Introduction
Mood disorders refer to a spectrum of conditions, including major depressive unipolar and bipolar disorders, which are amongst the most prevalent and severe psychiatric diseases with a tendency to be recurrent, chronic, and disabling.Reference Wittchen 1 , 2 These disorders constitute major public health concerns and leading conditions in the global burden of disease in terms of disability, morbidity, and premature mortality conferring high-suicidality risk. 1–4 Although mood disorders are very common mental disorders, their etiology is still unclear.Reference Malhi and Mann 5 However, in this context of major public health concern, the understanding of the mechanism involved in their development and maintenance should thus be considered as a priority to identify potential early markers that could help in improving diagnoses and treatments. Among promising markers, the sleep dimension is of increasing attention both for the study, diagnosis, and treatment of recurrent affective illness.Reference Geoffroy and Gottlieb 6 Indeed, sleep and circadian rhythm alterations are core symptoms present in almost all patients throughout the disorder,Reference Geoffroy 7 and are both causes and consequences of mood episodes with complex- and multi-directional relationships.Reference Geoffroy 7 Insomnia is a clinically significant feature of mood disorders and it is highly prevalent across their course, as many as 80% to 100% of people during the depressive episode and 45% to 55% during the bipolar inter-episode period experience insomnia. 6–13 Insomnia can be related to illness severity, cognitive dysfunctions, levels of hopelessness, increased risk of substance abuse, aggressive and impulsive behaviors, emotional dysregulation, and increased risk for suicidality. 6–17 In particular, it has been also demonstrated that insomnia complaints predict suicidal attempt, independently of all psychopathologies and sociodemographic characteristics.Reference Geoffroy, Oquendo and Courtet 18 In addition, it plays an important role in relapses and recurrences, it is considered an independent risk factor, as well as an early sign occurring prior to both depressive and manic episodes. 8–12 , 19–22 Recently, it has been shown that targeting sleep alterations such as insomnia may improve not only insomnia, but it may also favorably impact on the trajectory of the disorder itself. 23–25
Reduced duration and insufficient sleep, are important to the emergence of mood dysregulation characteristic of bipolar disorders. Reduced sleep need is a unique and highly prevalent feature of mania,Reference Harvey 26 , Reference Lewis, Gordon-Smith and Forty 27 experimental sleep deprivation can trigger mania in subjects with bipolar disorder and sleep loss predicts subsequent manic symptoms.Reference Bauer, Grof, Rasgon, Bschor, Glenn and Whybrow 28 Links between reduced sleep duration/insufficient sleep and mood swings, impulsive and aggressive behaviors have also been observed in community samples and across affective disorders.Reference Clinkinbeard, Simi, Evans and Anderson 29 , Reference Sivertsen, Harvey, Lundervold and Hysing 30
Compelling evidence has also suggested that mood disorders are also frequently associated with a malfunction of the circadian system that might play a pathogenetic and pathoplastic role (for an overview seeReference Geoffroy 7 , Reference Harvey 26 , Reference McClung 31 , Reference Logan and McClung 32 ).
According to the “circadian hypothesis of mood disorders” a de-synchronization of the central pacemaker would be typical of these conditions.Reference Geoffroy 7 , Reference Harvey 26 , Reference McClung 31 , Reference Logan and McClung 32 It has been shown that the majority of individuals with mood disorder show alterations in the circadian rhythmicity, with abnormalities in physiological and behavioral timekeeping processes across the 24 h including sleep/wake patterns, prior to and during the depressive or manic episodes, and even during euthymia.Reference Geoffroy 7 , Reference Kanady, Soehnera and Harvey 9 , Reference Geoffroy, Scott and Boudebesse 11 , Reference Palagini, Miniati and Caruso 17 , Reference Harvey 26 , Reference Caruso, Meyrel and Krane-Gartiser 33 , Reference Wirz-Justice and Benedetti 34 Biological rhythm’s dysregulation, has been associated with the severity of mood and insomnia symptoms, cognitive dysfunctions, emotional dysregulation, and suicidality in bipolar disorder.Reference Geoffroy 7 , Reference Kanady, Soehnera and Harvey 9 , Reference Geoffroy, Scott and Boudebesse 11 , Reference Palagini, Miniati and Caruso 17 , Reference Harvey 26 , 33–36 Similarly to insomnia treatments, chronotherapeutic interventions may favorably impact on the trajectory of mood disorders. 34–38
Several potential mechanisms, through which these conditions of disrupted sleep, might increase the risk or perpetuation of mood disorders, have been hypothesized. They include alterations in monoamine transmission, in stress and inflammatory systems, in hippocampal neurogenesis and neuropeptide signaling even during neurodevelopment (for an overview seeReference Geoffroy 7 , Reference Harvey 26 , Reference McClung 31 , Reference Logan and McClung 32 , Reference Wirz-Justice and Benedetti 34 , Reference Palagini, Bastien, Marazziti, Ellis and Riemann 39 , Reference Palagini, Domschke, Benedetti, Foster, Wulff and Riemann 40 ).
