 mg/ml (95% CI 0.01–0.16), with a good fit in the probit model. Artemisia absinthium exhibited a strong inhibitory egg-hatching effect (P < 0.01) at concentrations between 125 and 3.9 mg/ml, with an LC-50 value of 0.486 mg/ml (95% CI 0.21–1.09). Achillea millefolium and T. vulgare were effective at concentrations ranging between 125 and 1.95 mg/ml, followed by G. asclepiadea extract, in concentrations between 7.81 and 1.95 mg/ml. Centaurium erythraea showed low ovicidal effect and did not exhibit a concentration-dependent pattern.
Table 2. In vitro ovicidal activity* (% reduction, mean ± standard deviation, n = 5) and LC-50 of aqueous extracts (ten different concentrations) of six medicinal plants against Strongylus spp.
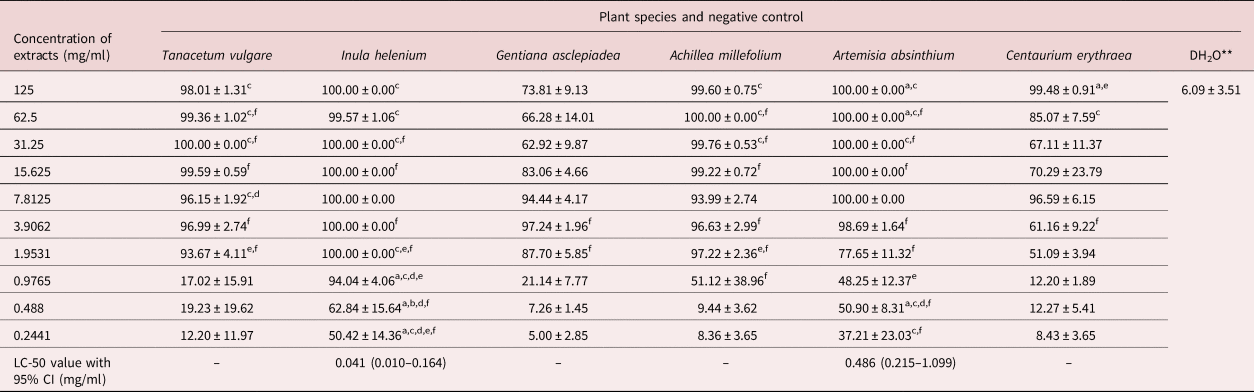
* Data are presented as mean of five independent assays.
** % reductions for all concentrations were statistically significant compared to the control group (P ≤ 0.01).
a–f Means with different subscript letters within a column are significantly different at P < 0.01.
LC-50, median lethal concentration; 95% CI, 95% confidence interval; DH2O, distilled water.
LDA
As previously observed in EHA, plant extracts manifested their highest larvicidal activity at concentrations between 125 and 1.95 mg/ml (table 3). Gentiana asclepiadea and I. helenium aqueous extracts showed the most potent inhibitory effects in terms of larval development inhibition at concentrations between 125 and 0.976 mg/ml, having LD-50 values of 0.36 (95% CI 0.19–0.72) and 0.41 (95% CI 0.27–0.62) mg/ml, respectively. The extracts of A. millefolium and T. vulgare exhibited strong larvicidal effect at concentrations ranging between 125 and 3.9 mg/ml, followed by A. absinthium (125-3.9 mg/ml). The weakest anthelmintic activity against L3 nematodes was exhibited by C. erythraea.
Table 3. In vitro larvicidal activity* (% reduction, mean ± standard deviation, n = 5) and LC-50 of aqueous extracts (ten different concentrations) of six medicinal plants against Strongylus spp.

* Data are presented as mean of five independent assays.
** % reductions for all concentrations were statistically significant compared to the control group (P ≤ 0.01).
a–f Means with different subscript letters within a column are significantly different at P < 0.01.
LC-50, median lethal concentration; 95% CI, 95% confidence interval; DH2O, distilled water.
Discussion
Over the past two decades, a rising awareness of the potential health hazards of anthelmintic drug residues in food and the growing prevalence of anthelmintic resistance in domestic animals has led to an increased interest in medicinal plants and their secondary metabolites. The most commonly used and affordable in vitro assays for plant extracts anthelmintic activity assessment are EHA and LDA.