During the last years as potential pathogenic factors involved in mood disorders neurodegeneration and neuroprogression have gained increased attention.Reference Kapczinski, Mwangi and Cassidy 41 , Reference Tang, Helmeste and Leonard 42 Neurodegeneration is characterized by mild changes in the motor, sensory, or cognitive neuronal populations that, over time, become chronic, affecting the neuronal functions. Neuroprogression refers to the pathological reorganization of the central nervous system (CNS), include mild degenerative processes and decreased plasticity. 41–43 These neurodegenerative and neuroprogressive mechanisms involve multiple and complex interactions among the CNS the endocrine and immune systems which includes inflammatory processes, oxidative stress, accumulation of neurotoxic proteins, and neuroprotection deficit that by leading to failed or incorrect reorganization in neuroplasticity would be related to recurrent and chronic trajectories of mood disorders. 41–43 Furthermore, a neuroplastic adaptation to pathology framework has been proposed emphasizing substantial plasticity and remodeling that may occur with the longitudinal evolution of mood disorders, beyond the classic neuroprogressive framework, which is characterized by loss of grey matter volume, decreased brain connectivity, and chronic inflammation.Reference Amad, Expert and Lord 44
Since the recent hypothesis of a link between insomnia, sleep loss, inappropriate circadian phasing of sleep, and neurodegenerative/neuroprogressive processes in neurodegenerative disorders 45–48 it might be possible that sleep disturbances could contribute to neurodegeneration and neuroprogression in mood disorders.
Therefore, the aim of this paper was to review the evidence of how these sleep disturbances, might lead to neurodegenerative/neuroprogressive processes in mood disorders in order to propose a novel and unified model for the pathophysiology and evolution of mood disorders.
Method
Materials and methods
We conducted a systematic review according to the preferred reporting items for systematic reviews and meta-analysis (PRISMA) guidelines.Reference Moher, Liberati and Tetzlaff 49
Objectives
The main aim of this paper was to systematically review findings from clinical and experimental works or review articles on the association among insomnia, conditions of sleep loss including short sleep duration, chronic sleep deprivation, sleep restriction, insufficient sleep, and such conditions experimentally induced, circadian sleep alterations, and neurodegenerative/neuroprogressive processes in mood disorders. We set out to systematically review the published literature on the topic in accordance with the PICOS process as follows: P—population: adult female and male patients with insomnia, short sleep duration, chronic sleep deprivation, sleep restriction, insufficient sleep, circadian sleep alterations, we included studies conducted in animals—I-intervention: not applicable, C—comparison: patients with insomnia, short sleep duration, chronic sleep deprivation, sleep restriction, insufficient sleep, circadian sleep alterations vs patients without these disorders; O—outcome: index of neuroinflammation, of activation of the stress system, of oxidative stress, of accumulation of neurotoxic proteins of neuroprotection deficit in relation with these sleep disturbances in mood disorders; S—study design: we included randomized controlled trials, cohort studies, case-control studies, follow-up studies, pilot studies, experimental studies, case series, or case reports.
Protocol and registration
This systematic review is not included in a research protocol. Eligibility Criteria: all studies published between 1990 and January 2020 using PubMed, PsycINFO, and Embase electronic databases were included, provided that they met the following criteria: (1) written in English; (2) original articles on studies with a longitudinal design; and (3) prospective or retrospective, observational (analytical or descriptive), experimental, controlled or non-controlled studies conducted in animals and humans. Reviews and non-original articles (ie, case reports, editorials, Letters to the Editor, and book chapters) were also included.
Information source and search strategy
The literature search was designed and performed independently in duplicate by two authors (LP, MM). The PubMed database was systematically screened using the following terms: [Neuroinflammation] OR [Activation of the stress system] OR [Oxidative stress] OR [Accumulation of neurotoxic proteins] OR [Neuroprotection deficit] AND [Insomnia or sleep deprivation or sleep restriction or sleep loss or short sleep duration or insufficient sleep or circadian sleep disorders] AND [Mood Disorders] leading to a total of 97 papers. Records after duplicates removed were 87 (Table 1).
Table 1. PRISMA Search Flow Diagram

Study selection
Two authors independently screened the resulting articles for their methodology and appropriateness for inclusion. Consensus discussion was used to resolve disagreements between reviewers.
Data collection process and data items
First, the title and abstract of each paper were assessed by two independent authors for language suitability and subject matter relevance, and the studies thereby selected were assessed for their appropriateness for inclusion and quality of method.
Data Synthesis
Due to the lack of homogeneity among the resulting studies, a meta-analysis could not be performed. Hence, this systematic review was presented as a narrative synthesis.
Limitations
Due to the lack of homogeneity among studies and to the fact that most of the studies were conducted in pre-clinical settings this systematic review was presented as a narrative synthesis with theoretical considerations.
Results
The systematic literature search retained 70 articles that were collectively selected and analyzed as follows: neuroinflammation (n = 16 articles), activation of the stress system (n = 17), oxidative stress (n = 8), accumulation of neurotoxic protein (n = 10), and neuroprotection deficit (n = 19) (Table 1).
It is widely accepted that insomnia, chronic sleep loss, and circadian rhythm abnormalities are considered risk factor for neurodegenerative disorders (for and overview see 45–48 , Reference Minakawa, Wada and Nagai 50 ). Although clinical data are reviewed elsewhere 45–48 , Reference Minakawa, Wada and Nagai 50 , Reference Cordone, Annarumma, Rossini and De Gennaro 51 it is of importance to underlie that sleep disturbances, including short or long sleep duration, poor sleep quality, circadian rhythm abnormalities, and insomnia are associated with a significant increase in the risk ratio for cognitive impairment and preclinical Alzheimer Diseases. 45–48 , Reference Minakawa, Wada and Nagai 50 , Reference Cordone, Annarumma, Rossini and De Gennaro 51
A consistent literature demonstrated the critical tie between sleep, synaptic function, and cognition. 52–54 Sleep is regulated by complex physiological processes where circadian mechanisms and homeostatic sleep processes are mutually linkedReference Borbély, Daan, Wirz-Justice and Deboer 55 and beyond, sleep itself serves important regulatory functions for CNS connectivity and plasticity. 52–54 Sleep plays a major role in restoring brain energy, in off-line processing of information acquired during wake, in the neuronal plasticity that underlies learning and memory consolidation.Reference Zhao, Zhao and Veasey 47 , Reference Cirelli and Tononi 53 , Reference Irwin and Cole 56 Sleep regulates the bulk removal of proteins and other molecules from the brain through regulation of “glymphatic” flow, a recently-described phenomenon whereby astrocytes facilitate extracellular fluid transit through the brain and accelerated clearance of exogenously-added β-amyloid peptide (Aβ) from the brain. 45–48 , Reference Minakawa, Wada and Nagai 50 , Reference Cordone, Annarumma, Rossini and De Gennaro 51 Melatonin and circadian rhythms regulation contribute to the clearance of neurotoxic products and melatonin exerts important antifibrillogenic and antioxidant activity.Reference Cardinali 57 A reciprocal interaction between homeostatic regulation of sleep and inflammatory mediators has been widely described.Reference Irwin and Cole 56 , 58–61 Sleep and immunity are bidirectionally linked: immune system activation alters sleep, and sleep in turn affects the innate and adaptive arms of our immune system.Reference Irwin and Cole 56 , 58–61 Sleep has also an important bidirectional link with the stress system across the lifetime.Reference Lo Martire, Caruso, Palagini, Zoccoli and Bastianini 62 Sleep represents a state with an increased antioxidant activity which promotes a brain protection against free radicals via a diminution in oxidant production (for an overview seeReference Villafuerte, Miguel-Puga and Rodríguez 63 ).
Accordingly, sleep disturbances can directly impair synaptic homeostasis leading to neuronal loss and damage.Reference Musiek and Holtzman 46 , 48–52 , Reference Van Someren, Cirelli and Dijk 64 , Reference Owen and Veasey 65
Sleep deprivation or fragmentation resulting from insomnia, conditions of sleep loss, or circadian clock dysfunctions have been hypothesized to causing increased neuronal activity, leading to elevated Aβ production and aggregation. Increased time spent awake increases sympathetic out hence suppressing glymphatic system function and decreasing clearance of pathogenic proteins including Aβ.Reference Musiek and Holtzman 46 , Reference Shamim, Warriach, Tariq, Rana and Malik 48 , Reference Minakawa, Wada and Nagai 50 , Reference Cordone, Annarumma, Rossini and De Gennaro 51 , Reference Besedovsky, Lange and Haack 61 , Reference Van Someren, Cirelli and Dijk 64 , Reference Owen and Veasey 65 Sleep disturbances might contribute to alterations in autophagy and promote loss of synaptic homeostasis, neuroinflammation, chronic activation of the stress system, and oxidative stress.Reference Musiek and Holtzman 46 , Reference Shamim, Warriach, Tariq, Rana and Malik 48 , Reference Minakawa, Wada and Nagai 50 , Reference Cordone, Annarumma, Rossini and De Gennaro 51 , Reference Besedovsky, Lange and Haack 61 , 64–66 In addition disturbed sleep may impair neuroprotection by downregulating the kynurenine pathway, the melatonin production and by altering the neurotrophin brain-derived neurotrophic factor (BDNF) production.Reference Musiek and Holtzman 46 , Reference Van Someren, Cirelli and Dijk 64 These combinations of insults may promote neurodegeneration and neuroprogression, which in turn may cause more circadian and sleep dysfunctions within the framework of a reciprocal interaction (Figure 1).

Figure 1. A proposed model: role of insomnia, sleep loss, and circadian sleep alterations in neurodegeneration and neuroprogression of mood disorders.
The evidence outlining the role of sleep disturbances in each key pathway involved in neurodegenerative and neuroprogressive processes in mood disorders will be summarized. The role of sleep disturbances will be reviewed in (1) neuroinflammation (2) activation of the stress system and (3) oxidative-stress, (4) the accumulation of β-amyloid peptide and in (5) deficit of neuroprotection related to alterations in BDNF production, in kynurenine and melatonin pathways. The following results sections will thus analyze these findings.
Sleep disturbances and neuroinflammation
A reciprocal interaction of inflammatory mediators and the homeostatic regulation of sleep has been widely described.Reference Irwin and Cole 56 , 58–61 In particular, chronic sleep deprivation poor sleep quality and insomnia, may lead to an over-activation of the Hypothalamic–Pituitary–Adrenal (HPA)-axis and sympathetic nervous system pathways which together contribute to an increased pro-inflammatory cytokine activity.Reference Irwin and Cole 56 , 58–61 Insomnia is associated with alterations in the relative distribution of immune cells, marked decreases in the numbers of T-cells, and higher levels of C-Reactive Protein (CRP).Reference Irwin, Olmstead and Carroll 67 , Reference Irwin and Piber 68 Individuals with poor sleep quality and short sleep durations have shown higher levels of both IL-6 and TNF.Reference Irwin, Olmstead and Carroll 67 , Reference Irwin and Piber 68
These findings are also supported by experimental sleep deprivation studies in humans. Available data showed that both prolonged partial and total night sleep deprivation increases CRP and IL-6, and even short periods of sleep restriction increases in TNF in men, and IL-1β, IL-6, and IL-17 in both sex.Reference Irwin, Olmstead and Carroll 67 , Reference Irwin and Piber 68 On this basis, a role for insomnia and prolonged sleep deprivation or conditions of sleep loss has been hypothesized in the initiation and maintenance of mood disorders by establishing a state of chronic activation of the inflammatory system.Reference Irwin, Olmstead and Carroll 67 , Reference Irwin and Piber 68 In animal models it has been shown that chronic sleep restriction or deprivation by altering the immune signaling milieu may trigger changes in microglial function in turn leading to morphologic microglial activation and astrocytic phagocytosis in the brain.Reference Wadhwa, Prabhakar and Ray 69 , Reference Bellesi, de Vivo, Chini, Gilli, Tononi and Cirelli 70 Indeed, it has been shown that both chronic sleep restriction and deprivation may promote microglial activation and their phagocytic activity, even in the absence of signs of neuroinflammation. Because low-level sustained microglia activation can lead to abnormal responses to a secondary insult, these results have suggested that chronic sleep loss, like many other stressors, through microglia priming, may predispose the brain to further damage. 69–71 Hence sleep deprivation induces not only elevated inflammatory cytokine levels in the hippocampus, but also gliosis, and morphological changes of microglia and astrocytes.Reference Madore, Yin, Leibowitz and Microglia 72 Microglia-astrocytes mediated synaptic pruning might be exacerbated directly by sleep deprivation, which might contribute to aberrantly prune, early synaptic loss, and neurodegeneration in mood disorders (for an overview seeReference Madore, Yin, Leibowitz and Microglia 72 ).