The objective of our study was to determine in vitro anthelmintic efficacy of six aqueous medicinal plant extracts against donkey strongyles, considering the fact that these plants are commonly found on pastures and, therefore, can be easily introduced in animal feed. In the results, five out of six tested plant extracts exhibited strong in vitro anthelmintic activity, the most effective being I. helenium. Two of the major bioactive compounds of I. helenium are sesquiterpene lactones and inulin (Petkova et al., Reference Petkova, Ognyanov, Todorova and Denev2015). Sesquiterpenes are known for their anthelmintic properties against GI nematodes like Haemonchus contortus and Ascaris suum (Foster et al., Reference Foster, Cassida and Turner2011; Williams et al., Reference Williams, Pena-Espinoza, Hansen, Boas and Thamsborg2016). Inulin also causes in vivo changes of parasite communities, but its anthelmintic mechanism of action cannot be fully explained (Jensen et al., Reference Jensen, Mejer and Mølbak2011; Peña-Espinoza et al., Reference Peña-Espinoza, Valente, Thasborg, Simonsen, Boas, Enemark, López-Muñoz and Williams2018). In our study, the aqueous extract of I. helenium showed strong ovicidal and larvicidal effects, with a maximum egg-hatching-reduction percentage of 100 (±0), and an LC-50 value of 0.041 mg/ml (95% CI 0.010–0.164). The maximum L3 reduction percentage was 100 (±0), with an LC-50 value of 0.408 (95% CI 0.271–0.615), in contrast to the research of Urban et al. (Reference Urban, Kokoska, Langrova and Matejkova2008), where the ethanol extract of I. helenium showed a strong larvicidal effect, but a moderate ovicidal effect. This can be explained by the differences between the concentrations, methods of extraction and secondary metabolite concentrations used (Ong, Reference Ong2004; De Falco et al., Reference Elsheikha2013; Russo et al., Reference Russo, Formisano, Rigano, Senatore, Delfine, Cardile, Rosselli and Bruno2013). Therefore, we believe that further research on the anthelmintic properties of I. helenium is needed.
Another tested aqueous extract was A. absinthium, a medicinal plant widely known and used in ethno-medicine for its anthelmintic potential. The aqueous extract of A. absinthium (wormwood) exhibited a strong ovicidal effect, with a maximum egg-hatching-reduction percentage of 100 (±0), an LC-50 value of 0.486 mg/ml (95% CI 0.21–1.09) and, to a lesser extent, a larvicidal effect. There have been studies demonstrating the in vitro anthelmintic (ovicidal and larvicidal) effect of both methanolic and aqueous extracts of A. absinthium on H. contortus, A. suum and horse strongyles (Urban et al., Reference Urban, Kokoska, Langrova and Matejkova2008; Peachey et al., Reference Peachey, Pinchbeck, Matthews, Burden, Mulugeta, Scantlebury and Hodgkinson2015; Váradyová et al., Reference Váradyová, Pisarčíková, Babják, Hodges, Mravčáková, Kišidayová, Königová, Vadlejch and Várady2018). Gentiana asclepiadea (willow gentian) had a statistically significant inhibitory effect on the development of L3, with the lowest LC-50 values of 0.36 (95% CI 0.19–0.72), and a moderate ovicidal effect. Willow gentian has been traditionally used against GI parasitic infections in humans (Oroian et al., Reference Oroian, Sămărghiţan, Coşarcă, Hiriţiu, Oroian and Tanase2019) and it is now available on the Romanian market in anthelmintic tea mixtures. However, there is no scientific evidence to support its anthelmintic properties, and further studies are necessary. The differences between the EHA and LDA results of both A. absinthium and G. asclepiadea led us to the conclusion that some plants may exhibit strong ovicidal effects and others strong larvicidal effects. Effective combinations that provide strong anthelmintic activity, both ovicidal and larvicidal, can only be achieved along with the understanding of the plants’ secondary metabolite action mechanisms.
Both A. millefolium and T. vulgare have shown good ovicidal and larvicidal effects, with similar results for A. millefolium being reported in other in vitro and in vivo studies of the GI nematodes of sheep and horses (Izadi et al., Reference Izadi, Sharif, Khalilian, Azadbakht and Zayaei2003; Tariq et al., Reference Tariq, Chishti, Ahmad and Shawl2008).
The importance of C. erythraea has been mentioned in the pharmacopoeias of different countries, being believed to possess a large variety of beneficial health effects, including antiparasitic effects (Tahraoui et al., Reference Tahraoui, Israili and Lyoussi2010). However, in our study, the aqueous extract of C. erythraea did not show a significant anthelmintic effect at any tested concentration, and we did not find any other studies regarding its anthelmintic activity.
Conclusion
The present study assessed the in vitro anthelmintic activity of six aqueous medicinal plants extracts, from the Romanian spontaneous flora already recommended as anthelmintics, against strongyle eggs and larvae isolated from donkeys. Based on the results, it can be concluded that the aqueous extract of I. helenium, followed decreasingly by A. absinthium, G. asclepiadea, T. vulgare and A. millefolium, possess significant anthelmintic effects against donkey GI nematodes, justifying their ethno-medicinal use. Additionally, we can conclude that some of the extracts may have higher ovicidal or larvicidal activity and do not necessarily exhibit both effects, except I. helenium, which showed significant ovicidal and larvicidal activity. However, further in vitro and in vivo studies are recommended to determine the bioactive metabolites responsible for their anthelmintic activity, their ovicidal and larvicidal action mechanisms, potential toxicity and therapeutic value.
Financial support
This project was funded by the Romanian Ministry of Research and Innovation through Program 1 – Development of the National Research and Development System, Subprogram 1.2 – Institutional Performance – Projects for Financing the Excellence in CDI (grant number 37PFE/06.11.2018). Project title: ‘Increasing the institutional performance through consolidation and development of research directions within the USAMVCN’.
Conflicts of interest
None.