The role of circadian sleep alterations in causing neuroinflammation in mood disorders has been widely described in mood and neurodegenerative disorders (for an overview seeReference Wirz-Justice and Benedetti 34 , Reference Musiek and Holtzman 46 ). Microglia and astrocytes have been shown to possess a circadian clock that influences inflammatory response. It has been hypothesized that microglial inflammatory responses can be tightly controlled by the circadian clock and, if altered, might predispose individuals to neurodegeneration and neuroprogression (for an overview seeReference Wirz-Justice and Benedetti 34 , Reference Madore, Yin, Leibowitz and Microglia 72 ). As such, disturbed sleep may contribute to mood disorders through dysregulation of the inflammatory system inducing neuroinflammation and a deficit in neuroprotection via feed-forward cascade on HPA-axis dysregulation.Reference Palagini, Bastien, Marazziti, Ellis and Riemann 39
In conclusion, it may be hypothesized that sleep disturbances may directly contribute to neuroinflammation by increasing pro-inflammatory cytokine, and indirectly by activating microglia with aberrantly pruning and by altering astrocyte-neuron signaling hence favoring glutamate-mediated excitotoxicity and neuronal damage. These processes may result in a positive feed-forward loop which increases inflammation and therefore neurodegeneration and neuroprogression (Figure 1).
Sleep disturbances and activation of the stress system
It has been widely shown that individuals suffering from insomnia display hyperactivation of the HPA-axis at both brain and peripheral levels (for review see.Reference Riemann, Spiegelhalder and Feige 73 , Reference Riemann, Nissen, Palagini, Otte, Perlis and Spiegelhalder 74 ). Increases in norepinephrine, epinephrine, and other markers of sympathetic outflow have been related to cognitive and emotional arousal and somatic hyperarousal in individuals suffering from insomnia; the hyperarousal is the key pathophysiological mechanism of insomnia.Reference Riemann, Spiegelhalder and Feige 73 , Reference Riemann, Nissen, Palagini, Otte, Perlis and Spiegelhalder 74 Changes observed in brain structures in these patients include a reduction in the volume of the prefrontal cortex, caudate head, and hippocampus, as well as an increase in the amygdala volume,Reference Riemann, Nissen, Palagini, Otte, Perlis and Spiegelhalder 74 modifications resembling those described in mood disorders. Given these similarities, it has been hypothesized that insomnia may impact mood disorder throughout the activation of the stress system and of its negative consequences on the brain including reduction in hippocampal and medial prefrontal cortex neurogenesis, synaptic plasticity.Reference Lo Martire, Caruso, Palagini, Zoccoli and Bastianini 62 , Reference McEwen and Karatsoreos 75 , Reference Meerlo, Havekes and Steiger 76 These factors may favor neuron loss and death causing neural injury, neuroinflammation, changes in myelin, and protein aggregation.Reference Owen and Veasey 65 Recent research has shown that sleep disruption should be considered as a neurobiological and physiological stressor with consequences that impair brain functions and contribute to the cumulative wear and tear on body systems (for an overview seeReference Lo Martire, Caruso, Palagini, Zoccoli and Bastianini 62 , Reference McEwen and Karatsoreos 75 , Reference Meerlo, Havekes and Steiger 76 ). In particular, chronic sleep loss would lead to irreversible neurodegeneration (for an overview seeReference Owen and Veasey 65 ). Experimental studies have also reported that chronic sleep restriction or disruption may gradually induce neurobiological changes very similar to those observed in depressed patients. In particular, when restricted sleep occurs chronically, it might cause a reduction of hippocampal and medial prefrontal cortex cell proliferation and neurogenesis, which may eventually lead to neural loss and death with reduction in these brain structure volume.Reference Owen and Veasey 65 The HPA axis receives a strong circadian input from the Suprachiasmatic nucleus (SCN) so that circadian sleep alterations have been considered as stressors since they directly alter catecholamine and cortisol release (for an overview seeReference McEwen and Karatsoreos 75 ). The association between altered circadian rhythms and activation of the stress system has been widely describedReference McEwen and Karatsoreos 75 and related to neurodegeneration caused by circadian dysfunctions.Reference Musiek and Holtzman 46 , Reference Madore, Yin, Leibowitz and Microglia 72
In summary, insomnia, sleep loss, or circadian sleep disruption may act as a neurobiological stressor which, by over-activating the stress system, may negatively influence neural plasticity causing neuron loss, death, injury, and pathological neural reorganization.Reference Besedovsky, Lange and Haack 61 , Reference McEwen and Karatsoreos 75 As such, disturbed sleep may contribute to neuroinflammation and to a reduction in neuroprotection through HPA-axis dysregulation (for a review seeReference Palagini, Bastien, Marazziti, Ellis and Riemann 39 ) hence favoring neurodegeneration and neuroprogression (Figure 1).
Sleep disturbances and activation of oxidative stress
The wakefulness involves a high neuronal metabolism to maintain neuronal electrical potentials, which requires a great amount of oxygen, resulting in a significant production of oxidants. The Reactive Oxygen Species (ROS) and other oxidative stress markers could be accumulated in the brain tissue during wakefulness.Reference Villafuerte, Miguel-Puga and Rodríguez 63 Thus, sleep represents a state with an increased antioxidant activity which promotes a brain protection against free radicals via a decrease in oxidant production (for an overview seeReference Besedovsky, Lange and Haack 61 ). Sleep has been hypothesized to be a dynamic-resting state with antioxidative properties.Reference Besedovsky, Lange and Haack 61
Some studies conducted in subjects with insomnia reported an elevation in certain oxidative stress biomarkers,Reference Gulec, Ozkol and Selvi 77 , Reference Belcaro, Saggino and Cornelli 78 and been shown in healthy humans after sleep deprivation.Reference Trivedi, Holger, Bui, Craddock and Tartar 79
Data in humans are supported by experimental studies conducted in animals. A recent systematic review conducted on 44 studies has shown the elevation of ROS levels in animals after sleep deprivation (for an overview seeReference Besedovsky, Lange and Haack 61 ).
Since ROS cells exhibit circadian oscillations in animal studies, it has been hypothesized that a disruption of the circadian system cause has been supposed to cause increased oxidative stress. As ROS are produced as byproducts of increased neuronal activity in the brain, the circadian clock may serve to temporally coordinate the expression of redox defense genes. Hence circadian sleep disruption has been related to neurodegeneration in neurodegenerative disorders via alteration in ROS homeostasis (for a review seeReference Musiek and Holtzman 46 , Reference Madore, Yin, Leibowitz and Microglia 72 ).
A recent study conducted in 52 females with bipolar disorder and 30 healthy controls has shown that alterations in circadian rhythms were related to alterations in redox metabolism in subjects with bipolar disorder. Authors hypothesized that disturbance of sleep and daily rhythms experienced in Bipolar Disorder may result in decreased antioxidant defence.Reference Cudney, Sassi and Behr 80 The disruption of normal circadian function in mood disorders might render the brain more vulnerable to oxidative injury and thereby promotes neurodegeneration and neuroprogression. It is thus plausible that these sleep disturbances may contribute to neurodegeneration and neuroprogression by contributing to alterations in ROS homeostasis (Figure 1).
Sleep disturbances and accumulation of neurotoxic proteins
Sleep regulates the bulk removal of proteins and other molecules from the brain through regulation of “glymphatic” flow.Reference Musiek and Holtzman 46 , Reference Shamim, Warriach, Tariq, Rana and Malik 48 , Reference Minakawa, Wada and Nagai 50 , Reference Cordone, Annarumma, Rossini and De Gennaro 51 , Reference Besedovsky, Lange and Haack 61 , Reference Van Someren, Cirelli and Dijk 64 , Reference Owen and Veasey 65 and has been supposed to enhance brain waste drainage.Reference Xie, Kang and Xu 81
Alterations in glymphatic system’s functioning are possibly involved in Aβ peptides and tau oligomers accumulation in neurodegenerative disorders. Imaging studies conducted in healthy humans, revealed associations between self-reports of less sleep duration or poor sleep quality and higher Aβ burden in the brain. 82–84
Recently, Chen et alReference Chen, Wang, Zhang, Wang and Gao 85 assessed the cerebrospinal fluid Aβ levels in 23 patients with chronic insomnia, to reveal the potential effects of chronic sleep lack on the pathogenesis of Alzheimer’s Disease. Authors found that CSF Aβ42 levels were significantly increased in insomniac patients, and Aβ levels significantly correlated with the poor sleep quality. The effect of a night of sleep deprivation was investigated in 20 healthy controls by using PET and 18F-florbetaben to measure brain Aβ.Reference Shokri-Kojori, Wang and Wiers 86 The results showed that a significant increase in Aβ burden in the right hippocampus and thalamus was associated with mood worsening but was unrelated to the genetic risk for Alzheimer’s Disease. Additionally, baseline Aβ burden in a range of subcortical regions and the precuneus was inversely associated with reported night sleep hours. Data deriving from animal studies using sleep deprivation and restriction supported the data collected in humans showing a relationship between sleep deprivation/restriction and increased in Aβ levels (for an overview seeReference Cordone, Annarumma, Rossini and De Gennaro 51 ). Several reviews published in the last years hypothesized a relationship between circadian rhythm alterations and Aβ accumulation in neurodegenerative diseases.Reference Musiek and Holtzman 46 , Reference Leng, Musiek, Hu, Cappuccio and Yaffe 87 Proteasomal degradation of proteins displays circadian oscillation and proteasome function is required for normal circadian clock timing (for an overview see van Oijen et alReference van Ooijen, Dixon, Troein and Millar 88 ). Since a circadian oscillation of autophagy markers has been described, it has been proposed that the circadian clock may regulate autophagy activities and consequently proteasomal degradation.Reference Musiek and Holtzman 46 , Reference Leng, Musiek, Hu, Cappuccio and Yaffe 87 Alterations in circadian rhythms might favor neurodegeneration by directly impairing proteasomal degradation and by altering autophagy activities, and, as such, they might contribute to the accumulation of neurotoxic agents and to the impairment of melatonin production and antioxidant defense, hence contributing to alter ROS homeostasis.
In conclusion, insomnia, reduced sleep duration, sleep deprivation, and circadian sleep alterations might contribute to the accumulation of neurotoxic proteins which have been involved in neurodegenerative processes by: (1) directly impairing proteasomal degradation, (2) altering autophagy activities, (3) impairing the removal of proteins and other molecules from the brain through regulation of “glymphatic” flow, and (4) impairing melatonin production and antioxidant defense. It is tempting to speculate that these sleep disturbances might lead to neurodegeneration in mood disorders via the impairment of neurotoxic proteins pathways of clearance and degradation hence contributing to their aggregation (Figure 1).
Sleep disturbances and neuroprotection deficit
Reduced neurotrophin BDNF
BDNF is involved in the homeostatic regulation of sleep.Reference Faraguna, Vyazovskiy, Nelson, Tononi and Cirelli 89 Decreased serum BDNF levels have been found in individuals with insomnia symptoms. 90–93 Individuals with short sleep duration have reported reduced serum BDNF levels.Reference Fan, Chen and Shi 92 , Reference Furihata, Saitoh and Otsuki 93 These findings, obtained from clinical samples, are supported by animal data showing reduced BDNF levels in prolonged sleep deprivation.Reference Meerlo, Havekes and Steiger 76 , Reference Schmitt, Holsboer-Trachsler and Eckert 94 Some authors hypothesized a mediating role of disturbed sleep in the link between stress experience and serum BDNF levels in insomnia.Reference Giese, Unternaehrer and Brand 90 , Reference Giese, Unternährer and Hüttig 91 Disturbed sleep would act as a neurobiological stressor throughout the activation of the stress system thus impairing BDNF levels with a negative effect on serotonin signaling, on synaptic plasticity, and neurogenesis (for an overview see Meerlo et alReference Meerlo, Havekes and Steiger 76 ).
A rhythmic regulation of BDNF and BDNF-mediated signaling might play an important role in the circadian regulation of SCN pacemaker sensitivity to light.Reference Katoh-Semba, Tsuzuki and Miyazaki 95 A disturbance of the light-induced phase shift is known to induce neuronal degeneration in both human and animal brains, as it is evident by the atrophy of the temporal cortex resulting from chronic jet leg.Reference Schaaf, Duurland, de Kloet and Vreugdenhil 96 This neuronal degeneration has been related to alterations in BDNF which appear to be involved in the regulation of diurnal rhythms in a variety of biological processes and vice versa.Reference Katoh-Semba, Tsuzuki and Miyazaki 95 , Reference Schaaf, Duurland, de Kloet and Vreugdenhil 96 Taken together these evidences might suggest that sleep and circadian rhythms alterations might impair BDNF production with a decrease of neurotrophic support, leading to neuronal atrophy, decreased hippocampal neurogenesis, and loss of glia (Figure 1).
Neurotoxic shift of kynurenine pathway
A recent study conducted in patients with bipolar disorder and healthy controls showed that individuals with sleep disturbances had significantly higher biomarkers of the kynurenine pathway’s neurotoxic branch than healthy controls. Poor sleep quality was associated with an activation of kynurenine pathway, alterations in tryptophan metabolism, and mood symptoms severity.Reference Mukherjee, Krishnamurthy and Millett 97
Again in 68 currently depressed, 26 previously depressed, and 66 never depressed subjects, sleep disturbances resulted related to the neurotoxic shift in the balance of kynurenine metabolites with decreases in the serum concentration of kynurenic acid and an increase in quinolinic acid in the first group.Reference Cho, Savitz, Dantzer, Teague, Drevets and Irwin 98
These data collected in humans were supported from findings collected from translational research showing that sleep deprivation and loss were related to alterations in the tryptophan metabolism degradation and kynurenine pathway.Reference Baratta, Buck, Buchla, Fabian, Chen and Mong 99 Since circadian rhythmicity of tryptophan metabolism via the kynurenine pathway has been demonstrated in humans,Reference Rapoport and Beisel 100 it is possible that circadian sleep alterations might influence tryptophan metabolism and shift the tryptophan toward the kynurenine pathway with a consequent decrease of 5HT levels and increase in quinolinic acid.
Diminished or misaligned melatonin rhythms
Diminished or misaligned melatonin rhythms have been shown to characterize not only circadian sleep disorders,Reference Cardinali 57 , Reference Zisapel 101 but also insomnia.Reference Zisapel 101 , Reference Riemann, Klein and Rodenbeck 102 The role of melatonin alterations and related circadian problems have been already pointed out in the context of neurodegenerative disorders including mood disorders (for and overview seeReference Logan and McClung 32 , Reference Musiek and Holtzman 46 , Reference Cardinali 57 , 101–103 ). We would hypothesize that both insomnia and circadian sleep alterations may contribute to neurodegenerative processes by altering melatonin rhythms and levels hence impairing its neuroprotective functions (Figure 1).
Discussion and Therapeutical Implications
Increasing evidence suggests that neurodegenerative processes, reflected in neuronal and glial cell atrophy or loss, and neuroprogression such as the pathological reorganization of the CNS might be factors involved in the pathophysiology and evolution of mood disorders.
Sleep disturbances may directly contribute to neuroinflammation by increasing pro-inflammatory cytokine, and indirectly by activating microglia with aberrantly pruning and by altering astrocyte-neuron signaling hence favoring glutamate-mediated excitotoxicity and neuronal damage. These processes may result in a positive feed-forward loop which increases inflammation and therefore neurodegeneration and neuroprogression. Insomnia, sleep loss, or circadian sleep disruption may act as a neurobiological stressor which, by over-activating the stress system, may negatively influence neural plasticity causing neuron loss, neural death, and neural injury. As such, disturbed sleep may contribute to neuroinflammation and to a neuroprotection deficit. In addition, insomnia, sleep loss, or circadian sleep disruption may favor the accumulation of neurotoxic proteins, the activation of ROS and may establish a state of neuroprotection deficit. Reduced BDNF, the tryptophan shift, diminished, or misaligned melatonin rhythms have been related to these sleep disturbances and may hence contribute to neurodegeneration/neuroprogression (Table 2).
Table 2. Consequences of Disturbed Sleep on Key Pathways Involved in Neurodegenerative and Neuroprogressive Processes: Summary of the Findings Regarding Neuroinflammation, Activation of the Stress System, Activation of Oxidative-Stress, the Accumulation of β-Amyloid Peptide, Deficit of Neuroprotection
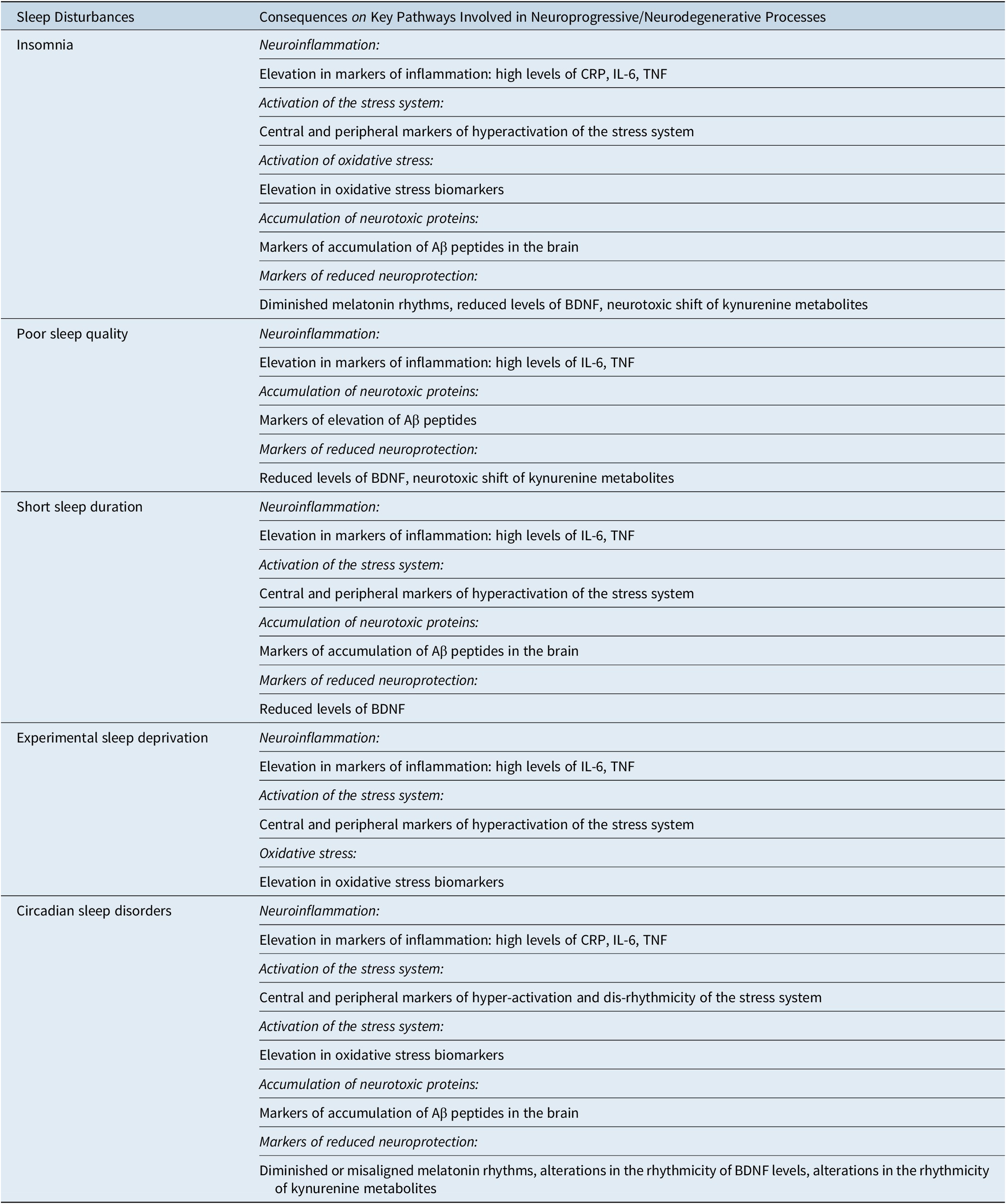
Abbreviations: Aβ, β-amyloid peptide; BDNF, neurotrophin brain-derived neurotrophic factor; CRP, C-reactive protein; (IL)-6, interleukin-6; TNF-α, tumor necrosis factor.
Hence, we propose a model in which sleep plays a key role in the regulation of those multiple systems involved in neurodegeneration and neuroprogression: the combination of insults may favor mood disorders (Figure 1). Evaluating and targeting sleep disturbances in mood disorders should be of importance and may have a neuroprotective role. A neuroprotective role has already been hypothesized for the treatment of insomnia and circadian sleep alterations in the context of neurodegenerative diseases.Reference Minakawa, Wada and Nagai 50 The evaluation of sleep disturbances, and namely of insomnia in subjects with mood disorders should be included in their clinical evaluation for its potential therapeutic implications. It has already been postulated, the treatment of sleep disturbances in mood disorder may improve the trajectory of the disorder.Reference Asarnow and Manber 25 , Reference Harvey 26 Taken together these considerations suggest that by targeting insomnia according to guidelines of insomnia treatment 104–106 we might interrupt processes of neurodegeneration in mood disorders. It is possible that by treating insomnia with current options which include Cognitive-Behavioral Therapy of Insomnia—CBT-I and melatonin 2 mg Prolonged Release supplementationReference Geoffroy, Micoulaud Franchi, Lopez and Schroder 37 , 104–108 we may intervene on the neuroprogressive processes of affective disorders. Indeed, considering the plasticity of the brain, a bidirectional nature of neuroprogression also exist and substantial plasticity and remodeling may also occur after treatments.Reference Amad, Expert and Lord 44
Similarly, it is plausible that circadian-oriented interventions targeting the circadian clock might mitigate symptoms, or perhaps even slower the course of the disease itself.Reference Cardinali 57 , Reference Zisapel 101 Melatonin supplementation and light therapy are two principal methods of synchronizing the circadian clock and they have been extensively studied in aging and Alzheimer’s.Reference Cardinali 57 , Reference Zisapel 101 , Reference Hardeland 109 Melatonin supplementation has been shown to be effective in arresting neurodegenerative phenomena observed in experimental models of neurodegenerative diseases.Reference Cardinali 57 , Reference Zisapel 101 , Reference Hardeland 109 , Reference Hardeland 110 It has already been suggested that chronobiology may help provide preventive strategies and/or improve the treatment of mood disorders.Reference Wirz-Justice and Benedetti 34 , Reference Geoffroy, Micoulaud Franchi, Lopez and Schroder 37 , Reference Gottlieb, Benedetti and Geoffroy 38 It is tempting to speculate that the re-synchronization of chronobiological rhythms may also have a neuroprotective value in mood disorders.Reference Brown, McIntyre, Rosenblat and Hardeland 103 , Reference Hardeland 109 , Reference Hardeland 110
In this framework is also plausible that lithium as psychotropic agent for mood disorders, with chronobiotic and neuroprotective properties, may contribute to prevent or mitigate neuroprogression/neurodegeneration in mood disorders by acting on both sleep and mood disturbances.Reference Moreira and Geoffroy 111 , Reference Palagini, Cipollone and Moretto 112
Sleep plays a key role in the regulation of those multiple systems involved in the pathogenesis of neurodegeneration and neuroprogression.
Particularly sleep disruption due to insomnia or to sleep restriction/deprivation/loss or circadian sleep alterations seems to contribute to neurodegeneration and neuroprogression in mood disorders throughout different forms of insult (1) Sleep disturbances may directly contribute to neuroinflammation by increasing pro-inflammatory cytokine, and indirectly by activating microglia with aberrantly pruning and by altering astrocyte-neuron signaling hence favoring glutamate-mediated excitotoxicity and neuronal damage. These processes may result in a positive feed-forward loop which increases inflammation, (2) insomnia, sleep loss, or circadian sleep disruption may act as neurobiological stressors which, by over-activating the stress system, may negatively influence neural plasticity causing neuronal loss, death, injury, and pathological neural reorganization. As such disturbed sleep may contribute to neuroinflammation and a neuroprotection deficit via feed-forward cascade on HPA-axis dysregulation, (3) insomnia, sleep loss, or circadian sleep disruption may favor the accumulation of neurotoxic proteins by: directly impairing proteasomal degradation, altering autophagy activities, impairing the removal of proteins and other molecules from the brain through regulation of “glymphatic” flow and by impairing melatonin production and antioxidant defense, (4) sleep disturbances related to insomnia, sleep loss, and circadian sleep alteration may favor elevated levels of ROS and oxidative stress biomarkers, (5) sleep disturbances may contribute to a deficit in neuroprotection. Reduced BDNF, a neurotoxic shift of kynurenine pathway, diminished or misaligned melatonin rhythms have been related to these sleep disturbances and may contribute to neurodegeneration and neuroprogression in mood disorders.
Conclusions
Sleep disturbances may act as a causal and worsening factor in mood disorders, by particularly favoring neurodegenerative, neuroprogression, and neuroplastic adaptation (Table 2 and Figure 1). Therefore to assess and target sleep disturbances in clinical practice should be a priority in order to prevent and treat mood disorders. Further studies are highly expected examining the trajectories of both sleep and mood disorders across the lifespan. Future research would benefit from the study of neuroprogressive/neurodegenerative processes related to insomnia and circadian sleep disturbances in mood disorders and from the study of potential neuroprotective value of their treatment in mood disorders.
Clinical implication points
-
(1) Sleep disturbances may favor neurodegenerative and neuroprogressive processes in mood disorders.
-
(2) Insomnia and circadian sleep disorders may contribute to neuroinflammation, to the activation of the stress system, to oxidative stress, accumulation of neurotoxic proteins, and to neuroprotection deficit.
-
(3) Targeting sleep disturbances in particular insomnia and circadian sleep disorders in mood disorders may hold a neuroprotective value.
-
(4) Treating insomnia with current options which include Cognitive-Behavioral Therapy of Insomnia—CBT-I and melatonin supplementation we might intervene on the neuroprogressive processes of affective disorders.
-
(5) Circadian-oriented interventions such as melatonin supplementation and light therapy targeting the circadian clock might slower neuroprogressive processes of affective disorders.
Funding
This research received no specific grant from any funding agency, commercial, or not-for-profit sectors.
Disclosures
Laura Palagini, Pierre A Geoffroy, Mario Miniati, Giulio Perugi, Giovanni Biggio Donatella Marazziti, Dieter Riemann do not have any conflicts of interest to disclose.